ABSTRACT
The variability of camel hair fibers physical-mechanical properties across sampling body areas and phaneroptics continue to be relatively unexplored topics in scientific literature. With the aim to valorize camel hair fibers, the present investigation has examined, through discriminant canonical analysis, fiber properties of Canarian camel hair regarding the body region sampled by comparing them with Merino sheep wool, world’s most demanded textile fiber. Additionally, camel hair attribute differences depending on phaneroptics such as coat or eye color and particularities have been determined. Diameter at the base of the fiber is higher for adult camels and increases from the mid-region to the back of the animal. Besides, the highest variability in both staple length and mean diameter was found for camel shoulder and hump fibers. Camel tail dock was the region with the finest fibers but also the one accounting for the highest percentage of residual dirt. Coat color and particularities were unlikely to significantly (p < .05) affect fiber diameter and strength but did affect the brightness of final textile products. These results may help to tailor strategies within camel farming systems seeking the maximization of their productive outcomes, which is of utter relevance in endangered animal genetic resources.
摘要
在科学文献中,驼毛纤维物理力学性能在采样身体区域和显象镜中的可变性仍然是相对未探索的主题. 为了对驼毛纤维进行估价,本研究通过判别规范分析,通过将加那利群岛驼毛与世界上需求量最大的纺织纤维美利奴羊毛进行比较,检验了其身体部位的纤维特性. 此外,驼毛属性的差异取决于显色剂,如毛被或眼睛的颜色和特殊性. 成年骆驼纤维基部的直径更高,并且从动物的中部到背部都会增加. 此外,骆驼肩和驼峰纤维的短纤维长度和平均直径的变异性最高. 骆驼尾码头是纤维最细的地区,但也是残留污垢比例最高的地区. 涂层颜色和特殊性不太可能显著影响纤维直径和强度(P < .05),但会影响最终纺织品的亮度. 这些结果可能有助于在骆驼养殖系统中制定策略,寻求其生产成果的最大化,这与濒危动物遗传资源完全相关.
Introduction
Camel hair fiber remained a minor income source for breeders for decades which made them relatively disregarded from textile manufacturers (Kerven, Russel, and Laker Citation2002). Their recent specialized research recognition as a long, strong, soft and light-colored fiber (Sharma and Pant Citation2013) has considerably increased the commercial significance and interest on this exotic animal fiber (Swami and Babel Citation2023; Zarrin et al. Citation2020). In fact, applied researches on the use of different chemicals to further increase the output of high-grade camel hair products and meet the increasing social demand, have appeared on the scene during the last years (Mokhtar and Moussa Citation2019; Zhenrui et al. Citation2021). Future studies should explore the environmental and economic sustainability of such chemical treatments and their long-term impact on the overall quality of camel hair.
The industrial performance and end-uses of camel hair fiber, as in other fiber-producing species, extendedly depend on physical structure-related properties such as mean diameter and staple length. Despite these are the main determinants of the fineness and sensory tactile perception of fiber fabrics (Allain and Renieri Citation2010), other phenotypic traits of commercial interest include color, fleece type and body region sampled (Gerken et al. Citation2019).
This has been demonstrated in other fiber-producing animal species. In this regard, Bathrachalam et al. (Citation2019) widely illustrated the genetic basis of coat color in alpacas, which indeed was supported by Solano and Raggi (Citation2019) who provided some evidence for differences in fleece weight and physical properties among coat colors and body regions in the aforementioned species, the most important fiber-producing member of the New World Camelidae group (Wuliji et al. Citation2000). Similar conclusions are reported for vicuña fleece (Quispe et al. Citation2014), sheep wool (Fish, Mahar, and Crook Citation2002; Tabbaa, Al-Azzawi, and Campbell Citation2001) and goat cashmere (McGregor and Butler Citation2008; McGregor, Kerven, and Toigonbaev Citation2009; Taddeo et al. Citation2000).
Particularly focusing on the dromedary species, Harizi et al. (Citation2007) and Bhakat (Citation2019) discussed the effect of the factor sampling body area on some physical and mechanical properties of Tunisian and Indian dromedary camel hair, respectively. For double-humped camels, Hasi, Amu, and Zhang (Citation2020) and Sahani et al. (Citation2003) discuss the properties and applications of this animal species’ hair, and highlight significant variations in hair properties at different body sites, with the mid-side region fibers having the lowest mean diameter and the highest strength. Other topics, such as the role of coat color as a source of variation contributing to differential quality attributes in camel hair, continue to be relatively unexplored issues in the literature (Almathen et al. Citation2018).
In light of the aforementioned context, the description of the qualitative phenotypic characteristics affecting fiber yield and product value contributes to an advanced understanding of underlying pleiotropic effects. Hence, it will help at designing adapted genetic improvement programs and proposing strategies for optimizing camel hair production traits (Galbraith Citation2005; Purvis and Franklin Citation2005) as sustainable, driving agents for market opportunities for these animal fibers. Moreover, delving into the impact coat color on fiber properties can provide valuable insights into the potential diversity and market preferences for camel hair-made products.
For these reasons, the present study aims to further test and compare the physical-mechanical properties of Canarian camel hair (an endangered dromedary camel breed) and Merino sheep wool (the world’s most demanded textile fiber) concerning the body region sampled. The comparative analysis with Merino sheep wool, the epitome of textile demand, provides a unique lens to scrutinize and appreciate the top attributes of camel hair fibers. Additionally, we performed a comparative analysis to unravel the differences in camel hair attributes in relation to coat color, given the huge variability reported for this phaneroptic trait in the studied camel breed. The endeavor to decipher the impact of coat color on these fibers further elevates the study’s applications to inform local breeders and stakeholders on the adapted design of animal breeding programs and market opportunities for Canarian camel hair fibers, hence promoting the long-term, sustainable breeding and conservation of the endangered, unique European camel breed. Overall, the results of the present study provide further comparative insights into the uniqueness and market potential of regional camel breeds.
Material and methods
Animal sample
A total of 139 Canarian dromedaries (77 males and 62 females; aged between 3 months and 35 years old; ) were sampled for outer hair fibers (Hasi, Amu, and Zhang Citation2020) during the molting season, a six-to-eight-week period starting in late spring (Babu Citation2015). Six different samples were collected from each animal (shoulder, hump, belly, rump, tail dock and tail skirt) and classified in regard to the coat color phenotypes according to the breed standard () (Fernández de Sierra and Fabelo Marrero Citation2017).
Figure 1. Coat color phenotypes accepted within the Canarian camel breed standard (Fernández de Sierra and Fabelo Marrero Citation2017).
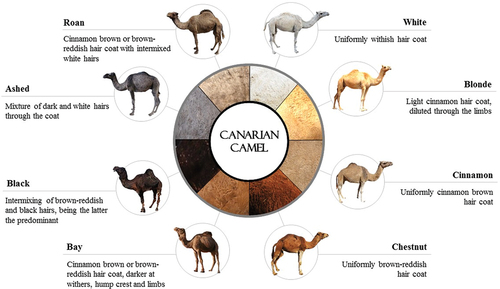
Table 1. Criteria for the classification of dromedary camel and sheep by age group.
Merino sheep wool from 395 animals (214 males and 181 females; aged between 3 months and 5 years old; ) was clipped with curved scissors for the regions of shoulder, belly and rump. For 55 out of 395 adult Merino sheep, wool samples from each body region were analyzed independently as well as a unique blended sample of them. The samples from the rest of the animals were analyzed once blended.
Sample pre-processing and physical-mechanical analysis
Prior to physical-mechanical analysis, raw hair samples were cleaned with a mix of solid surfactant (hexane) and hot water was used to remove impurities (vegetable matter, suint, and hair grease) (Allafi et al. Citation2022), and then dried at ambient temperature.
The analysis of the physico-mechanical properties of hair fibers were performed using an optical fiber diameter measurement tool (OFDA 2000, McLaughlin (Citation2000)). Hair attributes were measured and averaged for a minimum, representative number of 100 fibers for tail skirt and 1,500 fibers for the rest of sampled body regions, at a mean temperature and relative humidity of 25°C and 45.7%, respectively. The lower sample size for tail skirt fibers is justified by their comparatively lower density in this particular body region. Tail skirt fibers are typically sparser and exhibit a lower concentration per unit area compared to other regions, such as the shoulder or belly.
Parameter description
A list with the respective descriptors, acronyms and mean values of the fiber parameters measured by the optical fiber diameter analyzer across sampling site and depending on the phaneroptics for coat and eye color sorted by animal species and age is presented in Supplementary Table S1.
Statistical analysis
Following the methodology on González Ariza et al. (Citation2021), a discriminant canonical analysis was first used to develop a tool that evaluates the best possible linear combinations of physical-mechanical quality-related traits of hair fibers that shape within and between population clustering patterns across species/body region (Canarian camel and Merino sheep; shoulder, hump, belly, rump, tail dock and tail skirt).
A second discriminant canonical analysis was run to evaluate the linear combinations of physical-mechanical quality-related traits of hair fibers that are able to determine within population clustering patterns across coat color phenotypes (phaneroptics-related trait) in Canarian camels, excluding Merino sheep given that no variability is respectively officially registered.
Results
Statistical analysis
Discriminant canonical analysis model reliability
Following the multicollinearity analyses (to remove any redundant factor(s) from the set of explanatory variables) (J. Y. L. Chan et al. Citation2022), only specific variables were retained in the discriminant canonical analyses, namely: light, maxSD, sdD along 200um, %<15um, fiFromTip, spinF, SD Along Profile, numD, densSD, minSD, CEM, lightSD, baseD, large blob%, and length staple (with VIF values < 5; refer to ). The variables “light,” “lightSD,” “maxSD,” and “minSD” are related to the opacity and light reflection capacity of the hair fibers. At a practical level, such properties render potential for the manufacturing of distinct textile products depending on the preferred brightness. “sdD along 200um,” “%<15um,” “spinF,” “SD Along Profile,” “CEM,” and “baseD” refer to the mean diameter of the fibers and the variation of these physical properties along the staple, thus a reliable measure of fiber fineness. Moreover, “fiFromTip” concerns the millimeters from the tip to the finest point in the staple, hence an indicator for the point of break of the hair fibers. The diameter of the hair fibers, together with the length of the staple (“length staple”), hold paramount significance in textile manufacturing, ensuring the creation of fabrics with optimal strength, softness, and durability, elevating the overall quality of the end products. However, the final industrial performance of fibers can also be improved through the control of the percentage of residual dirt of fibers before textile processing (“large blob%”). In any case, a representative sample for optical analysis has to be procured (“numD” and “densSD”) in order to avoid the measurements being affected by the sample size (Walker et al. Citation2018) (Supplementary Table S1).
Figure 2. Summary of the value of tolerance and VIF after multicollinearity analysis of physical-mechanical of Canarian camel hair and Merino sheep quality-related traits when clustering patterns are phaneroptics (a) and sampling site (b), respectively. Interpretation thumb rule: VIF ≥ 5 (highly correlated); 1 < VIF < 5 (moderately correlated); VIF = 1 (not correlated).
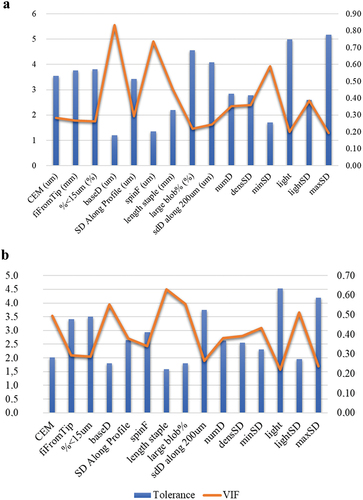
Pillai’s trace criterion was highly statistically significant when either phaneroptics (Pillai’s trace criterion: 1.0938; df1: 465; df2: 11940; p < .0001) or sampling site (Pillai’s trace criterion: 3.0593; df1: 330; df2: 21870; p < .0001) were considered clustering criteria. Hence, the validity of the discriminant canonical analysis is ensured (Lipovetsky Citation2015).
When phaneroptics (coat and eye color and particularities), four out of the fifteen functions revealed after the discriminant analysis were reported to be significant for their discriminant ability. However, ten out of the fifteen functions were revealed to be significant when the clustering pattern considered was the sampling site (). The discriminatory power of the F1 function was moderate for phaneroptics and high for sampling site (eigenvalues of 0.3288 and 11.9580, respectively; ) with 66.85% and 99.72% of the variance significantly explained by F1 to F4 and F1 to F10, respectively.
Figure 3. Canonical variable functions and their percentages of self-explained and cumulative variance.
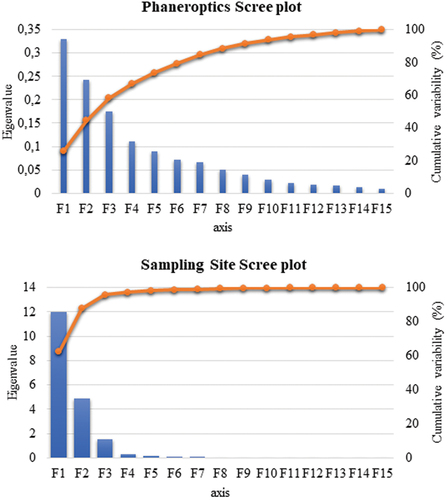
Table 2. Canonical discriminant analysis efficiency parameters to determine the significance of each canonical discriminant function.
Canonical coefficients and loading interpretation
The different variables studied in this research were ranked according to their discriminating ability. A test of equality of group means of physical-mechanical hair/wool quality-related traits was used as shown in . A better discriminating power is indicated by greater values of F and consequently, lower values of Wilks’ Lambda (El Ouardighi, El Akadi, and Aboutajdine Citation2007). The present analysis revealed that all physical-mechanical hair/wool quality-related traits highly significantly contributed (p < .0001) to the discriminant functions when Sampling site was considered the clustering criterion while, SD Along Profile (um), maxSD, spinF (um) and fiFromTip (mm) did not significantly contribute to the discriminant functions when Phaneroptics were considered the clustering criterion.
Table 3. Results for the tests of equality of group means to test for difference in the means across sample groups once redundant variables have been removed.
Standardized discriminant coefficients measure the relative weight of each trait across the established discriminant functions (). The two most relevant functions were used to depict a standardized discriminant coefficients biplot which captures the highest fraction of data variability (). Variables whose vector extends further beyond the origin more relevantly contributed to F1 and F2 discriminant functions.
Figure 4. Discriminant standardized coefficients for physical-mechanical hair/wool quality related traits in Canarian camel and Merino sheep in each canonical discriminant function. Each bar represents the relative weights (loadings) of each particular trait across the six significant discriminant functions evidenced by the discriminant canonical analysis.
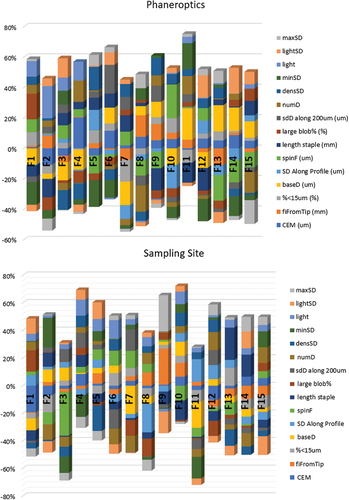
Figure 5. Territorial map depicting the centroids of the different variables (physical-mechanical hair/wool quality related traits) considered in the discriminant canonical analyses.
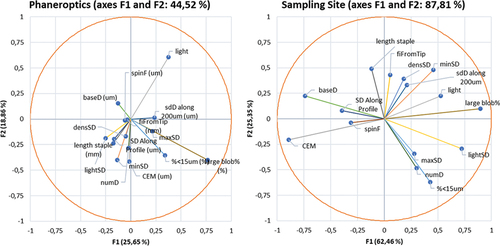
A Press’ Q value of 345.972 (n = 828; n’ = 119; K = 32) and of 4553.949 (n = 1481; n’ = 594; K = 23) were computed for Phaneroptics combinations (Coat color and particularities and Eye color) and Sampling sites (across species and age group), respectively. Thus, predictions can be considered to be better than chance at 95% (Y. H. Chan Citation2005).
Centroids from different phaneroptic possibilities and sampling sites considered in this study are calculated. The relative position of each centroid was determined by substituting the mean value for the observations depicted in the two first discriminant functions (F1 and F2).
Mahalanobis spatial representation data mining CHAID decision trees
Mahalanobis distances across phaneroptic possibilities () and sampling sites and species/age groups () were represented in two dendrograms. Mahalanobis distance measures the separation between two points in an n-dimensional space, taking into account the significance of each component and considering their correlations (Brereton and Lloyd Citation2016). CHAID (Chi-squared automatic interaction detection) decision trees are provided in Supplementary Table S2. The major benefit of CHAID analysis is that it identifies a relatively small number of explanatory variables (i.e., predictors) that have the highest significant power to discriminate among the levels of the clustering criterion. The selection of these predictors is based on their effectiveness in partitioning the data into meaningful categories (Dalal et al. Citation2023).
Figure 6. Cladogram constructed from Mahalanobis distances across phaneroptic combinations of coat color and particularities, and eye color in Canarian camels.
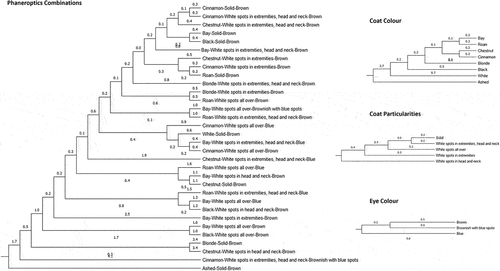
Figure 7. Cladogram constructed from Mahalanobis distances across sampling sites and age groups in Canarian camels and Merino sheep.
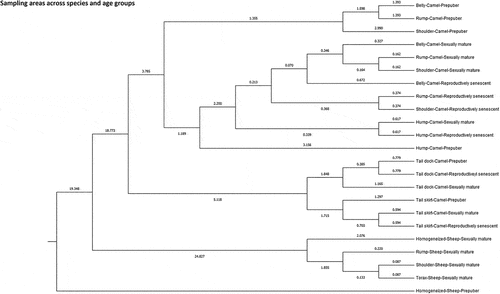
represents a dendrogram depicting the proximity across phaneroptics (coat and eye color and particularities), in regard to fiber parameters quantified by using an optical fiber diameter analysis. A clear distinction is made between darker and lighter coats and between depigmented areas and white coats. This also concerns eye color and particularities as the clustering patterns that are revealed focus on the similarities between solid coats with those in which clear and extensive areas (covering head, body and extremities) of depigmentation are shown, in opposition to those lighter colored coats and for which depigmentation circumscribes to rather limited areas as represented in the dendrograms for coat colors, coat particularities and eye colors. The respective CHAID decision tree suggest that light and minSD parameters, which are a measure of the light level next to fiber and indirectly a measure of the absorbance properties of the fiber, and the standard deviation of minimum gray level inside fiber, are the main discriminant parameters between camel hair fibers when phaneroptics is considered the clustering criterion.
The main ramification in the dendrogram in splits data into two clusters. This ramification bases upon the differences in baseD. First cluster comprises Homogenized samples from prepuber sheep, which is the group accounting for the lowest baseD while presenting the highest lightSD (>1.3) in comparison to the rest (<1.3) (CHAID decision tree).
Afterwards, two subclusters form the second cluster. First, a subcluster comprising sexually mature sheep which ramifies into two; the first separates samples per areas (with thorax and shoulder being closer than rump) while the second constitutes the homogenized sample from sexually mature sheep basing upon the fibers from these groups presenting baseD < 25.06 and CEM < 8.6, with independence from length staple (CHAID decision tree). The second subcluster ramifies into two additional subclusters, one comprising the fibers from the tail dock, and another one comprising the fibers from the tail skirt of camels. Within the last subcluster, sexually mature and reproductively senescent camels are clustered together while the fibers from the tail skirt of prepuber camels separate apart basing upon baseD > 42.5 and large blob% >0.10 (CHAID decision tree).
The last aggrupation consists on a distinction between prepuber camel fibers from rump, belly and shoulder, and fibers from belly, rump, shoulder, and hump of camel from either age subgroup. Fibers from the hump presents >0.8 sdD along 200 μm. Fibers from rump are characterized by 25.06 to 28.9 baseD and <0.95 sdD along 200 μm, while hair fibers from belly and shoulder are mostly characterized by a sdD along 200 μm < 0.87 (CHAID decision tree).
Discussion
The value of animal fibers depends significantly on mean fiber diameter and length. These quantitative attributes are regulated by qualitative traits that segregates according to Mendel’s laws (S. Bolormaa et al. Citation2021; Purvis and Franklin Citation2005). Contrastingly to other domestic fiber-producing species, there is limited empirical knowledge on the influence of qualitative traits such as body region and coat color influence camel fiber quality. Such a scarcity hinders the definition of selection criteria for genetic improvement purposes at camel production scenarios and the exploitation of industrial opportunities for camel hair fibers (Alshanbari et al. Citation2019). To address this research gap, this study provides a theoretical foundation on the quantitative attributes of camel hair fibers, differentially affected by the location of the fiber in body regions and its color. This analysis focuses on a distinct camel breed, the Canarian camel, known for its unique morphometry influenced by functionality (Iglesias et al. Citation2020; Schulz Citation2008) and notable intraherd variability for phaneroptic traits (Fernández de Sierra and Fabelo Marrero Citation2017). Considering the variations in both the number and density of fibers across different body regions (Supplementary Table S1), representative samples were procured for each body region to avoid the measurements being significantly affected by the sample size (Walker et al. Citation2018). In addition, to ensure the comparability of results while addressing this existing variability, we employed discriminant canonical analysis (DCA). This robust statistical technique allowed us to effectively account for variations attributable to factors like body region or coat color while minimizing the potential biases introduced by differences in sample size and density (Dhamnetiya et al. Citation2022).
Body region
From the mid-region to the back of the animal, the diameter at the base of the fiber is higher for adult camels’ hair. As previously stated by Petrie (Citation1995), the coarseness of animal coats is greater for animals inhabiting hot climates. This characteristic is closely linked to skin thickness (Taha Citation2010), which increases as age does (Nomura, Okuma, and Kitamura Citation2000); to the volume and internal diameter of the primary follicle (Abdou, Hekal, and Khamis Citation2006; Ansari-Renani et al. Citation2010); and to the diameter and secretory activity of the follicular papilla (Burns and Clarkson Citation1949) in camels.
Our results () show that the diameter at the base of adult camel fibers increase, in ascending order, for the rump (25.06–28.9 µm), hump (28.9–42.4 µm), tail dock (42.4–55 µm) and belly (>55 µm) regions. This pattern may be attributed to the local coarser skin due to greater exposure to sand abrasion (i.e. belly), proximity to pronounced articular joints (i.e. tail dock), and the combination of pressure and friction induced by saddles (i.e. hump and rump). In addition, the diameter at the base of the fiber and the general size of the hair shaft produced are stated to be influenced by both the density of the local primary follicles and the cellular volume and secretory activity of the follicular papilla found within this type of follicles (Chi, Wu, and Morgan Citation2013).
Contrarily, the hair fibers sampled at the shoulder region were found to not have a high diameter at their base, but they exhibited the greatest value for the variable CEM (Coarse Edge Micron) (>8.6 µm). This finding is likely influenced by the particularly homogeneous higher length and larger thickness of fibers in this body area of the studied camel breed (Fernández de Sierra and Fabelo Marrero Citation2017). From a genomics perspective, this unique characteristic could be further confirmed based on the research by Alhaddad and Alhajeri (Citation2019), who identified that variations in FGF5 gene are associated with hair length variability in dromedary camels.
In connection with the previous statement, significant variability is observed for fibers around the hump region in Canarian dromedary camels, encompassing both mean diameter and length values, as well as color or shade (Fernández de Sierra and Fabelo Marrero Citation2017). Our results confirm that the variability in mean diameter along the fiber is highest for fibers sampled from the hump of adult camels (>0.87 µm), contrasting with those from the shoulder (<0.77 µm). In contrast, the highest values for this trait in sheep pertain to fibers sampled from the rump (>0.95 µm).
In this context, Tridico (Citation2009) suggested, through low power microscopy evaluation, that camel hair has a more regular outline than sheep wool, providing benefits such as higher resistance to processing in the textile industry (Wang Citation2000) and a smoother tactile perception for customers wearing clothes made with this type of fiber (Ramalho, Szekeres, and Fernandes Citation2013). This last characteristic arises from the lower coefficient of friction of camel hair fibers due to the just referred homogeneous, straight scale overlapping along the staple (Shakyawar, Patni, and Gupta Citation2007). Thus, in those regions characterized by a reduced fiber diameter and/or higher variability for both mean diameter and length of the fibers, not only the tactile perception at the final textiles will be rough, but the tensile resistance is diminished (B. Bolormaa, Drean, and Enkhtuya Citation2008). This reduction in tensile resistance subsequently elevates the likelihood of fiber breakage, as highlighted by Li et al. (Citation2011). Additionally, the homogeneous surface along the length of camel hair may explain the comparatively higher capacity for light reflection in camel hair fibers (lightSD <1.3 nm) (Chen, Lin, and Tan Citation2019; Wortmann, Schulze zur Wiesche, and Bourceau Citation2004), offering advantages in the manufacturing of textile products with higher lightness.
Therefore, not only is the mean diameter along the fiber more variable for sheep wool, but also the variability in this fiber concerning its ability to reflect light (lightSD >1.3 nm), as expected based on the literature mentioned earlier. Furthermore, regarding the mentioned local variation in fiber length at some body areas in Canarian dromedary camels, this condition is also more prevalent in studied blended sheep wool samples (both over and below 50 mm). These samples are composed of fibers from different parts of the animal’s body and are routinely used in textile manufacturing. Hence, we highlight that although sheep wool is slightly larger than camel hair, the potential industrial yield and end uses of the former, based on its characteristics for diameter homogeneity and light reflection capacity, necessitate the innovative development of preparation technologies and the recognition of the industrial scope of this animal fiber.
In terms of fineness, the body region with the highest percentage of fibers with a mean diameter less than 15 µ is the tail dock in adult camels (>10%). However, this is also the body area with the greatest amount of residual dirt (>10%) that might impact the industrial performance of fibers. One possible explanation is the special sensitivity in this body region (Karolewicz and Paul Citation2001), where the thickness of the hair is lesser compared with the rest of the body, likely to favor the proper perception of surrounding stimuli. In addition, local cleanliness is relatively low and may be more pronounced in aged animals that spend less time on self-grooming. Despite being the finest fibers and thus having a higher market price, it is essential to note that preliminary processing of these fibers for the physical removal of impurities could reduce final yields and further increase their monetary value. To mitigate this inconvenient, intraherd management practices should include regular general hygiene for the animals and attention to the materials used as litters.
Comparing the results from the present research with others related to the same species and genus reveals quite different observations. For Indian camels, the lowest diameter was reported for the belly (32.98 µm) and scapula (34.49 µm) regions, while the highest values for this parameter were found in hump (39.02 µm) fibers (Bhakat Citation2019). Similar trends are observed for mid-side region fibers (21.00 µm for belly; 27.22 µm for shoulder; 33.59 µm for hump) in double-humped camels (Sahani et al. Citation2003). In contrast, Harizi et al. (Citation2007) discovered that the mean diameter of hair fibers sampled from the scapula, hump, and rump is greater (25 µm) than that of fibers from the belly (20 µm) region in Tunisian dromedaries. These differences between studies may be attributed to the animal morphotype (Frank, Hick, Lamas, et al. Citation2006), specific to each breed and influenced by environmental factors and functional destinations. These factors might, through pleiotropic effects, influence the phenotypic expression of hair attributes.
In other camelid and ruminant species in which selection for functional traits related to fiber production is well practiced, the existing literature is extensive. In New Zealand and Ecuador alpacas, the fleece tends to coarsen and enlarge from the mid-side (23.4 µm) body region to both the scapula (23.7 µm) and rump (23.9 µm) points, as well as toward distal points such as extremities (29.4 µm) and the neck (24.3 µm). This trend exhibits a negative correlation with the comfort factor as long as these quality attributes increase in value (Solano and Raggi Citation2019; Wuliji et al. Citation2000). For vicuñas, the average diameter of fibers is known to increase from the dorsal area (13.9 µm) to the flanks and extremities (14 µm), and from the anterior area (13.2 µm) toward the back region (13.6 µm) (Quispe et al. Citation2014). This finding aligns with those reported for Angora mohair (McGregor and Butler Citation2008; Taddeo et al. Citation2000), Bolivian and Peruvian alpacas (Aylan-Parker and McGregor Citation2002; McGregor, Ramos, and Quispe Peña Citation2012), Merino sheep wool (Fish, Mahar, and Crook Citation2002), and American bison wool (McGregor Citation2012). In terms of variability, the vicuña fleece has comparatively greater variance in fiber attributes among sampling sites but smaller within fibers of the same sampling area and along the length of the fiber. Thus, the vicuña fleece is more homogeneous than sheep wool, Angora mohair and alpaca fleece (Quispe et al. Citation2014), which is in turn comparable to our conclusions for camel hair.
Coat color
The phaneroptic variability documented for the studied camel breed (Fernández de Sierra and Fabelo Marrero Citation2017) is expected to influence the phenotype of hair fibers. The quantitative trait that best discriminate between coat colors is the differential ability to reflect light (). This can be translated into preferable appearances in terms of brightness during textile manufacturing. In fact, hair fibers from chestnut and bay camels, with discolored areas at extremities, head, and neck, have a lower capacity to reflect light (<101.7 nm). In contrast, fibers sampled from bay, cinnamon, blonde, and chestnut camels, either with solid color around the whole body or with defined white spots at extremities, showed a higher light reflection potential (>101.7 nm).
As suggested by Khattab and Tributsch (Citation2015), two kinds of apparently white hair can be found – transparent or solid white. Transparent hairs are present scattered along the fur of some other species, like polar bears, or grow in depigmented areas of skin, like white spotting due to local failure in melanocyte migration. Although these two types of white hair may appear white, light absorbance studies have revealed a greater ability to reflect light on white hair growing on pigmented skin. Transparent hair may absorb most of the incident radiation, behaving as solid darker-colored patterns. Such a particular behavior of the fibers needs to be taken into account by textile stakeholders when organizing the production and supply chains, and expected sales revenue. Contrastingly, in other fiber-producing species, variability in fiber characteristics attributed to coat color-related influences has been reported. This includes fiber coarseness, medullation, length, compressive strength, comfort factor, and yield in alpacas (Solano and Raggi Citation2019; Wuliji et al. Citation2000); fiber diameter in llamas (Frank, Hick, Gauna, et al. Citation2006) and cashmere goats (McGregor, Kerven, and Toigonbaev Citation2009); and density, length, diameter, and thermal insulation in sheep wool (Leite et al. Citation2020). Therefore, the significant variability in coat color, from dark to bright colors, in desert-living animals like camels might be attributed to the fact that coat color does not significantly affect other quality-related traits of hair fibers apart from lightness. What’s the same, the inner medullation of the hair fibers of camels might be the major influencing factor of thermal insulation in these animal species.
Genomic research in this field, utilizing knowledge from other livestock and companion animals (Chandramohan et al. Citation2013; Eizirik et al. Citation2003; Fontanesi et al. Citation2011; Kerje et al. Citation2003; Rieder et al. Citation2001), indicates that polymorphisms in the KIT (Holl et al. Citation2017), MC1R, and ASIP genes (Almathen et al. Citation2018; Bitaraf Sani et al. Citation2022), may be associated with coat color variation in dromedaries. More specifically, the TYRP1 gene was proposed as a candidate gene responsible for shade variability at brown coats in Arabian camels (Alshanbari et al. Citation2019).
Conclusions
The diameter at the base of the fiber is comparatively higher for camel hair fibers sampled from the belly, rump and tail dock of adult animals. This can be attributed to the higher thickness of skin at these areas due to their major risk of abrasion by sand and the saddles used for riding activities, but also to the pronounced volume and secretory activity of local follicular papillae. Concretely, the activity of the local follicular papillae is correlated with the size of the hair shaft produced and the general, homogeneous growth of the hair fibers, hence it may determine their improved industrial performance. By contrast, a notable variability, hence potentially impaired performance at textile industry scenarios, does exist for both staple length and mean diameter of hair fibers from the regions of shoulder and hump of this animal species. By contrast, such variability for Merino sheep wool is present at hair fibers obtained from the rump as well as for the blended samples, which are composed of fibers mixed from different body regions. In regard to fineness, camel hair fibers from tail dock are generally lower than 15 µ of diameter, with the inconvenient that this area is also the one with the highest percentage of residual dirt. Thus, pre-processing or preparation methods would be necessary to improve the final industrial performance of such fibers, which may increase their costs along the production chain and negatively impact their demand. Besides, our results show that selection for coat color and particularities is unlikely to significantly affect fiber diameter and strength but light reflection capacity of camel hair fibers, which in turn has a potential basis for the manufacturing of textile products depending on the preferred brightness. Overall, the study’s outcomes are intended to serve as a fundamental basis for the recognition of the industrial scope of camel hair fibers and the adapted definition of camel selection schemes and fiber pre-processing techniques depending on the targeted physical-mechanical attributes at the final textile products.
Highlights
Diameter at the base of the fiber is higher for adult camels and increases from the mid-region to the back of the animal.
Notable, intraregional variability does exist for staple length and mean diameter of camel shoulder and hump fibers.
Camel tail dock is the region with the finest fibers but also the one accounting for the highest percentage of residual dirt.
Coat color and particularities do not impact camel fiber diameter and strength but brightness of final textile products.
Adapted definition of camel selection schemes and fiber pre-processing techniques will aid at valorizing camel hair fibers.
Supplemental Material
Download Zip (192.5 KB)Acknowledgments
The authors would like to thank ‘Aires Africanos’ Aires Africanos” Eco-tourism Company, Oasis Park Fuerteventura and ‘Camelus’ Camellos de Almería, for their direct technical help and assistance.
Disclosure statement
No potential conflict of interest was reported by the author(s).
Data availability statement
The data that support the findings of this study are available from the corresponding author, F.J.N.G., upon reasonable request.
Supplementary Material
Supplemental data for this article can be accessed online at https://doi.org/10.1080/15440478.2024.2357237
Additional information
Funding
References
- Abdou, A. S. A., S. A. A. Hekal, and H. S. Khamis. 2006. “Effect of Supplementary Feeding Under Different Grazing Conditions on the Skin Follicles and Hair Coat in Camels Raised at Halaieb Shalateen and Abo-Ramad Triangle, Egypt.” Egyptian Journal of Animal Production 43 (2): 139–18.
- Alhaddad, H., and B. H. Alhajeri. 2019. “Cdrom Archive: A Gateway to Study Camel Phenotypes.” Frontiers in Genetics 10:48. https://doi.org/10.3389/fgene.2019.00048.
- Allafi, F. A., M. S. Hossain, M. Shaah, J. Lalung, M. O. Ab Kadir, and M. I. Ahmad. 2022. “A Review on Characterization of Sheep Wool Impurities and Existing Techniques of Cleaning: Industrial and Environmental Challenges.” Journal of Natural Fibers 19 (14): 8669–8687.
- Allain, D., and C. Renieri. 2010. “Genetics of Fibre Production and Fleece Characteristics in Small Ruminants, Angora Rabbit and South American Camelids.” Animal 4 (9): 1472–1481.
- Almathen, F., H. Elbir, H. Bahbahani, J. Mwacharo, and O. Hanotte. 2018. “Polymorphisms in MC1R and ASIP Genes Are Associated with Coat Color Variation in the Arabian Camel.” The Journal of Heredity 109 (6): 700–706.
- Al-Qarawi, A. A., H. A. Abdel-Rahman, M. S. El-Belely, and S. A. El-Mougy. 2000. “Age-Related Changes in Plasma Testosterone Concentrations and Genital Organs Content of Bulk and Trace Elements in the Male Dromedary Camel.” Animal reproduction science 62 (4): 297–307.
- Al-Qarawi, A. A., H. A. Abdel-Rahman, M. S. El-Belely, and S. A. El-Mougy. 2001. “Intratesticular Morphometric, Cellular and Endocrine Changes Around the Pubertal Period in Dromedary Camels.” The Veterinary Journal 162 (3): 241–249.
- Alshanbari, F., C. Castaneda, R. Juras, A. Hillhouse, M. N. Mendoza, G. A. Gutiérrez, F. A. Ponce de León, and T. Raudsepp. 2019. “Comparative FISH-Mapping of MC1R, ASIP, and TYRP1 in New and Old World Camelids and Association Analysis with Coat Color Phenotypes in the Dromedary (Camelus dromedarius).” Frontiers in Genetics 10:340. https://doi.org/10.3389/fgene.2019.00340.
- Anjali, S., and P. Suman. 2013. “Studies on Camel Hair-Merino Wool Blended Knitted Fabrics.” Indian Journal of Fibre & Textile Research 38:317–319.
- Ansari-Renani, H. R., M. Salehi, Z. Ebadi, and S. Moradi. 2010. “Identification of Hair Follicle Characteristics and Activity of One and Two Humped Camels.” Small Ruminant Research 90 (1–3): 64–70.
- Aylan-Parker, J., and B. A. McGregor. 2002. “Optimising Sampling Techniques and Estimating Sampling Variance of Fleece Quality Attributes in Alpacas.” Small Ruminant Research 44 (1): 53–64.
- Babu, K. M. 2015. “Natural textile fibres: Animal and silk fibres.” In Textiles and Fashion, edited by R. Sinclair, 57–78. Sawston, Cambridge, UK: Woodhead Publishing. https://doi.org/10.1016/C2013-0-17410-7.
- Bathrachalam, C., C. Nocelli, I. Pazzaglia, S. Pallotti, D. Pediconi, A. La Terza, and C. Renieri. 2019. “Interaction Between ASIP and MC1R in Black and Brown Alpaca.” In Advances in Fibre Production Science in South American Camelids and Other Fibre Animals, edited by L. M. Gutiérrez, R. Niznikowski, and M. Wurzinger, 163. Göttingen, Germany: University of Göttingen.
- Bhakat, C. 2019. “Effect of Certain Factors on Hair Quality Attributes in Indian Dromedary Camel Management in an Organized Farm.” The Indian Journal of Animal Sciences 71 (10): 992–994.
- Bitaraf Sani, M., J. Z. Harofte, M. H. Banabazi, A. Faraz, S. Esmaeilkhanian, A. S. Naderi, N. Salim, A. Teimoori, A. Bitaraf, and M. Zadehrahmani. 2022. “Identification of Candidate Genes for Pigmentation in Camels Using Genotyping-By-Sequencing.” Animals 12 (9): 1095.
- Bolormaa, B., J. Y. Drean, and D. Enkhtuya. 2008. “A Study of the Diameter Distribution and Tensile Property of Horse Tail Hair.” Journal of Natural Fibers 4 (4): 1–11.
- Bolormaa, S., A. A. Swan, P. Stothard, M. Khansefid, N. Moghaddar, N. Duijvesteijn, J. H. J. van der Werf, H. D. Daetwyler, and I. M. MacLeod. 2021. “A Conditional Multi-Trait Sequence GWAS Discovers Pleiotropic Candidate Genes and Variants for Sheep Wool, Skin Wrinkle and Breech Cover Traits.” Interaction Studies 53 (1): 1–14.
- Brereton, R. G., and G. R. Lloyd. 2016. “Re‐Evaluating the Role of the Mahalanobis Distance Measure.” Journal of Chemometrics 30 (4): 134–143.
- Burns, M., and H. Clarkson. 1949. “Some Observations on the Dimensions of Follicles and of Other Structures in the Skin of Sheep.” The Journal of Agricultural Science 39 (4): 315–334.
- Chan, Y. H. 2005. “Biostatistics 303. Discriminant Analysis.” Singapore medical journal 46 (2): 54.
- Chandramohan, B., C. Renieri, V. La Manna, and A. La Terza. 2013. “The Alpaca Agouti Gene: Genomic Locus, Transcripts and Causative Mutations of Eumelanic and Pheomelanic Coat Color.” Gene 521 (2): 303–310.
- Chan, J. Y. L., S. M. H. Leow, K. T. Bea, W. K. Cheng, S. W. Phoong, Z. W. Hong, and Y. L. Chen. 2022. “Mitigating the Multicollinearity Problem and Its Machine Learning Approach: A Review.” Mathematics 10 (8): 1283. https://doi.org/10.3390/math10081283.
- Chen, H., Z. Lin, and C. Tan. 2019. “Classification of Different Animal Fibers by Near Infrared Spectroscopy and Chemometric Models.” Microchemical Journal 144:489–494. https://doi.org/10.1016/j.microc.2018.10.011.
- Chi, W., E. Wu, and B. A. Morgan. 2013. “Dermal Papilla Cell Number Specifies Hair Size, Shape and Cycling and Its Reduction Causes Follicular Decline.” Development 140 (8): 1676–1683.
- Dalal, S., U. K. Lilhore, N. Faujdar, S. Simaiya, M. Ayadi, N. A. Almujally, and A. Ksibi. 2023. “Next-Generation Cyber Attack Prediction for IoT Systems: Leveraging Multi-Class SVM and Optimized CHAID Decision Tree.” Journal of Cloud Computing 12 (1): 137. https://doi.org/10.1186/s13677-023-00517-4.
- Dhamnetiya, D., M. K. Goel, R. P. Jha, S. Shalini, and K. Bhattacharyya. 2022. “How to Perform Discriminant Analysis in Medical Research? Explained with Illustrations.” Journal of Laboratory Physicians 14 (4): 511–520.
- Eizirik, E., N. Yuhki, W. E. Johnson, M. Menotti-Raymond, S. S. Hannah, and S. O’Brien. 2003. “Molecular Genetics and Evolution of Melanism in the Cat Family.” Current Biology 13 (5): 448–453.
- El Ouardighi, A., A. El Akadi, and D. Aboutajdine. 2007. “Feature Selection on Supervised Classification Using Wilks Lambda Statistic.” 2007 International Symposium on Computational Intelligence and Intelligent Informatics, 28-30 March, 2007, Agadir, Morocco, 51–55.
- Fernández de Sierra, G., and F. J. Fabelo Marrero. 2017. “El camello canario.” Lanzarote, Spain: Asociación de Criadores del Camello Canario. Aderlan.
- Fish, V. E., T. J. Mahar, and B. J. Crook. 2002. “Sampling Variation Over a Fleece for Mean Fibre Diameter, Standard Deviation of Fibre Diameter and Mean Fibre Curvature.” Wool Technology and Sheep Breeding 50 (4): 798–804.
- Fontanesi, L., S. Dall’olio, F. Beretti, B. Portolano, and V. Russo. 2011. “Coat Colours in the Massese Sheep Breed Are Associated with Mutations in the Agouti Signalling Protein (ASIP) and Melanocortin 1 Receptor (MC1R) Genes.” Animal 5 (1): 8–17.
- Frank, E. N., M. V. H. Hick, C. D. Gauna, H. E. Lamas, C. Renieri, and M. Antonini. 2006. “Phenotypic and Genetic Description of Fibre Traits in South American Domestic Camelids (Llamas and Alpacas.” Small Ruminant Research 61 (2–3): 113–129.
- Frank, E. N., M. V. H. Hick, H. E. Lamas, C. D. Gauna, and M. G. Molina. 2006. “Effects of Age-Class, Shearing Interval, Fleece and Color Types on Fiber Quality and Production in Argentine Llamas.” Small Ruminant Research 61 (2–3): 141–152.
- Galbraith, H. 2010. “Animal Fibre: Connecting Science and Production.” Animal 4 (9): 1447–1450.
- Gerken, M., C. Renieri, D. Allain, H. Galbraith, J. P. Gutiérrez, L. McKenna, R. Niznikowski, and M. Wurzinger. 2019. “Advances in Fibre Production Science in South American Camelids and Other Fibre Animals: Universitätsverlag Göttingen, Göttingen (Germany).”
- González Ariza, A., A. A. Arbulu, F. J. Navas González, J. V. Delgado Bermejo, and M. E. Camacho Vallejo. 2021. “Discriminant Canonical Analysis As a Validation Tool for Multivariety Native Breed Egg Commercial Quality Classification.” Foods 10 (3): 632.
- Harizi, T., S. Msahli, F. Sakli, and T. Khorchani. 2007. “Evaluation of Physical and Mechanical Properties of Tunisian Camel Hair.” The Journal of the Textile Institute 98 (1): 15–21.
- Hasi, S., G. Amu, and W. Zhang. 2020. “Camel Hair Structure, Properties, and Commercial Products.” In Handbook of Research on Health and Environmental Benefits of Camel Products, edited by O. Alhaj, B. Faye, and R. Agrawal, 328–347. Hershey, PA, USA: IGI Global. https://doi.org/10.4018/978-1-7998-1604-1.ch015
- Holl, H., R. Isaza, Y. Mohamoud, A. Ahmed, F. Almathen, C. Youcef, S. Gaouar, D. F. Antczak, and S. Brooks. 2017. “A Frameshift Mutation in KIT Is Associated with White Spotting in the Arabian Camel.” Genes (Basel) 8 (3): 102.
- Iglesias, C., F. J. Navas, E. Ciani, A. Arando Arbulu, A. González, C. Marín, S. Nogales, and J. Vicente Delgado Bermejo. 2020. “Zoometric Characterization and Body Condition Score in Canarian Camel Breed.” Archivos de Zootecnia 69 (265): 102–107.
- Karolewicz, D., and I. A. Paul. 2001. “Group Housing of Mice Increases Immobility and Antidepressant Sensitivity in the Forced Swim and Tail Suspension Tests.” European Journal of Pharmacology 415 (2–3): 197–201.
- Kerje, S., J. Lind, K. Schütz, P. Jensen, and L. Andersson. 2003. “Melanocortin 1‐Receptor (MC1R) Mutations Are Associated with Plumage Colour in Chicken.” Animal Genetics 34 (4): 241–248.
- Kerven, C., A. J. F. Russel, and J. P. Laker. 2002. Potential for Increasing producers’ Income from Wool, Fibre and Pelts in Central Asia. Vol. 45. Nairobi, Kenya: International Livestock Research Institute.
- Khattab, M. Q., and H. Tributsch. 2015a. “Fibre-Optical Light Scattering Technology in Polar Bear Hair: A Re-Evaluation and New Results.” Journal of Advanced Biotechnology and Bioengineering 3 (2): 38–51.
- Leite, J. H. G. M., R. Gomes Da Silva, L. A. Bermejo Asensio, J. Ernandes Rufino de Sousa, W. S. Tavares da Silva, W. E. da Silva, and D. A. Evangelista Façanha. 2020. “Coat Color and Morphological Hair Traits Influence on the Mechanisms Related to the Heat Tolerance in Hair Sheep.” International Journal of Biometeorology 64 (12): 2185–2194.
- Li, Q., C. J. Hurren, C. Ding, L. Wang, T. Lin, and X. Wang. 2011. “Ultrasonic Scouring of Wool and Its Effects on Fibre Breakage During Carding.” The Journal of the Textile Institute 102 (12): 1059–1064.
- Lipovetsky, S. 2015. “MANOVA, LDA, and FA Criteria in Clusters Parameter Estimation.” Cogent Mathematics 2 (1): 1071013. https://doi.org/10.1080/23311835.2015.1071013.
- McGregor, B. A. 2012. “Production, Properties and Processing of American Bison (Bison Bison) Wool Grown in Southern Australia.” Animal Production Science 52 (7): 431–435.
- McGregor, B. A., and K. L. Butler. 2008. “Variation of Mean Fibre Diameter Across Mohair Fleeces: Implications for within Flock Animal Selection, Genetic Selection, Fleece Classing and Objective Sale Lot Building.” Small Ruminant Research 75 (1): 54–64.
- McGregor, B. A., C. Kerven, and S. Toigonbaev. 2009. “Sources of Variation Contributing to Production and Quality Attributes of Kyrgyz Cashmere in Osh and Naryn Provinces: Implications for Industry Development.” Small Ruminant Research 84 (1–3): 89–99.
- McGregor, B. A., H. E. Ramos, and E. C. Quispe Peña. 2012. “Variation of Fibre Characteristics Among Sampling Sites for Huacaya Alpaca Fleeces from the High Andes.” Small Ruminant Research 102 (2–3): 191–196.
- McLaughlin, I. 2000. “Innovative Wool Testing - OFDA, Australian Wool Taskforce 2000.”
- Mokhtar, L. M., and E. I. Moussa. 2019. “Improve the Physical and Chemical Properties of camel’s Hair Fiber in Afif Province.” Journal of Nature, Life and Applied Sciences 3 (3): 47–70.
- Mysterud, A., T. Coulson, and N. C. Stenseth. 2002. “The Role of Males in the Dynamics of Ungulate Populations.” The Journal of Animal Ecology 71 (6): 907–915.
- Nieto, C. R., M. B. Ferguson, C. A. Macleay, J. R. Briegel, G. B. Martin, and A. N. Thompson. 2013. “Selection for Superior Growth Advances the Onset of Puberty and Increases Reproductive Performance in Ewe Lambs.” animal 7 (6): 990–997.
- Nomura, Y., Y. Okuma, and Y. Kitamura. 2000. “The Senescence-Accelerated Mouse As a Possible Animal Model of Senile Dementia.” In Central Nervous System Diseases. Contemporary Neuroscience, edited by D. F. Emerich, R. L. Dean, and P. R. Sanberg, 113–122. Totowa, NJ: Humana Press. https://doi.org/10.1007/978-1-59259-691-1_6.
- Petrie, O. J. 1995. Harvesting of Textile Animal Fibres. Rome, Italy: Food and Agriculture Organization of the United Nations (FAO). http://www.fao.org/docrep/v9384e/v9384e00.htm#con.
- Purvis, I. W., and I. R. Franklin. 2005. “Major Genes and QTL Influencing Wool Production and Quality: A Review.” Genetics Selection Evolution 37 (1): 1–11.
- Quispe, E. C., F. Sánchez, J. Bartolomé Filella, and L. Alfonso. 2014. “Variation of Commercially Important Characteristics Among Sampling Sites for vicuña (Vicugna Vicugna Mensalis) Fleeces.” Journal of Camelid Science 1:1–14.
- Ramalho, A., P. Szekeres, and E. Fernandes. 2013. “Friction and Tactile Perception of Textile Fabrics.” Tribology International 63:29–33. https://doi.org/10.1016/j.triboint.2012.08.018.
- Rieder, S., S. Taourit, D. Mariat, B. Langlois, and G. Guérin. 2001. “Mutations in the Agouti (ASIP), the Extension (MC1R), and the Brown (TYRP1) Loci and Their Association to Coat Color Phenotypes in Horses (Equus caballus.” Mammalian Genome 12 (6): 450–455.
- Sahani, M. S., B. Yadav, G. Mal, and R. S. Dhillon. 2003. “Quality Attributes of Double-Humped Camel Hair Fibres.” Indian Journal of Fibre & Textile Research 2:227–229.
- Schulz, U. 2008. El camello en Lanzarote. Lanzarote, Spain: Aderlan.
- Shakyawar, D. B., P. C. Patni, and N. P. Gupta. 2007. “Studies on Animal Fibre Blended Handmade Felts: Part II—Frictional, Compressional and Thermal Properties.” Indian Journal of Fibre & Textile Research 32:301–305.
- Solano, S. J. C., and L. Raggi. 2019. “Lanametric Determination of the Alpaca Fiber (Vicugna Pacos) in Tucayta, Province of Cañar.” Journal of Veterinary Science & Medicine 7 (1): 4.
- Swami, K., and S. Babel. 2023. “Current Utilisation Pattern of Camel Hair and Development of Value-Added Articles from Camel Hair.” The Pharma Innovation Journal 12 (7): 47–52.
- Tabbaa, M. J., W. A. Al-Azzawi, and D. Campbell. 2001. “Variation in Fleece Characteristics of Awassi Sheep at Different Ages.” Small Ruminant Research 41 (2): 95–100.
- Taddeo, H. R., L. Duga, D. Almeida, P. Willems, and R. Somlo. 2000. “Variation of Mohair Quality Over the Body in Angora Goats.” Small Ruminant Research 36 (3): 285–291.
- Taha, E. A. 2010. “Seasonal Changes in Some Coat Traits of Growing Dromedary Camels Under Egyptian Semi-Arid Conditions.” Egyptian Journal of Animal Production 47 (1): 65–74.
- Tridico, S. R. 2009. “Natural Animal Textile Fibres: Structure, Characteristics and Identification.” In Identification of Textile Fibers, edited by M. M. Houck, 27–67. Sawston, Cambridge: Woodhead Publishing.
- Walker, J. W., W. C. Stewart, R. Pope, S. L. Spear, M. Ebert, and T. W. Murphy. 2018. “Evaluation of Mean Fiber Diameter Measurements by FibreLux Micron Meter and OFDA2000 in Texas and Intermountain West Wool.” Small Ruminant Research 159:31–37. https://doi.org/10.1016/j.smallrumres.2017.12.008.
- Wang, X. 2000. “Predicting the Strength Variation of Wool from Its Diameter Variation.” Textile Research Journal 70 (3): 191–194.
- Wortmann, F. ‐. J., E. Schulze zur Wiesche, and B. Bourceau. 2004. “Analyzing the Laser‐Light Reflection from Human Hair Fibers. II. Deriving a Measure of Hair Luster.” International Journal of Cosmetic Science 26 (4): 219–.
- Wuliji, T., G. H. Davis, K. G. Dodds, P. R. Turner, R. N. Andrews, and G. D. Bruce. 2000. “Production Performance, Repeatability and Heritability Estimates for Live Weight, Fleece Weight and Fiber Characteristics of Alpacas in New Zealand.” Small Ruminant Research 37 (3): 189–201.
- Zarrin, M., J. L. Riveros, A. Ahmadpour, A. M. de Almeida, G. Konuspayeva, E. Vargas-Bello-Pérez, B. Faye, and L. E. Hernández-Castellano. 2020. “Camelids: New Players in the International Animal Production Context.” Tropical Animal Health and Production 52:903–913. https://doi.org/10.1007/s11250-019-02197-2.
- Zhenrui, L., S. Xiaohong, Z. Zhijun, and X. Xiangmin. 2021. “Slenderation Stretching Study of Camel Hair Through Reducing Processed by NaHSO 3.” Wool Textile Journal 49 (6): 27–31. https://doi.org/10.19333/j.mfkj.20200902805.