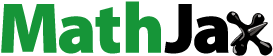
Abstract
In emergencies like the COVID-19 pandemic, the reuse or reprocessing of filtering facepiece respirators (FFRs) may be required to mitigate exposure risk. Research gap: Only a few studies evaluated decontamination effectiveness against SARS-CoV-2 that are practical for low-resource settings. This study aimed to determine the effectiveness of a relatively inexpensive ultraviolet germicidal irradiation chamber to decontaminate FFRs contaminated with SARS-CoV-2. A custom-designed UVGI chamber was constructed to determine the ability to decontaminate seven FFR models including N95s, KN95, and FFP2s inoculated with SARS-CoV-2. Vflex was excluded due to design folds/pleats and UVGI shadowing inside the chamber. Structural and functional integrity tolerated by each FFR model on repeated decontamination cycles was assessed. Twenty-seven participants were fit-tested over 30 cycles for each model and passed if the fit factor was ≥100. Of the FFR models included for testing, only the KN95 model failed filtration. The 3M™ 3M 1860 and Halyard™ duckbill 46727 (formerly Kimberly Clark) models performed better on fit testing than other models for both pre-and-post decontaminations. Fewer participants (0.3 and 0.7%, respectively) passed fit testing for Makrite 9500 N95 and Greenline 5200 FFP2 and only two for the KN95 model post decontamination. Fit testing appeared to be more affected by donning & doffing, as some passed with adjustment and repeat fit testing. A ≥ 3 log reduction of SARS-CoV-2 was achieved for worn-in FFRs namely Greenline 5200 FFP2. Conclusion: The study showed that not all FFRs tested could withstand 30 cycles of UVGI decontamination without diminishing filtration efficiency or facial fit. In addition, SARS-CoV-2 log reduction varied across the FFRs, implying that the decontamination efficacy largely depends on the decontamination protocol and selection of FFRs. We demonstrated the effectiveness of a low-cost and scalable decontamination method for SARS-CoV-2 and the effect on fit testing using people instead of manikins. It is recognized that extensive experimental evidence for the reuse of decontaminated FFRs is lacking, and thus this study would be relevant and of interest in crisis-capacity settings, particularly in low-resource facilities.
Introduction
The COVID-19 pandemic posed a severe threat to public health, occupational health, and local economies worldwide (Cassorla Citation2021), overwhelming healthcare systems and the stock of filtering facepiece respirators (FFRs) (Rowan and Laffey Citation2020). Despite being the last alternative in the hierarchy of controls, wearing appropriate and well-fitted FFRs is still one of the most effective preventative measures, particularly in poorly controlled areas and in settings where exposure is unknown or unpredictable (Sehgal and Milton Citation2021). However, the shortage of FFRs and the use thereof for periods beyond that recommended may contribute to anxiety and the high incidence of COVID-19 infections among health workers and other end users (De Kock et al. Citation2021; Ruskin et al. Citation2021).
With the possibility of future COVID-19 waves, among other airborne biological threats, the need for an adequate stock of quality FFRs to reduce the risk of exposure is of paramount importance and life-saving (Rashid et al. Citation2022). The Centers for Disease Control and Prevention (CDC) recognized the need to optimize the supply of N95 respirators and recommended contingency capacity strategies which include the reuse and extended use of N95 respirators (CDC Citation2021). Accordingly, healthcare workers could rotate FFRs over five days between each FFR use, providing time for pathogens to “die off” during storage (3M Science Citation2020). However, aside from pathogens with prolonged inanimate surface survival, the SARS-CoV-2 virus can survive on a mask for up to seven days (Cordoba-Lanus et al. Citation2021), implying that extended use in the absence of decontamination may pose an infectious risk to wearers (Schwartz et al. Citation2020; van Doremalen et al. Citation2020). Thus, the need for effective solutions prompted an international plethora of innovations in decontaminating FFRs for reuse in resource-limited contexts (Byrne et al. Citation2020; Rothe et al. Citation2020; Cassorla Citation2021; Cordoba-Lanus et al. Citation2021).
While SARS-CoV-2 has a global impact, people living in low- and middle-income countries (LMIC) were particularly impacted by COVID-19 (Bong et al. Citation2020). The impact transcended certain disposable respirators’ availability, leaving many nations that lacked manufacturing capacity in dire straits (Vanhooydonck et al. Citation2021). Local manufacturing was hampered by staggering costs and a lack of access to core materials used in product processing. Based on the unprecedented crisis and FFR shortages, decontamination and reuse as a crisis capacity strategy ensure continued availability for frontline workers in low-resource settings.
Decontamination or reprocessing of FFRs
Decontamination or reprocessing of single-use FFRs was accepted in 2020 as a crisis strategy to conserve available supplies (CDC Citation2020). Limited decontamination and reuse of FFR are advised for NIOSH-approved respirators only (CDC Citation2020). The CDC’s recommendations to conserve FFR supplies include extended use (longer wearing time and/or use with multiple patients), prioritizing use for those at the highest risk, and limited reuse (CDC Citation2020). This manuscript includes some preprint reports that have not yet benefited from a peer-review process.
Ultraviolet germicidal irradiation (UVGI) was selected as a decontamination method in the current study as it has been reported as one of the better decontamination methods available (Schumm et al. Citation2021; van der Vossen et al. Citation2022). Schumm et al. (Citation2021) conducted a systematic review of FFR reprocessing and reported 27 studies that evaluated UVGI. Several N95 respirators (n = 43) were treated with UVGI at doses between 1 and 2 J/cm2 and effectively decontaminated most pathogens (>3 log reduction), including influenza virus, MS2 bacteriophage, Bacillus spores, vesicular stomatitis virus, Middle East Respiratory Syndrome (MERS) virus, and SARS-CoV-2 without degrading respirator components.
The included studies had several limitations. Many were experimental laboratory-based studies except for two studies that examined clinical use. Only five studies directly evaluated SARS-CoV-2, whereas other studies inferred from other viral and bacterial surrogates Rothe et al. (Citation2020) highlighted that although a large volume of data on mask disinfection and reuse existed, much was not comparable as many studies lacked specificity on methods and tested a wide range of conditions on a small number of samples.
Considering there are over 530 NIOSH-certified FFRs, the results from previous studies cannot be generalized to all FFRs models, rather, specific FFR testing should be considered the FFR model (e.g., shape, material), decontamination method, and filtration performance, facial fit, and residual toxins (Wharton and Rieker Citation2020). In addition, independent confirmation of reused FFR safety and fit is warranted as most studies used manikins rather than FFR worn by humans (Allen et al. Citation2020; Arnett Citation2020). Of the few studies that assessed user fit, one reported good quantitative fit and filter function of one FFR after ten cycles of 216 mJ/cm2 to each side, an atypically low dose (Ou et al. Citation2020). Contrary, another study reported that all six samples of three models failed filter and human fit tests after 10 UVGI cycles without a reportedosesse (Rutala et al. Citation2019).
The UV-C intensity is critical to the method’s success at disinfection, as an insufficient dose will not inactivate infectious material (Boskoski et al. Citation2020; Liao et al. Citation2020). While the dose used in previous studies varied from 2 to 5 J/cm2 (Mills et al. Citation2018; Fischer et al. Citation2020), the recommended dose (1 J/cm2) reported by Heimbuch and Harnish (Citation2019) was 100 times higher than that required for hard surfaces (Grossman et al. Citation2020). Unlike solid surfaces, respirator material requires higher UVGI doses for decontamination because it needs to penetrate the material layer/s. The spatial distribution of UV-C intensity is not uniform and varies significantly with distance from the light source, which must be in the direct path of a pathogen to inactivate it; hence, multiple light sources or separate cycles for each side may be required (Heimbuch et al. Citation2011; Mills et al. Citation2018; Heimbuch and Harnish Citation2019; Jacobs et al. Citation2020).
Concerns regarding varied FFR styles, the potential for attenuation, shadowing, and strap damage have been raised (Tseng and Li Citation2007). FFR model differences as well as varying treatment doses cause significant variations in UVGI efficacy (Lindsley et al. Citation2015; Mills et al. Citation2018; Heimbuch and Harnish Citation2019; Fischer et al. Citation2020; Liao et al. Citation2020; Syphers Citation2020; Weaver et al. Citation2020). Therefore, it would be useful to categorize model types based on shape or determine how findings for a specific model can be generalized so that each model does not need to be evaluated (Derraik et al. Citation2020). It is thus essential to determine how many UVGI decontamination cycles an FFR model can sustain without compromising structural and functional integrity and effectively inactivating the target organism/s within the local context and based on the available resources. Considering the above, this study aimed to investigate a low-cost UVGI chamber for decontaminating FFRs contaminated with SARS-CoV-2. The study also evaluated the performance criteria (facial fit and filtration efficiency), structural integrity, odor, and user safety.
Methods
This experimental design evaluated the impact of 30 repeat decontamination cycles on seven FFR models and the functional performance using a low-cost UVGI chamber. Duplicate FFRs were tested before and after each decontamination cycle to a maximum of 30 cycles. One batch was used for fit testing and the second was for filtration testing. Twenty-seven consenting participants were included in the study, 7 were male and 20 females, of which 18 were Black Africans, 5 Asians/Indians, and 4 were White hence accounting for various facial dimensions. A batch of new FFRs without viral contamination was worn by the participants for the duration of fit testing and repeatedly until the 30th fit test cycle unless fit testing failed before the 30th cycle, and were coined “used” or “worn-in.” Post decontamination, the FFRs were visually and tactilely assessed for structural integrity and underwent quantitative facial fit testing to assess functional integrity.
Performance tests also included filtration efficiency testing and SARS-CoV-2 inactivation by UVGI. Ethical clearance for the study was acquired from the REDACTED.
Filtering facepiece respirator selection
Seven FFR models available to frontline and essential workers in South Africa at the beginning of the pandemic were included in this study, of which four models were N95s, two were FFP2s, and one was a KN95 (). The FFRs consisted of electrostatically charged polypropylene filters (electret filter media). All FFRs were verified from the same respective manufacturing batches/lots at the beginning of the study to reduce any batch-to-batch variation during testing.
Table 1. Specifications of the filtering facepiece respirators tested.
Decontamination method
A custom UVGI chamber was developed specifically for FFR decontamination applications to be low cost, simple to operate, safe, and use readily available components. The primary design principle is based on a drawer/rail mechanism that is either in an “OPEN” or “CLOSED” position, as shown schematically and physically in . To reduce the potential idle time of the process, FFRs are positioned onto a removable carrier frame to enable the FFR positioning procedure to occur simultaneously during a batch decontamination cycle. Predetermined positions were established for each FFR model, where the straps of the FFRs were carefully positioned onto locating rods of the carrier frame to open the respirators, avoiding shadows and contact with other respirators from hanging straps while preserving the structural integrity (). The chamber could accommodate different FFR model types classified as a “cup shape” (3M-1860-N95; 3M 8810SSA-FFP2; Makrite 9500-N95) or “fold shape” (duckbill 46727-N95 with a horizontal fold, or the KN95 CE 2703-N95 and Greenline 5200-FFP2 with vertical folds). However, FFRs made with pleated material such as the 3M V-flex 9105S-N95 were not considered suitable due to anticipated self-shadowing effects and were excluded from subsequent testing.
Figure 1. (a) A schematic of the UVGI chamber drawer concept in the OPEN and CLOSED positions, (b) a photograph of the chamber in the OPEN position, (c) the top view of the UVGI FFR chamber, and (d) a front view of the FFR carrier frame.
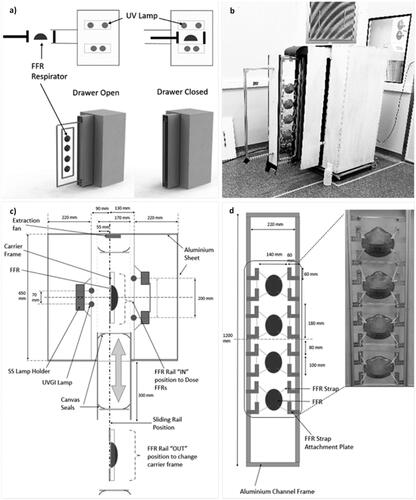
In the “OPEN” position, the carrier frame was loaded and unloaded onto a vertical drawer that slides into the chamber. Transient startup characteristics of UV-C lamps require a warm-up time for the output to stabilize. The configuration of the drawer/rail geometry eliminates the need to turn the lamps on or off between cycles, which is ideally suited to batch processing. The chamber, therefore, incorporated a long drawer-travel length with panel arrangements to protect operators (). Further details about the UVGI chamber design and process testing can be found in the supplementary material online.
Quality indicators for FFR reprocessing
Reprocessing of FFRs (decontamination and reuse) was measured using three quality indicators, including physical integrity/performance of the respirator (filtration efficiency and facial fit), safety for users, and effectiveness of pathogen reduction. In addition, each respirator was qualitatively evaluated for any soiling, damage, degradation of the FFR, or other defects. The FFRs were visually inspected pre-and-post decontamination and before fit testing to ensure all indelible markings are legible, based on an agreement between two people evaluating legibility (FDA Citation2020a).
Filtration efficiency
A total of 186 filtration efficiency tests were conducted including each FFR model after each decontamination and a control sample for each FFR model that was not subjected to decontamination. The test parameters and requirements for the inert aerosol collection efficiency testing were based on the SANS 50149 which is equivalent to EN 149. The filter material’s penetration was assessed by passing sodium chloride through the respirator at 95 L/min, using the TSI Certi Tester 8130 instrument (Protechnik Laboratories, a division of Armscor SOC LTD, South Africa). The instrument controls the generation of sodium chloride particle generation with the flame photometer as the detector. The test acceptance criteria were ≥94% filtration efficiency using a particle size distribution between 0.02 µm 2.03 µm equivalent aerodynamic diameter with a mass median diameter of 0.6 µm (or directional cumulative leakage of 6%).
Facial fit testing
Fit testing was performed on 27 participants following clearance for COVID-related symptoms. Subjects with beards or stubble, smoking within 30 min of fit testing, and those who were asthmatic or had respiratory conditions were excluded. All participants were briefed on the procedure’s principle, the correct donning and doffing, and conducting a seal check before they were fit tested. The respirator donning included adjustments until a satisfactory fit was felt; however, no adjustments were allowed during the fit testing. A calibrated TSI PortaCount Pro + Model 8038 (Bopp et al. Citation2020) using the OSHA-Modified (CNC) Fit Test Protocol with four exercises (bending over, talking, moving head from side-to-side and moving head up and down) (OSHA Citation2018) was used. These exercises aimed to stress the respirator seal in ways that simulate anticipated workplace motions. The PortaCount tester uses condensation nuclei counting (CNC) technology to count individual particles inside and outside the respirator to determine a quantitative estimate of the respirator fit factor. A batch of respirators was decontaminated with every cycle before each round of testing. For acceptance, the fit factor had to be ≥100, whilst a factor <100 was captured as the last test. Retesting was also discontinued if an FFR was damaged or soiled, or the user did not obtain a seal.
SARS-CoV-2 inactivation
The SARS-CoV-2 virus was propagated in Vero E6 cells ATCC CRL-1586 and used to inoculate the FFR swatches. Sterile stainless steel coupons (10 mm × 10 mm, 316 grade, M-Tech Diagnostics, Warrington) were used as controls.
A clinical isolate of SARS-CoV-2 (∼1.4 × 105 plaques forming units (PFU)/mL) at passage three was propagated in Vero E6 cells to generate a working stock. A confluent flask (≥90%) was infected with 1 mL SARS-CoV-2 and allowed to absorb for 1.5 hr in an incubator (ThermoForma, US) at 37 °C with 5% CO2, shaking every 15 min. After three days, when widespread cytopathic effect (CPE) was observed, the supernatant was collected, spun for 5 min at 3000 g on a benchtop centrifuge (Hettich, Germany), aliquoted in 2 mL tubes, and stored at −70 °C until analysis (Thermo Electron Corporation) (Campos et al. Citation2020).
For tissue culture infection and cell culture viability, the stock virus suspension aliquots were thawed using a water bath (37 °C) for 2 min (FMH Instruments) before use and inoculated into 24 hr old Vero E6 monolayer cells. The cultures were then incubated for approximately 72 hr in a 5% CO2 incubator and observed for cytopathic effects using an inverted microscope (Leica DMIL). Cell viability was determined using the Countess cell counter (Olympus, Japan).
Swatches excised (1 cm in diameter) from previously worn FFRs (i.e., FFRs used for fit testing) were decontaminated before impregnating the viral aliquots (150 μL) onto the outer surface to simulate the “real” contamination and were subjected to 1-hr adsorption at 25 °C. The swatches and metal disks were divided into two groups, A (not decontaminated) and B (decontaminated). Group B FFRs were exposed to UV within the chamber as indicated in and thereafter were subjected to extraction in 5 mL of viral transport media (VTM), vortexing, and centrifuging at 12,000 g for 20 min. A viral transport medium (5 mL) was used to extract SARS-CoV-2 from the contaminated FFR sample swatches (FFRs of Group A and B) and the metal disks.
The microbial log reductions were determined using a modification of the ASTM E-2197 quantitative swatch carrier method since there was no standardized method to evaluate the antimicrobial effectiveness of UVGI technologies. This was achieved by comparing the concentration recovered from the test swatches pre-and-post decontamination process (Havill et al. Citation2012). A set of non-spiked FFRs and metal disks were also tested to establish the baseline performance. For this study, decontamination was considered with a reduction of ≥3 log in pathogen load after reprocessing, corresponding to greater than 99.9% inactivation of least resistant pathogens (lipid or medium-sized viruses) (FDA Citation2020a, Citation2020b, Citation2020c).
Statistical analysis
All measurements were logged on datasheets and exported to STATA 16 (Stata Corp College Station, Texas, USA) for statistical analysis. Numerical variables were reported as mean and standard deviations or medians and interquartile ranges, while categorical variables were expressed as percentages. Changes in FFR fit were analyzed by comparing to the cutoff (≥100) and decontamination categories in intervals of five. The filtration penetration changes were determined by comparing the results of each decontamination cycle to the filtration efficacy cutoff (<6%). The log (formula 1) and percentage reduction (formula 2) were used to assess the reduction in the virus’s concentration after decontamination.
(1)
(1)
where CA and CB are concentrations of the virus, before and after decontamination.
(2)
(2)
Results
FFR physical observation
Four participants reported FFR 3M8810SSA strap failures across the 30 cycles performed, where the elastic bands were difficult to stretch post-decontamination and were prone to snapping (). Forty-eight reports (13%) regarding a burning smell after UVGI decontamination for all FFR models but mostly for 3M1860 (4.9%), 3M8810SSA (4.2%), and Duckbill (2.6%) were noted. No degradation was observed for any of the control FFRs that were not subjected to any decontamination.
Table 2. Observed structural and tactile defects following exposure to UVGI decontamination (N = 379).
Filtration efficiency
Filtration followed the last fit test which had a fit factor below 100. A total of 150 FFRs that were decontaminated for up to 30 cycles passed the filtration test using sodium chloride (NaCl, 0.6 μm) penetration, except the KN95 FFRs (n = 30) (). Control FFRs (n = 5) which were new FFRs that were not subjected to any decontamination cycle passed the filtration test, except for KN95. The overall averages (excluding KN95) ranged from 0.4 to 1.7%. The 3M8810SSA FFR had the lowest filtration (0.4%) followed by Makrite (0.9%), Greenline 5200 (1.5%), Duckbill 46727 (1.7%,) and 3M1860 (2.8%). The KN95 had the highest mean filtration penetration of 38.1%.
Table 3. Filter aerosol penetration (NaCl penetration %) per respirator for five FFR models using UVGI decontamination method for a maximum of 30 cycles.
Facial fit testing
A total of 815 facial fit tests were performed among 27 participants for the different FFR types (). The average fit factor baseline readings (i.e., first fit test performed) that were above the threshold (fit factor >100) were 3M8810SSA (174, SD:48.2), 3M1860 (157, SD:43.5), and Duckbill (127, SD:72.8) (). While, those below the threshold included Greenline 5200 (49, SD:50.2), Wenzhou (35, SD:57.3), and Makrite 9500 (27, SD:35.5) (). Several participants passed the fit test up to 30 cycles wearing 3M 1860 (n = 35), Duckbill (n = 13), 3M 8810SSA (n = 17), and Wenzhou KN95 (n = 5). Whereas the 5th cycle was the highest for participants using Makrite 9500 (n = 18) and Greenline 5200 (n = 20).
Table 4. Average respirator fit factor and fit factor range for categorized repeat decontamination cycles based on fit testing that passed (≥100).
SARS-CoV-2 inactivation
Post-decontamination there was a reduction in infected cells for all FFR samples with the highest reduction observed for Greenline 5200 (99.998%) and Duckbill (98.723%) (). The Greenline 5200 FFR achieved an average 4 log reduction. None of the other FFRs achieved ≥3 log reduction with repeat experiments (), which is important to demonstrate repeatability and reproducibility. However, single experiments demonstrated up to 5 log reductions for Duckbill, Makrite 9500, 3M8810SSA, Greenline 5200, and Wenzhou, but not 3M1860 (data not shown).
Figure 2. Graphical illustration of SARS-CoV-2 concentrations pre (A) and post (B) repeated decontamination experiments for several FFRS, with the asterisk indicating those achieving a 3 log reduction. Vflex was not tested for UVGI.
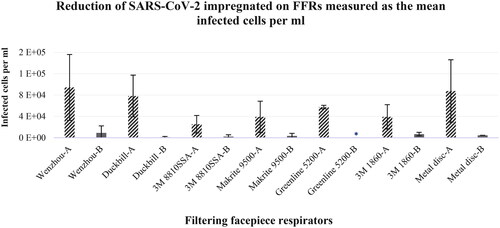
Table 5. Log reduction and percentage reduction of SARS-CoV-2 of impregnated FFRs measured as the mean infected cells per ml.
Discussion
Effect of UVGI decontamination on FFRs
The current study used a maximum of 1.2 J/cm2 with varying exposure times to achieve the intended dose for different FFR design configurations. With the targeted dosage, 1.1% strap failure was noted and 13% burn-like odor reports post UVGI decontamination. Our findings are similar to previous reports regarding the fragmentation of the elastic material in the straps when stretched (Schumm et al. Citation2021). Another study also reported an “intolerable odour” of one FFR treated with UVGI (Goyal et al. Citation2014). Although the odor phenomenon appears to be common for UVGI technology and nothing suspicious; an odor is not ideal. The odors appear to dissipate after approximately 4 hr, hence should be considered when using UVGI (Heimbuch and Harnish Citation2019).
Effect of repeated UVGI decontamination on filtration
The UVGI decontamination method preserved filtration efficiency for all FFRs tested except the Wenzhou KN95 respirator. Nevertheless, we found that 53 fit tests using the Wenzhou KN95 with repeated UVGI decontamination passed, although, the filtration efficiency test failed on all cycles; highlighting the importance of a comprehensive validation before implementation of any FFR decontamination protocol.
Effect of repeated UVGI decontamination on facial fit
Our study showed that depending on the FFR type, some could withstand 30 cycles of donning and doffing. We showed that wear and tear from donning and doffing with repeated use might damage the respirator faster than repeated decontamination. We demonstrate Makrite failure after five cycles of fit testing, possibly due to its firmer shape resulting in a poor facial fit and increased leakage. Therefore, donning and doffing practices, storage conditions, and the duration of use may be critical determinants of fit test failures (Bioquell Citation2016; Vuma et al. Citation2019; Smullin and Tarlow Citation2021). For this reason, FFRs should be assigned to one wearer even when reused as nose clips and headbands adjust to the wearer’s face and impact fit and seal (O’Kelly et al. Citation2021).
The researchers also demonstrated that fit testing alone might be insufficient in assessing FFR effectiveness as a few individuals passed despite the respirator failing the filtration efficiency test for Wenzhou KN95, even before decontamination. The results also show that it cannot be assumed that one respirator will fit a work cohort and that whichever FFRs are used are validated against the decontamination method. The number of decontamination cycles is limited by mechanical wear and/or soiling of FFRs, rather than cumulative UV-C exposure. The physical appearance, odor, and laboratory performance (filter aerosol penetration and filter airflow resistance) of six N95 FFRs models after three cycles of decontamination with UVGI were also not affected (Bergman et al. Citation2010). Our results corroborate other findings (Fischer et al. Citation2020) regarding the number of cycles while maintaining the fit testing integrity after more than one cycle.
The investigators had no operator symptom reports, and thus the prototype is safe for operators in the short term. The World Health Organization considers, among others, the use of UV-C in reprocessing PPE under severe shortage (Frigerio Citation2020). The UVGI cabinet designed for this study provides an easily constructed, cost-effective solution using locally available components, with sufficiently high and scalable throughput to meet the demands of small hospitals, clinics, and first responders who experience FFR shortages. The authors acknowledge that in some reduced or low-resourced settings, UVGI may not be feasible owing to infrastructure (electrical) or other resources.
Inactivation of UVGI decontamination on SARS-CoV-2 contaminated FFRs
The percentage reduction of SARS-CoV-2 impregnated on the six FFR models differed, with one (Greenline 5200 FFR) achieving a ≥ 3 log reduction (99.9%) using UVGI. These findings concur with the literature (Wilde et al. Citation2020), showing that a UV-C irradiation dose ≥ 1 J/cm2 at the FFR surface inactivates SARS-CoV-2 (Ozog et al. Citation2020) and its predecessor SARS-CoV (Heimbuch and Harnish Citation2019) by ≥ 3 log reduction on most tested N95 facepieces, while our study only achieved a ≥ 3 log reduction with SARS-CoV-2 on one FFR. Bergman et al. (Citation2010) also demonstrated the UVGI decontamination effectiveness of six N95 FFRs models after three cycles; however, their study’s limitation was that only the exteriors of the FFRs were exposed. UVGI rapidly inactivates SARS-CoV-2 on steel but more slowly on N95 fabric, likely due to its porous nature (Bergman et al. Citation2010), and is protected from inactivation if it penetrates deep into the layers of the FFR as UVGI may not penetrate the deeper layers (Lindsley et al. Citation2015). However, another study that “sandwiched” the spores strips between two N95 FFRs layers showed a 6-log inactivation in all locations after a single dose of 1 J/cm2 (Purschke et al. Citation2020). This is an important finding supporting our results that UVGI is suitable the for decontamination and reuse of FFRs. In addition, a report commissioned by the FDA (2019) reported that N95 samples with hydrophilic surfaces showed consistent mean viral reductions lower than 103 while those with hydrophobic surfaces showed mean viral reductions greater than 103 (Heimbuch and Harnish Citation2019). Another study reported similar findings (Ozog et al. Citation2020). UVGI decontamination demonstrated significant reductions in influenza virus recovery and viability from N95 even with soiling conditions (mucin and sebum-soiled facepieces). It has shown a significant reduction in the recovery of B. subtilis, inactivation of 99.9% to 99.999% of respiratory viruses such as Influenza A (H1N1), Avian Influenza A virus (H5N1), Influenza A (H7N9) A/Anhui/1/2013, Influenza A (H7N9) A/Shanghai/1/2013, MERS-CoV, and SARS-CoV and inactivation of SARS-CoV-2. The inactivation of the target microorganism/s is a critical factor in considering the effectiveness of any decontamination method. The FDA recommends a 6 log reduction corresponding to the highest level of resistance (bacterial spores) when a decontamination system is intended for multiple users due to the increased risk of transmission of individuals’ bioburden or a ≥ 3 log reduction of non-enveloped viruses/Gram-positive/Gram-negative bacteria for single-use to supplement existing CDC reuse recommendations (FDA Citation2020c).
Three studies showed virucidal inactivity of > 3 log reduction of SARS-CoV-2 using UVGI (Fischer et al. Citation2020; Ozog et al. Citation2020; Smith et al. Citation2021), however, each had a small FFR sample, mainly of the 3M models and no filtration efficiency testing was done in two of the studies, while two did fit testing on few cycles. Smullin and Tarlow (Citation2021) reported that SARS-COV-2 inactivation occurs spontaneously at room temperature; however, the timing depends on humidity, temperature, and surface material. Therefore, extended use without decontamination may increase the risk of exposure to microorganisms with long survival times. Owing to variability in room temperature conditions and FFRs, assigning a precise 3-log decay time for all used N95 FFRs is impossible (Smullin and Tarlow Citation2021). Moreover, the time to get from 1000 active particles to 1 active particle is the same as getting from 5,000 to 5 active particles. The fact that we did not achieve as high log reduction for all FFRs as others (Rothe et al. Citation2020) could imply that the doses used were insufficient to damage the vital macromolecules for replicating pathogens impregnated on different FFR materials and surfaces. Thus, further investigations into higher doses, which are still safe for the end-user, are warranted for the other FFRs.
According to public reporting, extended use and re-use of FFRs have become common in hospitals in areas where SARS-CoV-2 is high. However, the appropriate decontamination and subsequent re-use of FFRs should not pose a health risk to the already taxed healthcare workers (Kumar et al. Citation2020). Our study shows that certain FFR’s use can potentially be extended several-fold without degradation of functional integrity depending on the type of material used and natural variability due to the target agent’s “biological” nature. Care was taken to ensure controlled irradiance distribution over the FFR surface; however, the Vflex respirator was unsuitable for UVGI decontamination due to the pleated material.
While the actual efficacy of the UVGI system used may not have been fully optimized given the time constraints; extensive efforts were made to ensure the parameters are in line with what is used with success globally. The prototype design considered several challenges associated with UVGI for FFR applications, including shadowing and repeatable mask mounting. The final configuration resulted in a unit that can accommodate four FFRs per cycle, a system that is easy and safe to operate. It is noted that, in general, processes are effective within a specific operating environment/envelope only. The prototype can be enhanced to increase production throughput, and reduce the device’s size and cost.
Although the effectiveness of decontamination with UVGI is not in dispute, and the evidence indicates that the integrity of the respirators is not significantly affected using the decontamination process, some serious concerns are raised regarding quality assurance and user safety. Hence, based on our findings, it is suggested that decontamination be used only for single users and a protocol be established to manage the logistics and quality thereof. The loss of health workers to preventable illnesses due to inadequate access to quality RPE is unacceptable. Therefore, it is envisaged that the current findings add to the growing body of evidence regarding the efficacy of decontamination of FFRs for low-resource settings, among other settings.
The investigators recognize that placing liquid aliquots of SARS-CoV-2 on the outside of the FFR filter compared to the collection of infectious particles within a filter by inhalation is a limitation. However, using SARS-CoV-2 in these experiments is complex and sensitive to several factors (e.g., type of cell line, age of cell line, the pathogenicity of strain, the timing of infectivity, environmental conditions, etc.). Nonetheless, efforts were made to ensure the parameters are similar to what is used with success globally.
Conclusion
This study comprehensively evaluated the efficacy of a low-cost UVGI chamber as a promising method for decontaminating FFRs and verifying the bioburden reduction of SARS-CoV-2. A ≥ 3 log reduction of SARS-CoV-2 was achieved with worn-in Greenline 5200 FFRs using UVGI. The respirator’s ability for re-use was demonstrated even after multiple cycles (up to 30) of decontamination as the fit factor and filtration efficiency did not significantly deteriorate for 27 users and the FFRs types tested. The low-cost UVGI cabinet design can be used in facilities with low throughput. However, decontamination and re-use of FFRs are model-specific, thus, facilities must validate the methods for the type of FFR used.
Recommendations
The number of times an FFR can be reused is restricted by its failure in maintaining a good fit with repeated use rather than by the degradation from the decontamination process itself. Hence, manufacturers should consider using more durable straps. There is, unfortunately, no single FFR decontamination and reuse solution for all FFRs and settings. Each individual or facility must select and tailor a solution according to their needs, capability, and available resources. Given the current high demand, usage, and cost of FFRs, the fact that decontaminated FFRs still offer significant user protection, and the comparatively low cost of decontamination, the benefit of increasing FFR reserves should easily outweigh the application costs. If a third of the FFRs could be decontaminated and put back into the system, considerable costs could be saved as well as ensure the safety of frontline and essential workers, which is paramount. It must be reiterated that FFR decontamination should be implemented as a risk-mitigation strategy during crisis capacity conditions only and with proper engineering, industrial hygiene, and biosafety controls.
Author contributions
Tanusha Singh (TS), Thabang Duba (TD), Dikeledi Onnicah Matuka (DOM), Lufuno Muleba (LM), Daniel Glaser (DG), Edith Ratshikhopha (ER), Zubaydah Kirsten (ZK), Tobias van Reenen (TvR), Zibusiso Masuku (ZM), Dikeledi Singo (DS), Lebogang Ntlailane (LN), Tebogo Nthoke (TN), David Jones (DJ), Mary Ross (MR), Pieter du Toit (PdT); Conceptualization and study design: TS, TvR, ZM; Protocol development: TS, TD, DOM, DG, MR; Searching: LM, ZK, ER; Acquisition, analysis, and interpretation of analysis: DOM, TD, LM, DR, DS, LN, TN; Statistical analysis: TS, ER; Radiometry: DG, PdT; Results interpretation: TS, TvR, DG, TD, DOM, LM; First draft preparation: TS; Revisions and final drafting of the manuscript: All authors. Approval of the manuscript for submission: All authors. All the authors contributed to the planning, logistics, data analysis, and drafting of the manuscript.
Disclaimer
Current South African legislation and manufacturers’ instructions only permit the disposable FFR to be utilized as single-use. The study should in no way be used as justification for the reuse of the single-use FFR unless legislation is amended permitting such reuse. Data from this study will be made available upon reasonable request.
Supplemental Material
Download PDF (218.1 KB)Acknowledgments
We thank all participants for performing the fit testing. We also thank Njabulo Xulu for the acquisition of consumables, Ms. Zethembiso Ngcobo for laboratory assistance, and Mr. Felix Made for data analysis consultations. Special thanks to Prof. Bhavesh Kana from DST/NRF Centre of Excellence for Biomedical TB Research (CBTBR, South Africa) for donating the SARS-CoV-2 clinical strain. Thanks to the technical team from Protechnik Laboratories (South Africa) for conducting the filtration tests. We wish to acknowledge the contribution of our late colleague David Rangongo. The authors alone are responsible for the views expressed in this article, and they do not necessarily represent the views, decisions, or policies of the institutions with which they are affiliated.
Conflict of interest
No potential conflict of interest was reported by the author(s).
Additional information
Funding
References
- 3M Science. 2020. Decontamination of 3M filtering facepiece respirators, such as N95 respirators, in the United States – considerations. Technical Bulletin, September 2020, revision 11. 3M Science Applied to Life Technical Bulletin.
- Allen J, McCausland P, Farivar C. 2020. Trump administration paying huge premium for mask-cleaning machines. Which don’t do the job. 2020. NBCNews.com; [accessed 2021 Feb 04]. https://www.nbcnews.com/politics/white-house/trumpadministration-paying-huge-premium-mask-cleaning-machines-which-don-n1210896.
- Arnett D. 2020. Mask sterilizer’s safety questioned – The Boston Globe; [accessed 2021 Jan 19]. https://edition.pagesuite.com/popovers/dynamic_article_popover.aspx?artguid=41fc266cf827-4b40-b58c-7feb72d3184f.
- Bergman MS, Viscusi DJ, Heimbuch BK, Wander JD, Sambol AR, Shaffer RE. 2010. Evaluation of multiple (3-cycle) decontamination processing for filtering facepiece respirators. J Eng Fibers Fabr. 5(4):155892501000500–155892501000541.
- Bioquell. 2016. Bioquell HPV decontamination for reuse of N95 respirators: final report, 22 July 2016. Battelle, Columbus (OH). Contract No. HHSF223201400098C. Study no 3245. FDA Contracting Officer’s Representative Brenda Brooks.
- Bong CL, Brasher C, Chikumba E, McDougall R, Mellin-Olsen J, Enright A. 2020. The COVID-19 pandemic: effects on low- and middle-income countries. Anesth Analg. 131(1):86–92. doi:10.1213/ANE.0000000000004846.
- Bopp NE, Bouyer DH, Gibbs CM, Nichols JE, Ntiforo CA, Grimaldo MA. 2020. Multicycle autoclave decontamination of N95 filtering facepiece respirators. Appl Biosaf. 25(3):150–156. doi:10.1177/1535676020924171.
- Boskoski I, Gallo C, Wallace MB, Costamagna G. 2020. COVID-19 pandemic and personal protective equipment shortage: protective efficacy comparing masks and scientific methods for respirator reuse. Gastrointest Endosc. 92(3):519–523. doi:10.1016/j.gie.2020.04.048.
- Byrne JD, Wentworth AJ, Chai PR, Huang HW, Babaee S, Li C, Becker SL, Tov C, Min S, Traverso G. 2020. Injection Molded Autoclavable, Scalable, Conformable (iMASC) system for aerosol-based protection: a prospective single-arm feasibility study. BMJ Open. 10(7):e039120. doi:10.1136/bmjopen-2020-039120.
- Campos RK, Jin J, Rafael GH, Zhao M, Liao L, Simmons G, Chu S, Weaver SC, Chiu W, Cui Y. 2020. Decontamination of SARS-CoV‑2 and other RNA viruses from N95 level meltblown polypropylene fabric using heat under different humidities. ACS Nano. 14(10):14017–14025. doi:10.1021/acsnano.0c06565.
- Cassorla L. 2021. Decontamination and reuse of N95 filtering facepiece respirators: where do we stand? Anesth Analg. 132(1):2–14.
- CDC. 2020. Decontamination and reuse of filtering facepiece respirators; [accessed 2021 Jan 19]. https://www.cdc.gov/coronavirus/2019-ncov/hcp/ppe-strategy/decontamination-reuse-respirators.html.
- CDC. 2021. Summary for healthcare facilities: strategies for optimizing the supply of N95 respirators during shortages. National Center for Immunization and Respiratory Diseases (NCIRD), Division of Viral Diseases; [accessed 2022 Aug 24]. https://www.cdc.gov/coronavirus/2019-ncov/hcp/checklist-n95-strategy.html.
- Cordoba-Lanus E, Garcia-Perez O, Cazorla-Rivero S, Rodriguez-Esparragon F, Pinero JE, Clavo B, Lorenzo-Morales J. 2021. Persistence of SARS-CoV-2 infection on personal protective equipment (PPE). BMC Infect Dis. 21(1):1169. doi:10.1186/s12879-021-06861-7.
- De Kock JH, Latham HA, Leslie SJ, Grindle M, Munoz SA, Ellis L, Polson R, O'Malley CM. 2021. A rapid review of the impact of COVID-19 on the mental health of healthcare workers: implications for supporting psychological well-being. BMC Public Health. 21(1):104. doi:10.1186/s12889-020-10070-3.
- Derraik JGB, Anderson WA, Connelly EA, Anderson YC. 2020. Rapid review of SARS-CoV-1 and SARS-CoV-2 viability, susceptibility to treatment, and the disinfection and reuse of PPE, particularly filtering facepiece respirators. Int J Environ Res Public Health. 17(17):6117–6114. doi:10.3390/ijerph17176117.
- FDA. 2020a. Authorised Bioquell technology system. St. Paul, MN: US Food and Drug Administration.
- FDA. 2020b. Department of Health and Human Services. Recommendations for sponsors requesting EUAs for decontamination and bioburden reduction systems for surgical masks and respirators during the Coronavirus Disease 2019 (COVID-19) Public Health Emergency; [accessed 2021 Mar 10]. https://www.fda.gov/media/138362/download.
- FDA. 2020c. Recommendations for sponsors requesting EUAs for decontamination and bioburden reduction systems for surgical masks and respirators during the Coronavirus Disease 2019 (COVID19) Public Health Emergency Guidance for Industry and Food and Drug Administration Staff; [accessed 2021 Mar 15]. https://www.fda.gov/emergency-preparedness-and-response/mcm-issues/covid-19-relatedguidance-documents-industry-fda-staff-and-other-stakeholders.
- Fischer RJ, Morris DH, van Doremalen N, Sarchette S, Matson MJ, Bushmaker T, Yinda CK, Seifert SN, Gamble A, Williamson BN, et al. 2020. Effectiveness of N95 respirator decontamination and reuse against SARS-CoV-2 virus. Emerg Infect Dis. 26(9):2253–2255. doi:10.3201/eid2609.201524.
- Frigerio F, Borra M, Cottica D, Grignani E, Militello A, Mansi A, Tirabasso A, Tranfo G, Sisto R. 2020. Interim indications for the use of UV germicidal irradiation in the conjuncture of COVID-19 pandemic. Italian J Occup Environ Hyg. 11(1):1–51.
- Goyal SM, Chander Y, Yezli S, Otter JA. 2014. Evaluating the virucidal efficacy of hydrogen peroxide vapour. J Hosp Infect. 86(4):255–259. doi:10.1016/j.jhin.2014.02.003.
- Grossman J, Pierce A, Mody J, Gagne J, Sykora C, Sayood S, Cook S, Shomer N, Liang SY, Eckhouse SR. 2020. Institution of a novel process for N95 respirator disinfection with vaporized hydrogen peroxide in the setting of the COVID-19 pandemic at a large academic medical center. J Am Coll Surg. 231(2):275–280.
- Havill NL, Moore BA, Boyce JM. 2012. Comparison of the microbiological efficacy of hydrogen peroxide vapor and ultraviolet light processes for room decontamination. Infect Control Hosp Epidemiol. 33(5):507–512. doi:10.1086/665326.
- Heimbuch BK, Harnish D. 2019. Research to mitigate a shortage of respiratory protection devices during public health emergencies. Tech. Rep. HHSF223201400158C. 2019. Engineering Science Division, Panama City, FL. Applied Research Associates, Inc; [accessed 2021 Jan 20]. https://www.ara.com/news/ara-research-mitigate-shortage-respiratory-protection-devices-during-public-health-emergencies.
- Heimbuch BK, Wallace WH, Kinney K, Lumley AE, Wu C-Y, Woo M-H, Wander JD. 2011. A pandemic influenza preparedness study: use of energetic methods to decontaminate filtering facepiece respirators contaminated with H1N1 aerosols and droplets. Am J Infect Control. 39(1):e1–e9.
- Jacobs N, Chan K, Leso V, D'Anna A, Hollins D, Iavicoli I. 2020. A critical review of methods for decontaminating filtering facepiece respirators. Toxicol Ind Health. 36(9):654–680. doi:10.1177/0748233720964652.
- Kumar A, Kasloff SB, Leung A, Cutts T, Strong JE, Hills K, Vazquez-Grande G, Rush B, Lother S, Zarychanski R. 2020. N95 mask decontamination using standard hospital sterilization technologies. MedRxiv Preprint. doi: 10.1101/2020.04.05.20049346.
- Liao L, Xiao W, Zhao M, Yu X, Wang H, Wang Q, Chu S, Cui Y. 2020. Can N95 respirators be reused after disinfection? How many times? ACS Nano. 14(5):6348–6356.
- Lindsley WG, Martin SB, Jr, Thewlis RE, Sarkisian K, Nwoko JO, Mead KR, Noti JD. 2015. Effects of Ultraviolet Germicidal Irradiation (UVGI) on N95 respirator filtration performance and structural integrity. J Occup Environ Hyg. 12(8):509–517. doi:10.1080/15459624.2015.1018518.
- Mills D, Harnish DA, Lawrence C, Sandoval-Powers M, Heimbuch BK. 2018. Ultraviolet germicidal irradiation of influenza-contaminated N95 filtering facepiece respirators. Am J Infect Control. 46(7):e49–e55. doi:10.1016/j.ajic.2018.02.018.
- O’Kelly E, Arora A, Pirog S, Ward J, Clarkson PJ. 2021. Comparing the fit of N95, KN95, surgical, and cloth face masks and assessing the accuracy of fit checking. PLoS One. 16(1):e0245688. doi:10.1371/journal.pone.0245688.
- OSHA. 2018. TSI software and firmware wizard. Occupational Safety and Health Administration modified fit test protocol. Fitpro + v3.3.0; [accessed 2020 Oct 20]. https://www.tsi.com/support/tsi-software-and-firmware-wizard/.
- Ou Q, Pei C, Kinm SC, Abell E, David Y, Pui H. 2020. Evaluation of decontamination methods for commercial and alternative respirator and mask materials – view from filtration aspect. J Aerosol Sci. 150:105609. doi:10.1016/j.jaerosci.2020.105609.
- Ozog DM, Sexton JZ, Narla S, Pretto-Kernahan CD, Mirabelli C, Lim HW, Hamzavi IH, Tibbetts RJ, Mi Q-S. 2020. The effect of ultraviolet C radiation against different N95respirators inoculated with SARS-CoV-2. Int J Infect Dis. 100:224–229. doi:10.1016/j.ijid.2020.08.077.
- Purschke M, Elsamaloty M, Wilde JP, Starr N, Anderson RR, Farinelli WA, Sakamoto FH, Tung M, Tam J, Hesselink L, et al. 2020. Construction and validation of UV-C decontamination cabinets for filtering facepiece respirators. Appl Opt. 59(25):7585–7595. doi:10.1364/AO.401602.
- Rashid TU, Sharmeen S, Biswas S. 2022. Effectiveness of N95 masks against SARS-CoV-2: performance efficiency, concerns, and future directions. ACS Chem Health Saf. 29(2):135–164. doi:10.1021/acs.chas.1c00016.
- Rothe M, Rohm E, Mitchell E, Bedrosian N, Kelly C, String G, Lantagne D. 2020. A systematic review of mask disinfection and reuse for SARS-CoV-2. medRxiv Preprint. doi:10.1101/2020.11.11.20229880.
- Rowan NJ, Laffey JG. 2020. Challenges and solutions for addressing critical shortage of supply chain for personal and protective equipment (PPE) arising from Coronavirus disease (COVID-19) pandemic – case study from the Republic of Ireland. Sci Total Environ. 725:138532. doi:10.1016/j.scitotenv.2020.138532.
- Ruskin KJ, Ruskin AC, Musselman BT, Harvey JR, Nesthus TE, O'Connor M. 2021. COVID-19, personal protective equipment, and human performance. Anesthesiology. 134(4):518–525. doi:10.1097/ALN.0000000000003684.
- Rutala WA, Weber DJ, Healthcare Infection Control Practices Advisory Committee. 2019. Guideline for disinfection and sterilization in healthcare facilities, 2008 update: May 2019; [accessed 2021 Feb 5]. https://www.cdc.gov/infectioncontrol/guidelines/disinfection/sterilization/other-methods.html.
- Schumm MA, Hadaya JE, Mody N, Myers BA, Maggard-Gibbons M. 2021. Filtering facepiece respirator (N95 respirator) reprocessing: a systematic review. JAMA. 325(13):1296–1317. doi:10.1001/jama.2021.2531.
- Schwartz A, Stiegel M, Greeson N, Vogel A, Thomann W, Brown M, Sempowski GD, Alderman TS, Condreay JP, Burch J, et al. 2020. Decontamination and reuse of N95 respirators with hydrogen peroxide vapor to address worldwide personal protective equipment shortages during the SARS-CoV-2 (COVID-19) pandemic. Appl Biosaf. 25(2):67–70. Doi: 10.1177/1535676020919932.
- Sehgal NJ, Milton DK. 2021. Applying the hierarchy of controls: what occupational safety can teach us about safely navigating the next phase of the global COVID-19 pandemic. Front Public Health. 9:747894. doi:10.3389/fpubh.2021.747894.
- Smith JS, Hanseler H, Welle J, Rattray R, Campbell M, Brotherton T, Moudgil T, Pack TF, Wegmann K, Jensen S, et al. 2021. Effect of various decontamination procedures on disposable N95 mask integrity and SARS-CoV-2 infectivity. J Clin Transl Sci. 5(1):E10. doi:10.1017/cts.2020.494.
- Smullin SJ, Tarlow BD. 2021. Room temperature wait and reuse for bioburden reduction of SARS-CoV-2 on N95 filtering facepiece respirators. Appl Biosaf. 26(2):103–111. doi:10.1089/apb.20.0055.
- Syphers D. 2020. Determining exposure times for UV-C irradiation of ppe filtration facemasks for sterilization and potential reuse in times of shortages. 2020. Bowdoin College; [accessed 2020 Sep 25]. https://www.bowdoin.edu/profiles/faculty/dsyphers/pdf/determining-exposure-times.
- Tseng CC, Li CS. 2007. Inactivation of viruses on surfaces by ultraviolet germicidal irradiation. J Occup Environ Hyg. 4(6):400–405. doi:10.1080/15459620701329012.
- van der Vossen J, Fawzy A, Ouwens AMT, van Doornmalen J, de Samber M, Driessens R, Heerikhuisen M, Montijn RC. 2022. Effective ultraviolet C light disinfection of respirators demonstrated in challenges with Geobacillus stearothermophilus spores and SARS-CoV-2 virus. J Hosp Infect. 122:168–172. doi:10.1016/j.jhin.2022.01.021.
- van Doremalen N, Morris DH, Holbrook MG, Gamble A, Williamson BN, Tamin A, Harcourt JL, Thornburg NJ, Gerber SI, Lloyd-Smith JO, et al. 2020. Aerosol and surface stability of SARS-CoV-2 as compared with SARS-CoV-1. N Engl J Med. 382(16):1564–1567.
- Vanhooydonck A, Van Goethem S, Van Loon J, Vandormael R, Vleugels J, Peeters T, Smedts S, Stokhuijzen D, Van Camp M, Veelaert L, et al. 2021. Case study into the successful emergency production and certification of a filtering facepiece respirator for Belgian hospitals during the COVID-19 pandemic. J Manuf Syst. 60:876–892. doi:10.1016/j.jmsy.2021.03.016.
- Vuma CD, Manganyi J, Wilson K, Rees D. 2019. The effect on fit of multiple consecutive donning and doffing of N95 filtering facepiece respirators. Ann Work Expo Health. 63(8):930–936. doi:10.1093/annweh/wxz060.
- Weaver DT, McElvany BD, Gopalakrishnan V, Card KJ, Crozier D, Dhawan A, Dinh MN, Dolson E, Farrokhian N, Hitomi M, et al. 2020. UV decontamination of personal protective equipment with idle laboratory biosafety cabinets during the COVID-19 pandemic. MedRxiv Preprint. doi: 10.1101/2020.03.25.20043489.
- Wharton K, Rieker M. 2020. N95 respirator decontamination and reuse: current state of the evidence. AANA J. 31–36.
- Wilde JP, Baer TM, Hesselink L. 2020. Modeling UV-C irradiation chambers for mask decontamination using Zemax OpticStudio. Appl Opt. 59(25):7596–7605.