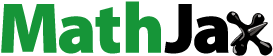
ABSTRACT
The research attempts to report the characterization of eight underexploited seed fibers viz. Adenium, Albizia, Blackboard, Munja, Plume thistle, Poplar, Reed, and Wild sugarcane fiber collected from various trees and shrubs in order to investigate their potential industrial applications. The fibers underwent detailed analysis of their physical properties and chemical components. Fourier transform infrared spectroscopy (FTIR), Thermal gravimetric analysis (TGA), scanning electron microscopy (SEM), and X-ray diffraction (XRD) were employed to determine the chemical, thermal, morphological, and crystalline analysis, respectively. The quantitative estimation of seed fibers by chemical component analysis revealed that the selected seed fibers are rich in cellulose (51.4–67.23%) with 7–25% lignin content. The SEM images depicted that the Munja, Poplar, Plume, and Wild sugarcane fiber are extremely fine with a fiber diameter of less than 10 μm. DTG analysis revealed the correlation between cellulose content and maximum peak temperature of decomposition of the fibers. Except for Adenium and Poplar fibers, all the fibers showed a crystalline index of more than 50%. Comprehensively, the selected seed fibers are comparable to cotton in fineness, density, moisture, and wax content. Gleaming whiteness of poplar fiber is comparable to cotton.
摘要
本研究试图报道从各种树木和灌木中收集的八种未被充分利用的种子纤维的特性,即Adenium、Albizia、Blackboard、Munja、Plume蓟、白杨、芦苇和野生甘蔗纤维,以研究其潜在的工业应用. 对纤维的物理性能和化学成分进行了详细分析. 采用傅立叶变换红外光谱(FTIR)、热重分析(TGA)、扫描电子显微镜(SEM)和X射线衍射(XRD)分别进行了化学分析、热分析、形态分析和结晶分析. 通过化学成分分析对种子纤维的定量估算表明,所选种子纤维富含纤维素(51.4–67.23%),木质素含量为7-25%. SEM图像显示,Munja、Poplar、Plume和Wild甘蔗纤维非常细,纤维直径小于10 μm. DTG分析揭示了纤维素含量与纤维分解的最高峰值温度之间的相关性. 除Adenium和Poplar纤维外,所有纤维的结晶指数均超过50%. 综合来看,所选种子纤维在细度、密度、水分和含蜡量方面与棉花相当. 杨纤维的消光白度与棉花相当.
Introduction
Most plant fibers are obtained from stems, leaves, and seeds, with seed fibers comprising an important category. Among these, cotton stands as the most valued seed fiber, followed by kapok and coir (Pandey, Jose, and Sinha Citation2022; Prasad et al. Citation2018). Seed fibers are generally finer compared to bast and leaf fibers. Cotton, renowned for its fineness, spinning efficiency, and moisture management, has gained prominence in apparel and basic wear (Pandey et al. Citation2022). Other seed fibers, such as kapok and coconut fiber, find application in technical textiles, particularly in home furnishing and agro-textiles, respectively (Prasad et al. Citation2018; Sinha and Pandey Citation2024).
The present work focuses on eight unreported seed fibers, namely Adenium, Albizia, Black-board tree, Munja, Poplar, Plume thistle, Reed, and Wild sugarcane, aiming to unravel their characteristics for potential utilization in the technical textile segment. While Munja, Reed, and Wild sugarcane hold traditional significance and are used for crafting handicraft articles and furniture (Pandey, Sinha, and Dubey Citation2023), their useful parts are typically their stems, with short fibers often discarded as waste. These fibers are naturally occurring, and apart from traditional uses, they are generally discarded (Pandey, Sinha, and Dubey Citation2023). Timber and ornamentals such as Poplar, Adenium, Albizia, and Blackboard, known for seed/floating fiber yield, have remained underutilized. Poplar biomass, for instance, finds application in biofuels, ethanol, and paper production. The dangling seed capsules of Poplar automatically burst at maturity, releasing male catkin Poplar fibers that float in the surrounding environment. Some reports suggest that these floating fibers may pose allergenic risks to humans (Pandey, Sinha, and Dubey Citation2023; Riyaz, Ignacimuthu, and Zuber Citation2020).
Existing uses of selected seed fibers include filling cushions, saddle pads, and pillows in rural areas. However, their proper utilization in papermaking, bioenergy, reinforced wood panels, acoustics, nonwovens, and the man-made textile manufacturing industry could be socially sustainable and economically advantageous for rural populations accustomed to handling these fibers for traditional uses (Pandey, Jose, and Sinha Citation2022; Pandey, Sinha, and Dubey Citation2023). Characterization of these fibers is crucial as it categorizes them based on their similar characteristics, facilitating their blending in industrial processes that require substantial amounts of raw materials (Adhia et al. Citation2021). Physico-chemical characterization of the fibers serves as the first step toward achieving this goal.
This research presents the physical and chemical characterization of eight selected seed fibers, which may provide guidance to environmentalists and stakeholders regarding the industrial potential of these waste seed fibers. The chemical composition of fibers significantly influences the mechanical properties of natural ligno-cellulosic fibers. Higher cellulose content enhances the strength and stability of fiber cell walls, supporting the load transfer mechanism. Hemicellulose acts as a cementing agent, binding with cellulosic microfibrils, while lignin contributes to thermally stable polymers. In bio-composites, desirable mechanical properties are achieved with high cellulose and moderate lignin content and low hemicellulose, moisture, ash, and wax content (Geremew et al. Citation2021; Lee, Khalina, and Lee Citation2021; Rajini et al. Citation2021; Thiagamani et al. Citation2023). The seed microfibers used in this study remain largely underutilized, except as filler material in cushions.
Fibers used as filler/reinforcement improves interfacial adhesion and physical, thermal, and flexural rigidity of composite materials. Finer fibers offer a higher surface-to-weight ratio, facilitating better dispersion and superior load transfer mechanisms. Plant fiber fillers promote rapid polymer crystallization during cooling and delay the glass transition period. Additionally, fine fiber fillers act as barriers during the varying thermal expansion of heterogeneous materials in a composite (Kumar et al. Citation2018; Lee, Khalina, and Lee Citation2021; Muthusamy et al. Citation2022; Ramalingam et al. Citation2023). Thus, this study aims to collect and characterize seed fibers from various trees and shrubs, potentially assisting researchers and small-scale industries in better utilization of the selected fibers.
Materials and methods
Fiber collection
Fiber collection was done manually. Seed pods, inflorescence, and seed capsules containing fibers were hand plucked. No additional preparation such as chemical or physical treatment was done to further enhance the characteristics of the fiber. All the fibers except poplar were collected from the interfluves of river Ganga and Yamuna in Kanpur, India. Poplar trees were growing in the foothills of Himalaya, India. Albizia, Munja, Plume thistle, Reed, and Wild sugarcane fibers were taken from mature dehiscent inflorescences (). Yellow-green Albizia floral fibers were collected from the handpicked inflorescence by the month of June to July. Wild sugarcane and tall Munja fibers/flowers bursting were apparent by early August and October, respectively. Plume thistle and Reed floral fibers were collected in April and November, respectively. Adenium and Blackboard tree fibers were collected from their seedpods on maturity. The fibers were hand plucked in late April. Poplar fiber used in the study was cultivated in Shimla (Populus ciliata) and Kashmir (Populus deltoid). Matured seed pods were plucked in the months of April to June.
Physico-chemical characterization
The physico-chemical properties and chemical composition of the selected fibers were performed as per our previous study (Pandey et al. Citation2021). The physical properties such as fiber length (AATCC 79–1992), color value (CIElab, hunterlab), CIE whiteness index, and yellowness index (ASTM D1925) were performed as per the standard protocols. The surface morphology of the samples was analyzed with the aid of a scanning electron microscope (Zeiss, Germany) at 200× magnification. The fiber samples were subjected to sputter coating before the analysis. The cellulose, lignin, ash, moisture, and wax content analysis were repeated five times (ASTM standard 1975). Fiber quantity required for cellulose, hemicellulose, lignin, and pectin content determination was 20 g for each of the tests. Moisture, wax, and ash content evaluation required 1.0 g fiber for each of the tests. Scanning electron microscope image analysis and the fiber diameter evaluation were done using ImageJ software.
The X-ray diffraction patterns were measured with PANalyticalXPert X Ray diffractometer, using CuKα radiation (k = 1.5406 A°) at 40 kV and 20 mA. The scattering was performed in the range of 2θ = 10° to 70°. The crystallinity index for each fiber type can be evaluated by using the Segal equation. The Segal equation for crystallinity index is
Where Imax is the intensity height of the highest peak present in the XRD and Iam is the intensity of the valley between the two crystalline peaks (or corresponds to the height of the amorphous peak) (da Silva et al. Citation2022).
The Fourier Transform Infrared Spectroscopy of the fibers was performed using a FTIR spectrometer (Shimadzu IR Prestige-21) using diamond single reflection ATR accessory from the wavenumber 4000−400 cm−1 with a resolution of 2 cm−1 and 49 scans.
To understand the thermal stability of the seed fibers, thermogravimetric (TGA) analysis was carried out using Netzsch TG 209 F3 Tarsus®. Thermal analysis was carried out using 30 ml/min flow rate of nitrogen atmosphere. The temperature ranges from 30 to 800°C with a 20°C/min heating rate was followed.
Results and discussion
Seed fiber classification
Seeds of the flora are an important source of lignocellulosic fibers. The classification of seed fibers presented in is based on seed pod shapes and characteristics. describes the phenotypes and current uses of eight fibers selected for the study.
Table 1. Classification of seed fibers.
Table 2. Phenotypes and economic potential of selected seed fiber plants/trees.
Seed inflorescence
Albizia, Munja, Plume thistle, Reed, and Wild sugarcane
Albizia () and Plume thistle () inflorescences shed within days of its arrival, whereas Munja, Reed, and Wild sugarcane inflorescences () remained intact with the plant for a longer duration (1–5 months). Prolong duration of fiber attachment to the plants allowed their procurement for an extended period. Tall Munja seed-burst yielded 60 g microfibers from 10 plant sticks. Wild sugarcane, also known as Kaans, yielded floral fibers like Munja. Plant was shorter in height as compared to Munja (Pandey, Sinha, and Dubey Citation2023). Cloudy white fibers did burst in early October, indicating end of monsoon and beginning of festive season in India.
Bean-shaped seedpod
Adenium and blackboard
Adenium is an ornamental small houseplant valued for its beautiful flower. Adenium and Blackboard fibers emerged in the pair of seedpods. Fibers () were collected from handpicked seed pods. Blackboard is an ornamental tree fluttering the roadside and distinctive from others due to its fragrant flowers and decorative bunch of seven leaves around petioles. The shady fragrant tree bore 50 to 80 mm long bean-shaped seed pods containing staple fibers.
Seed capsule
Poplar
The tree’s seed pods yielded soft and snowy stapled fibers ().
Physical characteristics
Fiber color and length
presents the physical properties of selected seed fibers. All the fibers were white in color except Adenium, Albizia, Reed, and Blackboard, which were yellowish and brown. Among the seed fibers, the whiteness of Poplar (P. ciliata) is incomparable and surpasses rest of the selected fibers (, ). Selected fibers are of short length (2.2–7.6 mm) and very fine. Length of all the eight fibers were in the range of 2 to 9 mm. Seed fibers have less density, which is suitable for lightweight composite fabrication (). The Plume thistle fiber has the least density (0.31 g/cc). comprehends cotton, milkweed, and tellicherry bark can be distinguished from the selected seed fibers in terms of fiber length. Cotton fiber length is four to ten times higher than the selected seed fibers. Thus, it indicates that the fibers are not suitable for spinning. Cotton fiber density and moisture content was found to be similar to the selected seed fibers; however, the whiteness index of all the fibers found inferior to cotton and Tellicherry bark and all of them have a yellowish tint (Pandey, Jose, and Sinha Citation2022). The highest whiteness index was registered with Poplar (P. Ciliata) fiber (17.8) and least with Albizia (−201.9).
Table 3. Comparative chart of physical properties of selected fibers used and popular seed fibers (Sinha and Pandey Citation2024).
Scanning electron microscopy
The surface morphology of the fiber samples was analyzed through SEM and is shown in . SEM micrograph shows a large surface area of microfibers along its length. Fibers collected from bean-shaped seedpods (Adenium and Blackboard) appear continuous, unbound, clean, uniform, and rod like. Albizia fiber micrograph appearance is curly shaped and in loosely packed bundles. Poplar and inflorescence microfibers having variable thickness along its length are segregated from each other. From , it is depicted that all the fibers have rod-like structure. In the Plume thistle fiber, the SEM images detected channel-like structure with variation in fiber diameter, whereas in the remaining three fibers, the diameter appears uniform. Fibers are small in size (2.0–6.4 µm) and length (2.2–7.6 mm). Fiber images are clean and continuous. ImageJ analysis provided a deeper understanding of the fiber topography.
The diameter and morphology of fiber particles has been analyzed by ImageJ software. The Adenium fiber is having micro strip kind of structure, which accumulates and form the rod shape with strips on the top. The diameter of the rod shape is 18.59 μm with average diameter of 3.846 μm strips on the surface (). However, Albizia is having dreadlocks structure, in which the single fiber is having average diameter of 2.025 μm and the bunches have 18.59 μm diameter (). The is of Blackboard tree, which is showing a nice flat strips kind of structure with a width of 16.66 μm. The structure of Munja fiber is very much similar to rod with an average diameter of 6.4 μm shown in .
is showing the SEM images of Plume thistle (), Poplar (), Reed (), and Wild sugarcane fibers (). All the fibers are having almost similar structure of rod shape with almost same diameter except Poplar. The Poplar is having rod shape with different diameters range from 3.205 μm to 8.974 μm, whereas the Plume thistle, Reed, and Wild sugarcane average diameter were 5.12, 5.77, and 5.128 μm, respectively.
Chemical characterization
The chemical component analysis of the seed fibers is shown in . It is observed from the that all the eight seed fibers are having good cellulosic content, which exceeds 50%. The highest cellulosic content was observed with Plume thistle and Adenium (66%) and the minimum with Poplar (P. deltoid) (51%). The highest and least lignin content was registered with Albizia (24.3%) and Blackboard tree (7.6%), respectively. The rest of the fibers have a lignin content in the range of 15–25%. Cotton has higher amount of cellulose and free from lignin and hemicelluloses in comparison to selected seed fibers (). Except Blackboard tree fiber, all the fibers have less wax content than cotton (Dorez et al. Citation2014). Presence of waxes (0.7–4.5%) affects the fiber color stability and enhances further processing (Gurarda et al. Citation2013).
Table 4. Comparative chart of chemical composition of selected seed fibers and popular seed fibers (Richard et al. Citation2019; Sinha and Pandey Citation2024).
Thermogravimetric analysis
Thermogram of the samples is given in the . For all the samples, three distinct regions were observed. At 105°C, all the fibers show similar weight loss due to the removal of moisture in the fibers. However, in the case of Blackboard fiber, less weight loss (3.13%) was observed than other fibers. Most of the fiber started to degrade after 240°C. Cellulose degradation of all fibers started at 240°C and continued till 325°C, which resulted in more weight loss. Final residual mass (800 ℃) for Munja fiber was found to be very less (10.88%), while it was highest in case of Reed fiber (24.36%). This higher residual mass is attributed due to the presence of high mineral matter in Reed fiber. In all three stages of degradation, Munja, Wild sugarcane, and Poplar fiber has minimum residual loss compared to other fibers. These three fibers have wax content less than 1% (). This could be the reason of their higher thermal stability. Adenium and Plume thistle having highest cellulose content exhibited minimum residual loss at second stage (400 °C) of degradation.
Figure 4. Fiber thermogram: (a) Adenium, (b) Albizia, (c) Blackboard tree, (d) Munja, (e) Plume thistle, (f) Poplar, (g) Reed, and (h) Wild sugarcane fiber.
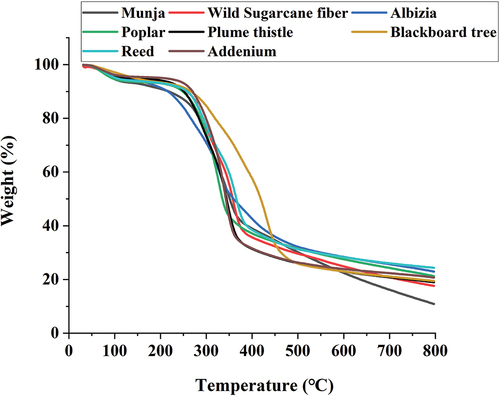
The first derivative of thermogram, i.e., derivative thermogravimetry (DTG) curves, provides valuable insights into the thermal degradation behavior of lignocellulosic materials, enhancing their characterization. The DTG curves of eight lignocellulosic seed fibers are presented in . Notably, Munja, Wild sugarcane, and Reed fibers exhibit distinct patterns compared to other fibers. These fibers display separate peaks corresponding to the decomposition of cellulose and hemicellulose. Conversely, Adenium, Albizia, Blackboard tree, Plume thistle, and Poplar fibers show a single peak, indicating the merging of hemicellulose and cellulose decomposition. No discernible peak for lignin was observed, which typically manifests above 440°C; this absence may be attributed to the experimental conditions involving an inert atmosphere with nitrogen gas. Furthermore, it was observed that the peak decomposition temperature is influenced by the cellulose content of the respective fiber. Fibers with higher cellulose content exhibits higher peak decomposition temperatures. The ascending order of peak temperatures observed is as follows: Poplar (327.9°C) < Albizia (330.3°C) < Adenium (341°C) < Plume thistle (346.4°C) < Munja (346.7°C) < Wild sugarcane (349.4°C) < Reed (363.7°C) < Blackboard tree (363.8°C).
Fourier transform infrared spectroscopy
Fourier transform infrared spectroscopy (FTIR) spectrum of all fibers was analyzed using attenuated total reflectance (ATR) assembly, and the corresponding spectra is given in the . The spectrum of all fibers exhibited almost similar peaks; however, depending upon the chemical composition, the intensity of the peaks varies. All the fibers showed the characteristics peaks of three natural polymers, namely cellulose, lignin and xylan. The spectra had broad band at 3300 and 2890–2900 cm−1 that correspond to stretching of hydrogen bonded –OH groups and CH2 units C-H stretching absorption at 2890 cm−1 of cellulose polymer of three fibers (Pandey, Jose, and Sinha Citation2022 a). Adenium fiber had strong peak at 1738 cm−1 due to the presence of higher amount of hemi cellulose. The sharp peak at 1029 cm−1 is due to the presence of C–O stretching of cellulose, hemicellulose, or lignin. The peak at 1238 cm−1 is assigned for –C–O–C and –C=O stretching in xylan side substituent and lignin aromatic C=O stretching (George et al. Citation2023; Singh et al. Citation2022)
X-ray diffraction analysis
The X-ray diffraction (XRD) patterns were measured for raw cotton fibers and their respective nanofibers with an X-ray diffractometer. The X-ray diffraction (XRD) patterns were measured for all eight seed fibers and is depicted in . In all the fibers, the diffractograms display a prominent peak of (200), which shows the characteristic of cellulose-I peak. The peak of (200), present at 2θ = 22.6 for Adenium, 2θ = 22.4 for Albizia, 2θ = 22.6 for Blackboard tree, 2θ = 22.5 for Munja, 2θ = 22.5 for Plume thistle, 2θ = 22.6 for poplar, 2θ = 22.5 for Reed, and 2θ = 22.4 for Wild sugarcane. This (200) peak in the Wilds sugarcane is much sharper as compared to other fiber’s XRD, which signifies higher crystallinity in nature of cellulose-I. Using Segal equation, the crystallinity index (%) for the different natural fibers is as follows: Wild sugarcane (55.57%), Munja (49.1%), Adenium (19.22%), Poplar (29.92%), Albizia (43.18%), Plum thistle (54.99%), Reed (55.69%), and Blackboard Tree (55.57%). The values indicates that the Wild sugarcane, Reed, Plum thistle, and Blackboard tree are showing higher crystalline (approximately 55%) over Munja, Poplar, Albizia, and Adenium. Overall, it can be observed in all eight fibers XRD that the nature of all fibers is more amorphous with little crystalline structure. There are multiple peaks available in all the fibers, which suggest presence of small amount of cellulose, lignin variants (Gong et al. Citation2017). The results of XRD and TGA analysis suggests that all eight fibers can be classified into two groups based on their characteristics. Fibers exhibiting higher crystallinity levels (49 to 55%) and better thermal stability (346 to 364 °C) include Plume thistle, Munja, Wild sugarcane, Reed, and Blackboard tree. On the other hand, fibers with lower crystallinity (19 to 43%) and reduced thermal stability (328 to 341 °C) consist of Adenium, Poplar, and Albizia. Furthermore, it is evident that the cellulose content in the fibers plays a significant role in influencing their crystallinity property. Adenium, Poplar, and Albizia fibers, which exhibit lower crystallinity, also have lower cellulose content compared to the other fibers.
Conclusion
The research is to give recognition to lesser-known fiber yielding wild flora to enable their proper utilization in technical textile segments. Fiber collection from selected species was simple, and no further chemical or mechanical processing was required to utilize them further. Comprehensively, the selected seed fibers are comparable to cotton in fineness, density, moisture, and wax content. Fiber length and cellulose content of seed fibers is lesser than cotton fibers. The less fiber length of selected seed fibers cause difficulty in spinning but make them usable in paper and anthropogenic cellulosic fiber industry. Thus, the application includes filler for mattress and pillows, preparation of paper, and composites. Abundant availability, ease of fiber collection, bulkiness, free from impurity, low density, thermal stability, and semi-crystalline structure of seed microfibers make them a promising fiber for insulation, paper, and composite industry. Poplar fibers were the whitest of all, whereas Adenium, Albizia, and Blackboard tree fibers were tinted with yellow to brown color. The chemical composition analysis revealed that the fibers are lignocellulosic. The SEM analysis showed the fine rod-like structure of the fibers. The X-ray diffraction analysis reveals the amorphous and crystalline nature of the fibers. The thermal stability in co-relation with the chemical composition has been revealed by TGA and DTG analysis. It is concluded that the characterization study of the selected seed fibers detailed in this research article could open a better opportunity for their utilization.
Highlights
Eight unreported seed fibers namely adenium, Albizia, blackboard tree, munja, poplar, plume thistle, reed, and wild sugarcane were characterized for their physical and chemical properties.
All the selected seed fibers were found to be fine and less dense than cotton.
The chemical composition analysis revealed that the fibers are lignocellulosic.
The SEM analysis showed the fine rod-like structure of the fibers. The X-ray diffraction analysis reveals the amorphous and crystalline nature of the fibers.
Author contribution
RP conceptualized the study and did preliminary work to form a base. Chemical characterization and work on figures was elucidated by AD, GKP, AA, and ASM. Physical characterization of the fibers was accomplished by RD and MKS. SJ provided the technical guidance and a final shape to the paper.
Ethical approval
We confirm that all the research meets ethical guidelines and adheres to the legal requirements of the study country. The research does not involve any human or animal welfare related issues.
Acknowledgments
The authors would like to express their gratitude to their respective institute for providing facilities to conduct the present research.
Disclosure statement
No potential conflict of interest was reported by the author(s).
References
- Adhia, V., A. Mishra, D. Banerjee, A. N. Appadurai, P. Preethan, Y. Khan, D. DeWagenaar, et al. 2021. Spinning Future Threads: The Potential of Agricultural Residues as Textile Fiber Feedstock. Vermont, USA: Institute for Sustainable Communities.
- da Silva, L. S., M. M. Biondo, B. D. A. Feitosa, A. L. Rocha, C. D. C. Pinto, S. X. Lima, C. D. L. Nogueira, et al. 2022. “Semiconducting Nanocomposite Based on the Incorporation of Polyaniline on the Cellulose Extracted from Bambusa Vulgaris: Structural, Thermal and Electrical Properties.” Chemical Papers 76 (1): 1–13. https://doi.org/10.1007/s11696-021-01844-z.
- Dorez, G., L. Ferry, R. Sonnier, A. Taguet, and J. M. Lopez-Cuesta. 2014. “Effect of Cellulose, Hemicellulose and Lignin Contents on Pyrolysis and Combustion of Natural Fibers.” The Journal of Analytical and Applied Pyrolysis 107:323–331. https://doi.org/10.1016/j.jaap.2014.03.017.
- George, S., S. Thomas, N. NandananNedumpillil, and S. Jose. 2023. “Extraction and Characterization of Fibers from Water Hyacinth Stem Using a Custom-Made Decorticator.” Journal of Natural Fibers 20 (2): 2212927. https://doi.org/10.1080/15440478.2023.2212927.
- Geremew, A., P. DeWinne, T. Adugna, and H. DeBacker. 2021. “An Overview of the Characterization of Natural Cellulosic Fibers.” Key Engineering Materials 881:107–116. https://doi.org/10.4028/www.scientific.net/KEM.881.107.
- Gong, J., J. Li, J. Xu, Z. Xiang, and L. Mo. 2017. “Research on Cellulose Nanocrystals Produced from Cellulose Sources with Various Polymorphs.” RSC Advances 7 (53): 33486–33493. https://doi.org/10.1039/C7RA06222B.
- Gurarda, A., E. Yukseltan, B. M. Kaplangiray, and M. Kanik. 2013. “The Effects of Various Lubricants on the Friction Properties of Sewing Threads.” Textile Research Journal 83 (12): 1273–1282. https://doi.org/10.1177/0040517512470199.
- Kumar, T. S. M., N. Rajini, M. Jawaid, A. V. Rajulu, and J. W. Jappes. 2018. “Preparation and Properties of Cellulose/Tamarind Nut Powder Green Composites.” Journal of Natural Fibers 15 (1): 11–20. https://doi.org/10.1080/15440478.2017.1302386.
- Lee, C. H., A. Khalina, and S. H. Lee. 2021. “Importance of Interfacial Adhesion Condition on Characterization of Plant-Fiber-Reinforced Polymer Composites: A Review.” Polymers 13 (3): 438. https://doi.org/10.3390/polym13030438.
- Muthusamy, A. R., S. M. K. Thiagamani, S. Krishnasamy, C. Muthukumar, S. M. Rangappa, and S. Siengchin. 2022. “Lignocellulosic Microfibrils from Phaseolus Lunatus and Vigna Radiata Biomass: Characterization and Properties.” Biomass Conversion and Biorefinery: 1–9. https://doi.org/10.1007/s13399-022-03428-7.
- Pandey, R., S. Jose, G. Basu, and M. K. Sinha. 2021. “Novel Methods of Degumming and Bleaching of Indian Flax Variety Tiara.” Journal of Natural Fibers 18 (8): 1140–1150. https://doi.org/10.1080/15440478.2019.1687067.
- Pandey, R., S. Jose, and M. K. Sinha. 2022. “Fiber Extraction and Characterization from Typha domingensis.” Journal of Natural Fibers 19 (7): 2648–2659. https://doi.org/10.1080/15440478.2020.1821285.
- Pandey, R., G. K. Prasad, A. Dubey, A. Arputhraj, A. S. M. Raja, M. K. Sinha, and S. Jose. 2022. “Tellicherry Bark Microfiber: Characterization and Processing.” Journal of Natural Fibers 19 (16): 13288–13299. https://doi.org/10.1080/15440478.2022.2089432.
- Pandey, R., M. K. Sinha, and A. Dubey. 2023. “Macrophyte and Wetland Plant Fibers.” In Sustainable Fibers for Fashion and Textile Manufacturing, edited by R. Nayak, 109–127. United Kingdom: Woodhead Publishing. https://doi.org/10.1016/B978-0-12-824052-6.00006-8.
- Prasad, G. K., V. Mageshwaran, R. Guruprasad, A. Arputhraj, T. Senthilkumar, P. G. Patil, and R. K. Jadhav. 2018. “Mechanical and Chemical Processability of Cotton/Kapok Blends.” Cotton Research Journal 9:24–26.
- Rajini, N., A. Alavudeen, V. Siengchin, S. Rajulu, N. Ayrilmis, and N. Ayrilmis. 2021. “Development and Analysis of Completely Biodegradable Cellulose/Banana Peel Powder Composite Films.” Journal of Natural Fibers 18 (1): 151–160. https://doi.org/10.1080/15440478.2019.1612811.
- Ramalingam, K., S. M. K. Thiagamani, H. Pulikkalparambil, C. Muthukumar, S. Krishnasamy, S. Siengchin, A. M. Alosaimi, M. A. Hussein, and S. M. Rangappa. 2023. “Novel Cellulosic Natural Fibers from Abelmoschus Ficulneus Weed: Extraction and Characterization for Potential Application in Polymer Composites.” Journal of Polymers and the Environment 31 (4): 1323–1334. https://doi.org/10.1007/s10924-022-02687-9.
- Richard, C., P. Cousin, M. Foruzanmehr, S. Elkoun, and M. Robert. 2019. “Characterization of Components of Milkweed Floss Fiber.” Separation Science and Technology 54 (18): 3091–3099. https://doi.org/10.1080/01496395.2018.1556691.
- Riyaz, M., S. Ignacimuthu, and S. M. Zuber. 2020. “Biosciences and Plant Biology.” International Journal of Current Research in Biosciences and Plant Biology 7:64–68. https://doi.org/10.20546/ijcrbp.2020.706.007.
- Singh, G., S. Jose, D. Kaur, and B. Soun. 2022. “Extraction and Characterization of Corn Leaf Fiber.” Journal of Natural Fibers 19 (5): 1581–1591. https://doi.org/10.1080/15440478.2020.1787914.
- Sinha, M. K., and R. Pandey. 2024. Non-Metallic Technical Textiles: Materials and Technologies. Boca Raton, FL: CRC Press.
- Thiagamani, S. M. K., P. Sivakumar, M. Srinivasan, S. N. B. Yagna, E. K. Hossein, M. Meena, S. M. Rangappa, and S. Siengchin. 2023. “Isolation and Characterization of Agro-Waste Biomass Sapodilla Seeds as Reinforcement in Potential Polymer Composite Applications.” Heliyon 9 (7): e17760. https://doi.org/10.1016/j.heliyon.2023.e17760.