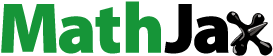
ABSTRACT
There is a growing demand for high-quality natural fibers, including flax fibers, which possess bioactive compounds with antioxidant, antibacterial, and anti-inflammatory properties. Traditional methods like dew retting are commonly used for flax fiber production, but alternative approaches are sought for enhanced fiber quality. This study presents a novel method involving cottonization to improve the quality of flax fibers obtained from low-quality decorticated fibers. The research evaluates the quality enhancement through various stages of the new method, comparing it with dew retting. Studies conducted on both transgenic (B14) and non-transgenic flax fibers revealed a decrease in fiber polymers and bioactive compounds after cottonization compared to dew retting. However, despite this reduction, the antioxidant potential of cottonized fibers increased significantly. Moreover, cottonized flax fibers exhibited high antimicrobial activity, particularly against Pseudomonas aeruginosa. Furthermore, extracts from both transgenic and non-transgenic cottonized flax fibers showed cytotoxic effects on hamster lung fibroblast cells, indicating potential future applications in cancer research. These findings highlight the potential of cottonization in enhancing the quality and bioactivity of flax fibers, paving the way for further exploration in various applications, including medical and textile industries.
摘要
人们对包括亚麻纤维在内的高质量天然纤维的需求越来越大,亚麻纤维具有抗氧化、抗菌和抗炎的生物活性化合物. 传统的方法,如露水脱胶,通常用于亚麻纤维生产,但也寻求其他方法来提高纤维质量. 本研究提出了一种新的方法,包括棉化处理,以提高从低质量的脱胶纤维中获得的亚麻纤维的质量. 研究通过新方法的不同阶段对质量提高进行了评估,并将其与露水脱胶进行了比较. 对转基因(B14)和非转基因亚麻纤维进行的研究表明,与脱胶相比,棉花化后纤维聚合物和生物活性化合物减少. 然而,尽管这种减少,棉化纤维的抗氧化潜力显著增加. 此外,棉化亚麻纤维表现出很高的抗菌活性,特别是对铜绿假单胞菌的抗菌活性. 此外,转基因和非转基因棉化亚麻纤维的提取物对仓鼠肺成纤维细胞具有细胞毒性作用,表明其在癌症研究中的潜在应用前景. 这些发现突出了棉花化在提高亚麻纤维质量和生物活性方面的潜力,为进一步探索包括医疗和纺织行业在内的各种应用铺平了道路.
Introduction
Flax (Linum usitatissimum L.) is one of the oldest plants used by humans for fiber production (Kozasowski, Mackiewicz-Talarczyk, and Allam Citation2012; Melelli et al. Citation2021). Today, the fibers originating from flax are still in use due to their quality, durability, and bio-medical properties (Akin Citation2013; Skórkowska-Telichowska et al. Citation2010). However, the method of obtaining flax fiber requires the separation of bast fiber from non-fiber components, and is traditionally achieved through dew retting processes. This renders flax processing disadvantageous, as the presence of fiber non-cellulosic constituents such as lignin, pectin, and hemicelluloses negatively influences the mechanical properties of fibers (Huo et al. Citation2013). The reason for this lies in the fact that cellulose is the strongest constituent in flax fiber (Yan, Chouw, and Jayaraman Citation2014). Currently, different methods are applied for obtaining improved homogeneous fibers without impurities, among them there are retting (including enzymatic retting) (Akin Citation2013; Chabbert et al. Citation2020), decortication, degumming (Zimniewska et al. Citation2022) and cottonization (Antonov et al. Citation2007).
Retting is a stage of processing flax straw, which consists in detaching bast fibers from the woody part of the stem. A commonly used method of flax retting is the “dry” method, also known as the dew retting method, in which the flax straw is spread out in the field, where soil microorganisms degrade the pectin, releasing bundles of fibers from the remaining parts of the straw (Wu et al. Citation2023). Only then can the fiber, initially separated from the wood, be subjected to further processing (staking, beating). The factors limiting this process are climatic conditions (humidity and temperature) and the degree of lignification of the cell wall. Too dry retting conditions affect the limited development of soil microorganisms, and thus slow down the pectin degradation process, while too high humidity can cause the development of cellulolytic microorganisms, and thus the obtained fiber will be of poorer quality, or it can lead to straw and fiber rotting (Akin Citation2013). Because of the disadvantageous lignification of the cell walls of the stems, flax intended for retting is harvested on the 103th day of its vegetation. At this stage of development, it is characterized by a relatively low degree of lignification (Henriksson et al. Citation1997) and sufficient development for obtaining fiber. However, the flax fiber obtained by retting methods is non-uniform and varies in terms of quality, which makes it difficult to use it for the production of high-quality linen products. In addition, these processes are time-consuming and labor intensive. Therefore, the replacement of this stage of flax straw processing with decortication and further modifications could contribute to the production of flax fiber with properties and alternative quality to the raw material produced so far, significantly expanding its multidirectional application possibilities.
The decortication process is a mechanical way of separating the flax fiber from the woody part of the plant by breaking, beating, or crushing, during which the fiber is initially split and partially shortened. As a result, a monomorphic fiber is obtained, but of low quality, thus successive steps of physical and mechanical processing of the material are necessary. The key benefit of decortication is not only the elimination of retting during the processing of flax straw but the possibility of obtaining a fiber of repeatable quality. The decortication technology does not require complicated lines of processing equipment, which significantly reduces the cost of fiber extraction and is limited to only one aggregate. During this process, the fiber can be damaged by mechanical defiberization, but due to the fact that the flax fiber is very durable, these damages are small (Amel et al. Citation2013). The decorticated fiber differs from conventional fiber by the presence of pectic substances that are not hydrolyzed. Therefore, it is necessary to carry out further modifications of the fiber, both physical and mechanical, in order to obtain fiber with alternative properties.
The osmotic modification process consists in physically detaching the phloem from the decorticated fiber in an aqueous environment using hydrodynamic defibration and temperature, which results in the diffusion of water molecules into the stem. Swelling under the influence of water tears the bast, which detaches from the fiber. At the same time, pectin in the cell wall is forced out by pressure, where they are washed away by water. Thus, it is a process that allows the extraction of the fiber without interfering with its natural properties. As a consequence, very good-quality fiber is extracted. It is an environmentally friendly process as the waste and odor that accompanies the retting process is eliminated (Różańska et al. Citation2012).
Cottonization of flax fiber is a chemical and mechanical process that aims to transform flax fibers into white, softer and more processable form, similar to cotton. This process is used to improve the properties of linen yarn, such as strength, softness, and elasticity, which enables the production of linen fabrics of better quality. Effective cottonization process of flax fibers was shown to obtain high quality of fibers without retting process, which led to shorter processing (Zimniewska et al. Citation2017). The degumming and cottonization processes are performed to obtain more homogeneous flax fibers, which can be industrially processed as cotton (Kozłowski et al. Citation2012), yet possessing superior properties due to the presence of bio-active compounds. Among these compounds, polyphenols, terpenoids, or polyamines with antioxidant, antibacterial, and anti-inflammatory properties are of high value especially in bio-medical applications of flax fibers, like production of dressings and bandages for the treatment of difficult to heal wounds, biodegradable packaging, composites for the production of scaffolds in tissue engineering and production of implants and surgical threads. Micronized fiber can be used as a carrier and stabilizer of active substances such as drugs, vitamins, and hormones (Czemplik et al. Citation2011; Dymińska et al. Citation2013). In our previous reports, we described genetically modified flax lines that were produced in order to further increase the bio-medical properties of flax fibers. They were successfully used for the production of medicinal materials (Kulma et al. Citation2015; Skórkowska-Telichowska et al. Citation2010).
In this study, we investigated the influence of the new method of fiber extraction on the properties of the fiber, including those resulting from the presence of bio-active compounds. Therefore, the subject of our investigations was not only the wild-type fibrous flax (var. Nike) but also a transgenic flax line bearing the β-1,3-glucanase gene from potato, and characterized by changed contents of bioactive compounds, more polyamines, and consequently a higher antioxidant capacity (B14 line) (Wojtasik et al. Citation2013, Citation2017; Wróbel-Kwiatkowska et al. Citation2004).
Our thesis was that the new method using decortication, osmosis, and cottonization should provide higher-quality flax fibers that could be processed like cotton but with the retained bio-activity. We have evaluated the content of bioactive compounds, cell wall composition, and biological properties of flax fibers after each processing stage, i.e. after decortication, wet degumming, and cottonization, and finally, we have compared the quality of flax fiber obtained with the new technology with flax fiber obtained after dew retting. In addition, we used flax straw from B14 transgenic flax with changed characteristics and transgenic flax to check how different methods of obtaining flax fiber affect its composition and properties.
Materials and methods
Research material
Two types of flax plants (Linum usitatissimum L.), namely Nike (fibrous cultivar) and B14 (genetically modified Nike), were used in the study. The Nike flax seeds were obtained from the flax and hemp collection of the Institute of Natural Fibers and Medicinal Plants-National Research Centre (INF&MP-NRC) of Poland. The B14 transgenic flax was obtained previously by the transformation of Nike cultivar using the plasmid pGAglubsens, containing cDNA encoding β-1,3-glucanase from potato (GenBank: AJ586575.1) under a 35S CaMV promoter and Nos terminator (Wróbel-Kwiatkowska et al. Citation2004). Flax cultivation was carried out at the Institute of Soil Science and Plant Cultivation (IUNG) in Puławy (Poland) with an experimental station in Jelcz-Laskowice (Poland). Both types were sown on an area of 1000 m2 in the experimental field of Wroclaw University, Lower Silesia, Poland, and current agrotechnical recommendations were implemented during their cultivation.
Dew retting method
After harvesting the plants on the 103rd day of cultivation, the plants were retted by the dew method (Akin Citation2013; Preisner et al. Citation2014) as described in (Wróbel-Kwiatkowska et al. Citation2007). The flax plants were spread out in a cultivation field for 6 weeks, the plants were turned every 14 days. During this time microorganisms from the field (bacteria, fungi) grew on the plants and released the fibers from the flax stems (Musialak et al. Citation2008). The average air temperature was 15.8°C and rainfall was about 65 mm. After retting, the straw was dried again and subjected to mechanical processing in order to separate the fiber and pre-clean it.
New approach for producing flax fiber
The process of obtaining flax fiber involved three main stages: decortication, degumming, and cottonization. Initially, the dry flax straw underwent decortication, resulting in a fragmented fiber type with high impurity content. The flax fiber decortication process involves a series of interconnected devices arranged in a technological line. This line executes successive cycles of breaking, shaking, and scutching to separate the fibers. The decortication trials were carried out at the Experimental Plant of INF&MP “Lenkon.” To improve the fiber quality, the second stage employed osmotic degumming in a closed device with adjustable pressure, followed by ultrasonic treatment in an open device.
The degumming process of flax fiber encompassed varying parameters such as the temperature of the flowing water (30°C or 40°C) and the duration of the process (24 h or 48 h). After the degumming process, the fiber was extracted from the cylinder and rinsed at 30°C and 40°C. Upon completion of the cycle, during which the fiber was exposed to flowing water at specific temperatures and durations, the fiber was extracted from the rotating cylinder and excess water was removed using a laboratory wringer. Subsequently, the wet fiber was manually loosened to prevent re-adhesion during the drying process. Drying was carried out in a laboratory dryer with controlled airflow at 60°C for all samples. The optimal conditions for the wet degumming process (fiber degumming at 30°C for 24 h and rinsing at 40°C) were selected based on previous research (Wojtasik et al. Citation2023). Subsequently, in the third stage, mechanical treatment was carried out on a carding machine adapted to the needs of the technology in order to cottonize the decorticated fibers (Jóźwik et al. Citation2017). Notably, ultrasound was exclusively used during the wet degumming step, while the final cottonization was achieved mechanically without the need for additional equipment. For a comprehensive understanding of this innovative flax fiber extraction technology, Zimniewska et al. (Citation2017) provided a detailed description.
Determination of phenolic compound content
Phenolic compound determination was performed with UPLC-MS according to Mierziak et al. (Citation2014). Briefly, 150 mg of flax fibers ground in a Retch mill were used for the extraction with methanol in an ultrasonic bath, centrifuged, dried, and re-suspended in pure methanol. Acquity UPLC BEH C18 (2.1 × 100 mm, 1.7 μm) column was used for compound separation. The separation conditions are presented in .
Table 1. UPLC separation conditions of phenolic and terpenoid compounds.
Determination of terpenoid compound content
The content of terpenoids was determined according to Boba et al. (Citation2018) from 150 mg of ground flax fibers. The fiber material was submitted to extraction sequentially with acetone, petroleum ether, and diethyl ether for 10 min in an ultrasonic bath, and centrifuged. The supernatants were dried and re-suspended in methanol:acetonitrile mixture (1v : 1v). The samples were then analyzed on UPLC with a 2996 PDA diode detector (Waters Acquity UPLC System) on a BEH C18 column, 2.1 × 100 mm, 1.7 μm (Waters Acquity). The separation conditions are presented in .
Determination of polyamine content
Polyamines were assayed with the method described previously by (Imai et al. Citation2004) with some modifications (Wojtasik et al. Citation2015). Flax fibers (100 mg) were ground in a Retsch mill and used for the extraction. Putrescine, spermidine, and spermine were determined in the free (soluble) polyamine and cell wall-bound polyamine fractions.
Determination of the antioxidant activity of flax fiber extracts
DPPH and FRAP methods were employed for the determination of the antioxidant potential of flax fiber methanol extracts. In the DPPH (1,1-diphenyl-2-picrylhydrazyl) method, first described by Brand-Williams, Cuvelier, and Berset (Citation1995), 0.2 mL of 0.1 mM DPPH methanol solution was added to 30 µL of flax fiber methanol extract. After 15 min, the absorbance at 515 nm was measured. The effect of the extracts on the inhibition of the formation of DPPH• radicals was estimated according to the equation:
In the FRAP (Ferric Ion Reducing Antioxidant Power) method, described by Benzie and Strain (Citation1996), 0.2 mL of FRAP reagent (300 mM acetate buffer pH 3.6, 20 mM FeCl3, 10 mM 2,4,6-tripyridyl-s-triazine, mixed in 10:1:1 volume ratio) were added to 6 μl of the extract. The samples were incubated at 37°C for 4 min. Absorption was measured at 595 nm. The antioxidant potential was calculated as the difference in the absorbance read at time 0 and after 4 min of incubation concerning the control, which was equal to 1.
Determination of cell wall structure components
Cellulose and lignin contents were assayed in 15 mg of dry flax fibers ground to powder. For the determination of cellulose, the fiber powder was first hydrolyzed with sulfuric acid, and then the anthrone method was employed as described by (Ververis et al. Citation2004). Total lignin content was determined with the acetyl bromide method, as described by (Iiyama and Wallis Citation1990).
For the isolation and fractionation of pectin and hemicellulose a modified version of the method described by Manganaris and Vincente was used (Manganaris et al. Citation2008). Any modifications applied to the method were described previously (Wojtasik et al. Citation2016). To release the polymers from the cell wall 1.5 g of dry, ground flax fibers were used. The resulting precipitate containing alcohol-insoluble components (AIR) was used for further assays. Three hundred milligrams of AIR was used to fractionate pectin and hemicelluloses. Three pectin fractions were obtained: a water-soluble fraction (WSF), a CDTA-soluble fraction (CSF) (CDTA − trans-1,2-diaminocyclohexane-N,N,N,N-tetraacetic acid), and an Na2CO3 and NaBH4 soluble fraction (NSF). Additionally, two hemicellulose fractions were obtained: 1 M KOH with 20 mM NaBH4 soluble fraction (K1SF) and 4 M KOH soluble fraction (K4SF).
The content of uronic acids was determined using the biphenyl method (Blumenkrantz and Asboe-Hansen Citation1973) after hydrolysis of the polysaccharides in sulfuric acid (Ahmed and Labavitch Citation1978) with some modifications (Wojtasik et al. Citation2013). The content of total soluble sugars was determined using the phenolic method after hydrolysis of the polysaccharides in sulfuric acid (Ahmed and Labavitch Citation1978) with some modifications (Wojtasik et al. Citation2016).
The content of total pectin was estimated as the sum of the uronic acids and other monosaccharides from three pectin fractions (WSF, CSF, and NSF) and the content of total hemicellulose was estimated as the sum of the uronic acids and other monosaccharides from two hemicellulose fractions (K1SF and K4SF).
Determination of fatty acid content
The analysis of fatty acids in the flax fiber was done according to (Mierziak et al. Citation2014). Fatty acids were converted into their methyl esters and analyzed using the GC-FID method.
Determination of phytosterol content
The flax fiber was subjected to a two-step extraction process using 10 mL of hexane each time. The mixture was vigorously vortexed for 15 min during each extraction. After the extractions, the resulting solutions were combined, and the solvent was removed by evaporation under nitrogen at 25°C until dryness was achieved. Trimethylsilyl derivatives of sterols were prepared (Shukla, Dutta, and Artz Citation2002) and analyzed with a 6890 N gas chromatograph equipped with an FID detector and the capillary column HP-5 30 m × 0.32 mm × 0.25 μm (Agilent Technologies, USA) using helium as a gas carrier, 5-cholestane as an internal standard for quantitative analysis, and ChemStation v. B.04.02 for calculation of the results.
Determination of the antimicrobial activity of flax fibers
To determine the antimicrobial activity of flax fiber extracts, the method described by (Zuk et al. Citation2014) with modifications was used. For the preparation of extracts, 0.25 g of powdered fibers (milled in Retch Mill) were suspended in 1 mL of methanol and submitted to an ultrasonic bath for 15 min. Then, after centrifugation (10 min. 12,000 × g, 4°C), the supernatant was poured into a separate probe, and the pellet was treated in the same manner two more times. The supernatants were pooled and dried under nitrogen flow – methanol fraction. The pellets were hydrolyzed overnight in 2 M NaOH at 37°C, acidified with HCl to pH 3, and extracted to ethyl acetate (1v:1v). The samples were then dried under nitrogen flow – hydrolyzed fraction. Both fractions were then resolved in 1 mL of sterile water. The microorganisms (S. aureus, E. coli, P. aeruginosa, C. albicans) were grown from a single colony overnight in LB medium, and fresh cultures were inoculated and grown to 108 cells per 1 mL. Then, 50 µL of LB, 50 µL of microorganisms from the fresh cultures, and 11 µL of extract were mixed and left for 6 h growing. After this time, the cultures were poured onto solid LB media, and the numbers of colonies were counted on the next day.
Determination of the effect of flax fiber extracts on V79 hamster fibroblast
Two extracts (methanol fraction and hydrolyzed fraction) were prepared analogously as described for antimicrobial activity determination. The material weight was 1 g – extracted to 10 mL of methanol (3 times), and the volume of water for the final suspension of the dried fractions was 1.2 mL. The pellet was hydrolyzed in 10 mL of 2 M KOH for 48 h. The detection of phenolic compounds was carried out using the UPLC method described earlier (Wojtasik et al. Citation2013).
Culture conditions of the hamster lung fibroblast V79 model cell line
The culture conditions of the V79 cell line were previously described by Wojtasik (Wojtasik et al. Citation2023). The V79 cell line was cultured under sterile, controlled conditions (37°C, 5% CO2) in a DMEM medium supplemented with 10% bovine serum, 100 U/mL penicillin, and 100 g/mL streptomycin. The cells were passaged after they covered 90% of the bottle surface. For trypsinization, 0.05% trypsin/1 mM EDTA in PBS was added, and after the cells detached, a fresh medium (5 times more by volume) was added to stop trypsinization. Cells were centrifuged at 800 × g for 5 min and then added to a fresh medium (approximately 7–10 times diluted).
Treatment of V79 hamster lung fibroblast cell lines with aqueous extracts of flax fibers
The V79 cell lines were cultured for 24 h in 24-well plates before the addition of 10 µL, 25 µL, or 50 µL the flax fiber extracts (obtained from Nike (control) and B14 flax line) in triplicate. After 24 h of incubation, the MTT test was performed for the viability assay.
For the transcript level analysis, V79 cell lines were cultured for 24 h in 6-well plates. Then, 125 µL of Nike and B14 flax fiber extracts were added into well plates in triplicate. After 24 h of incubation, the medium was removed, and RNA was isolated from the cells.
MTT cell viability assay
To determine cell viability, the MTT method with modifications previously described by Wojtasik was used (Wojtasik et al. Citation2023). Fifty microliters of MTT (3-[4,5-dimethylthiazol-2-yl]-2,5 diphenyl tetrazolium bromide) reagent (4 mg/mL) per 0.5 mL of medium was added to each well (treated with extracts and controls) and incubated for 4 h. Then, the medium was removed, and the cells were incubated for 30 min in 0.5 mL of DMSO to dissolve the formed formazan crystals. The absorbance of the obtained colored solution was quantified by measuring absorbance at 540 nm in an Asys UVM340 plate reader (Biochrom, UK). The experiment was performed in three biological repetitions.
Transcript level analysis
The level of gene transcripts was determined using the qPCR method (Wojtasik et al. Citation2023). Total RNA was isolated from the V79 cells using the RNeasy Plus Mini Kit (Qiagen) according to the producer’s protocol. The concentration and purity of the isolated RNA were checked spectrophotometrically (at 260 nm and 260/280 nm, respectively). The DNase I reagent kit (Invitrogen) was used to remove DNA contamination, according to the manufacturer’s protocol. Synthesis of cDNA was performed with the High Capacity cDNA Reverse Transcription Kit (Life Technologies) according to the manufacturer’s instructions. The real-time PCR was performed in the StepOnePlus™ Real-Time PCR Systems thermal cycler (Applied Biosystems). DyNAmo SYBR Green qPCR Kit (Thermo Scientific) was used for the reaction according to the manufacturer’s instructions. Five times diluted cDNA was used as a template. The annealing temperature of the primers was 57°C. The primer sequences are shown in Table S1. Real-time PCR reactions were performed in triplicate for each of the analyzed samples. Changes in the transcript levels are presented as relative amounts. For reference, GAPDH (glyceraldehyde-3-phosphoglyceraldehyde dehydrogenase) gene was used.
Statistical analysis
All of the experiments were independently repeated at least three times. The results are presented as the averages of independent replicates ± standard deviations. Statistical analyses were performed using Statistica 7 software (StatSoft, USA). The significance of the differences between the means was determined by Student’s t-test and marked with asterisks for p < .05.
Results
The content of bioactive compounds in flax fibers
The contents of bioactive compounds, including free and cell wall bound phenolic compounds, lutein, and polyamines, were assessed in the fibers obtained after decortication, wet degumming, and cottonization of the flax stem in comparison with the dry retting process ().
Table 2. The content of total free and bound to the cell wall phenolic compounds, terpenoic compounds, and polyamines in fibers obtained by retting, decortication, wet degumming, and cottonization of B14 and Nike flax stems. Data were obtained from the UPLC analysis. Results are mean ± SD (n = 5). Statistically significant changes are marked with asterisks (p < .05). bdl – below detection limit.
It was observed that the fibers obtained after decortication exhibited the highest levels of all bioactive compounds. However, in the fibers derived from transgenic B14 plants’ straw, these compounds were significantly lower compared to the fibers obtained from non-transgenic plant straw. Approximately 70% less free phenolic compounds and lutein, along with 28% less polyamines, were determined.
Following the wet degumming process, fibers were characterized by the lowest amounts of phenolic compounds, with free phenolic compounds falling below the detection threshold. In contrast, B14 fibers exhibited notably higher levels of bound phenolic compounds (34% more) and a threefold increase in lutein after this particular stage.
Fibers obtained after cottonization displayed the lowest concentrations of lutein and polyamines. However, after the cottonization process, B14 fibers showcased elevated levels of all the analyzed bioactive compounds. The study revealed a 2.5-fold increase in free phenolic compounds and a 1.4-fold increase in bound to the cell wall phenolic compounds and polyamines content.
The fibers obtained through the retting process contained fewer bioactive compounds compared to those obtained after decortication, yet more than the fibers after wet degumming and cottonization. Remarkably, the contents of these compounds were similar in both the Nike B14 fibers and the control non-transgenic fibers.
The highest content of free phenolic compounds was found in decorticated fibers, in which caffeic acid, vanillin, orientin-glycoside, apigenin-diglycoside, orientin-glycosides, isoorientin, orientin, and vitexin were identified (). However, B14 fibers contained significantly less of all tested compounds. Vanillin, isoorientin, orientin, and vitexin were determined in the cottonized fibers, with significantly more isoorientin, orientin, and vitexin in the B14 fibers.
Table 3. The content of free phenolic compounds in fibers obtained by retting, decortication, wet degumming, and cottonization of B14 and Nike flax stems. Data were obtained from the UPLC analysis. Results are mean ± SD (n = 5). Statistically significant changes are marked with asterisks (p < .05). bdl – below detection limit.
Among the phenolic compounds associated with the cell wall, vanillin, coumaric acid, ferulic acid, and vitexin were detected in all the tested fibers (). Among them, vanillin exhibited the highest abundance in the fibers after retting, while its content was the lowest in the fibers after cottonization. Notably, there were no significant differences in vanillin levels between the transgenic B14 fibers and the non-transgenic Nike fibers.
Table 4. The content of bound to the cell-wall phenolic compounds in fibers obtained by retting, decortication, wet degumming, and cottonization of B14 and Nike flax stems. Data were obtained from the UPLC analysis. Results are mean ± SD (n = 5). Statistically significant changes are marked with asterisks (p < .05). bdl – below detection limit.
The contents of coumaric acid, ferulic acid, and vitexin were the highest in the fibers after retting and wet degumming, with B14 fibers showing elevated levels of ferulic acid and vitexin. B14 fibers after wet degumming also displayed higher amounts of coumaric acid.
Moreover, fibers obtained from both Nike and B14 plants after decortication exhibited a substantial content of compounds that were exclusively identified after this particular process, such as caffeic acid, isoorientin, and orientin. On the other hand, the fibers after retting contained vanillic acid as the only one, which showed a lower content in the B14 fibers.
Furthermore, syringic aldehyde was determined in fibers after retting, wet degumming, and cottonization, while 4-hydroxybenzoic aldehyde was identified in fibers after retting, decortication, and cottonization. The highest levels of 4-hydroxybenzoic aldehyde were found in B14 fibers after cottonization.
The content of polyamines in transgenic flax line B14 and non-transgenic flax Nike fibers obtained after retting, decortication, wet degumming, and cottonization was shown as the sum of free polyamines, polyamines conjugated to phenolic compounds and polyamines bound to the cell wall. In separate polyamine fractions, the amounts of putrescine, spermidine, and spermine were determined ().
Table 5. The content of free polyamines (PA1) conjugated polyamines (PA2) and cell wall-bound polyamines (PA3), including individual polyamines: putrescine, spermidine, and spermine in fibers obtained by retting, decortication, wet degumming, and cottonization of B14 and Nike flax stem. Data were obtained from the UPLC analysis. Results are mean ± SD (n = 4). Statistically significant changes are marked with asterisks (p < .05). bdl – below detection limit.
Fibers after decortication contained the most polyamines in the fraction of polyamines bound to the cell wall (77.78 µg/g DW in Nike fibers), but their content was lower by 33% in B14 fibers compared to Nike fibers. This fraction contained the most spermidine, the content of which in B14 fibers was 43% lower. The fraction of free polyamines and polyamines conjugated to phenolic compounds contained similar amounts of polyamines. B14 fibers contained 35% more free polyamines (most of which was putrescine). The conjugated polyamine fraction contained the most spermidine, but 46% less in B14 fibers than in Nike fibers.
Fibers after cottonization contained the least amount of polyamines. After this process, polyamines bound to the cell wall were the most abundant in B14 fibers, 60% more than in Nike fibers. This was due to the higher amount of spermidine, putrescine, and spermine in this fraction of polyamines. In the fraction of free polyamines, a 60% lower content of polyamines in B14 fibers was observed, which was a consequence of the lower amount of putrescine.
Similarly to the cottonization process, after the retting process, the fraction bound to the cell wall contained 60% more polyamines in the B14 fibers, while in the fraction of free polyamines in these fibers there were 42% fewer compounds. The amounts of putrescine and spermidine were similar in all polyamine fractions; however, in the free polyamine fraction, there was 54% and 34% less putrescine and spermidine, respectively; in the conjugated polyamine fraction, there was 37% less putrescine and the PA3 fraction contained 2.7 times more spermidine.
The wet degumming fibers were characterized by a small amount of polyamines, with the largest amount of these compounds in the fraction of free polyamines, and among them the largest amounts were putrescine and spermidine. The contents of individual polyamines (putrescine, spermidine, and spermine) in the three fractions were the same for B14 fibers and Nike fibers.
The antioxidant activity of flax fibers
The antioxidant potential of fiber extracts obtained after decortication, wet degumming, cottonization, and retting of flax stem was determined by the DPPH and FRAP method (). The highest antioxidant potential was shown by the extract of free phenolic compounds from Nike fiber obtained after decortication (75% inhibition of free radicals). About 40% of free radical inhibition was found in extracts containing cell wall-bound phenolic compounds from Nike and B14 fibers after retting and decortication, and about 20% after cottonization. The extracts from fibers after wet degumming had the lowest antioxidant potential. Extracts from B14 fibers containing free phenolic compounds and terpene compounds after decortication were characterized by lower antioxidant activity compared to Nike fibers. On the other hand, extracts from B14 fibers containing free phenolic compounds and terpene compounds after cottonization were characterized by higher antioxidant activity compared to Nike fibers. The analysis of the antioxidant potential using the FRAP method confirmed the results obtained using the DPPH method, that the B14 fiber extracts containing free phenolic compounds and terpene compounds after decortication had a lower antioxidant potential and after the cottonization process had a higher antioxidant potential compared to Nike fiber extracts.
The content of cell wall polymers in flax fibers
The content of cell wall polymers, including cellulose, lignin, pectin, and hemicellulose, was determined in fibers obtained through retting, decortication, wet degumming, and cottonization processes of B14 and Nike flax stems (). Fibers obtained after decortication exhibited the highest cellulose content, while fibers after retting and cottonization displayed the lowest cellulose levels. B14 fibers generally demonstrated slightly higher cellulose content compared to Nike fibers, regardless of the specific flax straw processing method employed.
Figure 2. The content of cell wall polymers (cellulose, lignin, pectin, and hemicellulose) in fibers obtained by retting, decortication, wet degumming, and cottonization of B14 and Nike flax stems. Statistically significant changes are marked with asterisks (p < .05).
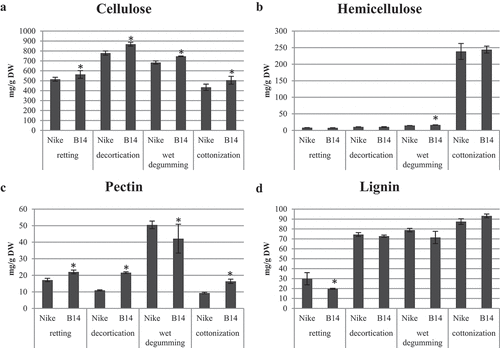
Among the various processes, fibers after cottonization exhibited the highest hemicellulose content, approximately 240 mg/g DW. On the other hand, fibers obtained through other methods contained significantly lower hemicellulose levels. Specifically, the hemicellulose content in fibers after retting was approximately 8 mg/g DW, after decortication, it was about 10 mg/g DW, and after wet degumming, it measured around 15 mg/g DW for Nike fibers and 16.5 mg/g DW for B14 fibers.
The fibers obtained after wet degumming showcased the highest amount of pectin, ranging from 40 to 50 mg/g DW. Conversely, the content of pectin after retting, decortication, and cottonization processes was comparable, ranging from 10 to 20 mg/g DW. Notably, B14 fibers contained lower amounts of pectin compared to Nike fibers after wet degumming, while B14 fibers obtained through the other three processes exhibited higher pectin levels.
Regarding lignin content, similar levels were observed in Nike and B14 fibers after decortication, wet degumming, and cottonization, ranging from 70 to 90 mg/g DW. However, after retting, the fibers displayed lower lignin content, with B14 fibers containing 20 mg/g DW and Nike fibers containing 30 mg/g DW.
The content of fatty acids in flax fibers
The fatty acid composition was analyzed in fibers obtained through retting, decortication, wet degumming, and cottonization processes from B14 and Nike flax stems (). The highest concentration of fatty acids was observed in Nike fibers after retting, reaching 617.36 mg/100 g DW, while the lowest content was found in Nike fibers after cottonization, with 221.14 mg/100 g DW. B14 fibers exhibited lower fatty acid levels after decortication and wet degumming, with reductions of 9% and 13%, respectively. However, after cottonization, B14 fibers showed a 23% increase in fatty acid content.
Table 6. The content of fatty acids in fibers obtained by retting, decortication, wet degumming, and cottonization of B14 and Nike flax stems. Data were obtained from the GC-FID analysis. Results are mean ± SD (n = 4). Statistically significant changes are marked with asterisks (p < .05).
Moreover, flax fibers were characterized by a higher proportion of saturated fatty acids and a lower proportion of unsaturated fatty acids. B14 fibers demonstrated reduced levels of C18:2 fatty acid by 24% after retting, 49% after decortication, and 30% after wet degumming. However, after cottonization, the content of C18:2 fatty acid increased by 3.4 times. The concentration of C18:3 fatty acid was 33% lower after retting, 44% lower after decortication, and 2.2 times higher after cottonization in B14 fibers. Additionally, B14 fibers after wet degumming exhibited a 15% decrease in the content of C18:1 fatty acid compared to Nike fibers.
B14 fibers also displayed lower levels of saturated fatty acids after decortication and wet degumming, with approximately 10% lower content of C18:0 fatty acid after each process. However, after cottonization, both C16:0 and C18:0 fatty acids increased in B14 fibers, reaching 1.2 times and 1.1 times higher levels, respectively.
The content of phytosterols in flax fibers
The contents of campesterol, stigmasterol, and β-sitosterol were determined in fibers obtained by retting, decortication, wet degumming, and cottonization of B14 and Nike flax stems (). The highest content of campesterol was found in B14 fibers after retting (22.07 mg/100 g DW), and the lowest content of this compound was found in B14 fibers after cottonization (8.56 mg/100 g DW). The amounts of campesterol did not differ between B14 fibers and Nike fibers, only after decortication in B14 fibers there was 21% less of this compound compared to Nike fibers.
Table 7. The content of phytosterols in fibers obtained by retting, decortication, wet degumming, and cottonization of B14 and Nike flax stems. Data were obtained from the GC-FID analysis. Results are mean ± SD (n = 4). Statistically significant changes are marked with asterisks (p < .05).
The content of stigmasterol was similar in the fibers after retting and wet degumming and in the fibers after decortication and cottonization and amounted to approx. 16–20 mg/100 g DW and 10–14 mg/100 g DW, respectively. In addition, B14 fibers after retting and decortication contained less by 11% and 25%, respectively.
Among the identified phytosterols, the fibers contained the most β-sitosterol (B14 fibers after retting 101.33 mg/100 g DW; 22% more than Nike fibers). Fibers after decortication and cottonization contained about 40 mg/100 g DW of β-sitosterol, while wet degumming fibers contained about 70 mg/100 g DW of this compound.
Antimicrobial activity of flax fibers
The influence of fiber extracts (methanolic and hydrolyzed) on four microorganism strains’ (Staphylococcus aureus, Escherichia coli, Pseudomonas aeruginosa, and Candida albicans) growth was investigated (). Among the methanolic extracts used in the experiment, those obtained from retted fibers of both Nike and B14 lines showed almost no effect of inhibition of the microorganism growth with one exception of P. aeruginosa inhibited by B14 extract by 14%. No inhibiting effects were noted for E. coli and C. albicans. On the other hand, the most pronounced inhibition was observed for the cottonized fibers with the highest inhibition again for P. aeruginosa (by 47.7%). The hydrolyzed extracts were far more effective, and the extracts had to be 10 times diluted to measure the inhibition of microorganism growth. The exception was C. albicans, against which non-diluted extracts were used, and still, the highest inhibition reached 28% for cottonized B14 fiber extract. Again, the highest efficacy was observed for the cottonized fibers, from both Nike and B14 lines with the highest inhibition of P. aeruginosa growth (by over 90% for Nike and B14 lines).
Table 8. The influence of extracts from fibers obtained by retting, decortication, wet degumming, and cottonization of flax stems on microorganisms’ growth was presented as the percentage of non-treated microorganisms (100%) ± standard deviation. Statistically significant changes are marked with asterisks (p < .05).
V79 cell viability after phenolic extract from flax fibers
For the treatment of the V79 hamster lung fibroblast cell line, aqueous extracts containing phenolic compounds from Nike and B14 flax fibers after retting, decortication, wet degumming, and cottonization were prepared. The type 1 extract derived from Nike fibers after decortication contained the highest amount of phenolic compounds (5134.76 µg/g DW), while the lowest levels were observed in both Nike and B14 fibers after wet degumming and cottonization (10–30 µg/g DW). For extract type 2, the most phenolic compounds were found in Nike fibers after decortication (1071.91 µg/g DW) and B14 fibers after retting (988.28 µg/g DW), while the lowest levels were detected in fibers after cottonization (10–14 µg/g DW). The detailed content of phenolic compounds is presented in .
Table 9. The content of free phenolic compounds in aqueous extract no. 1 from fibers obtained by retting, decortication, wet degumming, and cottonization of B14 and Nike flax stems. Data were obtained from the UPLC analysis.
Table 10. The content of bound to the cell wall phenolic compounds in aqueous extract no. 2 from fibers obtained by retting, decortication, wet degumming, and cottonization of B14 and Nike flax stems. Data were obtained from the UPLC analysis.
The type 1 extract obtained from Nike fibers after the decortication process exhibited the highest levels of isoorientin (1816.32 µg/g DW) and orientin (1101.54 µg/g DW). In contrast, the corresponding extract from B14 fibers contained significantly lower amounts of these compounds, with isoorientin being more than 5 times less abundant and orientin being 2.4 times less prevalent.
Extract 1 from B14 fibers, following decortication, demonstrated higher levels of vanillic acid and apigenin-glycoside compared to other compounds. On the other hand, the type 1 extract from B14 fibers after retting displayed elevated concentrations of all identified phenolic compounds in comparison to the extract derived from Nike fibers, reaching a maximum of 19.5 µg/g DW. The extracts obtained from fibers after wet degumming and cottonization exhibited the lowest content of phenolic compounds, with maximum concentrations of 7.5 µg/g DW and 5.9 µg/g DW, respectively.
The type 2 extract derived from B14 fibers after retting and Nike fibers after decortication contained the highest ferulic acid levels (326.94 µg/g DW and 386.88 µg/g DW, respectively). Notably, the type 2 extract from B14 fibers after retting contained 2.4 times more phenolic compounds compared to the extract from Nike fibers. However, after decortication, it contained 2.2 times fewer of these compounds. On the other hand, the content of phenolic compounds in both Nike and B14 fibers after wet degumming and cottonization did not show significant differences
The impact of aqueous extracts containing phenolic compounds extracted from Nike and B14 flax fibers subjected to retting, decortication, wet degumming, and cottonization processes, on the viability of hamster V79 lung fibroblast cell lines is presented in . The figure is divided into two panels: represents the results obtained with the type 1 extract, while represents the results obtained with the type 2 extract.
Figure 3. The influence of extracts from fibers obtained by retting, decortication, wet degumming, and cottonization of flax stems on fibroblast cell growth presented as the percentage of non-treated fibroblast cells (100%) ± standard deviation. Statistically significant changes are marked with asterisks (p < .05).
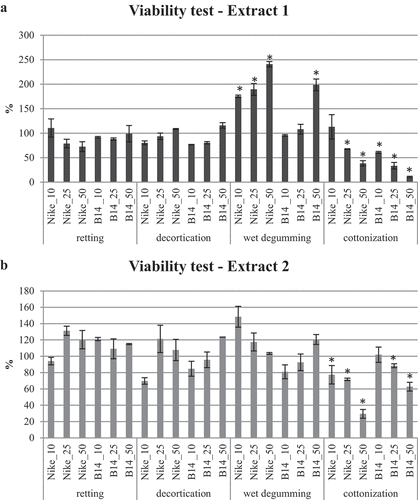
Neither the extracts obtained from Nike fibers nor those obtained from B14 fibers after retting, decortication, and wet degumming demonstrated cytotoxic effects on V79 cells. Furthermore, the type 1 extract from B14 fibers after wet degumming, at the highest concentration (50 µL), and the type 1 extract from Nike fibers after wet degumming, for all analyzed volumes, exhibited properties that promote the proliferation of V79 cells.
However, both extracts obtained from B14 and Nike fibers after cottonization displayed properties that inhibit the proliferation of V79 cells, except for Nike fiber extract 1 at a volume of 10 µL and B14 fiber extract 2 at a volume of 10 µL.
Influence of extract from flax fibers on inflammation-related genes in V79 cells
The alterations in the transcript levels of pro-inflammatory genes (IL6: interleukin 6 and MCP1: monocyte chemoattractant protein-1), anti-inflammatory genes (IL10: interleukin 10 and ICAM: intercellular adhesion molecule 1), and redox-related genes (CAT: catalase and SOD: superoxide dismutase) in the V79 cell line upon treatment with phenolic compound extracts derived from Nike and B14 fibers, obtained through retting, decortication, wet degumming, and cottonization processes, are illustrated in (type 1 extract) and (type 2 extract).
Figure 4. Transcript levels of pro-inflammatory (IL6 and MCP1), anti-inflammatory (IL10 and ICAM), and redox (CAT and SOD) genes in the V79 cell line treated with extracts (extract type 1 - 4A and extract type 2 - 4B) containing phenolic compounds from fibers obtained by retting, decortication, wet degumming, and cottonization of flax stems compared to control (untreated cells = 1). Statistically significant changes are marked with asterisks (p < .05).
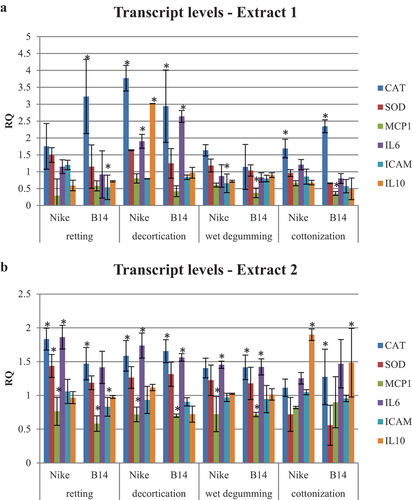
Based on the findings, it can be inferred that there are no noteworthy distinctions in the impact of type 1 and type 2 extracts obtained from B14 transgenic flax fibers, as well as type 1 and type 2 extracts derived from non-transgenic NIKE flax fibers, on the transcript levels of inflammation-related genes in the V79 cell line. However, variations are observed when comparing the mRNA levels of the examined genes in cells treated with fiber extracts to those of the control (untreated) cells.
Upregulation of the catalase gene transcript level was observed in the V79 hamster cell line after treatment with extract 1 derived from Nike and B14 fibers following the processes of decortication and cottonization, as well as from Nike fibers after retting. Regarding pro-inflammatory genes, the mRNA level of MCP1 gene transcript showed a decrease in the V79 hamster cell line when treated with extract 1 obtained from B14 fibers following wet degumming and cottonization, whereas the IL6 gene transcript level increased when exposed to extract 1 obtained from Nike and B14 fibers following decortication. Concerning anti-inflammatory genes, the transcript level of the ICAM gene was downregulated in the V79 hamster cell line by extract 1 derived from Nike fibers following retting and B14 fibers following wet degumming. Conversely, the IL10 gene transcript level was increased in the V79 hamster cell line when treated with extract 1 obtained from Nike fibers after decortication.
Upon treatment with type 2 extract, V79 hamster cell lines exhibited an upregulation in the expression of catalase, superoxide dismutase, interleukin 6, and interleukin 10 gene transcripts, while there was a downregulation in the expression of MCP1 and ICAM gene transcripts. Specifically, the catalase gene transcript level increased in the V79 hamster cell line following treatment with extract 2 obtained from Nike and B14 fibers after retting and decortication, as well as from B14 fibers after wet degumming and cottonization. Additionally, an increase in the level of superoxide dismutase (SOD) mRNA was observed only in V79 hamster cells treated with Nike fiber extract 2 after retting. Among the pro-inflammatory genes, the MCP1 transcript was downregulated in V79 hamster cells treated with Nike fiber extract 2 and B14 fibers after retting, decortication, and wet degumming, while the IL6 gene transcript was upregulated in V79 hamster cells treated with Nike fiber extract 2 and B14 fibers after decortication and wet degumming, as well as in Nike fibers after retting. Regarding anti-inflammatory gene transcripts, the ICAM mRNA was downregulated in V79 hamster cells treated with B14 fiber extract 2 after retting, and the IL10 mRNA was upregulated in V79 hamster cells treated with Nike and B14 fiber extract 2 after cottonization.
Discussion
The traditional extraction of flax fiber (dew retting) is a tedious and long-lasting process, and the effect does not always meet standardized requirements. Therefore, taking into account the expanding range of applications, the process of obtaining fiber should be as easy as possible, while, and more importantly, maintaining its unique properties. In the novel technological approach, the flax straw underwent decortication, resulting in the acquisition of a poorly separated single-type fiber characterized by significant impurity content. Subsequently, the aim of achieving high-quality fibers was pursued through the implementation of the osmotic degumming process within a sealed apparatus with adjustable pressure, followed by ultrasound processing using an open device. Following the wet processing, a mechanical treatment was employed to achieve the cottonization of the decorticated fibers (Zimniewska et al. Citation2017). Comparative analyses were conducted on all fiber types obtained at each processing stage in relation to the fibers obtained through dry retting.
We measured the amounts of cell wall biopolymers in the fibers obtained with the described methods. As mentioned, dew retting does not produce comparable fibers, and the results always rely on the conditions of the process, which is a limitation when obtaining fibers using this method. Fibers at all stages of processing and retted fibers are differentiated in terms of polymers content, with retted fibers having the lowest lignin content, while target fibers demonstrate the lowest pectin content. The processing of the fiber changes the content of polymers in it, the target fibers are characterized by a reduced level of cellulose and pectin and an increased amount of lignin and hemicelluloses. It is worth mentioning that the transgenic B14 line showed higher amounts of the biopolymers in all fiber extraction methods. Zimniewska et al., while analyzing Nike fibers, described decreased levels of pectin, hemicellulose, and lignin amounts in cottonized flax fibers. In the case of the main fiber polymer (cellulose), cottonized fibers did not show significant changes (Zimniewska et al. Citation2017). Differences in polymer content may most likely result from the methods used to determine them.
After evaluating the contents of bio-active compounds in the fiber after different stages of its extraction, it turned out that the decorticated fibers contain the largest amount of all secondary metabolites, both in the free form and in the form bound to the cell wall. In comparison, in retted fibers the cell wall bound phenolic and terpenoid compounds were on similar levels. Polyamine content was, on the other hand, strongly decreased in the retted fibers compared to the decorticated ones. Cottonized flax fibers were characterized by a reduced content of bound phenolic derivatives, terpenes, and polyamines compared to retted fibers.
In our next step, we investigated whether the chemical composition of fibers obtained with the described methods is reflected in their biological properties. Since the assayed bio-active compounds are characterized as antioxidants, we measured the antioxidant potentials of different extracts from flax fibers. We used two different methods (DPPH quenching and FRAP), as those may reflect different aspects of the antioxidant potential of the extracts (Rumpf, Burger, and Schulze Citation2023). As expected, the decorticated fibers, which possessed the highest amounts of phenolics, terpenoids, and polyamines, exhibited the highest potential to reduce DPPH radical, while wet degumming produced the worst results. In the case of cottonization process, which deprives the fiber of phenolic antioxidants to a much greater extent compared to the decortication process, the antioxidant potential measured with the DPPH method is comparable, especially for the cell-wall-bound fraction. This may result from the fact that the mechanical processing leading to shorter fibers, together with the removal of any loosely bound compounds leads to the exposition of reactive chemical groups (-OH, -COOH, etc.). The suggestion is consistent with our observation that micronization of the fiber increases its chemical reactivity and resulted in more available functional groups (hydroxyl, carboxyl), which were derived from fiber constituents (Dymińska et al. Citation2013). However, when FRAP assay was used, despite the differences in the bio-active chemical contents, all four fiber extractions gave comparable results. In the FRAP method, the ability to reduce ferric (Fe3+) to ferrous (Fe2+) ion is assayed. FRAP results can vary significantly depending on the time scale of the analysis. Fast-reacting phenols that bind iron or decompose into compounds with lower or different reactivity are best analyzed with short reaction times, for example 4 min. However, some polyphenols react slower and require longer response times for detection (e.g. 30 min). Therefore, the observed small differences may result from too short reaction time (Prior, Wu, and Schaich Citation2005).
The highest total level of fatty acids is found in retted fibers, both Nike and B14. Processing reduces the total level of fatty acids by about 2 times in the fibers of both types of plants. The biggest changes concern the content of unsaturated fatty acids 18:2 and 18:3 between Nike and B14 fibers. The content of sterols in fibers from both types of plants is comparable. The highest level of these compounds is manifested in the retted fiber, regardless of the type of plant it comes from. Cottonized flax fiber contains about 2 times less phytosterols compared to retted fiber.
PAs are found in all living organisms in free soluble, non-covalently conjugated, or covalently conjugated forms (Chen et al. Citation2018). One of the methods of polyamine level regulation in plants is their conjugation with other chemical compounds (e.g. phenolic acids). In some plant species, the polyamine reservoir can hold up to 90% of the total polyamine content in the cells, but the type of conjugated polyamines and their ratio to free forms are species- and tissue-dependent (Bagni and Tassoni Citation2001). Spermidine appears as the most predominant polyamine conjugated with the cell wall in flax fibers at every stage of processing and in retted fibers, which is consistent with the literature (Dong et al. Citation2015; Li et al. Citation2018). Detailed analysis of the polyamine fractions (free, soluble-conjugated, and cell wall bound) indicates that, although the decreases are observed for each of them in the retted fibers, the most pronounced change concerns the cell-wall-bound polyamines. This fraction is also the most represented one, meaning most of the polyamines are stored in this form as cell wall reinforcement, and can be released if necessary (Zeiss, Piater, and Dubery Citation2021).
On the other hand, when looking at the role of polyamines in living organisms, it should be remembered that some bacterial species do not have de novo polyamine biosynthesis mechanisms and require the uptake of polyamines from the host or the medium for growth (Joshi et al. Citation2011). The situation is different in the case of Staphylococcus aureus, which lacks polyamine biosynthesis genes but is hypersensitive to exogenous polyamines. Spermine and spermidine have been shown to have bactericidal activity against S. aureus (Douglas et al. Citation2022; Joshi et al. Citation2011). In the case of Pseudomonas aeruginosa, polyamines have been shown to increase sensitivity to antibiotics (Kwon and Lu Citation2006). However, recent reports show that these bacteria use host-derived spermidine to facilitate antimicrobial tolerance (Hasan et al. Citation2022). Data on the effect of exogenous polyamines on pathogenic microorganisms are contradictory and require further research.
In the next step, we investigated the antimicrobial properties of the obtained fibers. We used two kinds of fiber extracts, one containing soluble bio-active compounds, and the second obtained after cell wall hydrolysis, to release all chemically bound compounds. The most antibacterial effect was obtained for the fibers after cottonization. This was surprising at first, as these fibers contain less bioactive compounds than the decorticated fibers, yet have comparable or even better antimicrobial properties. Analysis of the composition of the extracts did not bring any answers, but considering the presence of free and soluble conjugated polyamines, these compounds may play a major role in the anti-bacterial properties of cottonized fibers. Perhaps, it is the combination of different phenolics and polyamine forms that are important for these properties, as the hydrolyzed extracts showed even more pronounced antibacterial (and antifungal) activity of the cottonized fiber. It is also possible that the much smaller amount of polyamines in cottonized fibers is responsible for the antibacterial properties by not providing the bacteria with enough polyamines to grow and develop. Unfortunately, the effects obtained for the fibers from transgenic flax were similar or even worse, suggesting that the fiber processing does not correlate directly with the better properties of living GM plants.
The negative effect of flax fiber extracts on live V79 cells (hamster fibroblasts) was shown only for cottonized fibers. This rather undesirable effect may constitute the presumptive evidence that the ratio of different bio-active compounds rather than their amounts is important for a given biological effect, like cell toxicity in this case. Searching for the possible background of such V79 cell response, we measured the transcript levels of genes involved in oxidative stress processing and genes connected with inflammation. However, no clear correlation was found. Hence, further investigation is necessary to find the mechanisms behind the observed cytotoxicity.
Conclusions
In the present study, a new technology involving three stages, decortication, wet degumming, and cottonization, was applied for flax fiber processing, and it was compared with the traditional process of flax straw retting (dew retting). The processing of the fiber changed the content of cell wall bio-polymers (reduction in cellulose and pectin content and increase in lignin and hemicelluloses), and reduced the contents of all secondary metabolites: free and cell wall-bound phenolic derivatives, terpenes, polyamines, phytosterols, as well as fatty acids. We believe that it is rather the ratio of particular bio-active compounds, not the quantities, that influence the biological properties of the fibers, and polyamines seem to be an underestimated factor that may differentially influence the antibacterial properties of flax fibers. The cytotoxic effect of the cottonized fibers may be utilized in the development of novel therapies against cancer cells, but further investigations are required. The new technology of flax fiber processing did not significantly affect the characteristics of B14 fibers. Like green plants, the fibers were characterized by an increased content of polyamines and a higher antioxidant potential. Moreover, B14 fibers obtained by the new method contained more phenolic compounds compared to Nike fibers.
Highlights
This study compares cottonization with traditional retting, evaluating quality improvements.
A decrease in fiber polymers and bioactive compounds after cottonization, yet a significant rise in antioxidant potential, was observed.
Cottonized flax fibers demonstrate potent antimicrobial activity, particularly against Pseudomonas aeruginosa.
Extracts from cottonized fibers display cytotoxic effects on lung fibroblast cells, hinting at potential cancer research applications.
Cottonization holds promise in augmenting flax fiber quality and bioactivity, with implications for medical and textile sectors.
Cottonized B14 linen fibers with a higher content of phenols and polyamines and a higher antioxidant potential than cottonized Nike fibers.
Abbreviation list
PA | = | polyamine |
DPPH | = | 1,1-diphenyl-2-picrylhydrazil |
FRAP | = | Ferric Ion Reducing Antioxidant Power |
AIR | = | alcohol insoluble components |
CDTA | = | trans-1,2-diaminocyclohexane-N,N,N,N-tetraacetic acid |
WSF | = | water-soluble fraction |
CSF | = | CDTA-soluble fraction |
NSF | = | Na2CO3 and NaBH4 soluble fraction |
K1SF | = | 1 M KOH with 20 mM NaBH4 soluble fraction |
K4SF | = | 4 M KOH soluble fraction |
MTT | = | 3-[4,5-dimethylthiazol-2-yl]-2,5 diphenyl tetrazolium bromide |
DW | = | dry weight |
GAPDH | = | glyceraldehyde-3-phosphate dehydrogenase |
IL6 | = | interleukin 6 |
MCP1 | = | monocyte chemoattractant protein-1 |
IL10 | = | interleukin 10 |
ICAM | = | intercellular adhesion molecule 1 |
CAT | = | catalase |
SOD | = | superoxide dismutase |
RQ | = | relative quantification |
Author contributions
Wioleta Wojtasik: Investigation, Methodology, Formal analysis, Visualization, Supervision, Writing – original draft, Writing – review & editing. Kamil Kostyn: Investigation, Writing – review & editing. Marta Preisner: Investigation, writing – review & editing. Taduesz Czuj: Investigation. Małgorzata Zimniewska: Conceptualization, Resources. Jan Szopa: Conceptualization, Supervision, Funding acquisition, Project administration. Magdalena Wróbel-Kwiatkowska: Writing – original draft.
Supplemental Material
Download MS Word (13.8 KB)Acknowledgments
Special thanks to the Institute of Natural Fibers and Medicinal Plants for the preparation of the research material.
Disclosure statement
No potential conflict of interest was reported by the author(s).
Supplementary material
Supplemental data for this article can be accessed online at https://doi.org/10.1080/15440478.2024.2368143
Additional information
Funding
References
- Ahmed, A. E. R., and J. M. Labavitch. 1978. “A Simplified Method for Accurate Determination of Cell Wall Uronide Content.” Journal of Food Biochemistry 1 (4): 361–24. https://doi.org/10.1111/j.1745-4514.1978.tb00193.x.
- Akin, D. E. 2013. “Linen Most Useful: Perspectives on Structure, Chemistry, and Enzymes for Retting Flax.” ISRN Biotechnology 2013:1–23. https://doi.org/10.5402/2013/186534.
- Amel, B. A., M. T. Paridah, R. Sudin, U. M. K. Anwar, and A. S. Hussein. 2013. “Effect of Fiber Extraction Methods on Some Properties of Kenaf Bast Fiber.” Industrial Crops and Products 46:117–123. https://doi.org/10.1016/j.indcrop.2012.12.015.
- Antonov, V., J. Marek, M. Bjelkova, P. Smirous, and H. Fischer. 2007. “Easily Available Enzymes as Natural Retting Agents.” Biotechnology Journal 2 (3): 342–346. https://doi.org/10.1002/biot.200600110.
- Bagni, N., and A. Tassoni. 2001. “Biosynthesis, Oxidation and Conjugation of Aliphatic Polyamines in Higher Plants.” Amino Acids 20 (3): 301–317. https://doi.org/10.1007/s007260170046.
- Benzie, I. F., and J. J. Strain. 1996. “The Ferric Reducing Ability of Plasma (FRAP) as a Measure of “Antioxidant Power”: The FRAP Assay.” Analytical Biochemistry 239 (1): 70–76. https://doi.org/10.1006/abio.1996.0292.
- Blumenkrantz, N., and G. Asboe-Hansen. 1973. “New Method for Quantitative Determination of Uronic Acids.” Analytical Biochemistry 54 (2): 484–489. https://doi.org/10.1016/0003-2697(73)90377-1.
- Boba, A., K. Kostyn, M. Preisner, W. Wojtasik, J. Szopa, and A. Kulma. 2018. “Expression of Heterologous Lycopene β-Cyclase Gene in Flax Can Cause Silencing of its Endogenous Counterpart by Changes in Gene-Body Methylation and in ABA Homeostasis Mechanism.” Plant Physiology & Biochemistry 127:143–151. https://doi.org/10.1016/j.plaphy.2018.03.023.
- Brand-Williams, W., M. E. Cuvelier, and C. Berset. 1995. “Use of a Free Radical Method to Evaluate Antioxidant Activity.” LWT - Food Science & Technology 28 (1): 25–30. https://doi.org/10.1016/S0023-6438(95)80008-5.
- Chabbert, B., J. Padovani, C. Djemiel, J. Ossemond, A. Lemaître, A. Yoshinaga, S. Hawkins, S. Grec, J. Beaugrand, and B. Kurek. 2020. “Multimodal Assessment of Flax Dew Retting and its Functional Impact on Fibers and Natural Fiber Composites.” Industrial Crops and Products 148:112255. https://doi.org/10.1016/j.indcrop.2020.112255.
- Chen, D., Q. Shao, L. Yin, A. Younis, and B. Zheng. 2018. “Polyamine Function in Plants: Metabolism, Regulation on Development, and Roles in Abiotic Stress Responses.” Frontiers in Plant Science 9:1945. https://doi.org/10.3389/fpls.2018.01945.
- Czemplik, M., A. Boba, K. Kostyn, A. Kulma, A. Mituła, M. Sztajnert, M. Wróbel- Kwiatkowska, M. Żuk, J. Szopa, and K. Skórkowska - Telichowska. 2011. “Flax Engineering for Biomedical Application.” In Biomedical Engineering, edited by K. M. Anna and O.-J. Sylwia, Vol. Chapter 17. 407–434. Rijeka: IntechOpen.
- Dong, X., Y. Gao, W. Chen, W. Wang, L. Gong, X. Liu, and J. Luo. 2015. “Spatiotemporal Distribution of Phenolamides and the Genetics of Natural Variation of Hydroxycinnamoyl Spermidine in Rice.” Molecular Plant 8 (1): 111–121. https://doi.org/10.1016/j.molp.2014.11.003.
- Douglas, E. J. A., A. H. Alkhzem, T. Wonfor, S. Li, T. J. Woodman, I. S. Blagbrough, and M. Laabei. 2022. “Antibacterial Activity of Novel Linear Polyamines Against Staphylococcus Aureus.” Frontiers in Microbiology 13:948343. https://doi.org/10.3389/fmicb.2022.948343.
- Dymińska, L., M. Szatkowski, M. Wróbel-Kwiatkowska, M. Żuk, A. Kurzawa, W. Syska, A. Gągor, et al. 2013. “Improved Properties of Micronized Genetically Modified Flax Fibers.” Journal of Biotechnology 164 (2): 292–299. https://doi.org/10.1016/j.jbiotec.2013.01.002.
- Hasan, C. M., S. Pottenger, A. E. Green, A. A. Cox, J. S. White, T. Jones, C. Winstanley, et al. 2022. “Pseudomonas aeruginosa Utilizes the Host-Derived Polyamine Spermidine to Facilitate Antimicrobial Tolerance.” JCI Insight 7 (22). https://doi.org/10.1172/jci.insight.158879.
- Henriksson, G., D. E. Akin, R. T. Hanlin, C. Rodriguez, D. D. Archibald, L. L. Rigsby, and K. L. Eriksson. 1997. “Identification and Retting Efficiencies of Fungi Isolated from Dew-Retted Flax in the United States and Europe.” Applied & Environmental Microbiology 63 (10): 3950–3956. https://doi.org/10.1128/aem.63.10.3950-3956.1997.
- Huo, S., C. Ulven, H. Wang, and X. Wang. 2013. “Chemical and Mechanical Properties Studies of Chinese Linen Flax and Its Composites.” Polymers & Polymer Composites 21 (5): 275–286. https://doi.org/10.1177/096739111302100502.
- Iiyama, K., and A. F. A. Wallis. 1990. “Determination of Lignin in Herbaceous Plants by an Improved Acetyl Bromide Procedure.” Journal of the Science of Food & Agriculture 51 (2): 145–161. https://doi.org/10.1002/jsfa.2740510202.
- Imai, A., T. Akiyama, T. Kato, S. Sato, S. Tabata, K. T. Yamamoto, and T. Takahashi. 2004. “Spermine is Not Essential for Survival of Arabidopsis.” FEBS Letters 556 (1–3): 148–152. https://doi.org/10.1016/s0014-5793(03)01395-4.
- Joshi, G. S., J. S. Spontak, D. G. Klapper, and A. R. Richardson. 2011. “Arginine Catabolic Mobile Element Encoded SpeG Abrogates the Unique Hypersensitivity of Staphylococcus Aureus to Exogenous Polyamines.” Molecular Microbiology 82 (1): 9–20. https://doi.org/10.1111/j.1365-2958.2011.07809.x.
- Jóźwik, W., S. Kozioł, K. Matecki, M. Neska, J. Przybylski, M. Wiśniewski, A. Zbrowski, et al. 2017. “A Prototype of a Technological Line for Processing Decorticated Flax Fibre.” Journal of Machine Construction and Maintenance-Problemy Eksploatacji 1 (104): 73–79.
- Kozasowski, R. M., M. Mackiewicz-Talarczyk, and A. M. Allam. 2012. “5 - Bast Fibres: Flax.” In Handbook of Natural Fibres, edited by R. M. Kozłowski, 56–113. Sawston, Cambridge: Woodhead Publishing. https://doi.org/10.1533/9780857095503.1.56.
- Kozłowski, R., Z. Czaplicki, S. Zaręba, and J. Mańkowski. 2012. “OE Cotton Yarns with a High Content of Enzyme-Modified Flax Fibers.” Journal of Natural Fibers 9 (3): 137–149. https://doi.org/10.1080/15440478.2011.630224.
- Kulma, A., K. Skórkowska-Telichowska, K. Kostyn, M. Szatkowski, J. Skała, Z. Drulis-Kawa, M. Preisner, et al. 2015. “New Flax Producing Bioplastic Fibers for Medical Purposes.” Industrial Crops and Products 68:80–89. https://doi.org/10.1016/j.indcrop.2014.09.013.
- Kwon, D. H., and C. D. Lu. 2006. “Polyamines Increase Antibiotic Susceptibility in Pseudomonas aeruginosa.” Antimicrobial Agents and Chemotherapy 50 (5): 1623–1627. https://doi.org/10.1128/aac.50.5.1623-1627.2006.
- Li, Z., C. Zhao, X. Zhao, Y. Xia, X. Sun, W. Xie, Y. Ye, X. Lu, and G. Xu. 2018. “Deep Annotation of Hydroxycinnamic Acid Amides in Plants Based on Ultra-High-Performance Liquid Chromatography–High-resolution Mass Spectrometry and its in silico Database.” Analytical Chemistry 90 (24): 14321–14330. https://doi.org/10.1021/acs.analchem.8b03654.
- Manganaris, G. A., A. R. Vicente, C. H. Crisosto, and J. M. Labavitch. 2008. “Cell Wall Modifications in Chilling-Injured Plum Fruit (Prunus salicina).” Postharvest Biology and Technology 48 (1): 77–83. https://doi.org/10.1016/j.postharvbio.2007.09.017.
- Melelli, A., D. U. Shah, G. Hapsari, R. Cortopassi, S. Durand, O. Arnould, V. Placet, et al. 2021. “Lessons on Textile History and Fibre Durability from a 4,000-Year-Old Egyptian Flax Yarn.” Nature Plants 7 (9): 1200–1206. https://doi.org/10.1038/s41477-021-00998-8.
- Mierziak, J., W. Wojtasik, K. Kostyn, T. Czuj, J. Szopa, and A. Kulma. 2014. “Crossbreeding of Transgenic Flax Plants Overproducing Flavonoids and Glucosyltransferase Results in Progeny with Improved Antifungal and Antioxidative Properties.” Molecular Breeding 34 (4): 1917–1932. https://doi.org/10.1007/s11032-014-0149-5.
- Musialak, M., M. Wróbel-Kwiatkowska, A. Kulma, E. Starzycka, and J. Szopa. 2008. “Improving Retting of Fibre Through Genetic Modification of Flax to Express Pectinases.” Transgenic Research 17 (1): 133–147. https://doi.org/10.1007/s11248-007-9080-4.
- Preisner, M., W. Wojtasik, A. Kulma, M. Zuk, and J. Szopa. 2014. “Flax Fiber.” ECT, edited by E. A. Danny, 5th ed. Vol. 1. 588–623. Russell Research Center, ARS-USDA.
- Prior, R. L., X. Wu, and K. Schaich. 2005. “Standardized Methods for the Determination of Antioxidant Capacity and Phenolics in Foods and Dietary Supplements.” Journal of Agricultural & Food Chemistry 53 (10): 4290–4302. https://doi.org/10.1021/jf0502698.
- Różańska, W., N. Kryszak, E. Nowaczkiewicz, R. Kozlowski, J. Wasim, and W. Podsiedlik. 2012. “Osmosis Phenomena Based Degumming of Bast Fibrous Plants as a Promising Method in Primary Processing.” Molecular Crystals and Liquid Crystals - MOL CRYST LIQUID CRYST 556 (1): 264–274. https://doi.org/10.1080/15421406.2012.635976.
- Rumpf, J., R. Burger, and M. Schulze. 2023. “Statistical Evaluation of DPPH, ABTS, FRAP, and Folin-Ciocalteu Assays to Assess the Antioxidant Capacity of Lignins.” International Journal of Biological Macromolecules 233:123470. https://doi.org/10.1016/j.ijbiomac.2023.123470.
- Shukla, V. K. S., P. C. Dutta, and W. E. Artz. 2002. “Camelina Oil and Its Unusual Cholesterol Content.” Journal of the American Oil Chemists’ Society 79 (10): 965–969. https://doi.org/10.1007/s11746-002-0588-1.
- Skórkowska-Telichowska, K., M. Żuk, A. Kulma, A. Bugajska-Prusak, K. Ratajczak, K. Gąsiorowski, K. Kostyn, and J. Szopa. 2010. “New Dressing Materials Derived from Transgenic Flax Products to Treat Long-Standing Venous Ulcers—A Pilot Study.” Wound Repair and Regeneration 18 (2): 168–179. https://doi.org/10.1111/j.1524-475X.2010.00578.x.
- Ververis, C., K. Georghiou, N. Christodoulakis, P. Santas, and R. Santas. 2004. “Fiber Dimensions, Lignin and Cellulose Content of Various Plant Materials and Their Suitability for Paper Production.” Industrial Crops and Products 19 (3): 245–254. https://doi.org/10.1016/j.indcrop.2003.10.006.
- Wojtasik, W., M. Czemplik, M. Preisner, L. Dymińska, G. Yuan, J. Szopa, and A. Kulma. 2017. “Pectin from Transgenic Flax Shives Regulates Extracellular Matrix Remodelling in Human Skin Fibroblasts.” Process Biochemistry 55:187–198. https://doi.org/10.1016/j.procbio.2017.02.001.
- Wojtasik, W., A. Kulma, L. Dymińska, J. Hanuza, M. Czemplik, and J. Szopa. 2016. “Evaluation of the Significance of Cell Wall Polymers in Flax Infected with a Pathogenic Strain of Fusarium oxysporum.” BMC plant biology 16 (1): 75. https://doi.org/10.1186/s12870-016-0762-z.
- Wojtasik, W., A. Kulma, L. Dymińska, J. Hanuza, J. Żebrowski, and J. Szopa. 2013. “Fibres from Flax Overproducing β-1,3-Glucanase Show Increased Accumulation of Pectin and Phenolics and Thus Higher Antioxidant Capacity.” BMC Biotechnology 13 (1): 10. https://doi.org/10.1186/1472-6750-13-10.
- Wojtasik, W., A. Kulma, K. Namysł, M. Preisner, and J. Szopa. 2015. “Polyamine Metabolism in Flax in Response to Treatment with Pathogenic and Non–Pathogenic Fusarium Strains.” Frontiers in Plant Science 6:291. https://doi.org/10.3389/fpls.2015.00291.
- Wojtasik, W., K. Majewska, L. Dymińska, J. Hanuza, M. Zimniewska, M. Preisner, J. Szopa, and M. Wróbel-Kwiatkowska. 2023. “Optimization of Hydrodynamic Degumming of Flax Fiber for Improved Biochemical Profile.” Industrial Crops and Products 206:117621. https://doi.org/10.1016/j.indcrop.2023.117621.
- Wróbel-Kwiatkowska, M., K. Lorenc-Kukula, M. Starzycki, J. Oszmiański, E. Kepczyńska, and J. Szopa. 2004. “Expression of β-1,3-Glucanase in Flax Causes Increased Resistance to Fungi.” Physiological and Molecular Plant Pathology 65 (5): 245–256. https://doi.org/10.1016/j.pmpp.2005.02.008.
- Wróbel-Kwiatkowska, M., J. Zebrowski, M. Starzycki, J. Oszmiański, and J. Szopa. 2007. “Engineering of PHB Synthesis Causes Improved Elastic Properties of Flax Fibers.” Biotechnology Progress 23 (1): 269–277. https://doi.org/10.1021/bp0601948.
- Wu, Z., J. Kang, C. Zhang, W. Zhang, and J. Ge. 2023. “Assessing the Promoting Effect of Compound Microbial Agents on Flax Dew Retting: Based on the Relationship Between Metabolites and Core Genera.” Bioresource Technology 385:129451. https://doi.org/10.1016/j.biortech.2023.129451.
- Yan, L., N. Chouw, and K. Jayaraman. 2014. “Flax Fibre and its Composites – a Review.” Composites Part B Engineering 56:296–317. https://doi.org/10.1016/j.compositesb.2013.08.014.
- Zeiss, D. R., L. A. Piater, and I. A. Dubery. 2021. “Hydroxycinnamate Amides: Intriguing Conjugates of Plant Protective Metabolites.” Trends in Plant Science 26 (2): 184–195. https://doi.org/10.1016/j.tplants.2020.09.011.
- Zimniewska, M., W. Różańska, A. Kicińska-Jakubowska, J. Mańkowski, M. Wiśniewski, K. Dziedziczak, and P. Baraniecki. 2022. “Influence of the Hydrodynamic Degumming Process on the Quality of Decorticated Flax Fibers.” Textile Research Journal 92 (17–18): 3316–3336. https://doi.org/10.1177/00405175211056982.
- Zimniewska, M., A. Zbrowski, W. Różańska, A. Majcher, J. Przybylski, K. Matecki, M. Wiśniewski, J. Mańkowski, and A. Kicińska-Jakubowska. 2017. “Cottonisation of Decorticated Flax Fibres.” Fibres & Textiles in Eastern Europe 25 (0): 26–33. https://doi.org/10.5604/01.3001.0010.1685.
- Zuk, M., A. Dorotkiewicz-Jach, Z. Drulis-Kawa, M. Arendt, A. Kulma, and J. Szopa. 2014. “Bactericidal Activities of GM Flax Seedcake Extract on Pathogenic Bacteria Clinical Strains.” BMC Biotechnology 14 (1): 70. https://doi.org/10.1186/1472-6750-14-70.