ABSTRACT
Near-ultraviolet (UV-A: 315–400 nm), “black-light,” electric lamps were invented in 1935 and ultraviolet insect light traps (ILTs) were introduced for use in agriculture around that time. Today ILTs are used indoors in several industries and in food-service as well as in outdoor settings. With recent interest in photobiological lamp safety, safety standards are being developed to test for potentially hazardous ultraviolet emissions. A variety of UV “Black-light” ILTs were measured at a range of distances to assess potential exposures. Realistic time-weighted human exposures are shown to be well below current guidelines for human exposure to ultraviolet radiation. These UV-A exposures would be far less than the typical UV-A exposure in the outdoor environment. Proposals are made for realistic ultraviolet safety standards for ILT products.
KEYWORDS:
Introduction
UV insect light traps (ILTs) have existed for at least 75 years. The use of candles and other lamps have been used for centuries to trap insects at night. The first electric ILTs used Edison's electric light bulb over a washtub of water. White-light electric lamps were first used as ILTs about 100 years ago. Prof. W.B. Hermes, a parasitologist at the University of California, reported on his 26 years of experiments with different colored visible lamps to attract insect pests and introduced the first “bug-zapper” for outdoor use in 1934.Citation[1] Ultraviolet ILTs followed within a few years. However, ILTs for indoor use in food plants, pharmaceutical laboratories and similar industries were not introduced until the 1960s.Citation[2] Current ILTs generally employ near-ultraviolet (UV-A) “black-light” type BL fluorescent tubes as an insect attractant.Citation[2–5] Depending upon the type of the ILT, the insects are normally electrocuted on a high-voltage grid or trapped on a glue-board. Professional ILT units are employed primarily in the food, healthcare, and pharmaceutical industries and are typically wall mounted. There are also portable models that can be suspended from the ceiling, wall mounted or floor mounted. Based on preferred movements and response of flies and night-flying insects, a strategic layout of low wall-mounted ILTs has been accepted as the best positioning to catch both flies and night flying insects. However, ceiling-hung ILTs are useful when night-fliers are the primary concern.Citation[4] Proper placement of ILTs correspond to spaces where people are not continuously exposed, since the strategy is to attract insects away from food (or other products) and the individuals producing them. ILTs are also most effective positioned in fly pathways—also away from people—to trap insects before they reach the most critical areas in the facility.Citation[4] Furthermore, the U.S. Food Code prohibits locating ILTs above processing areas.Citation[7,8] Thus, we always found ILTs placed near entrances, in entrance vestibules, at bottlenecks (next to doorways), all along flyways between dumpsters and areas needing protection, away from routinely occupied areas, i.e., where only momentary human exposure would result, such as walking through a doorway. The object of this study was to determine if the UV radiation emitted from ILTs expose persons in the vicinity above current exposure guidelines for UV radiant energy.Citation[8]
Most ILTs employ UV-A (“black-light”) fluorescent tubular lamps with emission spectra having bandwidths at half-maximum within the spectral region 335 and 380 nm. There are two principal categories: those with black-light-bulbs with a peak emission at ∼366 nm and those with a peak wavelength near 350 nm–somewhat below the 366-nm emission line of the low-pressure mercury discharge that helps to excite the fluorescent phosphor.
To achieve the objective of this study, it was necessary to assess any reasonably foreseeable, worst-case exposures from ILTs and determine if the daily cumulative radiant exposure to UV exceeded limits for either the UV-A or trace quantities of shorter-wavelength, more hazardous, un-wanted UV-B (280-315 nm) “leakage” radiation.Citation[9,10] There have not been eye or skin injuries reported to arise from ILTs, but it was expected from the outset that the UV exposures would be less than outdoor environmental exposures. Although retinal hazards are not considered significant in the UV-A since only trace levels reach the retina, some check-tests were made.
Methods
ILT siting and measurements
A wide variety of ILT installations were studied to determine reasonably foreseeable human exposure conditions. Exposure distances to fixtures were measured for use in the analysis. Since there are dual exposure limits for ultraviolet radiation—both the total irradiance limit and the actinic, spectrally weighted [S(λ)] “effective irradiance” were measured.Citation[10,11]
Instrumentation
Initial field measurements of a variety of UV-A ILT lamp fixtures were made using a broadband radiometer, an International Light Model 1400A radiometer/ photometer (S.N. 1402). Two detectors were used: a Model SEL240 detector with input optic T2ACT3 calibrated to read directly in terms of the ACGIH/ICNIRP UV-Hazard S(λ)-weighted effective irradiance;Citation[10] and a Model SEL033 UV-A detector (with input optic and UVA filter) calibrated to measure near-ultraviolet (UV-A) radiation between approximately 315 and 400 nm. However, the first (UV safety) detector appeared to be responding too strongly to the UV-A and provided high readings. Therefore, an attempt was then made to use a small, portable spectroradiometer to check these earlier readings: a calibrated Ocean Optics Spectroradiometer (Model USB2000 with a 180°-cosine-corrected irradiance probe). Although this instrument permitted cross-comparisons with the more portable IL-1400A hand-held meter, it also appeared to be recording noise in the UV-B range. These initial measurements demonstrated that we could only employ hand-held meters for field surveys if readings were corrected for measurement errors resulting from stray light and noise.Citation[9] Correction factors for the IL-1400A meter were obtained from further, more detailed laboratory measurements of at least ten representative ILT lamp fixtures using two well-characterized, digital spectroradiometers: an International Light Technologies, ILT950 wideband (25–1050 nm) spectroradiometer SN 1007094U1 with W4 and W2 receptors, calibrated within 3 months of the measurement, with SpectrlLight III, v3.4 software; and, a Mightex HRS-UV1-025 UV (230–450 nm), calibrated within 3 months of the measurements with software v2.11. The measured spectra permitted a calculation of the effective UV irradiances for comparison with the portable meter readings and derive a correction factor for field measurements of ILTs. There was no attempt to develop correction factors for the Ocean Optics spectrometer.
Reference lamp spectra
In addition to our own laboratory measurements, two lamp manufacturers provided reference spectra: (1) a spectrum of a Sylvania BLB fluorescent tube was obtained from the Osram-Sylvania laboratories in Danvers, MA, courtesy of Mr. David Gross, who employed an Optronics Model OL-750D double-monochromator based spectroradiometer system with two photomultiplier tubes and one silicon detector to obtain a highly reliable spectra with very low stray light; and (2) a similar laboratory reference spectrum of a typical type F40BLB (Black Blue) obtained with an Optronix Model 756 double monochromator spectrometer, provided by General Electric Co., Nela Park, Cleveland OH for a GE black light fluorescent lamp, courtesy of Mr. Mark Duffy.
Results
Exposure conditions in indoor workplaces
As a general observation, workers in food-processing plants, pharmaceutical laboratories, kitchens, offices or outdoor settings would rarely be within 30–100 cm from an ILT lamp fixture except for momentary encounters, and the total daily accumulated exposure durations would be less than one hour at a distance of 1–2 m. Ceiling-mounted fixtures were generally installed 8 ft (244 cm) or higher from the floor. Most indoor ILT installations were properly installed away from continuously occupied areas. The closest continuous-exposure ever noted by the ILT systems engineers at Gilbert Industries was 75–100 cm (∼2.5–3.3-ft), where a Gilbert Model 225GT fixture (with two 20-W bulbs) was improperly positioned above a food-washing sink where workers were at least indirectly exposed (as they looked downward). However, the effective UV irradiance limit and UV-A limit for an 8-hr exposure was only reached within 30 cm, so no potentially hazardous exposures existed. A photograph could not be taken of this location. Other representative locations are shown in and the exposures are described below.
First Installation (): An ILT was installed in a vestibule corner at an office building entrance. Typical daily, time-weighted and distance-weighted exposures were estimated as equivalent to 3 s/day at 1 m; however, the worst conceivable exposure for two persons talking in the entryway was 15 min at 1 m. The time-weighted-average (TWA) irradiance was below the 8-hr daily limit.
Second Installation (): A small, wall-mounted ILT unit was mounted 1.9 m above the floor in an office. The closest distance to an individual seated in the waiting room, facing the wall sconce was 3 m and a worst-case, but unlikely, TWA irradiance at this position did not exceed the 8-hr limit. However, visitors could be standing as close as 50 cm for as long as 30 minutes (although facing away from the wall), but exposures would not exceed the worst-case, UV limit for 2,000 sec at 50 cm.
Third Installation (). An ILT was next to a loading-dock vestibule in a pharmaceutical laboratory. This corner-mounted unit was positioned just outside an entry vestibule, where the inside door would not open until the outside door shut. This interior ILT caught insects that passed through both doors, despite another ILT (not shown) inside the vestibule. This was typical in many facilities, following the principle to catch insects near routes of entry. Some employees frequently passed by the fixtures at approximately 1 m, but the total, worst-case cumulative exposure was calculated to be ∼15 min (i.e., <1,000 sec) for exposure dose assessment—leading to a TWA irradiance well below the daily exposure limit.
Fourth Installation (). ILT in a shipping dock area. This large unit was positioned more than 3 m above the floor, perpendicular to the dock door. Employees prepared shipments or passed under the fixture momentarily while operating a fork-lift truck. Conservative, worst-case exposures were 8 hr in one day, but the exposure distance corresponding to a TWA irradiance limit for 8 hr was greater than 3 m.
Fifth Installation (): An ILT was 3 m above the floor, located in a pass-through area above refuse containers in a pharmaceutical laboratory. Worst-case cumulative daily exposures of any person were estimated at less than 15 min/day (i.e., <1,000 sec) at 2 m—leading again to a TWA irradiance well below the daily limit.
Sixth Installation (): A small, wall-mounted ILT unit was mounted 1.9 m above the floor, located in an employee refreshment area. Typical daily exposures were of the order of 60 sec; however, considering some congregation and talk, a conservative estimate was set at 15 min (<1,000 sec) at 1 m; or 1 hr at 2 m. Even if an employee were to wash dishes all day, they would not stare at the lamp.
Seventh Installation (): An ILT was located in a commercial kitchen. Most workers would receive only momentary exposures a few times each day as they passed by, and total ocular exposures at 60–100 cm would be less than 60 sec each day. Some workers would work nearby, albeit with their backs facing the ILT as close as 1.4 m, from time to time. In the worst conceivable case, a few were exposed as much as 8 hr/day, but since they wore long-sleeved smocks, there was only a few minutes of direct, facial exposure. The cumulative exposure was conservatively set at a TWA equivalent irradiance of 1 m for 30 min/day. Others could view the ILT from approximately 3 m across the food preparation table for up to 2 hr/day, although they would be looking downward. This exposure was conservatively placed at 2 hr/day with a TWA irradiance at 3 m—again, well below the daily limit.
Eighth Installation (): An outdoor ILT was suspended from a tree only 3 m (10 ft) from a picnic shelter—even though the manufacturers of outdoor ILTs recommend in their instructional materials to position these types of units well away from areas of human activity (e.g., 20–40 ft away, i.e., 6–12 m), and near bushes, wooded areas, adjacent to standing water (but not swimming pools). All other outdoor installations were at much greater distances and are therefore not reported here. Even if this unit were incorrectly placed in the middle of a picnic table, individuals would not likely sit at the table for more than a couple of hours. The worst case exposure duration might be 10,000 sec, but the daily equivalent TWA irradiance would not exceed the daily limit.
Additional Installations (): Four other industrial ILTs were measured that could be substituted in the previous eight installations and would have an effective irradiance below the 8-hr UV limit of 0.1 µW•cm−2 at 20 cm.
Figure 1. Typical industry applications of ILTs in indoor settings. Exposure rates (irradiances) at 100 cm only 3″ fell below 0.1 mW-cm2.
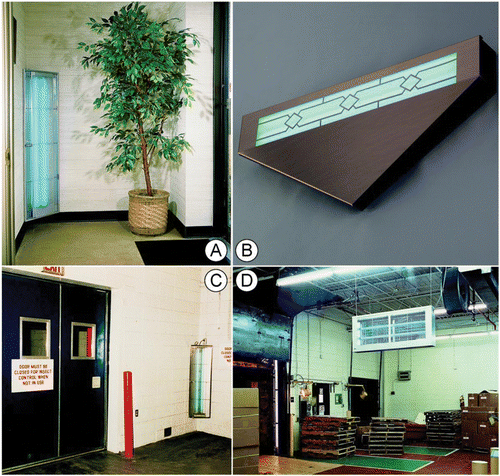
Maintenance and servicing
For proper operation ILTs may require periodic cleaning and lamp replacement. Normally, the lamps are turned off during this process, but if an individual attempted to clean or replace the lamps during UV emission, the eyes could be exposed as close as ∼50 cm for as much as one minute for each lamp fixture (<30 µJ·cm−2 at each fixture). However, even if the same individual were to service as many as 100 fixtures in a plant, the total ocular exposure would still not quite reach the daily actinic exposure limit of 3 mJ·cm−2 = 3,000 µJ·cm−2 and would be well below the conservative ICNIRP limit of 1.0 J·cm−2 for direct staring at a source.Citation[11] Hence, there is no realistic concern with regard to maintenance and service, since even by assuming unrealistic exposure conditions attempting to clean or service many units would pose no realistic hazard. Therefore, there is no justification for system safety features such as interlocks to preclude lamp operation during servicing.
Radiometric characteristics of the UV lamps
As noted earlier two BL lamp categories are common: those with a peak emission at ∼368 nm and those with a peak wavelength near 352 nm. Representative lamps from several lamp manufacturers were tested (). A highly reliable spectral irradiance measurement from Osram-Sylvania, a major supplier of these types of lamps, is shown in .
Table 1. ILTs (insect light traps) studied.
Figure 4A. Spectral irradiance of Osram-Sylvania F40/350BL 40-watt T12 tubular fluorescent lamp at a distance of 10 cm from the lamp tube surface. This high-quality well-calibrated spectrum was obtained using a double-monochromator with low stray-light (Model OL 750-D, Optronic Laboratories, Orlando, FL). Spectral radiance is 2 x the above vaues/sr.
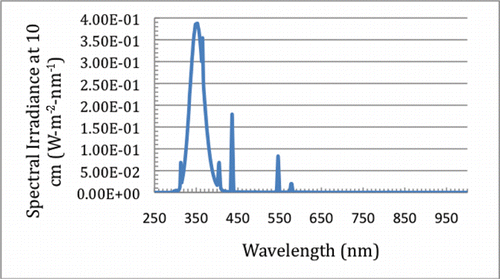
Figure 4B. Semi-logarithmic plot of spectral irradiance of Osram-Sylvania F40T12/350BL 40-watt tubular fluorescent lamp at a distance of 10 cm from the lamp tube surface. This high-quality well-calibrated spectrum was obtained using a double-monochromator with low stray-light (Model OL 750-D, Optronic Laboratories, Orlando, FL).
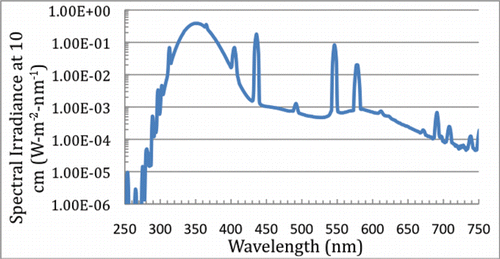
Irradiance as a function of distance
Total UV-A irradiance as a function of distance from three representative fixtures that had maximized irradiance with new tubes for each lamp-wattage type (15, 20, and 40 W) is shown in . The actual values summarized in are plotted as curves along with each measurement in on a semi-logarithmic scale to show that beyond approximately 50 cm, the irradiance decreased inversely as the square of the distance. The total UV-A irradiance was measured at a standard reference distance of 20 cm (specified in IESNA RP27.2 and RP 27.3, as well as CIE S009/IEC6247 to achieve reliable measurements of trace UV-B emissions).Citation[9,13–15] Most lamp systems (i.e., lamps with fixtures) easily met the 1.0-mW·cm−2 UV-A limit for the Exempt (RG0) risk group, but a few just met the 3.3-mW·cm−2 UV-A limit for Risk Group RG-1. No ILT units exceeded the UV-A 8-h irradiance limit at distances greater than 60 cm. However, the lamps systems also had to be assessed for actinic UV—the S(λ)-weighted (largely UV-B) irradiance.
Table 2. Insect light trap (ILT) maximum unweighted UVAFootnotea and effective actinic UVFootnoteb irradiances at relevant exposure distances.Footnotec The ultraviolet values were computed in a spread-sheet from absolute spectral irradiance data collected with a rapid scan single grating CCD spectroradiometerFootnoted while employing additional precautions to reduce errors from stray light.
Figure 5. Actinic S(λ)-spectrally weighted irradiances for all ILT units tested. Note that none of the units—even larger (Pelectrical > 100 W) units—exceeded the daily exposure limit of 0.1 µW·cm−2 at 200 cm.
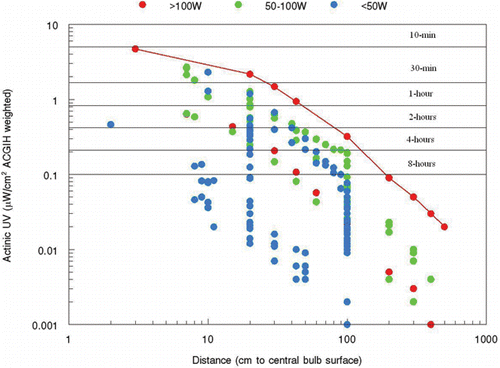
Figure 6. Non-weighted total (300–400 nm) irradiances for all ILT units tested. Note that none of the units—even larger (Pelectrical > 100 W) units—exceeded the daily ACGIH and ICNIRP exposure limit of 1-mW·cm−2 for skin at 50 cm. However, the highest-power units emitted total UV-A exceeding the more conservative ICNIRP (eyes only) limit of 33 µW·cm−2 for 8-hr out to as much as 250 cm. Of course continuous staring at such lamps for hours is totally unrealistic.
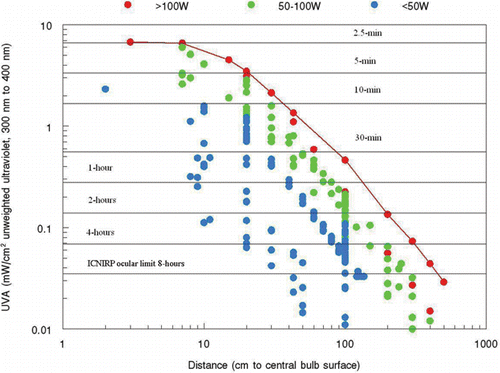
UV-hazard effective irradiance
The effective UV irradiance for safety assessment requires either spectroradiometric measurements that are then spectrally weighted using the UV-Hazard Spectral Weighting Function S(λ), as shown in , or a direct-reading instrument such as the IL1400A with an SEL240 detector with a spectral response to directly record S(λ)-weighted effective irradiance. Measuring trace amounts of actinic, UV-B radiation in the presence of far greater fractions of far less biologically effective (hazardous) UV-A radiation produces uncertainties from stray light in direct-reading instruments and single-monochromator spectroradiometers, leading to over calculation of the S(λ)-weighted irradiance. Although double monochromators introduce far less uncertainty from stray light, the reduced signal-to-noise ratio frequently introduces a false signal of UV-B and UV-C from noise. In either case, the apparent measurement will be always higher than the true value.Citation[9] We found that it was necessary to rely on the highly reliable laboratory spectroradiometric reference measurements at 10–20 cm to determine correct ratios of UV-B, UV-A, and UV-effective to the total irradiance prior to measuring effective irradiances at greater distances either with compact spectroradiometers or hazard meters. These ratios are given in
UV-effective irradiance as a function of distance
Although the broad-band (IL SEL240) safety meter provides a reliable reading for most white-light sources, it was found to over-respond in the UV-A compared to the highly reliable spectroradiometric reference measurements used to determine ratios of UV-B, UV-A,
and UV-effective to the total irradiance measurements with the spectroradiometers. For field studies, the spectral ratios in from the reference spectra were used to estimate the biologically effective UV-hazard irradiance as a function of distance based upon the total UV measurements presented in . The actinic UV values in provide for an overly conservative risk assessment, since reference spectra () are for new, freshly installed lamps emitting maximum irradiances. Laboratory spectroradiometric measurements were made of a wider variety of fixtures (with 100 lamps) as a function of distance as summarized in and 8. lists all of the types of lamps studied in the laboratory.
Table 3. List of lamps that were measured (studied).
Spectral radiance and radiance for retinal hazard assessment
The Osram-Sylvania spectroradiometric measurements were also converted to spectral radiance by dividing by an effective solid angle of 0.5 sr. Hence the full radiance was ∼3.6 mW·cm−2·sr−1. Since the B(λ) spectral weighting in the UV-A is 0.01 for all wavelengths less than 380 nm, the effective blue-light radiance LB is quite low—far below the 10 mW·cm−2·sr−1 for continuous exposure. The aphake A(λ) spectrally weighted radiance was 17 mW·cm−2·sr−1, corresponding a 98-min “stare time” at very close distance, which would be absurd, and not considered in lamp safety standards because of the unrealistic exposure.Citation[12–15]
Discussion
Ultraviolet damage to ocular tissues has been studied in the past in order to establish occupational exposure limits for individuals working with intense light sources and industrial UV sources. The International Commission on Non-Ionizing Radiation Protection (ICNIRP) and the American Conference of Governmental Industrial Hygienists (ACGIH) have published the most current occupational and public exposure guidelines for UV radiation (UVR).Citation[10,11] The rationale and an in-depth review of potential UV hazards were most recently updated and published in 2004;Citation[11] the ACGIH documentation includes updates through 2015.Citation[10]
The eye is well adapted to protect itself against optical radiation (ultraviolet, visible, and infrared radiant energy) from the natural environment and mankind has learned to use protective measures, such as hats and eye-protectors to shield against the harmful effects upon the eye from very intense ultraviolet radiation (UVR) present in sunlight over snow or sand. The eye is also protected against bright light by the natural aversion response to viewing bright light sources. However, the UV-A fluorescent lamps employed in ILT fixtures have been intentionally selected because they are not annoyingly bright to humans, but are attractively bright to insects. Hence, the ILT lamps are not very discomforting to view from the standpoint of visual brightness. However, because of the strong fluorescence of the crystalline lens,Citation[16] some aversion to lengthy viewing exists—particularly at close range of a meter or less—because of the visual annoyance produced by this effect. For this reason it is not reasonably foreseeable that anyone would stare into a UV-A source for a cumulative duration exceeding 1000 sec in any day, and this was also the basis for the UV-A Exempt criterion in lamp safety standards.Citation[12–15]
The ACGIH and ICNIRP provide exposure limits for optical radiation and the ANSI/IESNA RP-27-3 and CIE S009/IEC62471 lamp-safety standards provide at least five separate types of limits to protect against different optical hazards to the eye (from UV to infrared) that are currently recognized. Each of these should be evaluated for any product. Citation[13–15] Only two (possibly three) limits need to be addressed of these five: (1) UV photochemical injury to the cornea (photokeratitis); (2) UV photochemical effects in the lens (cataract); and (3) blue-light photochemical injury to the retina of the eye (principally 400–550 nm; unless aphakic, 310–550 nm), which was not deemed likely but was check-tested. Therefore, this article focuses on the potential photochemical effects of the ultraviolet radiant energy emitted from ILTs.
Ultraviolet exposure limits
UV exposure criteria have evolved over the last several decades based upon biomedical laboratory research, human epidemiological studies, and clinical experience.Citation[11,17–23] The current UV exposure limits are identical internationally for the skin, but differ for eye exposure durations greater than 1000 sec. The primary guideline to protect the skin and the cornea is an S(λ) weighted () daily (8-hr) exposure Heff of 3 mJ·cm−2 or 30 J·m−2 referenced to 270 nm, which corresponds to 5 J·cm−2 at 350 nm and 27 J·cm−2 at 365 nm.Citation[10,11] This S(λ) weighted daily exposure limit is also about 1/3 to 1/4 of a minimal erythemal dose for the skin and less than 1/2 the exposure necessary for clinically reported photokeratitis. The S(λ) action spectrum for safety studies was recently re-evaluated and revalidated in the 300–320 nm region, where some published threshold injury data had appeared to conflict with the exposure limits.Citation[18] Both ACGIH and ICNIRP recommend the same limit as a guideline value for skin exposure—a goal to try to achieve, but not as a ceiling value, as it is for the eye, since the risk varies greatly with skin types. In addition to the primary S(λ)-weighted limit, a second limit that is not spectrally weighted is provided to protect the lens from excessive exposure to the UV-A: it is a 1 J·cm−2 limit applying to all radiant energy between 315 and 400 nm. ACGIH applies this only to exposures less than 1,000 sec, and recommends a dose-rate limit of 1 mW·cm−2 for longer exposures. However, ICNIRP applies the 1 J·cm−2 limit to a full-day (30,000 sec) exposure.Citation[11] It is the latter limit that is most restrictive for 360–370 nm. This is a limit that considers chronic outdoor solar exposure over a lifetime, and would be excessively conservative to apply in the context of occasional exposure to UV-A from ILT fixtures. Indeed, the ANSI and CIE standards for photobiological safety of lamps and lighting systems, consider the 1 mW·cm−2 limit as a reasonably foreseeable worst-case time-weighted-average irradiance, which would still meet the ICNIRP limits in any one day.Citation[11–15]
To place the exposure in perspective, it is useful to consider environmental exposure to the UV in sunlight.Citation[19] Outdoor exposures—particularly in mid-summer—routinely exceed the ACGIH TLV of 1 mW·cm−2, and greatly exceed the ICNIRP UV exposure guideline of 1 J·cm−2 over a full day.Citation[23] If one calculates the permissible exposure duration for horizontal exposure in the prone position with the eyes directed to the sun (totally unrealistic), the exposure limits may be exceeded in 5–10 min on a bright summer day at noon time. However, under realistic outdoor situations, the ocular exposure does not actually exceed the limit for even greater exposure duration of several hours, except over sand and snow. Corneal examinations of humans exposed in a desert environment for much of the day just began to show the signs of threshold photokeratitis.Citation[20–21,23] Although an individual can readily look directly at a UV-A ILT lamp, the 1 mW·cm2 limit was not achieved from any of the fixtures at distances greater than 50 cm and cumulative exposures (TWA) cumulative exposures could not even exceed the overly conservative ICNIRP limit of 1 J·cm−2 extended to 8 hr.
Ultraviolet exposure limit formulations
The two limits that apply to near-ultraviolet exposures—a spectrally weighted limit to protect the cornea (Eq. (Equation1(1) )) and an un-weighted UV-A limit (Eq. (Equation2
(2a) )) intended to protect the lens and retina.Citation[10,11] These are:
(1) and
(2a) and
(2b)
To calculate the maximum direct viewing duration when either (Equation1(1) ) or (Equation2
(2a) ) is not satisfied, the maximum “stare time,” tmax, is found by inverting Eqs. (Equation1
(1) ) or (Equation2a
(2a) ) for a CW source with a weighted or un-weighted irradiance:
(3a)
(3b)
The ICNIRP UV-A exposure limit for the eye (not skin) would be: (1 J·cm−2)/(30,000 sec) = 33 µW·cm−2; however, as noted before this is considered greatly overly conservative to apply to any condition except staring into a source continuously, which is unrealistic for any lamp application, and therefore not applied in IESNA RP 27.3-05 or CIES009/IEC62471:2006 for defining the Exempt risk group. Sadly, an overzealous technician sometimes just ignores the concept of TWA, without thinking that the weighted irradiance limit of 0.1 µW•cm−2 just applies to the 8-hr (30,000 sec) TWA exposure limit. Then, if applied as if relevant at the 20-cm standardized measurement distance (chosen largely to minimize stray-light measurement problems) one could reach the absurd conclusion that there is actually a hazard, although no one would be so close for more than a minute!
Blue-light photochemical retinal hazard
The ACGIH TLVCitation[10] and ICNIRP guidelinesCitation[11] are identical for large sources and are designed to protect the human retina against photoretinitis, “the blue-light hazard” is an effective blue-light radiance LB spectrally weighted against the Blue-Light Hazard action spectrum B(λ) and integrated for t s of 100 J·cm−2·sr−1, for t < 10,000 sec, i.e.,
(4) and for t > 10,000 sec (2.8 hr):
(5)
To calculate the maximum direct viewing duration when (Equation5(5) ) is not satisfied, this maximum “stare time,” tmax, is found by inverting (Equation4
(4) ) for a CW source with a weighted radiance of LB:
(6)
The radiance values are averaged over a field of view which is not less than 11 mrad = 0.011 rad. The blue light hazard is evaluated by mathematically weighting the spectral irradiance, Eλ, against the blue-light hazard function to obtain EB and then converting this to a radiance LB by dividing by the solid angle of the lamp source. As an example, using the Osram-Sylvania reference measurement, this solid angle of uniform brightness was 0.5 sr. The total, un-weighted lamp radiance was 3.6 mW·cm−2·sr−1, and since B(λ) = 0.01 from 310–380 nm, then LB ∼ 0.04 mW cm−2·sr−1. Since the applicable limit Eq. Equation(5)(5) was 400 times greater, the retinal limits were not further checked for other lamps, since this potential hazard was clearly unrealistic.
Occupational UV-A exposures
All persons within the area of ILTs employing the UV-A fluorescent lamps may be exposed to some scattered UV-A from the lamp fixtures. However, it is not reasonably foreseeable that UV-A exposures over a long day would be directly viewed. Even if the ILTs were occasionally viewed directly, or if reflected by a mirror, the irradiance at the eyes of either a standing or sitting individuals is shown clearly to be far below the 1-mW·cm−2 irradiance—and even the overly conservative ICNIRP UV-A limit of 1 J·cm−2 at the cornea of the eye. The total irradiance at the face of anyone standing in front of an ILT would always be well below the ACGIH occupational exposure limitCitation[10] for lengthy viewing (t > 1,000 s) of 1 mW·cm−2 at distances greater than 50 cm. In point of fact, most of the energy absorbed in the crystalline lens of a normal eye is re-emitted as harmless, longer-wavelength fluorescence.Citation[16]
Conclusions
Laboratory and field measurements of a wide variety of ILTs ( and ) showed that UV-A radiation exposures during any reasonably foreseeable worst-case daily exposure condition would not exceed the currently recommended occupational exposure limits (TLVs) for UV-A (315–400 nm) radiant energy to protect the eye and skin. The limits are maximal routine TWA exposure doses to protect the skin, the crystalline lens and retina for daily workplace exposures over a lifetime. These spectrally dependent limits were based upon corneal damage studiesCitation[13–18] and skin erythema thresholds.Citation[13] The UV-A lamps in this study also had far too low a UV-A radiance to pose any retinal concerns from the “blue-light hazard,” and would not be stared into for lengthy periods by the very rare person who has no crystalline lens (aphakic); they would find it quite bright to look at.
With regard to the trace amounts of actinic UV-B radiation emitted from all fluorescent lamps, it is important to recognize that both the ACGIH and ICNIRP exposure guideline of 3 mJ·cm−2, i.e., 0.1 µW·cm−2 for 30,000 sec (8 hr), and certainly the ANSI/IEC emission limit for very low-risk lamps (Risk Group 1) of 0.3 µW·cm−2, would not be exceeded at conceivable working distances in normal use. The installation of these lamps would only rarely exceed the overly conservative 8-hr guideline of ICNIRP for direct ocular exposure (e.g., from : at 150 cm for one large unit).
ILTs serve a very important health benefit and some insects respond best to wavelengths less than 350 nm. The potential optical hazards are virtually non-existent. By any reasonable estimate of worst-case exposure conditions, users would never be exposed in excess of the currently recommended exposure limits of ACGIH or the IESNA RP27.3-2005, “Photobiological Safety of Lamps and Lamp Systems.” These UV-A exposures are considerably less than in the outdoor environment.Citation[17,19]
For an application standard in the IESNA RP27-series of recommended practices, it is recommended that ILTs that emit less than 0.1 µW·cm−2–effective [S(λ)-weighted] irradiance at 100 cm and less than 1 mW/cm2 UV-A (un-weighted) at 100 cm be considered in the Exempt (RG0 category). Risk Group 1 (very low potential hazard) could be applicable to commercial lamp products with assessment distances depending upon size, such as 150 cm for products less than 50 W; 300 cm for power inputs of 50–120 W and 300 cm for lamp products exceeding 120 W.Citation[24]
There is certainly no justification for any warning label or indication of a “hazard distance,” since TWA exposures are so low compared to irradiance distances for a fixed 8-hr exposure distance! Any such warnings or “hazard distances” would only raise needless concerns for the consumer and would be counter-productive to public health and safety by gross over-statement of risk.
Acknowledgments
The high-quality well-calibrated spectrum (plotted in two ways) in Figures 4a and 4b was provided courtesy of David Gross (Osram-Sylvania, Danvers, MA). Mr. Barry Grimes, an employee of Gilbert Industries, Inc., conducted some of the optical assessment testing which helped guide further studies, but did not appear in the article. Gilbert Industries, Inc., Gardener Manufacturing, Inc., and Armatron Company provided ILTs for this study.
References
- Windsor, H.: ‘Electric chair’ for insects aids farmers. Pop. Mechan. 61(3):406–407 (1934).
- Gilbert, D.: Insect electrocutor light traps, In Insect Management for Food Storage and Processing, Baur, F. J. (ed.). St. Paul, MN: American Association of Cereal Chemists, 1984. pp. 87–108.
- Thimijan, R.W., and L. G. Pickens: A method for predicting house fly attraction of electromagnetic radiant energy. J. Econ. Ent. 66(1):95–100 (1973).
- Weidhass, D.E., J.P. Hollingsworth, E.G. Thompson, and D.F. Davis: An evaluation of electrocuting 5145light traps for fly control in buildings. In Gilbert® Sponsored Insect Electrocuting Light Trap Research. Jonesboro, AR: Gilbert Industries, Inc., 1986. pp. 33–75.
- Lillie, T.H., and J. Goddard: Operational testing of electrocuting traps for fly control in dining facilities. J. Econ. Entomol. 80(4):535–538 (1987).
- Pickens, L.G.: Relative Attractiveness of Paired BL and BLB fluorescent bulbs for house and stable flies (diptera: muscidae). J. Econ. Entomol. 82(2):535–538 (1989).
- Code of Federal Regulations: U. S. Food Code Ch. 6 Physical Facilities, Section 6-202.13, Insect Control Devices and Installation (2013).
- Olsen, A.R., and T.S. Hammack: Isolation of salmonella ssp. from the housefly, musca domestica l., and the dump fly, Hydrotaea aenescens (Wiedemann) (Diptera: Muscidae), at Caged-Layer Houses. J. Food Protect. 63(7):958–960 (2000).
- Wengraitis S., D. Benedetta, and D. H. Sliney: Intercomparison of effective erythemal irradiance measurements from two types of broad-band instruments during June 1995. Photochem Photobiol. 68(2):179–182 (1998).
- American Conference of Governmental Industrial Hygienists (ACGIH): 2015 TLV's, and BEIs Based on the Documentation of the Threshold Limit Values for Chemical Substances and Physical Agents & Biological Exposure Indices, American Conference of Governmental Industrial Hygienists, Cincinnati, OH; with Documentation, Ultraviolet Radiation.
- International Commission on Non-Ionizing Radiation Protection (ICNIRP): Guidelines on limits of exposure to ultraviolet radiation of wavelengths between 180 nm and 400 nm (incoherent optical radiation), Health Phys. 87(2):171–186 (2004).
- International Commission on Illumination (CIE): Recommendation on Photobiological Safety of Lamps. A Review of Standards. CIE Report 134-3-1999, CIE Collection in Photobiology and Photochemistry. Vienna: CIE (1999).
- American National Standards Institute/Illuminating Engineering Society of North America (ANSI/IESNA): Photobiological Safety for Lamps & Lamp Systems – Measurement Systems, RP27.2-00 IESNA.
- American National Standards Institute/Illuminating Engineering Society of North America (ANSI/IESNA): Photobiological Safety of Lamps and Lighting Systems, RP27.3, New York, IESNA (2006).
- Commission International de I'Eclarage, the international Commission on Illumination (CIE): Standard S-009E-2002, Photobiological Safety of Lamps and Lamp Systems, Vienna, CIE, Joint-logo standard with IEC as: IEC64271-2006.
- Zuclich, J.A., F.H. Previc, B.J. Novar, and P.R. Edsall: Near-UV/blue light-induced fluorescence. J. Biomed. Opt. 104:044021-4 (2005).
- Sliney, D.H., and M.L. Wolbarsht: Safety with Lasers and Other Optical Sources, New York: Plenum Publishing Corp, 1980.
- Chaney, E., and D.H. Sliney: Re-validation of the ultraviolet hazard action spectrum—the impact of spectral bandwidth, Health Phys. 89(4):322–332 (2005).
- Sliney, D.H.: Ocular exposure to environmental light and ultraviolet; the impact of lid opening and sky condition, Dev. Ophthalmol. 27:63–75 (1996).
- Pitts, D.G.: The human ultraviolet action spectrum. Am. J. Optom. Physiol. Opt. 51(12):946–960 (1974).
- Pitts, D.G., A.P. Cullen, and P.D. Hacker: Ocular effects of ultraviolet radiation from 295 to 365 nm. Invest. Ophthalmol. Vis. Sci. 16(10):932–939 (1977).
- Zuclich, J.A.: Ultraviolet-induced photochemical damage in ocular tissues. Health Phys. 56(5):671–682 (1989).
- Sliney, D.H.: Estimating the solar ultraviolet radiation exposure to an intraocular lens implant. J. Cataract Refract. Surg. 13:296–301 (1987).
- Sliney, D.H., R. Bergman, and J. O'Hagan: Photobiological risk classification of lamps and lamp systems—history and rationale. Leukos. [In Press] DOI: 10.1080/15502724.2016.1145551.