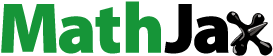
ABSTRACT
Environmental contamination poses a significant threat to the integrity of materials, including metals and composites. Addressing the degradation of natural fiber-reinforced polymer composites necessitates effective management strategies, particularly in mitigating UV and water-induced deterioration. This study explores the efficacy of Acrylated Epoxidized Soybean Oil (AESO) coating treatment following alkali treatment as a potential solution. Alkali treatment of Kapok fiber (KF) combined with polymer coating yielded composites exhibiting superior mechanical properties, particularly in tensile and flexural strength, following a 30-day aging period. Remarkably, the application of alkali and coating treatments led to a substantial enhancement in the mechanical strength of polyester composites, with the highest tensile strength recorded at 96.04 MPa. This represented a notable increase of 31.97% compared to untreated KF specimens. The observed enhancement in composite performance underscores the critical role of interfacial adhesion between fibers and the matrix, particularly under conditions of UV exposure and water immersion. Importantly, the study demonstrates that alkali treatment and polymer coating effectively mitigate degradation in Natural Fiber-Reinforced Polymer Composites, thereby offering promising avenues for enhancing their durability and longevity.
摘要
环境污染对包括金属和复合材料在内的材料的完整性构成重大威胁. 解决天然纤维增强聚合物复合材料的退化问题需要有效的管理策略,特别是在减轻紫外线和水引起的退化方面. 本研究探讨了碱处理后丙烯酸酯环氧化大豆油 (AESO) 涂层处理作为潜在解决方案的功效. Kapok纤维 (KF) 的碱处理与聚合物涂层相结合,在30天的老化期后,产生了具有优异机械性能的复合材料,特别是在拉伸和弯曲强度方面. 值得注意的是,碱和涂层处理的应用大大提高了聚酯复合材料的机械强度,最高抗拉强度为96.04 MPa. 与未处理的KF样本相比,这表示显著增加了31.97%. 观察到的复合材料性能的增强强调了纤维和基体之间界面粘附的关键作用,特别是在紫外线照射和浸水条件下. 重要的是,该研究表明,碱处理和聚合物涂层有效地减轻了天然纤维增强聚合物复合材料的降解,从而为提高其耐用性和寿命提供了有前景的途径.
Introduction
Composite materials are crucial for sustainable building due to their versatility and lightweight properties. However, they face durability challenges from environmental factors like water absorption and UV light, posing a significant challenge to the construction industry (Nor Arman, Chen, and Ahmad Citation2021). Natural fiber-reinforced polymer composites (NFRPs) are increasingly being used as sustainable alternatives to traditional materials. However, their performance is highly dependent on the adhesion between the polymer matrix and fiber additives. The hydrophilic and hydrophobic properties of natural fibers can lead to poor adhesion, reduced mechanical properties, and increased moisture absorption, ultimately compromising the durability of NFRPs (Wang et al. Citation2021).
Despite numerous advancements in enhancing the performance of natural fiber composites, their susceptibility to moisture, water absorption, and weathering remains a significant challenge. This vulnerability is primarily due to the lignocellulosic composition of natural fibers. While past research has explored various methods to improve these composites, such as surface modifications through alkali solutions, bleaching, and ultrasonication (Beg, Pickering, and Gauss Citation2023; Sheeba et al. Citation2023), there is limited understanding of the combined effects of these treatments, specifically Acrylated Epoxidized Soybean Oil (AESO) coating, on UV radiation resistance and water retention (Bakar and Kamke Citation2021; Liu and Tisserat Citation2018).
This study investigates the effectiveness of alkali chemical treatment and AESO coating in improving the mechanical properties, water absorption resistance, and UV light resistance of Kapok Fiber reinforced polymer composites. KF’s unique properties make it a promising composite reinforcement, and our study aims to explore its sustainability and durability in construction applications. This research integrates alkali treatment and AESO coating for fiber modification, assessing their synergistic effects on NFRPs performance, and focusing on KF, a less studied material in sustainable construction materials.
Materials and method
Materials
KF (Ceiba pentandra fiber) was collected in Lombok Tengah by manually extracting it from its tree bark. The tree bark was stripped and subjected to water retting involving immersion in water-filled basin for a period of 7–15 days, resulting in decay caused by bacterial action. After retting, the KF sheet was manually separated from the bark. The fiber sheet was then cleaned, dried, and set aside to be used as a composite reinforcement. The characteristics of KF are shown in . The methyl ethyl ketone peroxide (MEKP) and the unsaturated polyester resin employed in the study were sourced from PT Justus Kimia Raya, Surabaya, Indonesia. This resin plays a crucial role in the composite manufacturing process, serving as the matrix material that binds the KF fibers together.
Table 1. The physical characteristics, composition, and types of treatment of KF serve as polyester reinforcement.
Fiber treatment
Four different types of KF and their physical properties were used as reinforcement for unsaturated polyester resin, as outlined in . To undertake the alkaline treatment, a 7% potassium hydroxide (KOH) solution was used. Specifically, 50 g of KF was immersed in 5000 ml of 7% KOH solution for a duration of 120 min. Following this, the KF was rinsed multiple times to ensure the removal of any residual KOH. Subsequently, the fiber was sun dried and stored in an airtight plastic container.
The agents of AESO coating used consisted of Soybean oil, epoxidized, acrylate dan Hydroquinone monomethyl ether with each concentration of ≤ 100%, respectively ≥ 0.25, < 113°C. The density of AESO was 1.04 g/cm3 with flash point of no > 113°C (Sigma-Aldrich, Singapore). The preparation of AESO solution for coating the KF was based on a previous study (Liu and Tisserat Citation2018). The process began by mixing 100 g AESO in 1000 ml ethanol using magnetic stirrer for 30 min. Then, 0.05 g of Irgacure ® 819 was added to the mixture, which was then stirred for an additional 30 min under room temperature conditions. The resulting coating solution was stored at a room temperature and made ready for application. The photopolymerization of the KF was accomplished by immersing 100 g of KF in the coating solution for 10 min, followed by exposing its radiant light from a 450 Watts UV. This UV light source was positioned within the chamber, and the KF was placed beneath it to facilitate the photopolymerization process. Both the raw KF and KOH-treated KF were subjected to this coating using AESO polymer. Following this, the coated KF was ready to use as the composite reinforcement.
Composite fabrication
KF reinforced polyester composites were fabricated using hand lay-up method with longitudinal continuous fiber direction (see ). Each of the four types of KF composite was prepared using 30% of fiber volume fraction. The composites mold was made up of steel plates and silicone rubber based on the shape and dimensions of testing standards. The polyester resin was then mixed with 1% catalyst of the resin volume fraction until a homogeneous was achieved. The mixture was poured into the mold filled with KF until it wetted the fiber perfectly. The mold was covered with a steel plate, allowing an aging period of 24 h for the composite material to set. Then, the specimen was removed from the mold and prepared for mechanical property testing. Detailed information on the modification treatment and composition for each composite variation is provided in .
Bending test
The flexural resistance of the polyester composites was assessed using the three-point bending method, following the guidelines outlined in the ASTM D790 standard. Each specimen’s dimensions (p x l x h) were 150 mm × 25.4 × 6 mm, respectively. The testing was conducted utilizing a Universal Tensile Machine (UTM) RTG-1310. The span to depth ratio was 16:1. The crosshead speed during the testing was set to 5 mm/min, and the machine had a loading capacity of 20 kN. To ensure accuracy and precision, five results were determined for each specimen specification.
Tensile test
The Universal Tensile Machine (UTM) RTG-1310 was used for tensile testing on 20 composite specimens, with five specimens fractured at a loading speed of 5 mm/min. Using a gauge length of 150 mm, the tensile specimens for composites were tested in accordance with the ASTM D3039 standard in this study.
Degradation of tensile strength
The ASTM D 638–10 standard was followed to assess the impact of water adsorption on the mechanical properties of the composite. The composite samples, immersed in water for 30 days, underwent a tensile test to evaluate the impact of water adsorption on their resistance and mechanical behavior.
UV light testing
The composite samples were subjected to UV light irradiation in accordance with the ASTM D4329–99 standard. Four composites, totaling 20 samples, were exposed to UV light uniformly using weathering test equipment and accelerated with a LUV-II apparatus (Pushen, Shanghai, China). The automatic exposure system, equipped with heating equipment, exposed composite specimens to 60 W shortwave UV light for 30 days, followed by tensile tests to assess its mechanical properties.
Morphology (SEM)
The fracture defects and failure of composite specimens were studied using SEM (JEOL, JSM-SS200), coated with gold for conductive properties, and observed at 3 kV and 15 mA operating voltage.
Water absorption test
The study examined the water absorption of KF-reinforced composites by immersing them in distilled water, removing moisture, and measuring their initial weight. Prior to that, the composite samples were put in an oven to remove the adsorbed content or moisture at temperature of 105°C until their weight was stable. Then, the initial weight of the samples was precisely measured using analytical balance with a precision of 0.0001 g. The process was repeated three times for each composite type, resulting in 12 samples being tested. The percentage of water absorbed by the composite was calculated using Equation 1
Water absorption testing was conducted every 5 days of intermittent immersion until day 30. W1 represents the weight after immersion, while W0 represents the weight before immersion.
Results and discussion
Tensile strength
shows the tensile strength of KF reinforced composites, revealing that the Coating-KF composite did not yield a significant effect on tensile strength. The raw KF composite exhibited a tensile strength of 71.21 MPa, while the coated-KF composite has tensile strength of 75.39 MPa, representing an increase of 5.87%. This can be attributed to the fact that the coating layer on KF primarily functioned as a matrix and did not alter the composition of the KF. Furthermore, the result of the study indicated that coating treatment on KF did not substantially affect the polyester composite’s tensile strength, although it did lead to a reduction in bending strength. In contrast, following the alkali treatment, the tensile strength exhibited a significant increase of 31.97% compared to the raw-KF composite. This enhancement is attributed to the removal of lignin, wax, and hemicellulose during the alkali treatment process. These components interfere with the bonding sites between KF and the polyester matrix. Additionally, the water absorption test may have made the cellulose areas more accessible (Bakar and Kamke Citation2021). The composite mechanical strength on KF increased post-alkali treatment due to reduced inter-fibrillar materials and increased alkali solution concentration. This increased interfacial interaction, effective surface area, and load transfer potential were enhanced. The reduction in fiber diameter was linked to weight loss. The coated-fiber composite in NFRP exhibits lower water absorption compared to uncoated fiber composites, but polymer coating doesn’t significantly alter the mechanical properties (Liu and Tisserat Citation2018).
Figure 2. (a) Elongation; (b) tensile strength and tensile modulus of KF-reinforced polyester composites.
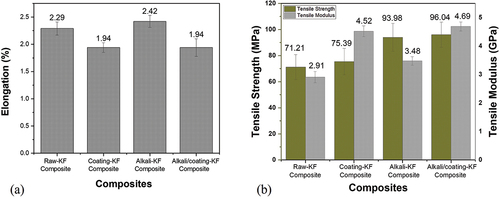
shows a correlation between elongation data and tensile properties of KF reinforced polyester composite. Raw-KF composite has a 2.91 GPa tensile modulus, while coating-KF composite has a 4.52 GPa modulus. Alkali/coating-KF composite has the highest modulus of 4.70 GPa, due to bond chain formation. The bond chain in the KF-reinforced polyester composite increases stiffness and brittleness due to its restriction of KF mobility during tensile loading. The alkali treatment of KF dissolves cementing components like hemicellulose and lignin, reducing their surface and causing fibrillation. This leads to increased interlocking between KF and polyester, enhancing deformation resistance and reducing tensile strains. Additionally, the treatment transforms cellulose I into cellulose II, resulting in tighter chains (Sheeba et al. Citation2023). This enhances the stiffness and strength of individual natural fibers.
The elongation data shows that composite elongation decreases after coating, with raw-KF composites decreasing to 1.94%. However, alkali-KF composites show an increase to 2.42% after alkali treatment, while alkali/coating-KF composites decrease to 1.94%. The alkali-KF composite’s elongation increases by 5.24%. This happens because of adding an AESO coating to KF that has been treated with alkali. The coating makes the composite less flexible and more brittle by mechanically bonding the KF to the AESO resin in the polyester matrix. It also increases the composite’s resistance to crack initiation and propagation. This, in turn, reduces the composite’s elongation.
Flexural strength
The flexural properties of KF-reinforced polyester composites show that alkali treatment significantly increases flexural strength, while coating on KF slightly increases it to 95.015 MPa, suggesting no significant impact on the composite’s bending strength due to impurities. The KF-polyester interface exhibits weak bonding, causing low bending strength and clumping of KF, while the AESO coating fails to effectively interlock with the KFs.
shows alkali treatment significantly increased flexural strength and composite stiffness in KF composites, with a 32.65% increase compared to raw-KF composites and a 35.21% increase in alkali/coating-KF composites, highlighting its impact on surface roughness (Gapsari et al. Citation2023). Also, the alkali treatment improves fiber incorporation by removing lignin and hemicellulose, enhancing the interaction between the fiber and the matrix. The alkali and coating treatment enhances flexural strength in KF reinforced composite due to bond interaction with polyester matrix, facilitated by AESO coating. However, coating treatment on fiber does not significantly impact flexural strength (Sheeba et al. Citation2023)
shows that the flexural modulus of KF-reinforced polyester composites increases after coating treatments. The AESO polymer coating increases KF’s stiffness, leading to interfacial bonds. The raw-KF composite’s flexural modulus increased by 43.79% after coating, while alkali-KF composites and alkali/coating-KF composites show higher modulus.
The composite’s fiber distribution is significantly influenced by the flow of polyester resin during pouring, with clumping or agglomeration of KF typically occurring during fabrication (Chen et al. Citation2022). Improper load transfer from resin to fiber can occur due to weak fiber-matrix interfacial adhesion, resulting in the resin supporting most of the load (Wang et al. Citation2021).
Water absorption
shows water absorption percentages in KF reinforced polyester composites. Raw-KF composites showed the highest absorption at 3.91%, followed by alkali-KF composites at 3.68%. Coated-Alkali KF composites had a lower absorption at 3.31% due to hydrophobic fatty acid chains and alkali-KF composites’ higher absorption.
The study shows that a two-stage treatment process using alkali and coating effectively reduces water absorption in composites, with the lowest percentage at 2.98%, due to reduced hemicellulose and lignin compounds (Abdel-Hakim, El Mogy, and Mourad Citation2024). The coating process creates a barrier, preventing water diffusion into the KF, resulting in the lowest water absorption of the alkaline/coating-KF composite compared to other materials.
KF, made of lignocellulose, is susceptible to water adsorption, causing degradation in dimension stability and mechanical properties of natural fiber-reinforced polyester composites. Moisture is typically absorbed by the fiber-matrix interface and the fiber itself through hydrogen bond. Natural fiber’s hydrophilic properties enhance water absorption in composites, influenced by resin cavities, high humidity and temperature conditions, and water molecules binding to polyester structure (Abdel-Hakim, El Mogy, and Mourad Citation2024). Hygrothermal environments cause fiber/matrix bond degradation due to local interfacial degradation, lowering adhesion energy, and preventing stress transfer and promoting surface mismatches (Wang et al. Citation2021, Citation2022; Wu et al. Citation2023).
UV-Light resistance
show that the tensile strength and flexural strength of KF composites increased after UV light exposure, with the highest strength and modulus observed in the alkali/coated-KF composite. UV light exposure accelerates degradation of composites due to over-evaporation of water in the fiber/composite, causing a decrease in tensile strength due to less dense interface bonds between the fiber and resin. The composite tensile modulus after UV light exposure varies, with the highest value (4.02 GPa) in alkali/coated-KF composite and the lowest (2.14 GPa) in raw-KF composite.
Figure 5. (a) Tensile strength and tensile modulus after UV light exposure; (b) flexural strength and flexural modulus after UV light exposure; (c) tensile strength and tensile modulus post water immersion; and (d) flexural strength and flexural modulus after water immersion of KF-reinforced polyester composites.
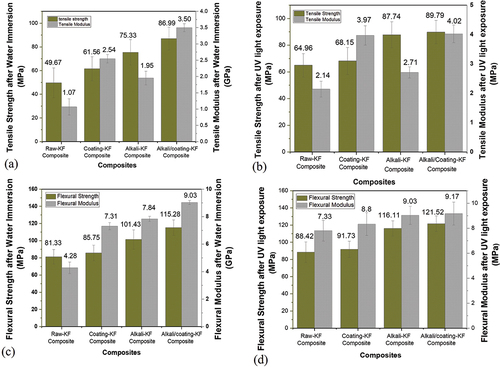
UV-sensitive polymer composites, particularly natural fibers, exhibit poor weathering resistance, leading to increased brittleness, cracks, and erosion, potentially reducing their mechanical properties and service life (Sheeba et al. Citation2023). Polymer degradation involves microstructural aging, weakening chemical covalent bonds, influenced by external factors like mechanical stress, radiation, and temperature, affecting material appearance and quality (Santos et al. Citation2023). UV radiation oxidizes cellulose, affecting fiber strength in composites. The aging rate of KF depends on composition, structure, intensity, and service environment, with photooxidative degradation accelerated by chromophore groups (Han et al. Citation2021).
The KF composite’s tensile strength remains consistent after 30 days of immersion, with the alkali/coating-KF composite exhibiting the highest strength at 86.98 MPa, attributed to enhanced interactions between KF-coated polyester layers (Gapsari et al. Citation2023). The tensile modulus of KF reinforced polyester composite showed similar behavior before and after immersion (). The tensile modulus of alkali/coating-KF composite is highest, indicating increased stiffness due to hydrophilic substance removal during alkali treatment and KF coating, resulting in stronger mechanical interfacial interlock. The immersion process produces a composite with higher tensile strength and modulus, while the tensile modulus decreases due to water damage to raw-KF and uncoated alkali-KF composites.
shows flexural properties of KF-reinforced polyester composite before and after water immersion and UV light exposure. The raw KF composite exhibited the lowest bending strength, which can be attributed to impurities on the surface compromising its flexural strength and resistance to damage. The flexural strength of a composite increases after coating and alkali treatment due to improved bond between KF and matrix. However, after immersion in water and UV light, the bending strength decreases due to fibers pulling away from the matrix. This reduction is more pronounced in KF composites exposed to UV light (Sergi et al. Citation2019). The treatment of KF with alkali and coating enhances interfacial adhesion, prevents water accumulation, and reduces hydroxyl group vulnerability, thereby reducing water uptake (Gapsari et al. Citation2023). KF composites experience swelling due to water molecules infiltrating the fiber-matrix interface, forming voids, and pulling fibers away. These voids act as water transportation pathways, reducing interfacial bonding more severely than UV exposure(Vijayan, Aparna, and Sahoo Citation2023). Chemical bonds between fibers and matrix facilitate strong interfacial adhesion, while rougher fiber surfaces enhance adhesion strength and resistance to phase slide.
UV exposure affects KF reinforced composite properties, increasing brittleness, cracking susceptibility, and erosion. Alkali/coating-KF composite shows higher bending properties compared to other composites. The two-step treatment of KF composites, involving alkali treatment and polymer coating, enhances cellulose content and mechanical properties. UV radiation degradation affects bending properties, but lignin in KF fibers preserves flexural strength and modulus, mitigating UV-induced degradation (Sergi et al. Citation2019). The comparison of chemical treatment effect to water absorption and UV light exposure summarizes in .
Table 2. Effect of fiber chemical treatment on water absorption and UV light exposure.
Morphology (SEM)
displays the fracture morphology of KF reinforced polyester composite, revealing fiber pullout in the KF and micro cracks on the polyester matrix.
Figure 6. SEM photos of the fracture of (a) alkali/KF-composite; (b) alkali/KF-composite after UV exposure; (c) alkali/KF-composite after immersion.
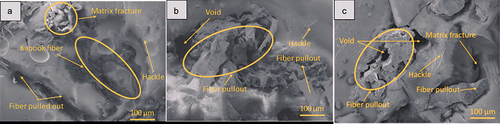
In a robust surface bond between KF and polyester is observed, this is a result of the removal of lignin compounds, waxes, and other impurities through KOH treatment. This strong surface bond enhances the mechanical strength of the composite significantly. However, in , a reduction in the surface bonding of KF with polyester is evident, which can be attributed to aging-induced changes in the composite’s mechanical properties. These changes result from the molecular decomposition reactions and chain reactions. Furthermore, surface degradation induced by UV radiation contributes to this effect (Santos et al. Citation2023). . illustrates a compromised KF-polyester surface bond and fiber pullout due to the presence of impurities on the KF surface. This phenomenon is associated with water absorption by the KF-reinforced polyester composite during immersion. Notably, clumping occurs on the KF reinforced polyester composite due to the immersion process. Additionally, the composite exhibits a relatively high Hackle region as a result of the interaction between AESO polymer and polyester.
Conclusions
The study investigated the effect of alkali treatment and KF coating on the slowing down of degradation in a polyester composite reinforced with KF. The treatment of natural fiber reinforced polyester (NFRP) composite with alkali and coating processes effectively mitigated its degradation when exposed to UV light and water immersion. Alkali and coating treatment improves the mechanical strength of polyester composites; the tensile strength increased significantly (31.97%), and the flexural strength increased significantly (35.21%) compared to the raw KF composite. The two-stage modification of KF effectively counteracted UV light-induced aging and water absorption, thereby enhancing the mechanical properties of polyester composites. The composite performance was significantly enhanced by the interfacial adhesion between fibers and the matrix following UV light exposure and water immersion. The study found that a combination of alkali treatment and KF coating significantly improved the resistance of polyester composites to degradation, especially when exposed to aging, UV radiation, and water immersion.
Highlights
One of the primary causes of materials degradation is environmental contamination.
The stability of a natural fiber-reinforced polymer composite (NFRP) against UV exposure and water is crucial for managing its degradation.
Composite degradation can be managed through coating treatment after an alkaline treatment.
The degradation of NFRP can be effectively controlled through alkali treatment and polymer coating on fibers.
Supplemental Material
Download MS Word (13.7 KB)Acknowledgement
This research was funded by the Riset Kolaborasi Indonesia (Collaborative Research) - RKI by three universities: Brawijaya University 801.14/UN10.C10/TU/2023, State University of Malang 9.5.81/UN32/KP/2023, and Sebelas Maret University, 590.1/UN27.22/HK.07.00/2023. We also thank to National Science, Research and Innovation Fund (NSRF) (Fundamental Fund 2024), and King Mongkut’s University of Technology North Bangkok (Project no. KMUTNB-FF-67-A-03) for collaboration and support.
Supplementary material
Supplemental data for this article can be accessed online at https://doi.org/10.1080/15440478.2024.2383970
Disclosure statement
No potential conflict of interest was reported by the author(s).
References
- Abdel-Hakim, A., S. A. El Mogy, and R. M. Mourad. 2024. “Impact of Fiber Coating with Hydrophobic Nanoparticles Polymer on the Physicomechanical Properties of Pisum Sativum L. Fibers/Styrene Butadiene Rubber Ecofriendly Composites.” Industrial Crops and Products 209:209. https://doi.org/10.1016/j.indcrop.2024.118054.
- Bakar, B. F. A., and F. A. Kamke. 2021. “Alkali Concentration and Diluent Effects on Properties of Grape Cane Fiber-Reinforced Polymer Composites.” Polymers 13 (23): 4055. https://doi.org/10.3390/polym13234055.
- Beg, M. D. H., K. L. Pickering, and C. Gauss. 2023. “The Effects of Alkaline Digestion, Bleaching and Ultrasonication Treatment of Fibre on 3D Printed Harakeke Fibre Reinforced Polylactic Acid Composites.” Composites Part A, Applied Science and Manufacturing 166:107384. https://doi.org/10.1016/j.compositesa.2022.107384.
- Chen, T., C. Su, Y. Zeng, Y. Chen, R. Qiu, and W. Liu. 2022. “Effects of Hydrogen Bonds on Soybean Oil-Based Thermosets and Their Bamboo Fibers Composites.” Composites Communications 33:33. https://doi.org/10.1016/j.coco.2022.101231.
- Gapsari, F., D. B. Darmadi, H. Juliano, S. Hidayatullah, S. M. Rangappa, S. Siengchin, and S. Siengchin. 2023. “Modification of Palm Fiber with Chitosan-AESO Blend Coating.” International Journal of Biological Macromolecules 242 (4): 125099. https://doi.org/10.1016/j.ijbiomac.2023.125099.
- Han, H. C., X. L. Gong, M. Zhou, C. Li, and H. B. Yang. 2021. “A Study About Silane Modification and Interfacial Ultraviolet Aging of Hemp Fiber Reinforced Polypropylene Composites.” Polymer Composites 42 (5): 2544–11. https://doi.org/10.1002/pc.26000.
- Liu, Z., and B. H. Tisserat. 2018. “Coating Applications to Natural Fiber Composites to Improve Their Physical, Surface and Water Absorption Characters.” Industrial Crops and Products 112:196–199. https://doi.org/10.1016/j.indcrop.2017.12.002.
- Nor Arman, N. S., R. S. Chen, and S. Ahmad. 2021. “Review of State-Of-The-Art Studies on the Water Absorption Capacity of Agricultural Fiber-Reinforced Polymer Composites for Sustainable Construction.” Construction and Building Materials 302 (July): 124174. https://doi.org/10.1016/j.conbuildmat.2021.124174.
- Santos, T., C. Santos, M. Aquino, S. Mavinkere Rangappa, S. Siengchin, J. H. O. Nascimento, and I. Medeiros. 2023. “Effects of UV Sensitivity and Accelerated Photo-Aging on Stab Resistance of ρ-Aramid Fabrics Impregnated with Shear Thickening Fluids (STFs).” Heliyon 9 (4): e15020. https://doi.org/10.1016/j.heliyon.2023.e15020.
- Sergi, C., J. Tirillò, M. C. Seghini, F. Sarasini, V. Fiore, and T. Scalici. 2019. “Durability of Basalt/Hemp Hybrid Thermoplastic Composites.” Polymers 11 (4): 603. https://doi.org/10.3390/polym11040603.
- Sheeba, K. R. J., R. K. Priya, K. P. Arunachalam, S. Avudaiappan, E. S. Flores, and P. Kozlov. 2023. “Enhancing Structural, Thermal, and Mechanical Properties of Acacia Pennata Natural Fibers Through Benzoyl Chloride Treatment for Construction Applications.” Case Studies in Construction Materials 19:19. https://doi.org/10.1016/j.cscm.2023.e02443.
- Vijayan, S. P., S. Aparna, and S. K. Sahoo. 2023. “Effect of Beeswax on Hydrophobicity, Moisture Resistance and Transparency of UV Curable Linseed Oil Based Coating for Compostable Paper Packaging.” Industrial Crops and Products 197:197. https://doi.org/10.1016/j.indcrop.2023.116645.
- Wang, X. Q., O. Büyüköztürk, C. K. Y. Leung, and D. Lau. 2022. “Atomistic Prediction on the Degradation of Vinylester-Based Composite Under Chloride and Elevated Temperature.” Composites Science and Technology 226:226. https://doi.org/10.1016/j.compscitech.2022.109539.
- Wang, X. Q., W. Jian, O. Buyukozturk, C. K. Y. Leung, and D. Lau. 2021. “Degradation of Epoxy/Glass Interface in Hygrothermal Environment: An Atomistic Investigation.” Composites Part B Engineering 206:108534. https://doi.org/10.1016/j.compositesb.2020.108534.
- Wu, R., X. Q. Wang, D. Zhao, J. A. Hou, C. Wu, D. Lau, and L. H. Tam. 2023. “Degradation of Fiber/Matrix Interface Under Various Environmental and Loading Conditions: Insights from Molecular Simulations.” Construction and Building Materials 390:131101. https://doi.org/10.1016/j.conbuildmat.2023.131101.