Abstract
During the beginning of the SARS-CoV-2 pandemic, there was a shortage of masks and respirators for the protection of health care professionals. Masks for noninvasive ventilation (NIV) in combination with viral-proof filters, worn by healthcare workers, could serve as an alternative protection measure. We determined the simulated protection factor (SPF) of such devices in comparison to conventional surgical masks, N95, and FFP3 respirators. Masks and respirators were mounted on a ventilated mannequin head in a test-chamber. Isotonic saline containing 150 MBq 99mTC-DTPA (99mTc-diethylenetriamine pentaacetate (DTPA) was nebulized inside the box. The aerosol had a mass median aerodynamic diameter of 0.6 ± 0.4 µm. SPFs were measured using radioactive DTPA particles in the mannequin test system by calculating the ratio of unfiltered particles (Pu) and filtered particles (Pf) for each tested device (SPF = Pu/Pf). Simulated protection factors were 15.6 ± 3.6 for a ResMed AcuCare mask plus filter, 3.5 ± 0.2 for a ResMed Mirage Quattro FX mask plus filter, 9.5 ± 0.8 for a Loewenstein JOYCEclinc FF mask plus filter, 1.9 ± 0.2 for a surgical mask with a rubber band, 2.7 ± 0.7 for a surgical mask with ribbons, 2.3 ± 0.3 for an FFP3 respirator, and 3.6 ± 1.3 for an N95 respirator. The ResMed AcuCare and the Loewenstein JOYCEclinic FF mask were more effective than any other of the tested devices (p < 0.001). In conclusion, masks normally used for NIV with viral-proof filters can effectively filter respirable particles.
Introduction
Health care workers exposed to infectious viruses such as SARS-CoV-2 need better respiratory protection. The U.S. Food and Drug Administration (FDA) recommends the use of N95 masks in health care settings for the protection of airborne particles since they are designed to achieve a very close facial fit and an effective filtration of airborne particles (FDA Citation2020a). In Europe, FFP2 or FFP3 (Filtering Face Piece) masks are recommended to protect health care workers from SARS-CoV-2 transmission (RKI Citation2020). Several countries have introduced a mask requirement in certain public areas for the general population.
The classification and nomenclature of face masks is complex and regulations are different between countries. Surgical masks are loose-fitting, disposable devices that create a physical barrier between the nose and the mouth of the wearer and potential contaminants in the immediate environment (FDA Citation2020a). They were initially designed to prevent the contamination of surgical sites by the operating staff and their effectiveness is measured by the prevention of bacterial contamination caused by the wearer (Frappier and Forte Citation1936; Gauthier Citation1937). N95 filtering facepiece respirators are designed for protection against harmful particulates. N95 respirators are approved by NIOSH for ≥95% efficiency at NIOSH particulate respirator test conditions by means of a sodium chloride-based aerosol according to the requirements under 42 CFR Part 84 (NIOSH Citation2020). The FDA is the competent authority that regulates the use of respirators after NIOSH approval. A surgical N95 respirator is a class II device, regulated by FDA under 21 CFR 878.4040 (FDA product code MSH; FDA Citation2020b). FFP respirators are regulated according to the European norm EN 149 (Lepelletier et al. Citation2020). European-certified FFP1, FFP2, and FFP3 respirators are expected to show minimum efficiencies of 80%, 94%, and 99%, respectively.
Shortage of disposable face masks raises the question, if multi-use filter protection devices could be a solution to the problem. Such a system could be built by the combination of masks which are normally used for noninvasive ventilation (NIV) in patients in combination with a viral/bacterial-proof filter, as shown in . Such a system has to consist of a non-vented NIV mask since vented NIV masks have a built-in leakage system. The filters we used are commercially available filters normally used in circuits of invasive mechanical ventilators with a filter performance of 99.99% for viruses according to the manufacturer’s information. We measured the simulated protection factors of such systems, as shown in , and compared them to disposable N95 and FFP3 respirators as well as surgical masks in an experimental setting including the simulation of spontaneous breathing.
Materials and methods
Experimental setup
In this study, a commercially available mannequin head form was used (Respironics, Murrysville, PA, USA; ). The head dimensions were: face length (mento-nasal root depression length) 12 cm, a nasal root breath of 1.9 cm, and face width (bizygomatic breath) of 14 cm. The head corresponds to medium standard NIOSH head form (Zhuang et al. Citation2007). Nasal and mouth openings of the mannequin connect to a tube that exits the head on the backside. The face was covered with a soft wound dressing (Allevyn plus Adhesive, Smith + Nephew, London, UK; Panel D) in order to simulate the elastic properties of human skin. Experiments were conducted inside an airtight transparent plastic box of 70 liters volume (Iris 70 liter, model # 135455, IRIS Ohyama, Sendai, Japan) (). Three connectors/outlets were installed on the back of the box with a two-component bonding agent (). A fourth connector/outlet on the front side (front1) of the box was connected to a nebulizer (Pari LC Sprint Star, Pari, Starnberg, Germany), and the exhalation port of the nebulizer was sealed with a filter (Iso-Gard #19212, Teleflex Medical GmbH, Felbach, Germany) in order to prevent room contamination. The three connectors on the backside of the box were utilized as follows ( and ): The first outlet (Test) was connected to the backside of the mannequin head inside the box by means of a piece of using a standard single-use ventilator tubing (1574000, Intersurgical, Sankt Augustin, Germany). The second outlet on the backside (Control) was connected to a tube that extended freely into the box and ended abreast to the frontside of the mannequin head. The third outlet on the backside (Air circulation) was inserted for free air circulation during simulated ventilation. Test, Control, and Air circulation outlets were equipped with a filter in order to collect the radioactive particles, as explained in the next section. Outlets Test and Control were then connected to separate chambers of an artificial lung (dual adult test lung model 5600i, Michigan Instruments, Kentwood, MI; ). The two chambers of the artificial lung were interlocked for simultaneous use.
Figure 2. Mannequin head. Nasal and mouth openings of the mannequin connect to a tube/outlet that exits the head on the backside (A–D). This outlet connects to a filter outside the measuring box (E). A soft wound dressing simulates the elastic properties of facial skin (D).
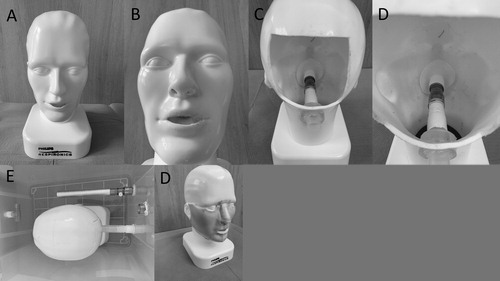
Aerosol generation and delivery
The authors nebulized isotonic saline containing 150 MBq 99mTC-DTPA (99mTc-diethylenetriamine pentaacetate (DTPA)) per mL by means of a Pari LC Sprint Star nebulizer (Pari, Starnberg, Germany; ). The nebulizer was filled with a 6 mL solution. The nebulizer output rate equals 360−500 mg/min. After a nebulization time of 20 sec, the particles were homogeneously distributed and dried for 30 sec. Water from the particles evaporates within a few seconds (less than 5 sec at a relative humidity of 50% and less than 10 sec at a relative humidity of 90%) (Haddrell et al. Citation2014). The mass median aerodynamic diameter (MMAD) of sodium chloride particles emitted from the Pari LC Sprint Star nebulizer is 2.8 µm with a geometric standard deviation (GSD) of 2 µm. The drying process described above thus will result in a particle spectrum of 0.6 ± 0.4 µm MMAD.
The authors selected the above particle size spectrum since it represents the usual particle sizes exhaled through human breathing (Scheuch Citation2020). For every test run the nebulizer delivered the aerosol continuously for 20 sec. Two fans inside the box were actuated after nebulization to ensure homogenization of the aerosol. The entire method has been described previously (Maurer et al. Citation2020).
Simulation of breathing
Breathing was simulated 30 sec after nebulization was stopped by manual movement of the two blocked chambers of the breathing simulator for 10 times within 50 sec, generating a volume of 1 L per lung-chamber with a flow rate of 0.4 l/sec. The volume was visually controlled by means of the build in volume scale of the Michigan lung. The respiratory rate was controlled by means of a metronome (EumLab for iPhone, Xanin Technology GmbH, Berlin, Germany).
Measurement sites and measurement of radioactivity
The filter “Control” refers to the filter sample of the unfiltered reference port, whereas the filter “Test” refers to the filter sample used with a test respiratory device placed on the mannequin face. A test run without filtering devices on the mannequin face revealed equal aerosol deposition on outlets Test and Control. We confirmed complete particle capture efficiency of the aerosol by a single filter by placing a second filter behind the first one which showed no radioactivity after a test run. Filters were then positioned under a Gamma camera (ECAM Scintron, Medical imaging electronics GmbH, Seth, Germany) and radioactivity was counted for 1 min. Regions of interest (ROIs, area around the filter where radioactivity was measured) were placed around the spots of Test and Control filters (). A third ROI apart from the filter spots measured background activity. Activity was measured in total counts per minute.
Test runs
The filtering devices tested in the study are shown in and . The devices were mounted onto the mannequin face as recommended by the manufacturer. Moldable nasal straps of surgical, FFP3, and N95 masks were adjusted to optimal fit the nose bridge. NIV masks were attached with the harness, the ventilation port was connected to a filter, as shown in . The test was repeated three times with new respirator samples. Unlike the NIOSH procedure we did not seal the tested devices with wax to a testing plate but measured the respective device mounted to a mannequin head which allows leakage around the respective mask.
Figure 6. Surgical masks and respirators that were tested on the mannequin head during simulation of spontaneous breathing as described in the Materials and Method section (A: Vlies mouth protector Typ 3 R,Wiros GmbH Willich, Germany; B: OP Mask 657000, Mölnlycke Health Care GmbH, Düsseldorf, Germany; C: FFP 3 mask P-3900, Ampri GmbH, Winsen/Luhe, Germany; D: SH 2950 respirator N95, San Huei United CO. LTD, Taipei, Taiwan).
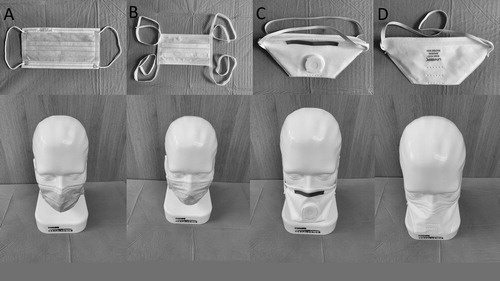
Figure 7. Tested NIV masks (A: AcuCare, ResMed, San Diego, USA; B: JOYCEclinic FF Loewenstein, Bad Ems, Germany; C: Mirage Quattro FX, ResMed, San Diego, USA) and a woven scarf (D, Passigatti, Neu-Ulm, German, 65% polyester, and 35% viscose) which were tested on the mannequin head during simulation of spontaneous breathing as described in the method section. The simulated protection factor of the different masks on the mannequin head is being determined by the filtration efficiency of the tested device and the leakage around the device on the dummy head. In order to determine the performance of a surgical mask without leakage, we clamped a surgical mask over a plastic cup which was connected instead of the mannequin head (E).
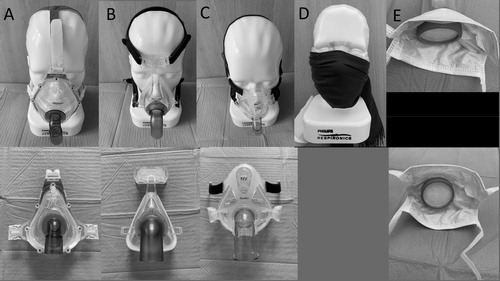
The authors also measured the filter performance of a surgical mask cloth that was stretched and fixed over a funnel as well as the simulated protection factor of a woven scarf wrapped around the mannequin head.
Analysis and statistics
In every test run the filter activity of the filter labeled “Test” represents the amount of filtered particles. Activity in the Control filter serves as the unfiltered reference. Background activity was subtracted from Test and Control filter counts. The ratio of Control filter (Pu) and Test-filter (Pf) represents the simulated protection factor (SPF = Pu/Pf) A similar approach has been described by Gao and coworkers (Gao et al. Citation2016). The SPF has a range of one (corresponding to zero filtration) and infinite. The authors used the Shapiro–Wilk test to check for normal distribution and Levene-test for equality of variances. Pairwise comparisons were done with a non-paired, two-sided t-test with Bonferroni adjustment for multiple comparisons. A p < 0.05 was considered significant. SPSS Version 26 (IBM Corp., Armonk, NY) was used for statistical analysis. Data sharing policy: The whole dataset is online available on the Mendeley data server (https://data.mendeley.com/research-data/?search=dellweg).
Results
Simulated protection factors differed in between the tested devices. Simulated protection factors were 15.6 ± 3.6 for a ResMed AcuCare mask plus filter, 3.5 ± 0.2 for a ResMed Mirage Quattro FX mask plus filter, 9.5 ± 0.8 for a Loewenstein JOYCEclinc FF mask plus filter, 1.9 ± 0.2 for a surgical mask with rubber band, 2.7 ± 0.7 for a surgical mask with ribbons, 2.3 ± 0.3 for a FFP3 respirator, 3.6 ± 1.3 for an N95 respirator, 7.8 ± 0.4% for the surgical mask cloth, and 1.7 ± 0.2% for the woven scarf (, ). shows the simulated protection factor for NIV masks, respirators, surgical masks, and other fabric materials. shows p values and 95% confidence intervals (CI) of the pairwise comparisons.
Table 1. Differences of the simulated protection factor between the tested devices.
shows increased concentration of radioactive particles around the nasal bridge area of the mask.
Discussion
The major finding of our investigation is that two of the three reusable NIV masks in combination with viral-proof filters had a higher simulated protection factor than surgical masks, N95, and FFP3 respirators. Overall, one can determine three types of leakages. First, the amount of aerosol penetrating the filter itself, second the aerosol passing in-between filter and skin, and third a so-called slack-leakage that passes the exhalation valve during early inspiration if an exhalation valve is built-in. NIOSH filter efficiency measurements of respirators require sealing of the respirators to a test plate by means of wax. Such a setup prevents leakage between the device and the facial skin. We coated or mannequin head with artificial skin as described in the method section, however as previously shown by other researchers (He et al. Citation2014) face-seal leakage cannot completely excluded in mannequin experiments. This explains why N95 and FFP respirators had lower simulated protection factors in our experiments. Images from the gamma camera suggest that the main leak flow occurs around the nasal edge of the respirators. The increased leakage of the measured FFP3 device as compared to the N95 filter could be due to the fact that the FFP3 device was equipped with an expiratory valve causing a slack-leakage.
ISO EN 149 testing specifications require to sample aerosols by means of a collecting probe that is passed through the filter medium and samples are taken directly behind the filter medium. A leakage flow in-between the facial skin and the respirator might not reach to this sampling site. We determined the radioactive aerosol concentration from a sample that already passed the upper airways. This ascertains that neither form of leakage flow is missed during the experiment. The airflow bypassing the filter decreases the simulated protection factor. We found that more than one-third of the particle load bypassed N95 and FFP3 respirators as well as surgical masks in our experimental setup.
NIV masks are more comparable to elastomeric respirators which were not tested in our experiments. The NIV mask cushion is less malleable compared to N95 and FFP respirator. This, in combination with a more stable harness which holds the mask in place, could explain the superior performance of NIV masks in our experiment. A mask fit test is required prior to respirator use according to the U.S. Department of Labor/Occupational Safety and Health Administration (OSHA). Such a fit test is based on the feedback of the wearer and cannot be performed on a mannequin. This fact may be in part responsible for the lower simulated protection factors of the N95 and FFP devices.
Two out of three NIV masks, however, showed significantly higher filtration rates. NIV masks are designed to achieve optimal air-tightness during positive pressure ventilation. For this purpose, some of the devices are designed in a way that positive pressure pushes an additional rubber lip toward the skin of the user. Among the tested devices, the ResMed Mirage Quattro FX mask has such a rubber lip over the bridge of the nose. When generating negative pressures during inspiration, there was a recognizable leakage in this area. Infection by pathogens such as viruses depend on many factors. The major factors are virus concentration, exposure time, breathing rate, and filter efficiency of respiratory protection. In general, relatively higher efficiency respirators are expected to provide better protection provided the respirators are properly selected and fit tested. If the lower simulated protection factors of some devices translate into an increased infection risk, is beyond the scope of our investigation. Previous laboratory studies have observed a significant inward leakage of N95 respirators especially with increasing inspiratory effort and breathing frequency (Grinshpun et al. Citation2009; He et al. Citation2014; Rengasamy et al. Citation2014). Grinshpun and coworkers were able to differentiate between the leakage through and around the filtration mask by conducting parallel measurements on human beings (experiment 1) and on a mannikin where the leakage around the mask was technically excluded (experiment 2). By using identical breathing patterns they found that leak flow around the mask was 7 to 20-fold higher than the leakage through the filter medium. This effect increased with increasing particle size. Rengasamy et al. confirmed in their manakin experiments that even small leakages can compromise filtration efficiency significantly. They also found increased leakage with increasing minute ventilation. Similar to the studies by Grinsphun and Rengasamy, we used aerosols in the sub-micron range (<1 µm), however we focused on the comparison of different filtering devices and did not distinguish the quantity of the different leakages nor did we apply different breathing patterns. Previous clinical studies have found significant infection rates despite the use of appropriate respirator systems (Loeb et al. Citation2009; MacIntyre et al. Citation2011, Citation2013; Radonovich et al. Citation2019). Two large field studies and one metanalysis even found that surgical masks were non-inferior to N95 respirators as measured by infection rates (Loeb et al. Citation2009; Smith et al. Citation2016; Radonovich et al. Citation2019). The results of this investigation point out the importance to avoid leakage and ensure close fitting of N95 and FFP3 respirators. Despite the fact that the tested masks for NIV were not designed for the purpose of infection control, they outperformed the N95 and FFP3 respirators as well as the surgical masks.
The purpose of surgical masks is to protect the environment (e.g., a surgical site) from contamination by the wearer. They are not designed to protect the wearer from inhalation of aerosols. When the surgical masks we used was attached to the mannequin head, the mask achieved relatively low simulated protection factors, most likely because of poor fitting. Fitting quality, however, is a major factor for surgical masks as well and previous studies have found poor fitting with high leakage for surgical masks (Pippin et al. Citation1987; Derrick et al. Citation2006; Lawrence et al. Citation2006; Brosseau and Harriman Citation2007).
Using a scarf for protection as frequently seen on public media is probably not sufficient to protect from viral transmission and cannot be recommended. We tested only a small proportion of available mask and respirator systems. More research is needed to investigate if our findings pertain for other devices.
Conclusion
In these experiments N95 and FFP3 respirators were less effective than non-vented, NIV masks in conjunction with viral filters when tested during simulation of spontaneous breathing in our bench model. Our simulated protection factor measured the penetration of particles through the filter medium together with particles that penetrated through a leakage since experiments were not done on sealed devices.
Non-vented, NIV masks in conjunction with viral filters could serve as an alternative in the face of an N95 or FFP respirator shortage. These systems are reusable and could solve a supply problem for single-use respirators and masks.
Limitations
The static bench model used in this study does not take head and/or body movements into account, which appears to be a relevant practical problem (Grinshpun et al. Citation2009). For this reason, our model could underestimate the leakage. The authors ventilated the model with room air which has less humidity than human air in natural airways. Any impact of humidity can therefore not be investigated with our model. The authors tested only one model each of N95 and FFP3 respirators and two surgical models. Further studies are needed to verify the results obtained in our studies.
Availability of data and materials
Data available on request from the authors.
Competing interest
None of the authors has a competing interest in the matter of subject.
Additional information
Funding
References
- Brosseau LM, Harriman K. 2007. Commentary on “In vivo protective performance of N95 respirator and surgical facemask”. Am J Ind Med. 50(12):1025–1026; author reply 1027–1029. doi:10.1002/ajim.20498
- Derrick JL, Li PT, Tang SP, Gomersall CD. 2006. Protecting staff against airborne viral particles: in vivo efficiency of laser masks. J Hosp Infect. 64(3):278–281. doi:10.1016/j.jhin.2006.06.021
- FDA. 2020a. N95 respirators and surgical masks (face masks). https://www.fda.gov/medical-devices/personal-protective-equipment-infection-control/n95-respirators-and-surgical-masks-face-masks.
- FDA. 2020b. Product classification, respirator surgical. https://www.accessdata.fda.gov/scripts/cdrh/cfdocs/cfPCD/classification.cfm?ID=MSH.
- Frappier A, Forte L. 1936. A bacteriological study of the new surgical mask "Jel". Can Med Assoc J. 34(5):547–549.
- Gao S, Koehler RH, Yermakov M, Grinshpun SA. 2016. Performance of facepiece respirators and surgical masks against surgical smoke: simulated workplace protection factor study. Ann Occup Hyg. 60(5):608–618. doi:10.1093/annhyg/mew006
- Gauthier PP. 1937. A comparative study of three surgical masks. Can Med Assoc J. 37(3):250–252.
- Grinshpun SA, Haruta H, Eninger RM, Reponen T, McKay RT, Lee SA. 2009. Performance of an N95 filtering facepiece particulate respirator and a surgical mask during human breathing: two pathways for particle penetration. J Occup Environ Hyg. 6(10):593–603. doi:10.1080/15459620903120086
- Haddrell AE, Davies JF, Miles RE, Reid JP, Dailey LA, Murnane D. 2014. Dynamics of aerosol size during inhalation: hygroscopic growth of commercial nebulizer formulations. Int J Pharm. 463(1):50–61. doi:10.1016/j.ijpharm.2013.12.048
- He X, Reponen T, McKay R, Grinshpun SA. 2014. How does breathing frequency affect the performance of an N95 filtering facepiece respirator and a surgical mask against surrogates of viral particles? J Occup Environ Hyg. 11(3):178–185. doi:10.1080/15459624.2013.848037
- Lawrence RB, Duling MG, Calvert CA, Coffey CC. 2006. Comparison of performance of three different types of respiratory protection devices. J Occup Environ Hyg. 3(9):465–474. doi:10.1080/15459620600829211
- Lepelletier D, Grandbastien B, Romano-Bertrand S, Aho S, Chidiac C, Gehanno JF, Chauvin F. 2020. What face mask for what use in the context of COVID-19 pandemic? The French guidelines. J Hosp Infect. 105(3):414–418.
- Loeb M, Dafoe N, Mahony J, John M, Sarabia A, Glavin V, Webby R, Smieja M, Earn DJ, Chong S, et al. 2009. Surgical mask vs N95 respirator for preventing influenza among health care workers: a randomized trial. JAMA. 302(17):1865–1871. doi:10.1001/jama.2009.1466
- MacIntyre CR, Wang Q, Cauchemez S, Seale H, Dwyer DE, Yang P, Shi W, Gao Z, Pang X, Zhang Y, et al. 2011. A cluster randomized clinical trial comparing fit-tested and non-fit-tested N95 respirators to medical masks to prevent respiratory virus infection in health care workers. Influenza Other Respir Viruses. 5(3):170–179. doi:10.1111/j.1750-2659.2011.00198.x
- MacIntyre CR, Wang Q, Seale H, Yang P, Shi W, Gao Z, Rahman B, Zhang Y, Wang X, Newall AT, et al. 2013. A randomized clinical trial of three options for N95 respirators and medical masks in health workers. Am J Respir Crit Care Med. 187(9):960–966. doi:10.1164/rccm.201207-1164OC
- Maurer L, Peris D, Kerl J, Guenther F, Koehler D, Dellweg D. 2020. Community masks during the SARS-CoV-2 pandemic: filtration efficacy and air resistance. J Aerosol Med Pulm Drug Deliv. doi:10.1089/jamp.2020.1635
- NIOSH. 2020. Approval of respiratory protective devices. Washington, DC. https://www.ecfr.gov/cgi-bin/retrieveECFR?gp=&SID=c9c15fd462ffe5c4f4e85b73f161b2e0&r=PART&n=42y1.0.1.7.67.
- Pippin DJ, Verderame RA, Weber KK. 1987. Efficacy of face masks in preventing inhalation of airborne contaminants. J Oral Maxillofac Surg. 45(4):319–323. doi:10.1016/0278-2391(87)90352-1
- Radonovich LJ, Jr., Simberkoff MS, Bessesen MT, Brown AC, Cummings DAT, Gaydos CA, Los JG, Krosche AE, Gibert CL, Gorse GJ, et al. 2019. N95 respirators vs medical masks for preventing influenza among health care personnel: a randomized clinical trial. JAMA. 322(9):824–833. doi:10.1001/jama.2019.11645
- Rengasamy S, Eimer BC, Szalajda J. 2014. A quantitative assessment of the total inward leakage of NaCl aerosol representing submicron-size bioaerosol through N95 filtering facepiece respirators and surgical masks. J Occup Environ Hyg. 11(6):388–396. doi:10.1080/15459624.2013.866715
- RKI. 2020. Empfehlungen des Robert Koch-Institutes zu Hygienemaßnahmen im Rahmen der Behandlung von Patienten mit einer Infektion durch SARS-CoV-2. https://www.rki.de/DE/Content/InfAZ/N/Neuartiges_Coronavirus/Hygiene.html.
- Scheuch G. 2020. Breathing is enough: for the spread of influenza virus and SARS-CoV-2 by breathing only. J Aerosol Med Pulm Drug Deliv. 33(4):230–234. doi:10.1089/jamp.2020.1616
- Smith JD, MacDougall CC, Johnstone J, Copes RA, Schwartz B, Garber GE. 2016. Effectiveness of N95 respirators versus surgical masks in protecting health care workers from acute respiratory infection: a systematic review and meta-analysis. CMAJ. 188(8):567–574. doi:10.1503/cmaj.150835
- Zhuang Z, Bradtmiller B, Shaffer RE. 2007. New respirator fit test panels representing the current U.S. civilian work force. J Occup Environ Hyg. 4(9):647–659. doi:10.1080/15459620701497538