Abstract
Chemical allergens represent a significant health burden in the workplace. Exposures to such chemicals can cause the onset of a diverse group of adverse health effects triggered by immune-mediated responses. Common responses associated with workplace exposures to low molecular weight (LMW) chemical allergens range from allergic contact dermatitis to life-threatening cases of asthma. Establishing occupational exposure limits (OELs) for chemical allergens presents numerous difficulties for occupational hygiene professionals. Few OELs have been developed for LMW allergens because of the unique biological mechanisms that govern the immune-mediated responses. The purpose of this article is to explore the primary challenges confronting the establishment of OELs for LMW allergens. Specific topics include: (1) understanding the biology of LMW chemical allergies as it applies to setting OELs; (2) selecting the appropriate immune-mediated response (i.e., sensitization versus elicitation); (3) characterizing the dose (concentration)-response relationship of immune-mediated responses; (4) determining the impact of temporal exposure patterns (i.e., cumulative versus acute exposures); and (5) understanding the role of individual susceptibility and exposure route. Additional information is presented on the importance of using alternative exposure recommendations and risk management practices, including medical surveillance, to aid in protecting workers from exposures to LMW allergens when OELs cannot be established.
INTRODUCTION
The term “chemical allergy” describes immune-mediated adverse health effects, including allergic sensitization and diseases, caused by exposures to chemicals.(Citation1, Citation2) These adverse health effects manifest as a diverse group of disorders caused by an inappropriate immune-mediated response in susceptible individuals following exposure to a foreign substance, or allergen, which is often not otherwise toxic at the concentrations that trigger the allergic response.(Citation3) The immune-mediated responses linked to chemical allergens are complex and vary based on multiple factors including the nature of the allergen, exposure scenario (e.g., route, duration, frequency and magnitude) and individual susceptibility.
illustrates a two-phase model frequently used to characterize the immune response to chemical allergens. The first phase, sensitization (also referred to as induction), follows initial encounters with an allergen that results in an adaptive immune response that primes the immune system for future exposures. The second phase, elicitation, occurs when the sensitized individual receives subsequent exposures to the allergen that triggers an enhanced immune-mediated response recognized clinically as an allergic reaction.(Citation1)
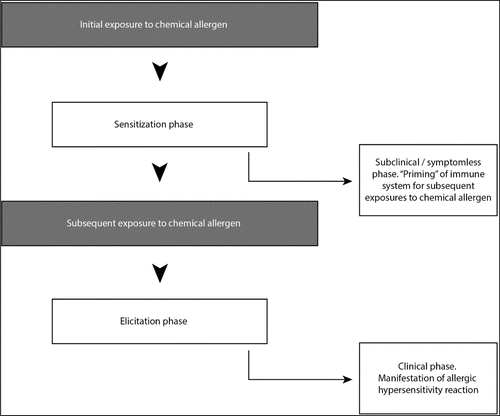
Chemical allergies are among the most common immunological manifestation in humans and represent a significant occupational health hazard.(Citation4) Hundreds of chemical allergens have the potential to cause allergic contact dermatitis (ACD), which accounts for 10–15% of all occupational diseases.(5-8) Allergic respiratory diseases are associated with high levels of worker morbidity.(Citation9) Some chemical allergens are known to be associated with immune-mediated responses of the respiratory tract.(10–12) One common response is asthma, which is a complex syndrome with varying definitions and underlining etiologies.(Citation13) An estimated 9–15% of all cases of adult asthma are associated with occupational factors.(Citation14) Both immunological (Citation14) and irritant mechanisms(15–18) appear to be etiological factors for occupational asthma cases. Other estimates indicate that occupational exposures account for 10–25% of the population attributable risk for adult asthma; equivalent to an incidence of new-onset occupational asthma of 250–300 cases per million annually.(19-23)
Allergens are commonly delineated into two categories based on their mass and the specific immune-mechanisms. Low molecular weight (LMW) allergens typically have a mass less than 5000 Daltons and comprise the majority of the chemical allergens described in this article. These chemicals are typically highly reactive and electrophilic, or are metabolized to reactive forms (prohaptens). Many LMW allergens function as haptens, or incomplete allergens, that can bind to a protein to form a hapten-protein complex that has sufficient mass for recognition by the immune system.(Citation24) Other mechanisms involving direct interaction with components of the immune system may facilitate the immune response for metals.(Citation25, Citation26) Examples of LMW allergens that are recognized occupational hazards include diisocyanates, organic anhydrides and certain metals, such as beryllium and nickel. In contrast, HMW allergens have a mass that exceeds 5000 Daltons allowing for their direct recognition by the immune system. Green and Beezhold identified more than 250 HMW allergens in various work settings ranging from seafood processing to detergent manufacturing.(Citation27)
Controlling occupational exposures to chemical allergens is critical for ensuring the health and well-being of workers. An important tool used to assess workplace exposures and ensure the effectiveness of risk management strategies is an occupational exposure limit (OEL). There is a long history of application of quantitative risk assessment approaches used to develop OELs for non-immune mediated health effects, including portal of entry irritation (i.e., irritation of the upper respiratory tract), non-cancer systemic effects (i.e., neurotoxicity, reproductive, and developmental effects) and cancer.(28-33) Although OEL derivation approaches are fairly well established, they are often less suitable for establishing OELs capable of protecting workers from chemical allergies.
Table 1 ACGIH Threshold Limit Values (TLVs) Based on Immune-mediated Health EndpointsA
provides a list of OELs that have been developed for chemicals allergens. These OELs are primarily based on limited human effects and exposure data. The data are capable of supporting OEL derivation, but significant uncertainties remain in the construction of a dose- or concentration-response relationship between the chemical and onset of allergic sensitization in worker populations. Most chemical allergens have insufficient data to establish a concentration-response relationship preventing the derivation of an OEL based on allergic sensitization or diseases as the critical endpoint. The absence of data also negatively impacts other critical considerations such as the selection of the appropriate immune-mediated response and the characterization of temporal exposure patterns, individual susceptibility, and multiple exposure routes on the development of allergies. For example, diisocyanates represent a group of chemicals highly used in commerce and are recognized respiratory allergens. Despite this profile, there are many open scientific questions that inhibit the full assessment of occupational risk.(Citation34)
The purpose of this article is to highlight the key scientific challenges associated with the establishment of OELs for chemical allergens. Additional information is presented on the use of alternative exposure recommendations and risk management practices to aid in protecting workers from the health risks associated with chemical allergens when OELs cannot be derived. Because of the inherent differences between LMW and HMW allergen response, limited information is presented on HMW allergens. The primary focus of this article is on understanding the process and challenges in establishing OELs for LMW allergens. This focus includes a discussion of the fundamental biological principles for characterizing data on sensitization and diseases associated with LMW allergens.
Key points covered in this article include:
highlighting the biological concepts associated with the onset of chemical allergies as it related to OEL setting;
characterization of the process and challenges associated with setting OELs for chemical allergens; and
exploring alternative exposure recommendations and risk management practices for addressing exposures to chemical allergens when OELs cannot be derived.
UNDERSTANDING THE BIOLOGY OF CHEMICAL ALLERGENS
An appreciation of the basic biological concepts of chemical allergy provides a platform for understanding how occupational risk assessments can more fully account for allergy. Chemical allergy can take a variety of forms. In the context of occupational disease, it is skin sensitization resulting in ACD, and sensitization of the respiratory tract associated with asthma and rhinitis that are of greatest significance. In all instances, chemical allergy, in common with other forms of allergic disease, develops in two phases. The first phase, sensitization, occurs when the naïve individual is exposed to the chemical. During this phase allergen specific effector and memory cells develop, proliferate, and migrate throughout the body, but with greater homing to the initial site of exposure. This phase is clinically silent. Following subsequent exposure, an immune response can be provoked that results in inflammation and the signs and symptoms of allergic disease. All forms of allergy, including chemical allergy, are dependent upon the induction of an immune response, and this distinguishes allergy from other adverse health effects, that may be associated with similar symptoms, but manifestations are the same in both immunologically sensitized and non-sensitized individuals.
Key aspects of immune-mediated responses associated with LMW allergens are summarized below to introduce occupational hygiene professionals to the terms and science encountered in reviewing the literature cited in OEL documents and in evaluating the basis for sensitization notations. More in-depth descriptions of the concepts presented in this section are available in the published literature.(4, 35-42)
Sensitization vs. Elicitation
As indicated above, sensitization describes a state of enhanced responsiveness to a chemical allergen due to immunological priming. The development of sensitization is initiated following recognition of the inducing chemical allergen by the immune system. In this process professional antigen presenting cells (APC) play a pivotal role. Such APC reside at the site of exposure, recognize, process, present, and transport allergen to regional lymph nodes where an adaptive immune response is initiated. The recognition, handling, and transport of antigen in this way involves a number of interacting cellular and molecular immunological mechanisms that together are required for the activation and directed movement of APC towards lymph nodes. In addition, the allergen (haptenated protein) can be carried through lymphatic vesicles to lymph nodes and the blood to the spleen where they are processed by APC and presented to T-cells. Molecular mediators of immunity—cytokines and chemokines—play important roles in orchestrating such events.
One critical event during the acquisition of sensitization is for the creation of a stable association between the chemical allergen and host protein. LMW allergens are too small to be recognized as foreign by the immune system. To acquire immunogenic potential, LMW allergens form what are known as hapten-protein conjugates, which initiate the immune response. Consequently, LMW allergens must be electrophilic, or must be transformed into an electrophilic species following exposure.
A central event in immune sensitization is the presentation of antigen by DC to antigen-responsive T lymphocytes. Antigen activation of responsive T lymphocytes causes clonal expansion and differentiation into various functional subpopulations of cells, some of which will migrate back to the site of exposure. At this point, the individual is sensitized and capable of mounting a clinically relevant hypersensitivity response to the same, or cross-reactive chemical allergen upon re-exposure. The immunological basis for sensitization can take a variety of immunological forms that are described below.
Forms of Chemical Allergy
As indicated above, the two forms of chemical allergy that are of greatest importance in the context of occupational health are skin sensitization resulting in allergic eczema and contact urticaria,(Citation43) in addition to sensitization of the respiratory tract associated with asthma and rhinitis. Both are caused by exposure of susceptible subjects to a LMW allergen, but the form that sensitization takes and the characteristics of subsequent allergic reactions will take different pathways.
Contact allergens are associated with cell-mediated immune responses and delayed type hypersensitivity reactions. In the acquisition of skin sensitization and the elicitation of contact hypersensitivity reactions, allergen-specific T lymphocytes play the major role. More specifically, the key T lymphocytes that mediate these effects are CD4+ Th1/Th17 cells and CD8+ Tc1/Tc17 cells. Conventionally it has been recognized that the key T lymphocytes that mediate these effects are CD4+ Th1 cells and CD8+ cells. However, other subsets of CD4+ (Th17, Th9, Tregs and Th22) and CD8+ (Tc1 and Tc17) are beginning to be elucidated.(Citation44) Recently, it has been recognized that the innate immune system may also be intricately involved by inducing innate effector mechanisms and signaling pathways providing the needed early inflammatory immune response for the activation of acquired immunity.(Citation45)
It is of some interest that while the majority of LMW allergens are associated primarily with skin sensitization and ACD, other allergens preferentially cause allergic sensitization of the respiratory tract resulting in asthma and rhinitis. It appears that the ability of LMW chemicals to elicit different forms of allergic disease is a function of the type and quality of immune response that they preferentially induce. Thus, whereas contact allergens favor the development of primarily Th1-type responses, chemical respiratory allergens are associated instead with preferential Th2-type immune responses. These Th2 cells are believed to play a pivotal role in the development of respiratory sensitization. Such cells also promote the induction of immunoglobulin E (IgE) antibody responses that have been implicated in various allergic diseases such as hay fever and food allergy. Although IgE antibodies are found in patients with chemical respiratory allergy, this is not always the case and there remains some uncertainty about the role played by IgE antibodies in some occupational asthmas. Innate immunity is also thought to play key roles in the development of respiratory allergy and asthma, although little to no work has been conducted looking at the role in LMW allergic respiratory diseases. Specifically invariant natural killer T cells and innate lymphoid cells (ILCs) have been implicated in asthma and allergic disease.(Citation46,Citation47) ILCs have been shown to produce a variety of cytokines associated with Th2 and other CD4+ cellular subsets as well as interact with other innate and adaptive immune cells involved in asthma. MicroRNAs (small, non-coding regulatory RNA) have also recently been suggested to play a role in asthma pathogenesis.(Citation48)
CHALLENGES INVOLVED IN THE DEVELOPMENT OF OCCUPATIONAL EXPOSURE LIMITS FOR CHEMICAL ALLERGENS
The biology of immune-mediated responses presents significant challenges in applying the traditional OEL derivation process to chemicals. The primary challenges that make the derivation of OELs for LMW allergens difficult include:
selection of an appropriate immune-mediated response to serve as the basis of the OEL (e.g., sensitization versus elicitation);
characterization of the concentration-response relationship (e.g., absence of human effects and exposure data and validated animal test protocols for sensitization of the respiratory tract);
effects of temporal exposure patterns on response (e.g., complex or unknown relationships between cumulative or peak exposures and sensitization);
level of sensitization and severity of elicited response (e.g., severity response is variable based on individual susceptibility and degree of sensitization);
role of exposure route in chemical allergies (e.g., relationship between dermal contact and sensitization of the respiratory tract is poorly understood); and
characterizing workplace exposures in a biologically meaningful way.
Selection of the Appropriate Immune-Mediated Response
A major consideration during the development of OELs is the identification of the appropriate health endpoint as the basis of the risk assessment. For LMW allergens, the critical question focuses on whether the OEL should protect from induction of sensitization in naïve individuals or from elicitation of a hypersensitivity response in persons who are already sensitized. OELs based on preventing sensitization are expected to protect naïve individuals from becoming sensitized to the chemical allergen. For sensitized individuals, elicitation of clinical symptoms may occur at or below an OEL derived to prevent induction of sensitization in naïve individuals. For example, elicitation of an asthmatic response has been reported in some sensitized workers following toluene diisocyanate (TDI) inhalation challenge at the ACGIH threshold limit value Time Weighted Average (TLV-TWA) of 5 parts per billion (ppb).(Citation49) Similarly, O’Brien et al. reported challenge elicitation of TDI-induced asthma in a subgroup of highly sensitized workers at ≤ 1 ppb.(Citation50) Based on these occupational studies, it is generally accepted that both early diagnosis and concurrent removal from exposure result in a better outcome for the sensitized worker. Vandenplas concluded that reduction of exposure may lead to improvement or resolution of symptoms in some workers;(Citation51) however, continued exposures to respiratory chemical allergens usually results in worsening of symptoms and poorer prognosis.(Citation52) These observations indicate that predicting a safe exposure level for sensitized individuals is difficult due to high variability in individual susceptibility. For this reason, prevention of sensitization in naïve individuals, rather than elicitation in already sensitized persons, appears to be a more suitable and health protective health endpoint to serve as the basis of an OEL.
Characterization of the Concentration-Response Relationship for Sensitization
A critical component of the risk assessment process is the establishment of the concentration-response relationship that describes the anticipated magnitude of response (i.e., adverse health outcome) associated with a particular amount of exposure. This relationship is used to identify the point of departure (i.e., extrapolation starting point) for deriving the OEL.(Citation33) Unlike non-allergenic chemicals which generally exhibit a no adverse effect level, individual susceptibility to sensitizers makes deriving such a threshold much more difficult. Hence, full characterization of the concentration-response relationship for sensitization is optimal for setting OELs for LMW allergens.
Most LMW allergens do not have sufficient human effects or exposure data to establish a concentration-response relationship for sensitization and thereby necessitate the use of alternative data sources. Unfortunately, no animal test protocols are available that have been validated (i.e., demonstrated to be consistent and reliable) and can identify with a high degree of specificity that will distinguish chemical allergens that preferentially produce respiratory allergic disease vs. ACD, or accurately predict its potency to produce respiratory sensitization.(4,24,53-56) The absence of well-accepted animal models for such an important endpoint reflects several considerations including the complexity of the immune system, species differences in respiratory tract and immune system physiology and anatomy and experimental challenges involved in conducting inhalation exposure experiments compared to skin application studies.(Citation24,Citation57,Citation58)
Another option for characterizing the concentration-response relationships for respiratory chemical allergens could be the use of biomarkers of immune sensitization or recognition such as specific IgE antibodies. Detection of specific antibodies (e.g., IgE or IgG) is commonly employed for the assessment of HMW and some LMW allergens and screening of exposed workers.(Citation30) Although scientific consensus on the use of sensitization biomarkers has not been established for LMW allergens, the use of such biomarkers in constructing a concentration-response relationship holds promise if key conditions are addressed. More precisely, this approach would require: (1) the detection of circulating antibodies (i.e., IgE) specific for the allergen of interest; (2) the detection the specific antibodies prior to the development of clinical disease; and (3) the quantification of the specific antibodies at levels at the sensitization threshold.
Establishing a concentration-response relationship for LMW chemicals directly for respiratory allergies is difficult because of the absence of inhalation studies and lack of validate animal models. Thus, risk assessment decisions are frequently based on the sensitization potential of chemicals as determined via dermal screening and animal test protocols. Animal models to identify contact allergens have been developed and validated. Modification of the standard murine local lymph node assay has been reported where specific cell surface markers and cytokine profiling are used to segregate Th1 and Th2 skewing chemical allergens.(Citation59) These tests vary in design, but typically involve the application of the test agent to the skin of a test animal (or human if clinical patch test is being done). presents descriptions of common human and animal dermal sensitization assays, which are validated and capable of confirming sensitization and potentially predicting potency. The findings of these assays are commonly reported in OEL documentation. For this reason, it is crucial for occupational hygiene professionals to develop a basic understanding of the assays to ensure the correct interpretation of the test results and their application in protecting workers from chemical allergens.
Effects of Temporal Exposure Patterns on Response
The relationship between the temporal patterns of exposure and the resulting effective dose associated with the induction of allergic sensitization is an important consideration in the development of OELs. The effective dose, if identifiable, could serve as the point of departure on which an OEL can be based or aid in the construction of the concentration-response relationship. Determining the effective dose associated with allergic sensitization is difficult due to the large variability in temporal exposure patterns. For example, workplace exposures can generically be characterized as repeated (i.e., cumulative) exposures or short-term, peak (i.e., acute) exposures. Both temporal patterns may contribute to the development of respiratory allergic diseases, but there is a lack of information on whether one temporal exposure pattern is of greater relevance to the onset of allergic sensitization and diseases.(Citation52)
Cumulative dose is the amount of chemical in the body or target organ system, integrated over repeated exposure events. Available evidence indicates that cumulative dose is an important predictor of response. Several diisocyanate studies where exposure was estimated as cumulative exposure rates have reported associations between exposure and hypersensitivity disease.(Citation60, Citation61) The role of cumulative or repeated exposures may relate to accumulation of the dose and biological response (e.g., build-up of antibodies). In addition, the dose of TDI can be increased by increasing exposure time, with dose × time–response relationships.(Citation62) Malo et al. (1999) studied the pattern of respiratory response (decline in forced expiratory volume in one second (FEV1)) following inhalation challenges to diisocyanates. Several dose-response patterns were observed, with the most common being a linear drop in FEV1 with cumulative dose (exposure challenges from 1–2 hr per day over 3+ days), although a quadratic response relationship were observed in several subjects.(Citation62) The authors speculated that the response patterns observed may be due to an accumulation of isocyanate haptenated protein in the body over the test period and changes in immune response components. In contrast, insufficient data are available to determine the role of acute exposures on the induction of respiratory sensitization.
For most chemical respiratory allergens, the profile, biology, and impact of temporal exposure patterns is poorly understood.(Citation24,Citation52) These uncertainties make it difficult to select the best time duration to serve as the basis of an OEL. More precisely, these limitations complicate the evaluation of the appropriate short-term exposure limit (STEL) for an LMW allergen when compared to an 8-hr TWA-OEL. The STEL is a 15-min TWA exposure that should not be exceeded within a single 8-hr day. Banks et al. demonstrated that a 15-min exposure at the 8-hr TWA-OEL (at that time the OEL was 20 ppb) to TDI was sufficient to elicit an asthmatic attack in a sensitized worker.(Citation63) What remains unknown is the role of such exposures on induction of sensitization in naïve individuals. In comparison, available data support a potential relationship between cumulative dose and immune response. Cumulative dose not only would account for repeated inhalation exposures, but also encounters with short-term, peak exposures to LMW allergens. Until the sufficient data are available to characterize the temporal exposure patterns and the associated biological responses, it appears that OELs based on cumulative dose associated with repeated inhalation exposures may be more achievable for protecting workers from the induction of allergic sensitization than the STEL.
Level of Sensitization and Severity of Elicited Response
The immune-mediated processes that result in naïve individuals developing sensitization do not stop once elicitation of an allergic disease has occurred. Subsequent exposures can result in increased sensitization to the allergens and elicitation of a more severe immune response. The exposure required to elicit a hypersensitivity response in a sensitized individual (threshold) will depend on the sensitization status. Theoretically, the threshold to induce or elicit the hypersensitivity response is related to the amount of haptenated protein, but this is complicated by the (dose × time) relationship as haptenated proteins have varying elimination rates from the body. Several diisocyanates studies where exposure was extrapolated as cumulative exposure rates have reported associations between exposure and hypersensitivity disease.(Citation61) In addition, the immunological processes that produce sensitization do not stop once a clinical response is elicited by subsequent exposure. While subsequent allergen exposure can boost immune sensitization, the sensitization (and inversely, the elicitation) dose-response curve can be bimodal. The exposure dose required to elicit a hypersensitivity response in a sensitized individual (threshold) will depend on the sensitization status. Thus, a high concentration exposure would be required to elicit a response in a minimally sensitized worker (or one who has become tolerant), and inversely a low concentration exposure may elicit a hypersensitivity response in a highly sensitized worker. This phenomenon is consistent with the finding from studies of exposure to diisocyanates and organic acid anhydrides.(Citation64,Citation65)
Role of Exposure Route in Chemical Allergies
In occupational settings, inhalation and dermal contact are the primary routes of exposure. LMW allergens have been demonstrated to produce allergic sensitization and diseases via both routes.(66–69) However, once an individual or animal is sensitized, elicitation of an allergic response to subsequent chemical exposure may occur independent of the sensitization exposure route.(54,70–73) Skin exposures to LMW allergens, such as isocyanates and beryllium, may result in, or exacerbate, respiratory hypersensitivity diseases with subsequent airways exposure.(Citation4,Citation68) This is contradictory to the historic perception that respiratory sensitization is achieved primarily, if not exclusively, via inhalation exposures to the chemical allergen.(Citation4) In contrast, available data illustrate that it is the immune system that is sensitized and not just the respiratory tract or skin (although allergen specific T-cells will preferentially home back from the lymph node to the site of exposure). For this reason, the contribution of both exposure routes resulting in allergic sensitization to the onset of hypersensitivity reactions, such as asthma or ACD, should not be discounted during the development of OELs or assessment of workplace exposures to allergens.
Most OELs for chemicals that are not allergens are intended to characterize airborne exposure levels that would protect from adverse health effects (i.e., cancer, irritation, or development toxicity) from the inhalation route. These OELs for general toxicity endpoints do not typically rely on dermal toxicity studies as their basis. In contrast, OELs for chemical allergens may need to reflect the combination of inhalation and dermal data. This reflects the absence of quantitative exposure-response data from inhalation studies and contribution of total systemic exposures to the allergic response. While there are methods available to quantitate inhalation exposure to many chemical allergens (especially chemically stable allergens such as beryllium), the current practice of occupational hygiene is ill equipped to quantitate most dermal or aggregate exposures. Until the science of dermal exposure assessment matures, the focus of OELs for chemical allergens will continue to be placed on inhalation limits with efforts to support qualitative risk management practices for dermal exposures.
Exposure Assessment Challenges for LMW Allergens
An important area not adequately addressed in the current science surrounds accurate characterization of complex exposures to LMW allergens in the work environment. Some LMW allergens are inherently reactive and may occur in multiple chemical states, such as an oxidized byproduct that may be more potent as an allergen.(Citation74) Capturing and stabilizing both the parent and all byproducts adds substantial analytical challenges. Another exposure assessment challenge reflects the difficulties in characterizing chemical mixtures of allergens. For example, many products contain mixtures of diisocyanates that may have varying allergic potencies, which has led to proposal of establishing both analytical methods and OELs for total diisocyanates.(Citation75) Whether real-time chemical specific instruments can be developed to quantify mixed allergen exposures in workplace atmospheres is an open area of research. An additional complicating factor is exposures via multiple routes. As previously discussed, allergic sensitization and diseases are systemic in nature and the proportional contributions of the individual exposure routes are usually unknown. Techniques to measure dermal exposures for LMW allergens, in many cases, do not exist. Further, the condition of the skin barrier at the time of exposure is often neglected despite the importance of the stratum corneum barrier in dermal penetration.
Collectively, these exposure assessment challenges fundamentally inhibit the derivation and use of OELs for chemical allergens in two primary ways. First, exposure measurements in existing occupational epidemiological studies may be inaccurate and prevent their use as the basis of an OEL. Second, the workplace environment is dynamic resulting in field level exposure measurements that are subject to the same challenges when used for occupational risk assessment (i.e., comparison of the exposure to the OEL). Improvements in exposure science may facilitate the collection of reliable measurements that accurately describe the workplace conditions.(Citation76)
Techniques for Developing OELs for Chemical Allergens
Despite the challenges in setting OELs for respiratory chemical allergens a variety of approaches have been used or are being developed. Most organizations that set OELs rely primarily on human effects and exposure data. However, research into in vivo toxicology methods using laboratory animals shows promise.(58, 77-79) Alternative approaches that support risk assessments include the use of predictive in vitro and in silico methods as tools for identifying potential toxicological endpoints for OEL assignment. The following sections highlight each of these techniques in greater detail.
OELs Based on Human Effects and Exposure Data
OELs have been established for a small number of chemical allergens (see ). Despite their chemical diversity, these chemicals share the feature of having sufficient human effects and exposure databases that serves as the basis of the OELs. The unique features of the immunological concentration-response behavior of allergens as noted above supports the emphasis on reliable human effects data. This is reflected in the basis for OELs established for known respiratory sensitizers, all of which relied directly on concentration-response data from worker populations. Data of sufficient quality are often not available, which hampers the OEL development process and has led to significant efforts to develop validated animal models for sensitization of the respiratory tract.
Other sources of human data used to confirm allergic sensitization in individuals exhibiting symptoms with a suspected etiology are diagnostic tests. These tests are designed to assess responses in sensitized individuals and are not intended to describe a concentration-response. The results of such tests are useful for hazard characterization purposes, but are not capable of serving as the basis of an OEL.
OELs Based on Data from Animal Test Protocols
Animal data are commonly employed to derive OELs based on non-immune-mediated health endpoints (e.g., cancers, systematic toxicity and irritation) when human effect and exposure data are unavailable.(Citation33) Use of animal toxicology data represents an alternative approach for the derivation for OELs for chemical allergens. Several animal test protocols have been proposed for characterizing and quantifying immune-mediated respiratory diseases, in addition to potentially allowing for construction of a concentration-response relationship for respiratory sensitization.(4,58,66,78,80–82) The current suite of experimental study protocols rely on different animal species (i.e., guinea pig, mouse, rat), exposure regimens (i.e., route, dose and timing) and health endpoints of interest (i.e., airway response, airway histopathology, biomarkers of exposures.(Citation4,Citation58,Citation77,Citation78) These techniques have not been validated and their ability to assist in setting OELs for chemical allergens has not been widely accepted.
Currently, efforts are underway to refine and validate in vivo respiratory sensitization assays including the respiratory local lymph node assay (LLNA) and other protocols that include direct measures of respiratory response in rodents.(53–56,78) Several factors influence generation of pulmonary exposures for these tests, including chemical solubility and stability in the vehicle, vehicle selection, and variability in lung deposition between species and cross route exposure (i.e., dermal exposure). As such, requirements for sophisticated equipment and associated expenses have increased the difficultly of these types of tests.(Citation58) Direct measures of sensitization that may serve as potential points of departure in this model may include production of hapten-specific IgE, cell proliferation in pulmonary-associated lymph nodes, production of specific cytokines, mRNA, miRNA, and proteomic profile. Despite the promise of this approach, the utility of these alternative models is still questionable. Additionally, few chemical allergens have been examined and the usefulness of data generated from these animal test protocols for OEL development process is unclear. Among the various experimental methods under development, data generated by the Brown Norway rat protocol has been used to derive an OEL for TDI based on the elicitation of allergic respiratory response in sensitized animals.(Citation83) A potential limitation of this model is the inability to distinguish between immune mediated and irritant inflammatory and airway responses from the responses. Despite the use of this model to develop an OEL, it is unclear if this can be widely applied to numerous LMW respiratory allergens. Another potential shortcoming is the use of elicitation of an allergic respiratory response as the basis of the OEL instead of respiratory sensitization. As previously discussed, it would ideal if OELs for LMW allergens were based on a concentration-response relationship for sensitization rather than elicitation.
provides brief descriptions of well-validated animal testing protocols capable of assessing the potential of LMW allergens to cause sensitization following dermal application. Of these protocols, the LLNA may be the most widely applied for new chemical hazard characterization. This animal test protocol estimates a concentration of the test chemical that yields a 3-fold increase in lymphocyte proliferation in treated mice (i.e., the EC3) when compared to a control substance.(Citation84) The EC3 value has been suggested as a potential point of departure for quantitative risk assessments when ACD is the critical health endpoint.(Citation85) For select strong respiratory sensitizers,(Citation55, Citation56, Citation86, Citation87) dermal LLNA data may serve as a potential source of information considered during the derivation of an OEL developed via route-to-route extrapolation. To conduct route-to-route extrapolation, several core assumptions must be made that result in uncertainties associated with exposure pathway differences and potency. These assumptions are as follows.
The potential for a chemical to cause sensitization is independent of exposure route. This assumption attempts to account for the biological mechanistic differences between the induction of sensitization via the skin and respiratory routes.
The potency of the chemical is dependent on the internal concentration of the chemical regardless of route of administration.
The absorbed dose is assumed to be 100% of the applied dose used during the LLNA study; this allows for the estimation of a respiratory dose equivalent.
These uncertainties have precluded the direct derivation of an OEL from dermal LLNA data. Additional understanding of route specific mechanisms of allergic sensitization and diseases would overcome uncertainties and allow for the use of dermal sensitization data as the quantitative basis of OELs.
Table 2 Overview of Animal Test Protocols Designed to Determine Skin Sensitization
Table 3 Qualitative Hazard Notations Designations for Chemical Allergens
Table 4 Risk Management Guidance for Chemical Allergens
Additional Approaches and Impacts on OEL Derivation
Incorporation of data based on chemical and physical similarities to known allergens (i.e., structure-activity relationship approaches) may also provide a means to derive an OEL. The final approach relies on relative potency factors (RPFs), which can be defined as ratios of the toxic potency of a selected chemical relative to an index chemical that exhibits the same toxic mechanism.(Citation88) A method has been previously applied to characterize the comparative toxicity of specific categories of chemicals, including organophosphates and polycyclic aromatic hydrocarbons, in human health risk assessments.(Citation89,Citation90) Using the RPF approach for chemical allergens that produce their immune effects through similar mechanisms and especially if they are of the same chemical class may allow for the establishment of scientifically-defensible OELs for chemicals that independently do not have sufficient data. For example, many diisocyanates have been identified as chemical allergens with varying sensitization potency;(Citation4) however, availability of data on the individual diisocyanates varies greatly. Application of the RPF approach may allow for the development of OELs for diisocyanates with limited data based on the datasets for those members of the chemical family that are well studied.
The quantitative risk assessments and the development of OELs using the RPF approach may be enhanced through the inclusion of in vitro and in silico information sources that can provide supplemental information in the absence of human effect and exposure data on each chemical.(9, 91–95) Efforts to validate these models have been the subject of significant review.(Citation94,Citation96) These techniques have not yet been adopted as the basis for an OEL on their own, but they have been used for qualitative hazard characterization, including assignment of hazard notations or as one input into a hazard banding process.(32,97–100)
Table 5 Checklist Summary of Key Considerations During Assessment of Health Risks Associated with Chemical Allergens
DISCUSSION
Many OELs for non-allergen chemicals are based on human health effect and exposure data.(Citation101) Unfortunately, such data are not available for most chemical allergens. This absence of human data coupled with a lack of established and validated animal models capable of characterizing the concentration-response relationships for respiratory sensitization makes it difficult to develop OELs based on allergic effects as the critical endpoint. Instead, OELs for many chemicals with some potential for sensitization are derived from other non-immune endpoints, such as respiratory irritation or systemic toxicity. The ability of these OELs to be protective against allergic sensitization and diseases is unknown leaving workers potentially vulnerable to life-threatening immune-mediated effects. Until the critical challenges in setting OELs for chemical allergens are addressed, greater emphasis must be placed on the implementation of comprehensive risk management strategies designed to specifically prevent or limit workplace exposures to chemical allergens via the dermal and inhalation pathways. Failure to do so could result in sensitized workers that, upon repeat exposure to an allergen, may experience a continuum of immune-mediated responses ranging from mild ACD to life-threatening cases of asthma.
In the absence of established quantitative risk assessment techniques capable of developing OELs for chemical allergens, numerous qualitative approaches have been applied to provide alternative exposure recommendations that provide guidance on the health hazards of allergens and assist in the development of a well-informed risk management strategy. Two common approaches are (1) qualitative hazard notations and (2) hazard banding (occupational exposure banding).
Qualitative hazard notations or designations function as a communication tool used for risk management purposes. They are intended to identify specific health concerns, such as sensitization rather than characterize airborne concentrations of a chemical capable of resulting in allergic responses. provides an overview of hazard notations established for chemical allergens by numerous OEL-setting organizations. The criteria, level of documentation and data requirements used to assign these notations vary widely between organizations. For example, NIOSH outlines a systematic approach based on weight of evidence for the assignment of multiple hazard notations that target specific dermal health hazards, including sensitization (SEN).(Citation98) Each notation is accompanied by a robust summary of the critical data and rationale used to assign a specific notation. However, the NIOSH notations are limited to dermal hazards and do not address respiratory sensitization.(Citation98,Citation99) In comparison, the Globally Harmonized System for the Classification and Labeling of Chemicals (GHS) assigns notations for several endpoints, including respiratory and dermal sensitization.(Citation100) Governmental agencies, private industry and other groups are using this approach both domestically and internationally to characterize the health and environmental hazards of chemicals. Depending on the organization that generates the GHS notations, the documentation, rationale and data used to assign the GHS notations may not be available to the public. The second qualitative approach is hazard banding, which attempts to systematically categorize chemicals in “bands” or categories using predefined criteria based on selected physicochemical and toxicological properties. Criteria typically cover a variety of toxicological endpoints ranging from acute toxicity to systematic toxicity from repeated exposures to special endpoints of concern (i.e., irritation, sensitization, developmental toxicity and carcinogenicity). Many banding systems link predefined criteria for these health endpoints with hazard labeling and classification schemes, such as GHS. The banding approach has been successfully applied within the pharmaceutical industry for decades to limit workers’ exposures to potent chemicals (not all of which are sensitizers) and is increasingly being applied across other industries.(Citation32,Citation97) The hazard bands identified are commonly used to guide the selection of exposure control stringency.(Citation102, Citation103) Application of hazard banding provides a tool to identify potential respiratory and dermal sensitizers, in addition to assisting in making informed risk management decisions.
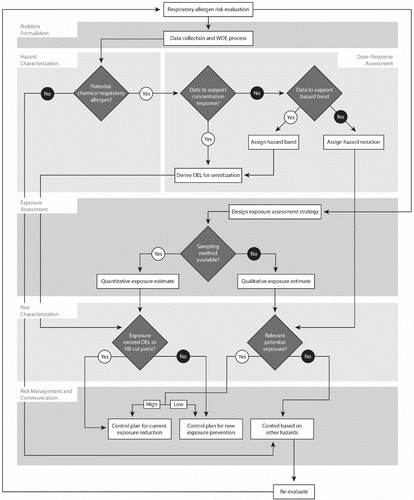
A comprehensive risk management strategy intended to protect workers from allergens incorporates several core elements. Primary prevention involves implementing strategies before induction of sensitization occurs. Interventions in preventing sensitization include avoidance of sensitizing agents, modifying the agent (i.e., encapsulation of agent), reduced exposure by containment or personal protective equipment and education.(Citation21) Secondary prevention involves early detection and limiting progression of disease, such as limiting the clinical manifestations in a sensitized individual. Secondary preventive interventions include: (1) workplace (air and biological) monitoring;(Citation104) (2) medical surveillance;(30, 105-107) and (3) application of the hierarchy of controls.(Citation104, Citation108, Citation109) These important monitoring and surveillance strategies do not need to be limited to secondary prevention. provides generalized recommendations for controls to prevent or limit workplace exposures to allergens. and are included to assist users in addressing critical issues and developing risk management strategies aimed at controlling workplace exposures to chemical allergens.
CONCLUSIONS
Chemical allergens represent a significant health hazards for workers. A limited number of OELs have been established for respiratory LMW allergens based almost exclusively on epidemiology and human exposure data that allow for the extrapolation of concentration-response relationships. For most respiratory allergens, such data sets are not available to construct the concentration-response relationship. The absence of human effect and exposure data coupled with other critical limitations, such as the absence of animal test models capable of predicting respiratory sensitization potential, currently inhibits the ability to develop OELs for most LMW allergens. Approaches, such as the in vivo assays and biomarkers of sensitization, hold great promise in assisting in the development of concentration-response relationships between exposures to LMW allergens and onset of immune-mediated respiratory diseases and subsequently the development of OELs. Until these approaches are appropriately vetted and refined, the health risk of allergens in the workplace must be addressed via the combination of appropriate exposure control technologies and practices and qualitative hazard characterization approaches, such as hazard banding and hazard notations, with well-informed risk management efforts.
ACKNOWLEDGMENTS
The authors would like to express their sincere appreciation to the following individuals for their technical reviews and input on this manuscript: Carrie Redlich, M.D., M.P.H. (Yale University), Paul Schulte, Ph.D. (NIOSH), Maryjane Selgrade Ph.D. (ICF International), and Chris Whittaker, Ph.D. (NIOSH). The findings and conclusions in this article are those of the author(s) and do not necessarily represent the views of the National Institute for Occupational Safety and Health.
REFERENCES
- Kimber, I., D.A. Basketter, and R.J. Dearman: Chemical allergens–what are the issues? Toxicology 268(3):139–142 (2010).
- Kimber, I., D.A. Basketter, J.P. Thyssen, R.J. Dearman, and J.P. McFadden: Chemical allergy in humans: fresh perspectives. J. Immunotoxicol. 11(3):203–204 (2014).
- Janeway, C.A. Jr., and P. Travers: Allergy and hypersensitivity. In Immunobiology: The Immune System in Health and Disease. New York: Garland Publishing Inc., 1997. pp. 12:11–11:25.
- Kimber, I., D.A. Basketter, G.F. Gerberick, C.A. Ryan, and R.J. Dearman: Chemical allergy: translating biology into hazard characterization. Toxicol. Sci. 120 Suppl 1:S238–268 (2011).
- Enk, A.H.: Allergic contact dermatitis: understanding the immune response and potential for targeted therapy using cytokines. Mol. Med. Today 3(10):423–428 (1997).
- Gerberick, G.F., C.A. Ryan, P.S. Kern et al.: Compilation of historical local lymph node data for evaluation of skin sensitization alternative methods. Dermatitis 16(4):157–202 (2005).
- Kern, P.S., G.F. Gerberick, C.A. Ryan, I. Kimber, A. Aptula, and D.A. Basketter: Local lymph node data for the evaluation of skin sensitization alternatives: a second compilation. Dermatitis 21(1):8–32 (2010).
- Santos, R., and A. Goossens: An update on airborne contact dermatitis: 2001-2006. Contact Dermatitis 57(6):353–360 (2007).
- Lalko, J.F., I. Kimber, R.J. Dearman, G.F. Gerberick, K. Sarlo, and A.M. Api: Chemical reactivity measurements: potential for characterization of respiratory chemical allergens. Toxicol. In Vitro 25(2):433–445 (2011).
- Quirce, S., and J.A. Bernstein: Old and new causes of occupational asthma. Immunol. Allergy Clin. North Am. 31(4):677–698 (2011).
- Quirce, S., and J. Sastre: New causes of occupational asthma. Curr. Opin. Allergy Clin. Immunol. 11(2):80–85 (2011).
- Zacharisen, M.C., and J.N. Fink: Hypersensitivity pneumonitis and related conditions in the work environment. Immunol. Allergy Clin. North Am. 31(4):769–786 (2011).
- Maier, A., M.J. Vincent, B. Gadagbui et al.: Integrating asthma hazard characterization methods for consumer products. Regul. Toxicol. Pharmacol. 70(1):37–45 (2014).
- Mapp, C.E., P. Boschetto, P. Maestrelli, and L.M. Fabbri: Occupational asthma. Am. J. Respir. Crit. Care Med. 172(3):280–305 (2005).
- Casas, L., and B. Nemery: Irritants and asthma. Eur. Respir. J. 44(3):562–564 (2014).
- Dumas, O., E. Laurent, J. Bousquet et al.: Occupational irritants and asthma: an Estonian cross-sectional study of 34 000 adults. Eur. Respir. J. 44(3):647–656 (2014).
- Vizcaya, D., M.C. Mirabelli, J.-M. Antó, et al.: A workforce-based study of occupational exposures and asthma symptoms in cleaning workers. Occup. Environ. Med. 68(12):914–919 (2011).
- Baur, X., P. Bakehe, and H. Vellguth: Bronchial asthma and COPD due to irritants in the workplace-an evidence-based approach. J. Occup. Med. Toxicol. 7(1):19 (2012).
- Kogevinas, M., J.P. Zock, D. Jarvis et al.: Exposure to substances in the workplace and new-onset asthma: An international prospective population-based study (ECRHS-II). Lancet 370(9584):336–341 (2007).
- Smith, A.M.: The epidemiology of work-related asthma. Immunol. Allergy Clin. North Am. 31(4): 663–675 (2011).
- Tarlo, S.M., and C. Lemiere: Occupational asthma. N. Engl. J. Med. 370(7):640–649 (2014).
- Torén, K., and P.D. Blanc: Asthma caused by occupational exposures is common–a systematic analysis of estimates of the population-attributable fraction. BMC Pulm. Med. 9(1):7 (2009).
- Mazurek, J.M., G.E. Knoeller, J.E. Moorman, and E. Storey: Occupational Asthma Incidence: Findings from the Behavioral Risk Factor Surveillance System Asthma Call-Back Survey—United States, 2006–2009. Journal of Asthma 50(4): 390–394 (2013).
- Arts, J.H.E., C. Mommers, and C. de Heer: Dose-response relationships and threshold levels in skin and respiratory allergy. Crit. Rev. Toxicol. 36(3):219–251 (2006).
- Thierse, H.J., K. Gamerdinger, C. Junkes, N. Guerreiro, and H.U. Weltzien: T cell receptor (TCR) interaction with haptens: metal ions as non-classical haptens. Toxicology 209(2):101–107 (2005).
- Wang, Y., and S. Dai: Structural basis of metal hypersensitivity. Immunol. Res. 55(1–3):83–90 (2013).
- Green, B.J., and D.H. Beezhold: Industrial fungal enzymes: an occupational allergen perspective. J. Allergy (Cairo) 2011:1–11 (2011).
- Heederik, D.: Are we closer to developing threshold limit values for allergens in the workplace? Curr. Opin. Allergy Clin. Immunol. 1(2):185–189 (2001).
- Baur, X.: I are we closer to developing threshold limit values for allergens in the workplace? Ann. Allergy Asthma Immunol. 90(5 Suppl 2):11–18 (2003).
- Rijnkels, J.M., T. Smid, E.C. Van den Aker et al.: Prevention of work-related airway allergies; summary of the advice from the Health Council of the Netherlands. Allergy 63(12):1593–1596 (2008).
- Dankovic, D.A., B.D. Naumann, A. Maier, M.L. Dourson, and L. Levy: The scientific basis of uncertainty factors used in setting occupational exposure limits. J. Occup. Environ. Hyg. 12(0):S55–S68 (2015).
- Deveau, M., C.-P. Chen, G. Johanson et al.: The global landscape of occupational exposure limits—Implementation of harmonization principles to guide limit selection. J. Occup. Environ. Hyg. 12(0):S127–S144 (2015).
- Wheeler, M.W., R. Park, A.J. Bailer, and C. Whittaker: Historical context and recent advances in exposure-response estimation for deriving occupational exposure limits. J. Occup. Environ. Hyg. 12(0):S7–S17 (2015).
- Lockey, J.E., C.A. Redlich, R. Streicher et al.: Isocyanates and human health: multistakeholder information needs and research priorities. J. Occup. Environ. Med. 57(1):44–51 (2015).
- Karlberg, A.T., M.A. Bergstrom, A. Borje, K. Luthman, and J.L. Nilsson: Allergic contact dermatitis—Formation, structural requirements, and reactivity of skin sensitizers. Chem. Res. Toxicol. 21(1):53–69 (2008).
- Maestrelli, P., P. Boschetto, L.M. Fabbri, and C.E. Mapp: Mechanisms of occupational asthma. J. Allergy Clin. Immunol. 123(3):531–544 (2009).
- De Vooght, V., V. Hox, B. Nemery, and J.A. Vanoirbeek: Mechanisms of occupational asthma caused by low-molecular-weight chemicals. In Occupational Asthma, T. Sigsgaard and D. Heederik (eds.). Basel, Switzerland: Birkhäuser Basel, 2010. pp. 141–162.
- Farahani, R., R. Sherkat, M.G. Hakemi, N. Eskandari, and R. Yazdani: Cytokines (interleukin-9, IL-17, IL-22, IL-25 and IL-33) and asthma. Adv. Biomed. Res. 3:127 (2014).
- Kimber, I., R.J. Dearman, D.A. Basketter, and D.R. Boverhof: Chemical respiratory allergy: reverse engineering an adverse outcome pathway. Toxicology 318:32–39 (2014).
- Holsapple, M.P., D. Jones, T.T. Kawabata et al.: Assessing the potential to induce respiratory hypersensitivity. Toxicol. Sci. 91(1):4–13 (2006).
- Kimber, I., and R.J. Dearman: What makes a chemical a respiratory sensitizer? Curr. Opin. Allergy Clin. Immunol. 5(2):119–124 (2005).
- Kimber, I., D.A. Basketter, G.F. Gerberick, and R.J. Dearman: Allergic contact dermatitis. Int. Immunopharmacol. 2(2-3):201–211 (2002).
- Bourrain, J.L.: Occupational contact urticaria. Clin. Rev. Allergy Immunol. 30(1):39–46 (2006).
- Dhingra, N., A. Shemer, J. Correa da Rosa et al.: Molecular profiling of contact dermatitis skin identifies allergen-dependent differences in immune response. J. Allergy Clin. Immunol. 134(2):362–372 (2014).
- Martin, S.F., P.R. Esser, F.C. Weber et al.: Mechanisms of chemical-induced innate immunity in allergic contact dermatitis. Allergy 66(9):1152–1163 (2011).
- DeKruyff, R.H., S. Yu, H.Y. Kim, and D.T. Umetsu: Innate immunity in the lung regulates the development of asthma. Immunol. Rev. 260(1):235–248 (2014).
- Yu, S., H.Y. Kim, Y.J. Chang, R.H. DeKruyff, and D.T. Umetsu: Innate lymphoid cells and asthma. J. Allergy Clin. Immunol. 133(4):943–950; quiz 951 (2014).
- Kai, W., X.U. Qian, and W.U. Qun: MicroRNAs and asthma regulation. Iran J. Allergy Asthma Immunol. 14(2):120–125 (2015).
- Banks, D.E., R.J. Rando, and H.W. Barkman, Jr.: Persistence of toluene diisocyanate-induced asthma despite negligible workplace exposures. Chest 97(1):121–125 (1990).
- O’Brien, I.M., M.G. Harries, P.S. Burge, and J. Pepys: Toluene di-isocyanate-induced asthma. I. Reactions to TDI, MDI, HDI and histamine. Clin. Allergy 9(1):1–6 (1979).
- Vandenplas, O.: Reduction of exposure in the management of occupational asthma. Curr. Opin. Allergy Clin. Immunol. 11(2):75–79 (2011).
- Kimber, I., R. Agius, D.A. Basketter et al.: Chemical respiratory allergy: opportunities for hazard identification and characterisation. The report and recommendations of ECVAM workshop 60. Altern. Lab. Anim. 35(2):243–265 (2007).
- Zhang, X.D., M.E. Andrew, A.F. Hubbs, and P.D. Siegel: Airway responses in Brown Norway rats following inhalation sensitization and challenge with trimellitic anhydride. Toxicol. Sci. 94(2):322–329 (2006).
- Zhang, X.D., J.S. Fedan, D.M. Lewis, and P.D. Siegel: Asthmalike biphasic airway responses in Brown Norway rats sensitized by dermal exposure to dry trimellitic anhydride powder. J. Allergy Clin. Immunol. 113(2):320–326 (2004).
- Zhang, X.D., A.F. Hubbs, and P.D. Siegel: Changes in asthma-like responses after extended removal from exposure to trimellitic anhydride in the Brown Norway rat model. Clin. Exp. Allergy 39(11):1746–1753 (2009).
- Pauluhn, J., and A. Poole: Brown Norway rat asthma model of diphenylmethane-4,4’-diisocyanate (MDI): determination of the elicitation threshold concentration of after inhalation sensitization. Toxicology 281(1–3):15–24 (2011).
- Pauluhn, J., R. Dearman, J. Doe, P. Hext, and T.D. Landry: Respiratory hypersensitivity to diphenylmethane-4,4’-diisocyanate in guinea pigs: comparison with trimellitic anhydride. Inhal. Toxicol. 11(3):187–214 (1999).
- Arts, J.H., and C.F. Kuper: Animal models to test respiratory allergy of low molecular weight chemicals: a guidance. Methods 41(1):61–71 (2007).
- Manetz, T.S., and B.J. Meade: Development of a flow cytometry assay for the identification and differentiation of chemicals with the potential to elicit irritation, IgE-mediated, or T cell-mediated hypersensitivity responses. Toxicol. Sci. 48(2):206–217 (1999).
- Sastre, J., M. Fernandez-Nieto, A. Novalbos, M. De Las Heras, J. Cuesta, and S. Quirce: Need for monitoring nonspecific bronchial hyperresponsiveness before and after isocyanate inhalation challenge. Chest 123(4):1276–1279 (2003).
- Pronk, A., L. Preller, M. Raulf-Heimsoth et al.: Respiratory symptoms, sensitization, and exposure response relationships in spray painters exposed to isocyanates. Am. J. Respir. Crit. Care Med. 176(11):1090–1097 (2007).
- Malo, J.L., H. Ghezzo, and R. Elie: Occupational asthma caused by isocyanates: patterns of asthmatic reactions to increasing day-to-day doses. Am. J. Respir. Crit. Care Med. 159(6):1879–1883 (1999).
- Banks, D.E., J. Sastre, B.T. Butcher et al.: Role of inhalation challenge testing in the diagnosis of isocyanate-induced asthma. Chest 95(2):414–423 (1989).
- Cassidy, L.D., D.M. Molenaar, J.A. Hathaway et al.: Trends in pulmonary function and prevalence of asthma in hexamethylene diisocyanate workers during a 19-year period. J. Occup. Environ. Med. 52(10):988–994 (2010).
- Butcher, B.T., J.E. Salvaggio, H. Weill, and M.M. Ziskind: Toluene diisocyanate (TDI) pulmonary disease: immunologic and inhalation challenge studies. J. Allergy Clin. Immunol. 58(1 PT 1):89–100 (1976).
- Kimber, I., J. Hilton, D.A. Basketter, and R.J. Dearman: Predictive testing for respiratory sensitization in the mouse. Toxicol. Lett. 86(2–3):193–198 (1996).
- Bello, D., C.A. Herrick, T.J. Smith et al.: Skin exposure to isocyanates: reasons for concern. Environ. Health Perspect. 115(3):328–335 (2007).
- Redlich, C.A.: Skin exposure and asthma: is there a connection? Proc. Am. Thorac. Soc. 7(2):134–137 (2010).
- Redlich, C.A., and C.A. Herrick: Lung/skin connections in occupational lung disease. Curr. Opin. Allergy Clin. Immunol. 8(2):115–119 (2008).
- Petsonk, E.L., M.L. Wang, D.M. Lewis, P.D. Siegel, and B.J. Husberg: Asthma-like symptoms in wood product plant workers exposed to methylene diphenyl diisocyanate. Chest 118(4):1183–1193 (2000).
- Kimber, I., and R.J. Dearman: Chemical respiratory allergy: role of IgE antibody and relevance of route of exposure. Toxicology 181-182:311–315 (2002).
- Johnson, V.J., J.M. Matheson, and M.I. Luster: Animal models for diisocyanate asthma: answers for lingering questions. Curr. Opin. Allergy Clin. Immunol. 4(2):105–110 (2004).
- Vanoirbeek, J.A., M. Tarkowski, J.L. Ceuppens, E.K. Verbeken, B. Nemery, and P.H. Hoet: Respiratory response to toluene diisocyanate depends on prior frequency and concentration of dermal sensitization in mice. Toxicol. Sci. 80(2):310–321 (2004).
- Karlberg, A.T., K. Magnusson, and U. Nilsson: Air oxidation of d-limonene (the citrus solvent) creates potent allergens. Contact Dermatitis 26(5):332–340 (1992).
- Bello, D., S.R. Woskie, R.P. Streicher et al.: Polyisocyanates in occupational environments: a critical review of exposure limits and metrics. Am. J. Ind. Med. 46(5):480–491 (2004).
- National Research Council: Exposure Science in the 21st Century: A Vision and a Strategy. Washington, DC: The National Academies Press, 2012.
- Pauluhn, J., and U. Mohr: Experimental approaches to evaluate respiratory allergy in animal models. Exp. Toxicol. Pathol. 56(4–5):203–234 (2005).
- Arts, J.H., W.H. de Jong, J.J. van Triel et al.: The respiratory local lymph node assay as a tool to study respiratory sensitizers. Toxicol. Sci. 106(2):423–434 (2008).
- Kimber, I., D.A. Basketter, J.P. McFadden, and R.J. Dearman: Characterization of skin sensitizing chemicals: a lesson learnt from nickel allergy. J. Immunotoxicol. 8(1):1–2 (2011).
- Boverhof, D.R., B.B. Gollapudi, J.A. Hotchkiss, M. Osterloh-Quiroz, and M.R. Woolhiser: A draining lymph node assay (DLNA) for assessing the sensitizing potential of proteins. Toxicol. Lett. 193(2):144–151 (2010).
- Dearman, R.J., C.J. Betts, N. Humphreys et al.: Chemical allergy: considerations for the practical application of cytokine profiling. Toxicol. Sci. 71(2):137–145 (2003).
- Pauluhn, J.: Development of a respiratory sensitization/elicitation protocol of toluene diisocyanate (TDI) in Brown Norway rats to derive an elicitation-based occupational exposure level. Toxicology 319:10–22 (2014).
- Pauluhn, J.: Derivation of occupational exposure levels (OELs) of Low-toxicity isometric biopersistent particles: how can the kinetic lung overload paradigm be used for improved inhalation toxicity study design and OEL-derivation? Part Fibre Toxicol. 11(1):72 (2014).
- Anderson, S.E., P.D. Siegel, and B.J. Meade: The LLNA: a brief review of recent advances and limitations. J. Allergy (Cairo) 2011:1–10 (2011).
- Griem, P.: Uncertainty factors and risk assessment for skin sensitizers. In IPCS Harmonization Project Document No. 5: Skin Sensitization in Chemical Risk Assessment. Geneva: World Health Organization, 2008. pp. 46–53.
- Kuper, C.F., W.H. Heijne, M. Dansen et al.: Molecular characterization of trimellitic anhydride-induced respiratory allergy in Brown Norway rats. Toxicol. Pathol. 36(7):985–998 (2008).
- Kuper, C.F., R.H. Stierum, A. Boorsma et al.: The contact allergen dinitrochlorobenzene (DNCB) and respiratory allergy in the Th2-prone Brown Norway rat. Toxicology 246(2–3):213–221 (2008).
- Environmental Protection Agency: “Guidance on Cumulative Risk Assessment of Pesticide Chemicals that Have a Common Mechanism of Toxicity.” Available at http://www.epa.gov/pesticides/trac/science/cumulative_guidance.pdf.
- Environmental Protection Agency: “Organophosphorus Cumulative Risk Assessment: 2006 Update.” Available at http://www.epa.gov/pesticides/cumulative/2006-op/op_cra_main.pdf.
- Environmental Protection Agency: “Development of a Relative Potency Factor (RPF) Approach for Polycyclic Aromatic Hydrocarbon (PAH) Mixtures.” Available at http://cfpub.epa.gov/ncea/cfm/recordisplay.cfm?deid=194584.
- Isola, D., I. Kimber, K. Sarlo, J. Lalko, and I.G. Sipes: Chemical respiratory allergy and occupational asthma: what are the key areas of uncertainty? J. Appl. Toxicol. 28(3):249–253 (2008).
- Kimber, I., J.S. Pichowski, C.J. Betts, M. Cumberbatch, D.A. Basketter, and R.J. Dearman: Alternative approaches to the identification and characterization of chemical allergens. Toxicol. In Vitro 15(4–5):307–312 (2001).
- Seed, M.J., P. Cullinan, and R.M. Agius: Methods for the prediction of low-molecular-weight occupational respiratory sensitizers. Curr. Opin. Allergy Clin. Immunol. 8(2):103–109 (2008).
- Enoch, S.J., M.J. Seed, D.W. Roberts, M.T. Cronin, S.J. Stocks, and R.M. Agius: Development of mechanism-based structural alerts for respiratory sensitization hazard identification. Chem. Res. Toxicol. 25(11):2490–2498 (2012).
- Roggen, E.L.: In vitro approaches for detection of chemical sensitization. Basic Clin. Pharmacol. Toxicol. 115(1):32–40 (2014).
- Teubner, W., A. Mehling, P.X. Schuster et al.: Computer models versus reality: how well do in silico models currently predict the sensitization potential of a substance. Regul. Toxicol. Pharmacol. 67(3):468–485 (2013).
- Naumann, B.D., E.V. Sargent, B.S. Starkman, W.J. Fraser, G.T. Becker, and G.D. Kirk: Performance-based exposure control limits for pharmaceutical active ingredients. Am. Ind. Hyg. Assoc. J. 57(1):33–42 (1996).
- NIOSH: Current Intelligence Bulletin 61: A Strategy for Assigning New NIOSH Skin Notations. Cincinnati, OH: U.S. Department of Health and Human Services, Centers for Disease Control and Prevention, National Institute for Occupational Safety and Health, DHHS (NIOSH) Publication No. 09-147, 2009.
- Dotson, G.S., C.P. Chen, B. Gadagbui, A. Maier, H.W. Ahlers, and T.J. Lentz: The evolution of skin notations for occupational risk assessment: a new NIOSH strategy. Regul. Toxicol. Pharmacol. 61(1):53–62 (2011).
- UNECE (United Nations Economic Commission): “Globally harmonized system of classification and labelling of chemicals (GHS): 4th revised edition.” Available at http://www.unece.org/trans/danger/publi/ghs/ghs_rev04/04files_e.html.
- ACGIH (American Conference of Governmental Industrial Hygienists: Introduction to the Chemical Substances: Definitions and Notations. Cincinnati, OH: American Conference of Governmental Industrial Hygienists. CD-ROM, 2014.
- NIOSH: Qualitative Risk Characterization and Management of Occupational Hazards: Control Banding (CB). A Literature Review and Critical Analysis. Cincinnati, OH: U.S. Department of Health and Human Services, Centers for Disease Control and Prevention, National Institute for Occupational Safety and Health, DHHS (NIOSH) Publication No. 09-152, 2009.
- Zalk, D.M., and D.I. Nelson: History and evolution of control banding: A review. J. Occup. Environ. Hyg. 5(5):330–346 (2008).
- AIHA: The Occupational Environment: Its Evaluation, Control, and Management. Falls Church, VA: American Industrial Hygiene Association, 2011.
- Lacasse, Y., and Y. Cormier: Hypersensitivity pneumonitis. Orphanet. J. Rare Dis. 1:25 (2006).
- Ott, M.G.: Occupational asthma, lung function decrement, and toluene diisocyanate (TDI) exposure: a critical review of exposure-response relationships. Appl. Occup. Environ. Hyg. 17(12):891–901 (2002).
- Simons, F.E., L.R. Ardusso, V. Dimov et al.: World Allergy Organization Anaphylaxis Guidelines: 2013 update of the evidence base. Int. Arch. Allergy Immunol. 162(3):193–204 (2013).
- EPA: “Design for the environment program alternatives: assessment criteria for hazard evaluation.” Available at http://epa.gov/dfe/alternatives_assessment_criteria_for_hazard_eval.pdf.
- OSHA: “Safety and Health Management Systems eTool .” Available at https://www.osha.gov/SLTC/etools/safetyhealth/comp3.html.
- Basketter, D.A.: The human repeated insult patch test in the 21st century: a commentary. Cutan. Ocul. Toxicol. 28(2):49–53 (2009).
- Zhai, H., and H.I. Maibach: Dermatotoxicology. Boca Raton, FL: CRC Press LLC, 2004.
- Cal/OSHA: “Sensitizing substances: advisory meetings.” Available at http://www.dir.ca.gov/DOSH/DoshReg/5179Meetings.html.
- EC: “Regulation (EC) No 1272/2008 of the European Parliament and of The Council of 16 December 2008 on classification, labelling and packaging of substances and mixtures, amending and repealing Directives 67/548/ECC and 1999/45/EC, and amending Regulation (EC) No 1907/2006.” Available at http://eur-lex.europa.eu/LexUriServ/LexUriServ.do?uri = OJ:L:2008:353:0001:1355:EN:PDF.