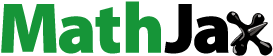
ABSTRACT
In conceptual exposure models, the transmission of pollutants in an imperfectly mixed room is usually described with general ventilation multipliers. This is the approach used in the Advanced REACH Tool (ART) and Stoffenmanager® exposure assessment tools. The multipliers used in these tools were reported by Cherrie (1999; http://dx.doi.org/10.1080/104732299302530) and Cherrie et al. (2011; http://dx.doi.org/10.1093/annhyg/mer092) who developed them by positing input values for a standard Near-Field/Far-Field (NF/FF) model and then calculating concentration ratios between NF and FF concentrations. This study revisited the calculations that produce the multipliers used in ART and Stoffenmanager and found that the recalculated general ventilation multipliers were up to 2.8 times (280%) higher than the values reported by Cherrie (1999) and the recalculated NF and FF multipliers for 1-hr exposure were up to 1.2 times (17%) smaller and for 8-hr exposure up to 1.7 times (41%) smaller than the values reported by Cherrie et al. (2011). Considering that Stoffenmanager and the ART are classified as higher-tier regulatory exposure assessment tools, the errors is general ventilation multipliers should not be ignored. We recommend revising the general ventilation multipliers. A better solution is to integrate the NF/FF model to Stoffenmanager and the ART.
Introduction
A standard Near-Field/Far-Field (NF/FF) model is the simplest model to describe concentration gradient near a source in an imperfectly mixed room. Since the 1950 s, when the model was introduced,[Citation1] it has been one of the basic mathematical tools for occupational hygienists and is well accepted in regulatory exposure modeling's.[Citation2] The standard NF/FF model is also used to the NF and FF concentration ratios for different room volumes and ventilation settings. The concentration ratios are called as a general ventilation multipliers, which are used to scale the concentration level predicted for a standard environment to other environments. A higher multiplier means a lower dilution than in the reference environment, i.e., smaller room and poorer ventilation. Conceptual exposure models Stoffenmanager v.7.1[Citation3–6] (hereinafter referred to as Stoffenmanager) and the Advanced REACH Tool v.1.5 (hereinafter referred to as ART)[Citation7–14] describes the dilution by using the general ventilation multipliers calculated by using the standard NF/FF modelings by Cherrie[Citation15] and Cherrie et al.,[Citation16] respectively. This is the first study revising the NF and FF concentrations and the ventilation multipliers calculated by Cherrie[Citation15] and Cherrie et al.[Citation16] This is critical considering that Stoffenmanager and the ART are recommended tools for regulatory exposure assessment by European Chemicals Agency (ECHA).[Citation17]
Methods: The standard NF/FF model
In a standard NF/FF model a room is divided into NF volume (VNF) which compromises the source, ERi (mg min−1), for mass entering the room and the FF volume (VFF) which compromise rest of the room (i.e., Vtot = VNF + VFF). The room is ventilated via FF volume (Q, m3 min−1) and there is limited air exchange between the NF and FF volume (β, m3 min−1). The concentrations are assumed to be fully mixed at all the times at the NF and FF volumes. The mass is removed from the room by the FF ventilation. The standard NF/FF model is described in detail by Nicas.[Citation18] Zhang et al.[Citation19] provides a Bayesian statistical framework for the model and Ganser and Hewett[Citation20] introduces different ventilation configurations and shows how the model can be calibrated for different environmental configurations.
The standard NF/FF model mass balance is mathematically described as:
(1)
(1)
where CNF (mg m−3) and CFF (mg m−3) are the NF and FF concentrations, respectively. Here, we verified that the NF/FF calculations using the Eq. Equation(1)
(1)
(1) produced the same results as an modeling example given by Ganser and Hewett[Citation20] and IHMod[Citation21] at high-resolution mode (data not shown).
Results
The general ventilation multipliers according to Cherrie (1999)
Cherrie[Citation15] used the standard NF/FF model Eq. Equation(1)(1)
(1) to calculate the 8-hr average NF and FF relative concentrations (RCNF and RCFF, respectively) and their ratios (RCNF/RCFF) for rooms with various ventilation rates and room volumes. Cherrie's calculations involved two normalizations. The first normalization factor was not explained but it was most likely dividing the NF and FF concentrations with a factor of 10. The other normalization was made by using a concentration in a large room (VFF = 3,000 m3) with a moderate air exchange rate (AER) of 3 hr−1, and β of 10 m3 min−1 resulting here to CNF of 10.6 mg m−3 (). However, it is expected that the normalization factors are constant and the same for both NF and FF concentrations in Cherrie's calculations and thus do not affect to the concentration ratios.
Table 1. Average LONG-TERM (8-hr) NF and FF concentrations calculated using a standard NF/FF model for a source emitting mass at rate of 100 mg min−1.
Following Cherrie's procedure, we calculated the NF and FF concentration ratios. Cherrie's Table II has transcription errors that were not corrected later by publishing an erratum or by studies[Citation4,Citation16] using the results by Cherrie.[Citation15] When excluding the errors and values below 0.1 in Cherrie's Table II, the relative NF and FF concentrations given by Cherrie[Citation15] are on average 9.6 ± 0.8 and 9.1 ± 1.4 times smaller than the respective NF and FF concentrations calculated in this study (). Variation in normalization factor shows that the second normalization factor was not constant or there was an error in the NF and FF concentration calculations. Comparison of the RCNF/RCFF ratios given by Cherrie[Citation15] with our calculated CNF/CFF ratios varied from 0.8–1.3 ().
Cherrie[Citation15] gives the recommended general ventilation multipliers for NF and FF (dgv,NF and dgv,FF, respectively) to reconstruct exposure levels (see Cherrie's Table IV). The general ventilation multipliers were calculated as “The NF multiplier was obtained by taking the average relative NF concentration for the room sizes and ventilation conditions described in the table, re-normalized to the average value for large rooms with good ventilation. The FF multiplier was then calculated by dividing the NF multiplier by the average ratio of the NF to FF concentration obtained in the simulation.” By following the instructions we calculated the general ventilation multipliers from values. The dgv,NF and dgv,FF multipliers calculated in this study ( last two columns) were up to 2.8 times higher as reported by Cherrie.[Citation15] This was because the relative NF and FF concentrations given by Cherrie[Citation15] were different than the concentrations calculated in this study.
Table 2. Recommended general ventilation multipliers for reconstructing 8-hr exposure levels.
The general ventilation multipliers according to Cherrie et al. (2011)
Cherrie et al.[Citation16] calculated the NF and FF multipliers for short-term and long-term exposures by calculating 1-hr and 8-hr average NF and FF concentrations at β = 10 m3 min−1, which were normalized with a reference concentration calculated for NF at VFF = 1,000 m3 and AER = 10 hr−1 (). By following the instructions we calculated the NF and FF multipliers from values ( and ). The NF and FF multipliers calculated in this study are for 8-hr exposure up to 1.7 times lower and for 1-hr exposure up to 1.2 times lower as reported by Cherrie et al.[Citation16]
Table 3. NF and FF multipliers for long-term exposure (8-hr) calculated according to Cherrie et al.[Citation16]
Table 4. NF and FF multipliers for short-term exposure (1-hr) calculated according to Cherrie et al.[Citation16]
Discussion
In a standard NF/FF model a general ventilation is one of the main factor defining the NF and FF exposure levels. The general ventilation multipliers from Cherrie[Citation15] are used in Stoffenmanager and the NF and FF multipliers from Cherrie et al.[Citation16] are used in the ART to describe the general ventilation effect on the NF and FF concentration levels. The recalculated general ventilation multipliers were up to 280% higher and the NF and FF multipliers were up to 44% lower than reported earlier.[Citation15,Citation16] Highest differences were observed for small room volumes and low AER values. The error propagation is not clear, because Stoffenmanager and the ART contains nonlinear calibration factors, the models are based on exposure determinants and not physical quantities, and in Stoffenmanager mass is not conserved.[Citation5,Citation12] In general, the ventilation multiplier overestimation results overestimation in exposure level and vice versa.
Considering that Stoffenmanager and the ART are classified as higher tier exposure assessment tools,[Citation5,Citation17] both are recommended by ECHA for regulatory exposure assessment,[Citation22] and that Stoffenmanager has alone over 32,000 users,[Citation3] the errors is general ventilation multipliers should not be ignored. The studies[Citation5,Citation22–30] concerning the tools evaluation, validation, applicability, and sensitivity analysis should be revised and corrected before the tools are used in regulatory risk assessment or before implementing them to tools combining different exposure models, such as a Translation Tool to Support the Use of Regulatory Occupational Exposure Models (TREXMO;[Citation31] see also letter to the editors,[Citation31–33]) SUN decision support system,[Citation34] or risk governance tools developed in caLIBERAte.[Citation35]
In our opinion, the regulatory exposure modeling should rely only on mathematical models following general physical principles, such as conservation of mass, rather than conceptual models based on non-physical models or exposure determinants. Physical units should not be converted to indices or scores to ensure models transparency. For example, in Stoffenmanager the general ventilation multipliers were normalized by using a concentration in a large room (Vtot = 3008 m3) and an AER of 3 hr−1 while in the ART the NF and FF multipliers were normalized by using a concentration in a large room with a volume of 1,000 m3 and an AER of 10 hr−1, which makes comparison of the models more challenging.
We believe that the errors found here would have been revealed much earlier if the tools would rely, e.g., on a standard NF/FF model. Physical and mathematical mass balance models are easy to understand and to develop according to future needs and their performance is superior as compared to conceptual modes.[Citation2,Citation18,Citation36] Thus, it is highly recommended that regulatory exposure modeling is based on physical mass balance models.
Conclusions
Considering the extensive use of Stoffenmanager and the ART in regulatory exposure assessment, the tools general ventilation multipliers should be revised. A better solution is to integrate a well-known NF/FF model to Stoffenmanager and the ART rather than using a quasi-two box model. Regulatory exposure modeling should be based on physical and mathematical mass balance models where unit conversions to indices and scores should be avoided. This would increase the models transparency which reduces risk of errors and makes them easier to develop according to future needs.
Acknowledgements
This work was supported by Danish Centre for Nanosafety II (WP1.3). The authors thank Prof. Paul Hewett (Exposure Assessment Solutions, Inc., Morgantown, WV) for his assistance with checking the calculations.
References
- Hemeon, W.C.: Plant and Process Ventilation. 2nd ed. New York: Industrial Press, 1963. pp. 38–43.
- Jayjock, M.A., T. Armstrong, and M. Taylor: The Daubert standard as applied to exposure assessment modeling using the two-zone (NF/FF) model estimation of indoor air breathing zone concentration as an example. J. Occup. Environ. Hyg. 8:D114–D122 (2011).
- Stoffenmanager: Online exposure and risk tool. Available at https://stoffenmanager.nl/ (accessed December 7, 2017), 2017.
- Marquart, H., H. Heussen, M. Le Feber, et al.: ‘Stoffenmanager’, a web-based control banding tool using an exposure process model. Ann. Occup. Hyg. 52:429–441 (2008).
- Spinazzè, A., F. Lunghini, D. Campagnolo, et al.: Accuracy evaluation of three modelling tools for occupational exposure assessment. Ann. Work Expo. Health. 61:284–298 (2017).
- van Duuren-Stuurman, B., S.R. Vink, K.J. Verbist, et al.: Stoffenmanager Nano version 1.0: A web-based tool for risk prioritization of airborne manufactured nano objects. Ann. Occup. Hyg. 56:525–541 (2012).
- The Advanced Reach Tool (ART): Online exposure tool. Available at https://www.advancedreachtool.com/ (accessed December 7, 2017), 2017.
- Fransman, W., J.W. Cherrie, M. van Tongeren, et al.: Development of a mechanistic model for the Advanced REACH Tool (ART) Version 1.0, TNO report V9009, Zeist, 2010.
- Fransman, W., J.W. Cherrie, M. van Tongeren, et al.: Development of a mechanistic model for the Advanced REACH Tool (ART) Version 1.5, TNO report V9009. Zeist, 2013.
- Fransman, W., M. Van Tongeren, J.W. Cherrie, et al.: Advanced REACH Tool (ART): Development of the mechanistic model. Ann. Occup. Hyg. 55:957–979 (2011).
- Tielemans, E., N. Warren, W. Fransman, et al.: Advanced REACH Tool (ART): Overview of Version 1.0 and research needs. Ann. Occup. Hyg. 55:979–956 (2011).
- Schinkel, J., N. Warren, W. Fransman, et al.: Advanced REACH Tool (ART): Calibration of the mechanistic model. J. Environ. Monit. 13:1374 (2011).
- Schinkel, J., P. Ritchie, H. Goede, et al.: The Advanced REACH Tool (ART) – Incorporation of an exposure measurement database. Ann. Occup. Hyg. 57:717–727 (2013).
- McNally, K., N. Warren, W. Fransman, et al.: Advanced REACH Tool: a Bayesian model for occupational exposure assessment. Ann Occup Hyg. 58:551–565 (2014).
- Cherrie, J.W.: The effect of room size and general ventilation on the relationship between near and far-field concentrations. Appl. Occup. Environ. Hyg. 14:539–546 (1999).
- Cherrie, J.W., L. Maccalman, W. Fransman, E. Tielemans, M. Tischer, and M. Van Tongeren: Revisiting the effect of room size and general ventilation on the relationship between near- and far-field air concentrations. Ann. Occup. Hyg. 55:1006–1015 (2011).
- European Chemicals Agency: Guidance on Information Requirements and Chemical Safety Assessment. Chapter R.14: Occupational exposure assessment. Version 3.0. Available at: https://echa.europa.eu/documents/10162/13632/information_requirements_r14_en.pdf (accessed August 21, 2017), 2016.
- Nicas, M.: Estimating exposure intensity in an imperfectly mixed room. Am. Ind. Hyg. Assoc. J. 57:542–550 (1996).
- Zhang, Y., S. Banerjee, Yang, C. Lungu, and G. Ramachandran: Bayesian modeling of exposure and airflow using two-zone models. Ann. Occup. Hyg. 53:409–424 (2009).
- Ganser, G.H., and P. Hewett: Models for nearly every occasion: Part II – Two box models. J. Occup. Environ. Hyg. 14:58–71 (2017).
- Drolet, D., T. Armstrong, and M. Jayjock: Exposure Assessment Strategies Committee: IH MOD Excel Exposure Models Suite. Available at https://www.aiha.org/get-involved/VolunteerGroups/Documents/IHMOD_Korean-AIHA-MathModel209.xls (accessed January 11, 2018).
- van Tongeren, M., J. Lamb, J.W. Cherrie, L. MacCalman, I. Basinas, and S. Hesse: Validation of lower tier exposure tools used for REACH: Comparison of tools estimates with available exposure measurements. Ann. Work Expo. Health 61:921–938 (2017).
- Schinkel, J., W. Fransman, H. Heussen, H. Kromhout, H. Marquart, and E. Tielemans: Cross-validation and refinement of the Stoffenmanager as a first tier exposure assessment tool for REACH. Occup. Environ. Med. 2:125 (2010).
- Lamb J., S. Hesse, B.G. Miller, et al.: Evaluation of Tier 1 Exposure Assessment Models under REACH (eteam) Project. www.baua.de/dok/6914104 (accessed December 7, 2017), 2015.
- Landberg H.E., P. Berg, L. Andersson, U., et al.: Comparison and evaluation of multiple users' usage of the exposure and risk tool: Stoffenmanager 5.1. Ann. Occup. Hyg. 59:821–835 (2015).
- Landberg, H.E., A. Axmon, H. Westberg, and H. Tinnerberg: A study of the validity of two exposure assessment tools: Stoffenmanager and the Advanced REACH Tool. Ann. Work. Expo. Health. 61:575–588 (2017).
- Riedmann, R.A., B. Gasic, and D. Vernez: Sensitivity analysis, dominant factors, and robustness of the ECETOC TRA v3, Stoffenmanager 4.5, and ART 1.5 Occup. Expos. Mod. Risk Anal. 35:211–225 (2015).
- Koivisto, A.J., A.C.Ø. Jensen, M. Levin, et al.: Testing a Near Field/Far Field model performance for prediction of particulate matter emissions in a paint factory. Environ. Sci. Process. Impacts 17:62 (2015).
- Mc Donnell, P.E., J.M. Schinkel, M.A. Coggins, et al.: Validation of the inhalable dust algorithm of the Advanced REACH Tool using a dataset from the pharmaceutical industry. J. Environ. Monit. 13:1597–1606 (2011).
- Bekker, C., E. Voogd, W. Fransman, and R. Vermeulen: The validity and applicability of using a generic exposure assessment model for occupational exposure to nano-objects and their aggregates and agglomerates. Ann. Occup. Hyg. 60:1039–1048 (2016).
- Savic, N., D. Racordon, D. Buchs, B. Gasic, and D. Vernez: TREXMO: A translation tool to support the use of regulatory occupational exposure models. Ann. Occup. Hyg. 60:991–1008 (2016).
- Savic N., B. Gasic, and D. Vernez: Stoffenmanager® algorithm within Version 6 differs from the published algorithm within old versions and TREXMO. Ann. Work Expos. Health. 61:607–610 (2017).
- Heussen, H., and A. Hollander: Stoffenmanager® exposure model algorithms within TREXMO and Stoffenmanager® lead to different outcomes. Ann. Work Expos. Health 61:604–606 (2017).
- Subramanian, V., E. Semenzin, D. Hristozov, et al.: Sustainable nanotechnology decision support system: Bridging risk management, sustainable innovation and risk governance. J. Nanopart. Res. 18:89 (2016).
- EU H2020 NMP30-2015 caLIBRAte (nr: 686239): www.nanocalibrate.eu/home, (accessed December 7, 2017).
- Nazaroff, W.W., and Cass G.R.: Mathematical modeling of indoor aerosol dynamics. Environ. Sci. Technol. 23:157–165 (1989).