Abstract
Mobilization of asbestos fibers from a 60-year-old corrugated asbestos cement roof was studied in material samples collected from a domestic house in Southern-Norway. Samples included pieces of the roof itself, rainfall simulated run off-water and moss growing on the roof surface. Weathered roof debris below the rain water system was investigated for asbestos fibers leached from the roof, and air samples were collected for asbestos concentrations in ambient air. Micrographs obtained by scanning electron microscopy show that weathering exposed asbestos fibers on the deteriorated roof surface. A high number of fibers found in the run off-water as well as in the weathered roof debris sample illustrates that exposed fibers are prone to mobilization. Investigation of weathering-induced magnesium depletion by scanning electron microscopy energy dispersive X-ray spectroscopy of asbestos fibers collected from the moss, roof underside and surface revealed that fibers had MgO weight % comparable to reference material. The major element chemistry of the fibers is therefore unaltered. Our findings demonstrate that care must be taken when working on and handling old corrugated asbestos cement roof as asbestos fibers might be released. In order to limit exposure to mobile fibers, moss covering asbestos cement roof should not be removed.
Introduction
The use of asbestos construction materials were banned in Norway more than 30 years ago. Still, such materials are frequently found in old houses. Excellent heat resistance, weatherproofing, and durability are some valued characteristics of asbestos construction materials. Corrugated asbestos cement sheets were extensively used as roof panels in domestic houses and many types of other buildings from the 1930s up to the mid-1980s. These roof sheets contain about 10–15% asbestos fibers with chrysotile as the main asbestos component. Amosite and crocidolite are often present in smaller amounts (HSE Citation2012). Still, and like many other countries, there is no clear overview of the abundance of asbestos in old buildings in Norway.
Years with wind and rain might result in decay of the cement matrix. Asbestos cement products are especially prone to weathering if calcium hydroxide in cement come in contact with acidic rain or dissolves slowly in water (Dyczek Citation2006). Chrysotile fibers are more resistant to weathering than the cement matrix and can appear as a layer on the surface (Burdett Citation2007). Asbestos fibers—loosely bound to the cement matrix—may then be mobilized by wind into air and soil, or be washed out by rainwater. Previous investigations have shown increased asbestos air concentrations in vicinity of buildings with corrugated asbestos cement roofs and asbestos cement panels (Spurny Citation1989; Krakowiak et al. Citation2009). The elevated concentration in air was attributed to breakdown of the cement matrix seen from a mean corrosion velocity of 0.024 mm/year in clean environments (Spurny Citation1989). In a study by Campopiano et al. (Citation2009), a high quantity of asbestos fibers was found in friable material collected from gutters. In South Africa, asbestos fibers were found in the soil around all of the 61 studied houses with corrugated asbestos cement roofs (Phillips et al. Citation2009). This finding was related to weathering of the corrugated asbestos cement roofs or cleaning with high pressure jets. A study by Bornemann and Hildebrandt (Citation1986) indicated that the majority of the mobilized asbestos was washed out by rainwater. Oberta et al. (Citation2018) studied asbestos cement roofs exposed to ambient weathering for nine months. The results showed that free fibers were visible on the surface and confirmed that fibers can be released from dry cement roofs. Asbestos fibers in drain water from asbestos cement roofing has also been suggested to increase the risk of stomach cancer seen among lighthouse workers who used drain water as the main water supply (Andersen et al. Citation1993; Kjaerheim et al. Citation2005).
Another effect of weathering including acidic rain is magnesium depletion, which has been observed for chrysotile fibers (Spurny Citation2000). In addition to climatic conditions, organic acids such as oxalic acid from biological organisms that grow on the roofs can react with the exposed chrysotile asbestos fibers (Favero-Longo et al. Citation2005; Burdett Citation2007). It was found that the relative content of MgO decreases by approximately 15–20% where the fibers are in contact with the vegetative body of lichens. An effect of the MgO depletion is that a modification of surface charge (from positive to negative) was observed with dissolution of the outer brucite layer (Mg(OH)2) (Bales and MorganCitation1985; Holmes and Lavkulich Citation2014). In addition, fungi and lichens extracted iron from the fiber surface, which led to a reduction in free radical generation in laboratory experiments (Daghino et al. Citation2005; Favero-Longo et al. Citation2005). Both the surface charge and the generation of free radicals are linked to fiber toxicity and it has therefore been hypothesized that weathering results in less hazardous asbestos (Turci et al. Citation2007; Favero-Longo et al. Citation2009). Nevertheless, coverage of lichens and moss might also have a preventive effect by reducing airborne release (Burdett Citation2007).
In Norway, many buildings with asbestos construction materials are placed along the coast where seawater spray as well as wet and windy conditions contribute to weathering. This work is a case study of asbestos mobilization from an old corrugated asbestos roof. The study is limited to one domestic house in the southern Norway, which had been exposed to weathering for more than 50 years. Run-off water, weathered roof debris from the gutter, moss growing on the roof, and an air sample were collected from and around the house and investigated by scanning electron microscopy (SEM). The chemical composition of fibers in various samples from the roof were obtained to investigate if any chemical changes like magnesium depletion had occurred to weathered asbestos.
Method
By Köppens' classification, the southern coastal part of Norway is classified as Cfb, meaning temperate, without dry season and warm summers (Peel et al. Citation2007). The wooden house built in the 1940s was renovated in the 1960s and was fitted with a corrugated asbestos cement roof and asbestos wall ties.
Sampling
Material samples of roof tiles and moss growing on the roof were randomly collected from the roof of a domestic house in southern-Norway for qualitative determination of asbestos fibers (). After the dry samples were collected, approximately 0.5 L of water was poured on the roof and allowed to run down the length of the roof to simulate rain before it was collected in a plastic bottle. In addition, one ambient air sample was collected for 7 hours. More details regarding the sampling is found under the respective subchapters.
In addition to the various material samples from the roof of the investigated house, material roof samples collected from six different houses in other parts of Norway were investigated to see if the same weathering is found in other corrugated asbestos roofs. This investigation included light microscope and SEM analysis directly on the roof surface.
Determination of asbestos in material samples
Three randomly selected parts of all samples were investigated in a light microscope before qualitative or quantitative analysis in a SU6600 FESEM (Hitachi, Tokyo, Japan) with a Quantax 200 energy dispersive X-ray (EDX) microanalysis system (Bruker - AXS Microanalysis GmbH, Berlin, Germany). The material samples were coated with approximately 10 nm platinum in a Cressington 208HR sputter coater (Cressington Scientific Instruments Ltd., Watford, UK) to minimize charging effects.
In secondary electron (SE) imaging mode, samples were qualitatively investigated for asbestos fibers at a working distance of 10 mm, and acceleration voltage of 15 kV. The asbestos fibers in all samples, except for the moss sample, were analyzed by SEM-EDX.
Material samples—roof, weathered roof debris, and run-off water
The run off-water and weathered roof debris samples were prepared in the same way as the roof sample, except for the very first step in the preparation. In the first step, a small part of the roof sample was ground with a handheld drill. Second, a randomly selected part of the sample was dispersed in water with a low concentration (0.05 M) of hydrochloric acid (HCl) to dissolve calcium carbonate. The samples were then sonicated for one min in a Sonorex Super (Bandelin GmbH, Berlin, Germany). For the run off-water sample, approximately 10 drops of run-off water was pipetted out using a plastic pipette. A polycarbonate (PC) (Isopore, Merck Millipore) filter with pore size 0.8 µm was placed on a sintered glass disk in a Büchner filtration apparatus and water in the sonicated sample was filtered with the help of a vacuum pump. Water was added again before a second filtration to assure a good dispersion of the particulate matter on the filter. Next, an approximately 8 × 8 mm piece of the filter was cut out, fixed on a 10 mm aluminum stub covered with double-sided carbon adhesive disks (Agar Scientific, Ltd.). Spots of carbon cement (Leit-C, Agar Scientific Ltd., Stansted, UK) was added to the sides of the stub to assure good conductivity between filter and stub. Finally, samples were coated with a 10-nm platinum layer before the qualitative analysis with SEM-EDX.
Three randomly selected part of the roof was fixed on a 10-mm aluminum stub covered with double-sided carbon adhesive disks and coated with 10-nm platinum layer before the qualitative analysis with SEM-EDX.
Material samples—moss
Samples (after inspection in the light microscope, ) of roof-growing moss was cut out with a scalpel. The sample was fixed as flat as possible with a tweezer onto a 25-mm aluminum stub covered with double-sided carbon adhesive disks. The samples were coated with a 10-nm platinum layer. This coating was not enough to get rid of charging-effects in SEM, and variable pressure mode (100 Pa) in SEM was therefore applied to the moss sample.
Air sample
At the time of measuring, the house in question was due for asbestos removal and scaffolding with plastic sheeting had been erected at the house. Particulate matter including asbestos fibers were collected within the plastic sheeting on a gold-coated filter for 420 min. An in-house built pump (NIOH, Oslo, Norway) specially made for the purpose of asbestos sampling at the flow of 2 L/min with the cassette was placed in the scaffolding at the height of the roof. After sampling, a piece of about 8 × 8 mm was cut out of the gold-coated filter with a scalpel and fixed to an aluminum stub covered with a carbon tab.
The ISO 14966 method for determination of numerical concentration of inorganic fibrous particles was followed for quantification (ISO14966, 2019). A total of 426 counting fields, at 2000x magnification were investigated for asbestos fibers to investigate 1 mm2 of the filter. Additional 0.25 mm2 was investigated when no fibers were found on the first 1 mm2.
Magnesium depletion
To investigate if fibers from the roof experienced weathering-induced MgO-depletion, fiber bundles from the roof surface, moss and roof underside were investigated. A thin layer of the roof followed the moss when it was collected, and fiber bundles from this layer were picked out with a tweezer for further investigations by SEM-EDX. Exposed and uncovered chrysotile fiber bundles were pulled out the roof surface samples with a tweezer. Asbestos fibers were not visible on the roof underside and a drill was therefore used to collect material from this side. Detached material and fiber bundles were suspended in water and treated in an ultrasonic bath for 1 min. The rest of the preparation follows the preparation steps of all material samples detailed above. In the SEM, the fiber bundles were analyzed with EDX spot analysis where selected spots on the fiber were investigated. Fiber bundles larger than 1 µm in geometric diameter were selected for chemical analysis since thin fibers increase the possibility for excited X-rays from other sources than the fiber itself.
Results
Roof, weathered roof debris, and run-off water
The roof was clearly deteriorated by weathering, and moss was growing on the roof tiles (). In the roof, weathered roof debris and run-off water samples, fibers of chrysotile, amosite and crocidolite were identified. Asbestos fibers were clearly exposed on the surface of the corrugated cement roof (). In comparison, the roof underside had almost no visible fibers (). The other six investigated corrugated asbestos roofs from houses all around Norway showed similar deterioration. However, as weathering is not a homogenous process the deterioration varied across the samples. Small patches of roof paint were sometimes visible on the samples. When the roof paint was intact, few fibers were visible on the surface.
Figure 3. (A) SE image of exposed asbestos fibers on the roof surface. (B) SE image of the underside of the asbestos cement roof.
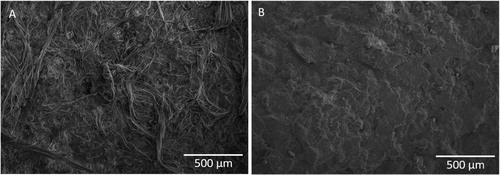
Chrysotile fibers were found in the weathered roof debris sample collected in the roof gutter (). The weathered roof debris sample was also heavily loaded with other particulate matter ().
Figure 4. (A) Overview of the weathered roof debris sample with soil particulates and asbestos fibers. (B) A close-up of an example of chrysotile asbestos fiber in the weathered roof debris sample in (B).
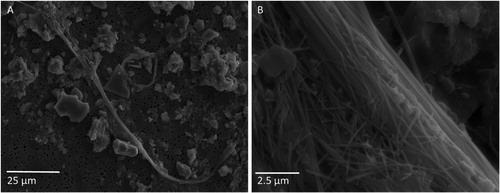
A high number of fibers were found in the sample from the run-off water.
Air sample
No fibers were detected on the 1.25 mm2 investigated in SEM. As no fibers were detected in the air sample, the air concentration is below the detection limit of 0.0012 fibers/cm3.
Magnesium depletion in asbestos fibers
The average chemical composition in oxide weight (wt) %, normalized on anhydrous basis of chrysotile fibers found on the moss, roof-surface and -underside are shown in . Asbestos fibers attached to the moss had slightly lower average MgO concentration than the roof surface fibers. However, the fibers in contact with the moss had a higher wt % of Al2O3 and CaO. SiO2 wt % was slightly higher in the roof underside compared to the moss, and the FeO (FeO total of Fe3+ and Fe2+) were similar in all samples. Magnified images revealed small cement particles attached to the fiber bundles, which influenced the analysis of the fibers. An EDX spectrum from a fiber and from an attached particle is shown in the Supplement ().
Discussion
Asbestos fibers on the outer surface of the corrugated asbestos cement roof were more exposed than on the underside (). Such existing roofs in Norway and many other countries are by now aged, though the extent of weathering depend on location and weather. Investigations of other asbestos roof of this age (∼40–60 years) from Norway showed similar weathering effects. The asbestos fibers on the outer surface were also relatively loose, seen by the high number of fibers evenly distributed on the investigated filter from the run-off water. Also, asbestos fibers were found in the weathered roof debris sample indicating that asbestos fibers were previously mobilized by wind or rainwater. The asbestos fibers are probably more mobile due to weathering of the roof from rain, sea salt spray as well as biological growth like moss.
A high number of asbestos fibers were found in the run-off water sample (). This indicates that the asbestos fibers are easily mobilized. Sampling uncertainties are too large to quantify the asbestos fibers in the weathered roof debris, moss and run-off water samples. These uncertainties include, the sample load - which is very dependent on the total amount of dispersed material, and sample loss (collected in plastic bags/bottles). A smaller uncertainty is linked to the uncertainties in counting fibers (Cossio et al. Citation2018).
Figure 7. Overview image of the water sample to the left (A), and a magnified SEM image of a chrysotile fiber from the run-off water sample to the right (B).
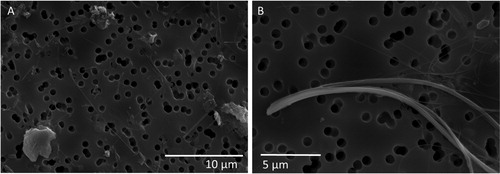
The urban background concentration of fibers has been reported as high as up to 0.0198 fibers/cm3 (Krakowiak et al. Citation2009). These concentrations were measured in areas with a high number of houses built with asbestos containing material. Still, no fibers were detected in the collected air sample, which means that the concentration of fibers in air was low. Krakowiak et al. (Citation2009) reports that a light wind of 1 m/s can carry moveable asbestos fibers up to a few hundred meters. Our samples were collected in a scaffolding covered by plastic sheeting that had been erected at the house for a planned asbestos removal at the house. The plastic sheeting of the scaffolding resulted in protection from wind at the time of the measurements.
EDX information was not obtained from the moss material samples for several reasons. Firstly, the samples were not flat and shading effect might occur. Secondly, asbestos fibers were tangled into the moss and/or laid on top of the moss leaves (). In variable pressure mode, a skirt effect (Danilatos Citation1988), will influence by broadening the energy beam (Goldstein et al. Citation2017). The EDX spectra would therefore be highly influenced by the background. As moss is a known cation exchanger (Gjengedal and Steinnes Citation1990), the background might be variable and a background correction would hence not be applicable. Based only on morphological observations, both chrysotile and amphibole asbestos types were observed.
The slight difference in the average MgO wt % found between investigated fibers was probably biased by the cementitious particles attached to the fiber bundles. As previously described, leaching reactions are reported to occur at discrete sites rather than in uniform layers (Gronow Citation1987) and variations within one fiber might therefore exist. SEM-EDX spot analyses was used because of the challenges related to the heterogeneous field samples, where small cement and other inorganic particles can influence analyses. By spot analyses, such artifacts can be avoided and is therefore limited. Still, this method is restricted by the investigated area of the fibers. Some fibers, or parts of fibers, might be depleted of MgO as the roof has been exposed to rain, seawater and moisture from the moss for several decades. However, given the variability between individual fibers in addition to attached or surrounding particles, no significant MgO depletion was observed. Major element chemistry of the majority of chrysotile fibers released from the roof does not appear to have been affected by weathering. Moss were clearly growing on the roof surface () whereas lichens were not observed. Most of the literature on weathering of minerals consider lichens to be responsible for the surface corrosion (Chen et al. Citation2000) as lichens are known to release significant amounts of oxalic acid (Adeleke et al. Citation2017). A layer of the roof separated and accompanied the collection of the moss samples, which demonstrate the influence of weathering by moss. Removing the moss will potentially mobilize, not only the fibers attached to the moss, but also asbestos fibers left exposed on the surface.
Conclusion
Weathering of asbestos cement roof results in a layer of loosely bound fibers on the surface. Caution is therefore required when washing, working and walking on or removing old roofs as this potentially mobilize high numbers of fibers. Exposed work groups involves chimneysweepers and roofers. Also, when cleaning the gutters, friable material in the gutters might contain asbestos fibers that are not bound in a cement matrix. Even though moss accelerates weathering of the roof, mosses already settled on old roof surfaces can prevent asbestos release. Moss should therefore not be removed as this might increase the number of mobile fibers.
One important aspect to consider when investigating chemical composition of fibers is the possible bias from small particles trapped in between the fibrils. Characterization of fibers found in the moss, on the roof surface and underside did not reveal any signs of MgO depletion. The fibers, prone to release into air, water and weathered roof debris from weathering are therefore considered chemically unchanged.
Supplemental Material
Download PDF (375 KB)Acknowledgments
The authors would like to thank the Regional Safety Representative Foundation for funding, Vidar Larsen and Sture Beiermann (Fellesforbundet), for identifying the house and for contributing to sampling. Jon Hovik helped with sampling and Ole Synnes (STAMI), made the sampling pumps. This work was supported by the Regional Safety Representative Foundation.
The authors declare no conflict of interest relating to the material presented in this article. Its contents, including any opinions and/or conclusions expressed, are solely those of the authors. The study data are available upon request from the corresponding author.
Additional information
Funding
References
- Adeleke R, Nwangburuka C, Oboirien B. 2017. Origins, roles and fate of organic acids in soils: a review. S Afr J. 108:393–406. doi:10.1016/j.sajb.2016.09.002
- Andersen A, Glattre E, Johansen BV. 1993. Incidence of cancer among lighthouse keepers exposed to asbestos in drinking water. Am J Epidemiol. 138(9):682–687. doi:10.1093/oxfordjournals.aje.a116905
- Bales R, Morgan J. 1985. Surface charge and adsorption properties of chrysotile asbestos in natural waters. Environ Sci Technol. 19(12):1213–1219. doi:10.1021/es00142a013
- Bornemann P, Hildebrandt U. 1986. On the problem of environmental pollution by weathering products of asbestos cement. Staub Reinhalt Luft. 46:487–489.
- Burdett G. 2007. Investigation of the chrysotile fibres in an asbestos cement sample. Project: HSL/2007/11. Derbyshire, Buxton: Health & Safety Laboratory.
- Campopiano A, Ramires D, Zakrzewska AM, Ferri R, D’Annibale A, Pizzutelli G. 2009. Risk assessment of the decay of asbestos cement roofs. Ann Occup Hyg. 53(6):627–638. doi:10.1093/annhyg/mep036
- Chen J, Blume H-P, Beyer L. 2000. Weathering of rocks induced by lichen colonization - a review. CATENA. 39(2):121–146. doi:10.1016/S0341-8162(99)00085-5
- Cossio R, Albonico C, Zanella A, Fraterrigo-Garofalo S, Avataneo C, Compagnoni R, Turci F. 2018. Innovative unattended SEM-EDS analysis for asbestos fiber quantification. Talanta. 190:158–166. doi:10.1016/j.talanta.2018.07.083
- Daghino S, Martino E, Fenoglio I, Tomatis M, Perotto S, Fubini B. 2005. Inorganic materials and living organisms: surface modifications and fungal responses to various asbestos forms. Chemistry. 11(19):5611–5618. doi:10.1002/chem.200500046
- Danilatos GD. 1988. Foundations of environmental scanning electron microscopy. Adv Electronics Electron Phys. 71:109–250. doi:10.1016/S0065-2539(08)60902-6.
- Dyczek J. 2006. Surface of asbestos-cement (AC) roof sheets and assessment of the risk of asbestos release. Paper Presented at International Seminar on Asbestos Risk Reduction & Measurement of Asbestos Fibre Concentration, Cracow, Poland.
- Favero-Longo S, Turci F, Tomatis M, Compagnoni R, Piervittori R, Fubini B. 2009. The effect of weathering on ecopersistence, reactivity, and potential toxicity of naturally occurring asbestos and asbestiform minerals. J Toxicol Environ Health A. 72:305–314. doi:10.1080/15287390802529864.
- Favero-Longo SE, Turci F, Tomatis M, Castelli D, Bonfante P, Hochella MF, Piervittori R, Fubini B. 2005. Chrysotile asbestos is progressively converted into a non-fibrous amorphous material by the chelating action of lichen metabolites. J Environ Monit. 7(8):764–766. doi:10.1039/b507569f
- Gjengedal E, Steinnes E. 1990. Uptake of metal ions in moss from artificial precipitation. Environ Monit Assess. 14(1):77–87. doi:10.1007/BF00394359
- Goldstein JI, Newbury DE, Michael JR, Ritchie NW, Scott JHJ, Joy DC. 2017. Scanning electron microscopy and X-ray microanalysis. New York (NY): Springer.
- Gronow JR. 1987. The dissolution of asbestos fibres in water. Clay Miner. 22(1):21–35. doi:10.1180/claymin.1987.022.1.03
- Holmes E, Lavkulich L. 2014. The effects of naturally occurring acids on the surface properties of chrysotile asbestos. J Environ Sci Heal A. 49(12):1445–1452. doi:10.1080/10934529.2014.928558
- HSE. 2012. Asbestos: The survey guide, HSG264. 2nd edition. Norwich (CT): Health and Safety Executive Books.
- Kjaerheim K, Ulvestad B, Martinsen JI, Andersen A. 2005. Cancer of the gastrointestinal tract and exposure to asbestos in drinking water among lighthouse keepers (Norway). Cancer Causes Control. 16(5):593–598. doi:10.1007/s10552-004-7844-1
- Krakowiak E, Górny R, Cembrzynska J, Sakol G, Boissier-Draghi M, Anczyk E. 2009. Environmental exposure to airborne asbestos fibres in a highly urbanized city. Ann Agric Environ Med. 16:121–128.
- Oberta AF, Poye L, Compton SP. 2018. Releasability of asbestos fibers from weathered roof cement. J Occup Environ Hyg. 15(6):466–473. doi:10.1080/15459624.2018.1448401
- Phillips J, Norman G, Renton K. 2009. Asbestos in soil around dwellings in Soweto. Occup Health Southern Africa. 15:24–27.
- Peel MC, Finlayson BL, McMahon TA. 2007. Updated world map of the Köppen-Geiger climate classification. Hydrol Earth Syst Sci. 11(5):1633–1644. doi:10.5194/hess-11-1633-2007
- Spurny KR. 1989. On the release of asbestos fibers from weathered and corroded asbestos cement products. Environ Res. 48(1):100–116. doi:10.1016/S0013-9351(89)80089-1
- Spurny KR. 2000. Aerosol chemical processes in the environment. Boca Raton, FL: CRC Press.
- Turci F, Favero-Longo SE, Tomatis M, Martra G, Castelli D, Piervittori R, Fubini B. 2007. A biomimetic approach to the chemical inactivation of chrysotile fibres by lichen metabolites. Chemistry. 13(14):4081–4093. doi:10.1002/chem.200600991