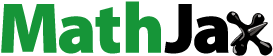
Abstract
A small pilot study was conducted to test whether the technique of in vivo neutron activation analysis could measure bone aluminum levels in 15 miners who had been exposed to McIntyre Powder over 40 years prior. All miners were over 60 years of age, had worked in mines that used McIntyre Powder, and were sufficiently healthy to travel from northern to southern Ontario for the measurements. Individual aluminum levels were found to be significantly greater than zero with 95% confidence (p < 0.05) in 7 out of the 15 miners. The inverse variance weighted mean of the 15 participants was 21.77 ± 2.27µgAl/gCa. This was significantly higher (p < 0.001) than in a group of 15 non-occupationally exposed subjects of a comparable age from Southern Ontario who had been measured in a previous study. The inverse variance weighted mean bone aluminum content in the non-occupationally exposed group was 3.51 ± 0.85µgAl/gCa. Since the use of McIntyre Powder ceased in 1979, these subjects had not been exposed for more than 40 years. Calculations of potential levels at the cessation of exposure in the 1970s, using a biological half-life of aluminum in bone of 10 to 20 years predicted levels of bone aluminum comparable with studies performed in dialysis patients in the 1970s and 1980s. This pilot study has shown that the neutron activation analysis technique can determine differences in bone aluminum between McIntyre Powder exposed and non-exposed populations even though 40 years have passed since exposure ceased. The technique has potential application as a biomarker of exposure in cross-sectional studies of the health consequences of exposure to McIntyre Powder.
Introduction
Inhaled aluminum toxicity
Aluminum is ubiquitous in 21st-century society and is generally treated as innocuous, being commonly used, for example, in cooking utensils. However, there are circumstances in which there are toxic effects on humans (Igbokwe et al. Citation2019). Some early data were obtained from observation of renal patients, who were exposed to high levels of aluminum in dialysate. Neurological effects were observed and the term “dialysis encephalopathy syndrome” (Alfrey et al. Citation1976) was coined emphasizing the neurological degeneration, which could prove fatal. In addition, aluminum accumulation in bone in patients suffering from renal failure was noted in 1971 (Parsons et al. Citation1971) and this was later associated with renal osteodystrophy, particularly osteomalacia (Ott et al. Citation1982). Again, the presumed route of exposure was through dialysate. These issues appear largely to have been resolved by the removal of aluminum from the treatment. (Nebeker and Coburn Citation1986). While it is clear that aluminum directly injected into the bloodstream can be harmful, the effects of aluminum inhalation are less clear. Other routes of exposure to excess aluminum were found in factory workers bagging aluminum silicates for cat litter (Musk et al. Citation1980), aluminum fumes from welding (Elinder et al. Citation1991), and working in aluminum foundries (Sińczuk-Walczak et al. Citation2003) to name a few.
The American Conference of Governmental Industrial Hygienists (ACGIH®) have a threshold limit value (TLV®) set as an 8-hr time-weighted average of 1 mg/m3 for aluminum metal and insoluble compounds measured in respirable particulate matter (ACGIH Citation2008). This TLV is set on the basis of pneumoconiosis, lower respiratory tract irritation, and neurotoxicity (ACGIH Citation2008). The current Ontario Ministry of Labor, Training and Skills Development (MLTSD) occupational exposure limit (OEL) is also 1 mg/m3 (respirable) (Ministry of Labour, Training and School Development Citation2020).
The ACGIH (Citation2008) refers to the McIntyre Powder treatment of miners and the study by Rifat et al. (Citation1990), showing “that the exposed group had an impaired cognitive test function.” The occurrence of central nervous system (CNS) effects has also been reported in welders with relatively high urinary aluminum levels (ACGIH Citation2008) along with a number of other studies. The ACGIH (Citation2008) notes that “prospective studies will be required to better define the exposure threshold for initiation of adverse neurological effects.” Exposures to McIntyre Powder also exceeded the ACGIH excursion criteria resulting in both lung overload (Zarnke et al. Citation2019) and transport via the mucociliary escalator to the gastrointestinal tract providing another route of exposure and pathway for increased body burden. Lung overload on deceased workers identified that after high exposure, even high/short-term exposure, can result in months or even years of ingestion via the mucociliary escalator system (Finkelstein and Dufresne Citation1999).
More recently, a meta-analysis of eight epidemiological studies found that chronic Al exposure was significantly associated with increased risk of AD (OR = 1.71, 95% CI: 1.35–2.18) (Wang et al. Citation2016). Krewski et al. (Citation2007) also indicated that approximately 60% of the body burden is in the bone. Aluminum in bone has a long half-life (likely several years) and is slowly released to the blood (Poddalgoda et al. Citation2021).
The results from this study combined with results of a study by Zarnke et al. (Citation2019), where miners inhaled Al nanoparticles mostly in the form of aluminum hydroxide are useful when trying to understand both the bioavailability and body burden of McIntyre Powder.
Ontario workers and McIntyre powder
In the 1930s, Denny and coworkers (Denny et al. Citation1939) put forward and investigated the hypothesis that exposure to aluminum could help to prevent silicosis. As a result, mines in Northern Ontario and other jurisdictions introduced the practice of miners being required to be in an exposure chamber, into which McIntyre Powder was introduced before, and in some cases after, each shift. The McIntyre Powder was a finely ground mixture of aluminum and aluminum oxide dusts. Workers sat in the chamber for several minutes and were forced to inhale the aluminum particulate. This practice was in place between 1943 and 1979 (Jorgenson and Sandlos Citation2021).
Eventually, the practice was discontinued, because there was no clear evidence of prophylaxis and concerns were raised that the exposure to aluminum might itself be causing harm to the miners (Rifat et al. Citation1990). Although Rifat et al. did not find any clear association between exposure to aluminum powder and specific neurological conditions, they did report a decline in cognitive test scores associated with the exposure. Most recently, Demers et al. (Citation2020) found that “miners who had been exposed to McIntyre Powder had a 34% greater incidence rate of Parkinson’s disease (RR 1.34, CI 1.14–1.57) compared to miners that had never been exposed to McIntyre Powder, based on self report” (Demers et al. Citation2020, executive summary, page ii, lines 15–17).
Aim of the study
The aim of this investigation was to conduct a small pilot study to determine whether in vivo neutron activation analysis (NAA) could be used to assess aluminum body burden in 15 Ontario workers exposed to McIntyre Powder between 1943 and 1979. If the technique can indeed measure Al in these workers, it would have future possible application in cross-sectional studies that aim accurately to relate potential health consequences of exposure to aluminum dust to particular levels of long exposure. A pilot study was deemed necessary because it was not known if the exposure levels were either high enough to be distinguishable from non-McIntyre Powder exposed men, or whether any uptake into the skeleton would have been retained over a 40–60-year period.
Typically, "biological monitoring of exposure" has traditionally utilized assays of aluminum in urine or blood. Urine and blood samples do not necessarily tell the whole picture since, as stated earlier, the body burden of aluminum from longer-term exposure results from aluminum accumulating in bone. Bone biopsies are both invasive and impractical. Hence, the method outlined in this study provides a practical noninvasive method which will be invaluable for future follow-up studies.
Materials and methods
Participants
The Occupational Health Clinics for Ontario Workers (OHCOW) approached miners whose information had been collected in a voluntary database assembled by J. Martell and coworkers. Candidates for inclusion in this pilot study met the following criteria:
have been exposed to McIntyre Powder through their work in mines; and
be sufficiently healthy to be able to travel from northern Ontario, where most of them currently live, to Hamilton in southern Ontario, where McMaster University is situated.
All the participants were male and older than 60 years; the last exposure to McIntyre Powder had been in 1979, 40 years before these measurements. The attempt was made to select participants with a variety of exposure histories as presumed from their work locations and length of employment. However, there were constraints imposed by the long times since exposure and the current fitness of potential participants. Fifteen people chose to participate. The study had been submitted to the Hamilton Integrated Research Ethics Board and granted approval for 2019–2020 (project #7112). Participants were informed of the procedure to be followed before leaving northern Ontario and each gave consent before traveling. On arrival at McMaster and having been shown the NAA measurement facility, each subject was also given the opportunity to withdraw. Miners came to McMaster in groups of two to five, along with the investigators from OHCOW who were accompanying them, between October and December 2019.
In vivo neutron activation analysis
In vivo neutron activation analysis is a method of measuring the amount of an element in a living subject. The person, or a part of their body, is exposed to neutrons, which induce nuclear reactions. The most frequently used of these involves absorption of a neutron by an isotope, resulting in a new, radioactive isotope, which decays, resulting in the emission of one or more gamma-rays, which can be measured external to the body. Two examples are 48Ca(n,γ)49Ca and 27Al(n,γ)28Al. 28Al releases one gamma ray per decay at 1.78 MeV, and 49Ca releases two gamma rays per decay, at 3.08 MeV and 4.0 MeV, respectively. The measurement has been used for several decades in research studies having been developed as a noninvasive measurement of aluminum in response to concerns over dialysis encephalopathy (Williams et al. Citation1980). Further information can be found in an extensive review of the use of in vivo neutron activation analysis for measurement of aluminum published by Mohseni and Chettle (Citation2016). The particular facility for in vivo neutron activation analysis at McMaster was developed around the Tandetron accelerator. Studies of various elements have been undertaken within this facility over the last two decades, including a pilot study, comparing the level of bone aluminum in people suffering from Alzheimer’s disease to control subjects (Mohseni et al. Citation2016).
Bone aluminum measurement
The bone measurement protocol was identical to that used previously in the study of Alzheimer’s patients (Mohseni et al. Citation2016). Participants were positioned so that their hand was in an irradiation cavity. Their arm was placed in a water sleeve and the sleeve was filled with water until the participant reported that the fit was snug. This was to reduce radiation, particularly neutrons streaming down the hand access aperture, thus limiting the exposure to radiosensitive tissues and organs in the participant’s torso. Neutrons were produced by the 7Li(p,n)7Be reaction. Protons of energy 2.3 MeV were directed onto a thick lithium target at a current of 400 µA for 45 s. This had previously been determined to result in an effective dose of 210µSv for the participant. For comparison, a chest x-ray normally results in an effective dose of 100µSv (Radiologyinfo.org Citation2019). As well as the time of the irradiation, the integrated current and the number of counts from a neutron monitor located in the irradiation cavity were recorded. The integrated current and neutron counts were consistent within normal statistical variation across the measurements. Each participant wore an alarming personal dose monitor as did the attendant standing with the participant. All the recorded doses were reported to Health Physics. Participants then transferred to the nearby counting facility which is an array of 9 NaI(Tl) crystals configured in a near 4π geometry (Byun et al. Citation2004). The average transfer time was 57.3 s. A series of 10 one-minute counts was performed. Primary analysis was undertaken of the first three one-minute counting cycles, by which time, the activity of the 28Al had declined to 40% of its level at the start of the counting procedure.
Two in vivo measurements did not follow the normal protocol. During measurements of the third participant, an equipment malfunction occurred, resulting in only the first 60-s cycle being recorded. The fourth participant had the unirradiated hand initially placed into the cavity of the 4π detector, this was noticed after 3 min, at which point the irradiated hand was placed in the cavity. To correct for this, the next three cycles after the first 3 min were used to calculate the concentration of aluminum.
Data analysis
Aluminum concentrations were calculated by fitting a mathematical model to the spectrum and extracting the aluminum signal from a background. Specifically, a Levenberg-Marquardt least squares fitting algorithm was employed to fit the combined aluminum/chlorine (Al/Cl) peaks and the separate calcium (Ca) peak. Goodness of fit was evaluated using the Pearson Chi-square test statistic. The algorithm modeled the signal as a Gaussian function on a linear background. The signal (in counts) for the Al γ-ray peak was thus calculated by multiplying the mathematically determined amplitude of the Al peak by the determined width and a factor of The same procedure was repeated with the Ca peak. The fitting algorithm produces a one sigma (standard deviation) estimate of the uncertainty of each parameter estimated. From these, the uncertainty in the area of, for example, aluminum counts is calculated. Similarly, the uncertainty in the Al to Ca ratio is derived and hence the uncertainty in the quoted result, shown in .
Table 1. Estimates of bone aluminum level for the 15 participants.
There are several negative values in the results, which can be confusing as they are not physically possible. They result from the data extraction procedure which fits a function to the gamma-ray spectrum. This fits the Al signal and a background simultaneously. The background intensity is determined from points on either side of the Al signal. Both signal and background are subject to fluctuation. The outcome can be an apparent negative value, especially when the signal is small in relation to the background fluctuation. McNeill et al. (Citation1999) noted this issue in the context of in vivo X-ray fluorescence of lead in bone. Hasan et al. (Citation2020) insisted on including these apparent negative results in assessing a group of measurements in their study using in vivo neutron activation to measure aluminum in bone. Any result in which the estimate of the Al signal is not greater than or equal to twice the uncertainty in that fitted signal is reported as “not detected”. That is, only results where there is >95% confidence that the signal is greater than zero are reported as a value to the participant.
Analyses were performed of individual values and of the group. Mean and inverse variance weighted mean data are shown in . Inverse variance weighted means are calculated because the uncertainty in the measurement varies from participant to participant. An inverse variance weighted mean placed more emphasis on estimates with better precision.
This signal could be converted to units of aluminum concentration by relating the signal to a calibration line. The system was calibrated by using standard addition phantoms containing constant concentrations of Cl, Mg, Na, and Ca as well as varying amounts of Al (0 to 50,000 μg). These phantoms were measured by being placed in the irradiation cavity of the Tandetron and were subject to the same parameters as the participants.
In order to account for any discrepancies in the fluence of the neutron beam, as well as the varying amount of calcium in each participant, per Mohseni et al., accounts for this by normalizing the data to the 3.08 MeV calcium peak.
A challenge in in vivo measurements of aluminum is that there is an overlapping signal from chlorine. Since the Al and Cl peaks overlapped, the data were fit therefore as a double Gaussian on a linear background, with position and width of the 28Al peak being fixed. These values were calculated by taking the average values of several phantom concentrations:
(1)
(1)
Since the Ca peak was fully resolved from any surrounding peaks, it was only fit with a single Gaussian on a linear background.
The calibration line was created using the same method as described in Mohseni et al. (Citation2016), except Al concentrations went up to 50,000 μg Al instead of 5,000 μg Al. The areas of the Al and Ca peaks were calculated, and the ratio of the two areas was computed. After this the ratio of areas was plotted against the actual ratio of Al to Ca in the phantom to create a calibration curve. This curve was then fit with a linear function to find the slope. This slope value was used for the in vivo data to convert the signal from the number of counts to μgAl/gCa.
Results
Bone aluminum
The results of the bone aluminum measurements are shown in , along with years of exposure.
The highest value among these 15 participants, 206.36 ± 14.76µgAl/gCa (mean ± standard deviation) was considerably higher than the next highest value of 67.88 ± 9.67 µgAl/gCa. The mean and inverse variance mean were also calculated excluding this value, resulting in a mean of 3.93 ± 34.92 µgAl/gCa and an inverse variance weighted mean of 17.30 ± 2.30 µgAl/gCa. There was no correlation between bone aluminum and years of exposure (n = 14, r = 0.443, t = 1.712; n = 15, r = −0.087, t = −0.316).
Discussion
These bone aluminum results can be compared to those from the control group of 15 subjects measured for comparison with Alzheimer’s subjects in the study previously conducted in our NAA facility (Mohseni et al. Citation2016). The control group were of a similar age to participants in this study. The inverse variance weighted mean of this control group was 3.51 ± 0.85 µgAl/gCa, and the highest individual value was 15.0 ± 4.86 µgAl/gCa. Even when the highest individual value was excluded from the McIntyre Powder exposed group, the inverse variance weighted mean was still significantly higher than the earlier control group inverse variance weighted mean (17.30 ± 2.30 vs. 3.51 ± 0.85 µgAl/gCa, p < 0.001). shows the individual data from the Mohseni study, both the control subjects and the cases. The inverse variance weighted mean of the Alzheimer’s Disease subjects was 12.35 ± 0.97 µgAl/gCa, with high levels of 37.4 ± 5.35 µgAl/gCa and 31.6 ± 6.11 µgAl/gCa. There is overlap between the Alzheimer’s Disease subjects and these retired miners, but the miners had higher bone Al levels, based on the inverse variance weighted means.
Table 2. Estimates of bone aluminum level from Mohseni et al. (Citation2016).
A comparison can also be made with an extensive set of bone aluminum measurements made in biopsy samples by ICP-MS (Hellström et al. Citation2005). These data are presented in a graph of log(ngAl/gdry bone) vs. age. We estimated from the article that the geometric mean of the 54 subjects aged between 60 and 80 was 3.1 µgAl/gCa and the highest value was 16 µgAl/gCa. The geometric standard deviation was ±2.2, so this means that the 68% confidence range was from 1.4 to 7.0 µgAl/gCa. This quite extensive and different data set returns a value for normal bone aluminum which is very close to that of the small control group measured at McMaster University. The control subjects whose bone aluminum had previously been measured by in vivo neutron activation analysis were from southern Ontario, whereas the miners were from northern Ontario. It is possible that the difference in aluminum levels relates to different environmental levels of aluminum, but this seems unlikely, especially given the close agreement between the control levels in Southern Ontario and those measured by Hellström et al. in Sweden.
The use of McIntyre powder ceased in 1979. So, the last exposure for these miners was in 1979 and for some it had ceased before then. A history of all occupational exposures can be found in the supplementary data; no other exposures to high levels of aluminum are known. Data on aluminum levels in water were not tracked, other Al exposures could have been possible, but none are known. The biological half-life for aluminum in bone has been estimated to be between 10 and 20 years (Priest Citation2004). Priest’s study was based on 26Al tracer experiments and should be seen as reliable. However, it is only one study and it does give a rather wide range in estimates of half-life. Hasan et al. (Citation2020) show their strongest relationship between bone aluminum and a cumulative exposure index calculated over 15 years, which doesn’t conflict with Priest’s data, but cannot be regarded as strong confirmation. If Priest’s data are used then this means that the bone aluminum can be estimated to have been between 4 times (two half-lives) and 16 times (four half-lives) higher when exposure ceased than when it was measured in 2019. Such extrapolations should be treated with caution, both because they are quite extreme extrapolations and because there is a paucity of data on the half-life of aluminum in human bone.
The data from the McIntyre Powder-exposed participants can also be compared to earlier data from people undergoing dialysis in the 1980s, where the highest level was 760 µgAl/gCa (Ellis et al. Citation1988). The mean of 10 subjects was 209 ± 253 µgAl/gCa. They can also be compared to a recent study of occupationally exposed subjects, where the highest level was 260 µgAl/gCa (Hasan et al. Citation2020). The mean of Hasan et al.’s 43 subjects was 62.37 ± 74.37 µgAl/gCa. Hasan et al. quote their results in µgAl/g dry bone. However, they quote their detection limit as 11.13 µgAl/g dry bone, which they state is equivalent to 44.5 µgAl/gCa, so we have used this equivalence to convert their results. gives summary data for the Ellis et al. and Hasan et al. studies along with the present study and our earlier Alzheimer’s Disease study. also gives detection limits. In our studies, the detection limit is two times the median uncertainty in the set of data, which is why it is higher in this study compared to our earlier study, in part because of the anomalous data collection problems noted above. We do not know whether Ellis et al. and Hasan et al. estimated their detection limits in the same way. Allowing for biological elimination with a half-life of 10 to 20 years, these data are roughly comparable with, or higher, than those from the dialysis study and higher than the contemporary occupationally exposed subjects.
Table 3. Summary data from the present study, compared to some previous studies.
A recent cohort study by Demers et al. investigated the association of McIntyre exposure with neurodegenerative diseases. Data were pulled from the Mining Master File (MMF), an electronic database recording medical records and work history from 90,000 miners across Ontario. During the period of McIntyre powder use, 28% of all underground miners were exposed, with the peak being 24.5% of all miners in 1961.
Of the 90,000 records, 36,994 files were included based on matching to available health samples. Of these 168 were female, and the remaining 36,826 were male, with 9,548 (25.9%) of the workers being exposed to McIntyre powder sometime in their career. Neurological disease follow-up period was from January 1992 to December 2018. Workers who were deceased, or lost contact were excluded, as were workers aged 100 years or over.
Of these exposed miners, 90% were exposed after 1956. No association was found between exposure to McIntyre Powder and Alzheimer’s disease (rates were similar or less than that of unexposed miners), however when looking at the rate of Parkinson’s and parkinsonism, there was a 27% and 14% increase in incidence rates of both compared to the general population. When compared with miners that had never been exposed to McIntyre Powder, there was a 34% and 19% increase in incidence of both.
Though the study did not have access to the entire medical history of the individual miners, the higher rates of both Parkinson’s and parkinsonism suggest that the use of McIntyre powder may be linked to an increase in Parkinson’s and other diseases like it.
There are a number of limitations to the current study. There were only 15 study subjects. It should very much be considered a pilot, rather than a substantive study. Furthermore, there were problems with data collection for two of the subjects, as noted above. The detection limit for bone aluminum was not as good as in our earlier study, although still comparable with other in vivo bone aluminum data. Another limitation is that exposure data gives only the number of years exposed and the date range in which that exposure occurred. Exposure practices were not uniform, and some people would have had jobs that involved explicit aluminum exposure in addition to the pre-shift and in some cases post-shift exposure. Also, there was no direct comparison group for these former miners. The control group measured a few years earlier provides a reasonable comparison, but it is not as compelling as a group of similarly aged subjects currently living in northern Ontario would have been.
Conclusions
This pilot study was able to demonstrate that aluminum can be measured in the bones of miners exposed to McIntyre Powder over 40 years ago. This technique could potentially be applied in further cross-sectional studies of health effects in this group (or similar groups) of workers.
There was significant accumulation of aluminum in bone of these miners, with the maximum concentration measured being 206.36 ± 14.76 µgAl/gCa. The inverse variance weighted mean level of bone aluminum was 21.77 ± 2.27 µgAl/gCa, which was significantly higher than the inverse variance weighted bone aluminum level observed in a similar age control group of volunteers living in southern Ontario in our laboratory in a previous study. The increased bone aluminum was detectable in about half the subjects measured even 40 years after the exposure to McIntyre Powder had ceased. With adjustments for biological removal of aluminum from bone over time, the maximum concentration detected is in line with values reported from a previous study measuring Al levels in dialysis patients, suggesting similar initial exposure levels.
Supplemental Material
Download MS Word (56 KB)Acknowledgments
We would like to acknowledge the tremendous support provided by Justin Bennett in managing, reconstructing, maintaining, and running the Tandetron accelerator. Without his efforts these measurements could not have taken place.
Data availability statement
The authors confirm that the data supporting the findings of this study are available within the article and its supplementary materials.
Conflict of interest
J. M. was instrumental in assembling the database of miners and is viewed by the participants as advocating for their interests and wellbeing, so she recused herself from determining the selection of participants and analysis of data. None of the other investigators has a conflict of interest to declare.
Additional information
Funding
References
- Alfrey AC, LeGendre GR, Kaehny WD. 1976. The dialysis encephalopathy syndrome – possible aluminum intoxication. N Engl J Med. 294(4):184–188. doi:10.1056/NEJM197601222940402
- American Conference of Governmental Industrial Hygienists (ACGIH). 2008. Documentation of the Threshold Limit Values. Aluminum metal and insoluble compounds.
- Byun SH, Prestwich WV, Chin K, McNeill FE, Chettle DR. 2004. Efficiency calibration and coincidence summing correction for a 4pi NaI(Tl) detector array. Nucl Instrum Meth. 535(3):674–685. doi:10.1016/S0168-9002(04)01469-X
- Demers PA, Arrandale VH, DeBono N, Berriault C, MacLeod J, Zeng X, Harris A. 2020. Investigation of McIntyre powder exposure and neurological outcomes in the mining master file cohort: final report. OCRC Document submitted to the Workplace Safety and Insurance Bureau of Ontario, 12th March.
- Denny JJ, Robson WD, Irwin DA. 1939. The prevention of silicosis by metallic aluminum II. Can Med Assoc J. 40(3):213–228.
- Elinder CG, Ahrengart L, Lidums V, Pettersson E, Sjogren B. 1991. Evidence of aluminium accumulation in aluminium welders. Br J Ind Med. 48(11):735–738. doi:10.1136/oem.48.11.735
- Ellis K, Kelleher S, Raciti A, Savory J, Wills M. 1988. In vivo monitoring of skeletal aluminum burden in patients with renal failure. J Radioanal Nucl Chem. 124(1):85–95. doi:10.1007/BF02035508
- Finkelstein MM, Dufresne A. 1999. Inferences on the kinetics of asbestos deposition and clearance among chrysotile miners and millers. Am J Ind Med. 35(4):401–412. doi:10.1002/(SICI)1097-0274(199904)35:4<401::AID-AJIM12>3.0.CO;2-4
- Hasan Z, Rolle-McFarland D, Liu Y, Zhou J, Mostafaei F, Li Y, Fan Q, Zhou Y, Zheng W, Nie LH, et al. 2020. Characterization of bone aluminum, a potential biomarker of cumulative exposure, within an occupational population from Zunyi, China. J Trace Elem Med Biol. 59:126469. doi:10.1016/j.jtemb.2020.126469
- Hellström HO, Mjöberg B, Mallmin H, Michaëlsson K. 2005. The aluminum content of bone increases with age, but is not higher in hip fracture cases with and without dementia compared to controls. Osteoporos Int. 16(12):1982–1988. doi:10.1007/s00198-005-1981-6
- Igbokwe IO, Igwenagu E, Igbokwe NA. 2019. Aluminium toxicosis: a review of toxic actions and effects. Interdiscip Toxicol. 12(2):45–70. doi:10.2478/intox-2019-0007
- Jorgenson M, Sandlos J. 2021. Dust versus dust: aluminum therapy and silicosis in the Canadian and global mining industries. Can Hist Rev. 102(1):1–26. doi:10.3138/chr-2019-0049
- Krewski D, Yokel RA, Nieboer E, Borchelt D, Cohen J, Harry J, Kacew S, Lindsay J, Mahfouz AM, Rondeau V. 2007. Human health risk assessment for aluminium, aluminium oxide and aluminium hydroxide. J Toxicol Environ Health B Crit Rev. 10(sup1):1–269. doi:10.1080/10937400701597766
- McNeill FE, Stokes L, Chettle DR, Kaye WE. 1999. Factors affecting in vivo measurement precision and accuracy of 109Cd K X-ray fluorescence measurements. Phys Med Biol. 44(9):2263–2273. doi:10.1088/0031-9155/44/9/313
- Ministry of Labour, Training and Skill Development. 2020. Current occupational exposure limit for Ontario workplaces required under regulation 833; [accessed 2020 Jul 5]. https://www.labour.gov.on.ca/english/hs/pubs/oel_table.php.
- Mohseni HK, Chettle DR. 2016. A history of in vivo neutron activation analysis in measurement of aluminum in human subjects. J Alzheimers Dis. 50(4):913–926. doi:10.3233/JAD-150595
- Mohseni HK, Cowan D, Chettle DR, Milic AP, Priest N, Matysiak W, Atanackovic J, Byun SH, Prestwich WV. 2016. A pilot study measuring aluminum in bone in Alzheimer's disease and control subjects using in vivo neutron activation analysis. JAD. 53(3):933–942. doi:10.3233/JAD-160194
- Musk AW, Greville HW, Tribe AE. 1980. Pulmonary disease from occupational exposure to an artificial aluminium silicate used for cat litter. Br J Ind Med. 37(4):367–372. doi:10.1136/oem.37.4.367
- Nebeker HG, Coburn JW. 1986. Aluminum and renal osteodystrophy. Annu Rev Med. 37:79–95. doi:10.1146/annurev.me.37.020186.000455
- Ott SM, Maloney NA, Coburn JW, Alfrey AC, Sherrard DJ. 1982. The prevalence of bone aluminum deposition in renal osteodystrophy and its relation to the response to calcitriol therapy. N Engl J Med. 307(12):709–713. doi:10.1056/NEJM198209163071202
- Parsons V, Davies C, Goode C, Ogg C, Siddiqui J. 1971. Aluminium in bone for patients with renal failure. Br Med J. 4:272–275.
- Poddalgoda D, Hays SM, Kirman C, Chander N, Nong A. 2021. Derivation of biomonitoring equivalents for aluminium for the interpretation of population-level biomonitoring data. Regul Toxicol Pharmacol. 122(2021):104913. doi:10.1016/j.yrtph.2021.104913
- Priest ND. 2004. The biological behaviour and bioavailability of aluminium in man, with special reference to studies employing aluminium-26 as a tracer: review and study update. J Environ Monit. 6(5):375–403. doi:10.1039/b314329p
- Radiologyinfo.org. 2019. Radiation doses in X-ray and CT exams; [accessed 2020 Aug 10]. https://www.radiologyinfo.org/en/pdf/safety-xray.pdf.
- Rifat SL, Eastwood MR, McLachlan DRC, Corey PN. 1990. Effect of exposure to miners to aluminum powder. Lancet. 336(8724):1162–1165. doi:10.1016/0140-6736(90)92775-D
- Sińczuk-Walczak H, Szymczak M, Raźniewska G, Matczak W, Szymczak W. 2003. Effects of occupational exposure to aluminum on nervous system: clinical and electroencephalographic findings. Int J Occup Med Environ Health. 16(4):301–310.
- Wang Z, Xiamon W, Yaang J, Suo J, Jinning S, Jingy C, Xianchen L, Zhao X. 2016. Chronic exposure to aluminium and risk of Alzheimer's disease: a meta-analysis. Neurosci Lett. 610(2016):200–206.
- Williams ED, Elliott HL, Boddy K, Haywood JK, Henderson IS, Harvey I, Kennedy AC. 1980. Whole-body aluminum in chronic-renal-failure and dialysis encephalopathy. Clin Nephrol. 14(4):198–200.
- Zarnke A, Rasmussen PE, Marie-Odile D, Housam E, Kennedy K, Hedges K, Irick T, Thome C, Pirkkanen J, Boreham D. 2019. Physical and chemical characterization of McIntyre powder: an aluminum dust inhaled by miners to combat silicosis. J Occup Environ Hyg. 16(11):745–756. doi:10.1080/15459624.2019.1657581