Abstract
Firefighters are exposed to carcinogenic and mutagenic combustion emissions, including polycyclic aromatic hydrocarbons (PAHs). Fire service and firefighter cancer advocacy groups recommend skin cleaning using wipes or washing with detergent and water after exposure to smoke, although these strategies have not been proven to reduce exposures to harmful combustion products such as PAHs. This study assessed dermal decontamination methods to reduce PAH exposures by firefighters participating in live fire training scenarios. Study participants (n = 88) were randomly assigned to an intervention group (i.e., two types of commercial skin wipes, detergent and water, or a control group who did not use any skin decontamination). PAHs were measured in personal air (during the fire) and dermal wipe samples (before and after fire suppression and after dermal decontamination). PAH metabolites and mutagenicity were measured in urine samples before and after fire suppression. Airborne PAH concentrations during the fire ranged between 200 and 3,970 μg/m3 (mean = 759 μg/m3, SD = 685 μg/m3). Firefighters had higher total PAHs and high-molecular-weight PAHs on their skin after the fire compared to before (1.3- and 2.2-fold, respectively, p < 0.01). Urinary PAH metabolites increased significantly following exposure to the training fires by 1.7 to 2.2-fold (depending on the metabolite, p < 0.001). Urinary mutagenicity did not differ significantly between pre- and post-fire for any of the decontamination methods. Detergent and water was the only intervention that removed a significant amount of total PAHs from the skin (0.72 ng/cm2 preintervention vs. 0.38 ng/cm2 postintervention, p < 0.01). However, fold changes in urinary PAH metabolites (i.e., pre- vs. post-exposure levels) did not differ among any of the dermal decontamination methods or the control group. These data suggest that despite on-site attempts to remove PAHs from firefighters’ skin, the examined interventions did not reduce the internal dose of PAHs. Future work should investigate preventing initial exposure using other interventions, such as improved personal protective equipment.
Introduction
Firefighters face serious occupational hazards, including heat, stress (physical/mental), and toxic chemicals. Epidemiological studies have shown that these exposures can have lasting effects on firefighters, resulting in an increased risk of cancer and other serious diseases compared with the general population (Jalilian et al. Citation2019; Soteriades et al. Citation2019; Casjens et al. Citation2020). In fact, the International Agency for Research on Cancer has classified the occupation of firefighting as “carcinogenic to humans” (i.e., Group 1) (Demers et al. Citation2022). Exposures to carcinogens can occur from firefighters’ inherent contact with combustion emissions that can contain benzene, chloroform, acrolein, formaldehyde, asbestos, lead, and polycyclic aromatic hydrocarbons (PAHs) (Bolstad-Johnson et al. Citation2000; Golka and Weistenhöfer Citation2008). PAHs are also mutagenic and teratogenic and are of particular concern due to their ubiquitous formation during combustion events (IARC Citation2010).
Several studies have assessed firefighters’ exposures to PAHs by measuring PAHs in air, on skin, and on personal protective equipment (PPE)/clothing (Keir et al. Citation2020; Banks et al. Citation2021a, Citation2021b). For example, Keir et al. (Citation2020) found that air PAH concentrations sometimes exceeded occupational exposure limits and that firefighters’ experienced significant increases of PAHs on skin and PPE. Urinary PAH metabolites have also been broadly used to assess PAH exposure and uptake (i.e., internal dose), and several studies have found increased PAH metabolites associated with suppression of emergency municipal fires (Keir et al. Citation2017; Hoppe-Jones et al. Citation2021), training fires (Wingfors et al. Citation2018), staged fires (Fent et al. Citation2020), and wildfires (Cherry et al. Citation2021). Urinary mutagenicity is another noninvasive tool that permits a nontargeted exposure assessment to mutagenic aromatic compounds excreted via urine; it does not require a priori information on the identity of the putative toxicants. Increases in urinary mutagenicity have been measured in firefighters engaged in emergency fire suppression (Keir et al. Citation2017).
Several researchers have noted that dermal penetration is likely the predominant PAH exposure pathway (Laitinen et al. Citation2012; Fent et al. Citation2014; Keir et al. Citation2017). Firefighters’ airways are protected by their self-contained breathing apparatus, whereas skin is typically exposed to combustion emissions, and elevated temperatures encountered during fire suppression can enhance skin permeability (Chang and Riviere Citation1991; Jones et al. Citation2003; Park et al. Citation2008). In fact, several studies have found significantly elevated postfirefighting levels of PAHs on firefighters’ skin, such as on the neck (Fent et al. Citation2014; Keir et al. Citation2017). Given the weight of the evidence above that firefighters are exposed to PAHs through their skin, postexposure removal of dermally deposited PAHs could potentially reduce overall exposure and internal dose (Fent et al. Citation2014, Citation2017; Fernando et al. Citation2016; Keir et al. Citation2017). As such, dermal decontamination has been suggested as an effective means of removing PAHs deposited on skin. Indeed, skin-cleaning wipes are now often deployed for post–fire suppression dermal decontamination. The intention is to reduce postexposure dermal absorption of carcinogens via on-scene removal of contaminants deposited on skin, that is, prior to showering upon return to the fire station. However, the efficacy of dermal cleaning procedures for reduction of PAH exposures and internal dose has not been broadly investigated. Fent et al. (Citation2017) found that baby wipes were able to significantly reduce postexposure PAH levels on firefighters’ necks by a median of 54%. However, they only considered external PAH contamination levels; they did not consider PAH absorption and internal dose (e.g., urinary metabolite concentration). Moreover, skin-cleaning wipes can contain a range of ingredients, and it is not clear whether the specific types of wipes and/or wiping procedures can alter the ability to remove PAHs deposited on skin. This study aimed to determine whether skin decontamination procedures (i.e., select skin wipes, detergent and water) reduce PAHs deposited on skin and internal dose (i.e., urinary PAH metabolites and mutagenicity). The study results will permit firefighters and decision-makers to make evidence-based choices regarding the most appropriate way to reduce dermal exposures to combustion emissions.
Methods
Study design
Research ethics approval was obtained from the University of Ottawa Research Ethics Board (i.e., H-08-18-867) and Health Canada’s Research Ethics Board (i.e., REB 2018-0020).
Firefighters from the Ottawa Fire Services and the Canadian Armed Forces were recruited via email or during classroom training sessions. Eligible participants were nonsmokers who did not live with smokers, who agreed to avoid consumption of charbroiled foods and non-occupational exposures to combustion sources for the duration of enrollment, and who did not participate in fire suppression activities in the previous 72 hours. Each participant completed a detailed questionnaire about their personal habits, overall health, and the nature of their employment (i.e., duration, secondary employment, and so on).
Sampling was conducted during particle board training fires in modified shipping containers (40’ length × 8’ width × 8.5’ height) because they are controlled environments where multiple people are exposed under similar conditions. The shipping containers could hold six to eight firefighters at a time. The training involved instructors near the fire at one end of the shipping container, controlling the fire’s growth. The other firefighters, including the study participants, were grouped in the same container in a static position, moving occasionally in a circular pattern to take turns applying water on the fire with a nozzle. Activity, fuel load, and conditions were identical for all fires to ensure equivalent exposure.
Firefighters were randomly assigned to one of four post-fire decontamination methods on the day of sampling with a minimum of four participants recruited per fire (i.e., one per decontamination group). A second set of participants could be sampled (i.e., maximum of eight firefighters) per fire.
The decontamination methods included the following:
Commercial wipe A: premoistened, non-rinse, 7 × 7 inch disposable cloths marketed for cleaning of skin after exposure to substances such as grease or paint. The first two listed ingredients were water and an alcohol.
Commercial wipe B: premoistened, fragrance-free baby wipes. The first two listed ingredients were water and aloe vera extract.
The two commercial wipes ranged in cost and ingredients.
Detergent and water: applied using a washcloth dipped in a bucket with an approximately 4 tablespoons of dish detergent in 5 L of water. This mixture was chosen due to its effectiveness at removing PAHs from contaminated gear (Fent et al. Citation2017).
Control group: no dermal decontamination was conducted.
Those assigned to a decontamination method were instructed to wash their skin in the same way they would after removing their PPE, including cleaning their arms, legs, neck, face, and hands. Decontamination occurred immediately upon exiting the fire and removal of their PPE (i.e., <10 minutes). Each decontamination group (i.e., three types of dermal cleaning and the control) contained 22 individuals.
Skin wipe samples
Skin PAH concentrations were assessed using a previously employed methodology (Keir et al. Citation2017). Briefly, skin wipe samples were collected using AlphaWipes (Texwipe Inc., Kernersville, NC, USA) prewet with 70% isopropyl alcohol. A 5- × 6-cm template was used to collect skin wipe samples from the forehead, neck, and wrist. Wipe samples were taken on the left side of the forehead and neck and the left wrist for the pre- and post-fire samples. After decontamination, wipe samples were taken on the right side of the forehead and neck and the right wrist to ensure that removal of contaminants was strictly from the decontamination and not from previous skin surface sampling. The three wipes (i.e., neck, forehead, and wrist) were pooled together as one sample for each sampling period: pre-fire, post-fire, and post-decontamination.
Air samples
Personal air samples of at least one participant per fire were collected using the methodology employed previously (Keir et al. Citation2017). Briefly, GilAir Plus pumps (Levitt Safety, Ottawa, ON) ran at 2.5 L/min with a polyurethane foam cartridge (URG, Chapel Hill, NC) and a QM-A 25-mm quartz filter (Whatman, Maidstone, UK). The sampling pump was placed in the inside pocket of the PPE coat and connected to the sample collection cartridge using polypropylene tubing along the inside of the coat. The sample collection cartridge was affixed to the back of the collar of the Bunker gear coat with a hook-and-loop fastener. Sample collection started immediately before entering the structure and continued for the full duration of the fire; collection stopped upon exit of the structure. Pumps were calibrated before sampling using a Gilian Gilibrator-2 calibrator (Levitt Safety, Ottawa, ON) for quality control. Field and travel blanks were also collected and are further described in the supplementary information.
Air and wipe PAH analyses
The concentrations of the U.S. Environmental Protection Agency 16 priority PAHs, plus a few others, were determined for skin surface and air samples at the Laboratory for the Analysis of Natural and Synthetic Environmental Toxicants (LANSET), University of Ottawa. Air samples were analyzed as previously described in Keir et al. (Citation2020). Briefly, all samples were spiked with a recovery standard of five deuterated PAHs (i.e., naphthalene-d8, acenaphthene-d8, phenanthrene-d10, benzo(a)anthracene-d12, and perylene-d12; Cambridge Isotope Laboratories Inc., Tewksbury, MA). Air samples were extracted with dichloromethane using accelerated solvent extraction (ASE 200, Dionex Corporation, Sunnyvale, CA). Skin surface wipe samples were extracted via sonication twice with 15 mL of 3:1 hexane:acetone. Extracts from pooled wipes were evaporated to approximately 30 mL. High-performance liquid chromatography (HPLC)-grade water was added, and the organic layer was decanted off. Method blanks for both sample types were extracted following the same procedures. Extracts were concentrated under a gentle nitrogen stream to approximately 1 mL in 2,2′,4-trimethylpentane before being spiked with an internal standard (p-terphenyl-d14; Cambridge Isotope Laboratories, Tewksbury, MA). Samples were analyzed by gas chromatography (Agilent 7890B)–mass spectrometry (Agilent 5977B, Agilent Technologies, Santa Clara, CA, USA) on an HP-5MS UI 60-m, 0.25-μm, 250-μm column with selective ion monitoring. PAH concentrations were method blank corrected, then recovery corrected using the deuterated PAHs added to the samples at the time of extraction. High- and low-molecular-weight (MW) PAHs were calculated using the sum of PAHs with four or more rings or three or fewer rings, respectively.
Urine sampling and analyses
Spot urine samples were collected in sterile 120-mL polypropylene containers prior to fire training. Participants were instructed on proper technique to prevent contamination of the specimen. Post-fire urine samples were collected for 18 hours after fire training in 3-L Urisafe 24-hr urine collection containers (Simport Scientific, Saint-Mathieu-de-Boleil, QC) to collect most of the PAH metabolites excreted pertaining to the fire exposure (i.e., 3+ half-lives). Subjects were instructed to keep the 18-hr urine samples in the fridge. Spot urine samples were stored chilled until transported to the University of Ottawa, where aliquots were stored at −20 °C until analysis.
Urine aliquots were sent to the ISO/IEC 17025– and ISO/IEC 17043–accredited Human Toxicology Laboratory of the National Institute of Public Health of Quebec (INSPQ; Quebec City, QC, Canada) for analysis of urinary PAH metabolites via gas chromatography–tandem mass spectrometry (Gaudreau et al. Citation2016). Briefly, urinary metabolites were deconjugated with β-glucuronidase in pH 5.0 sodium acetate buffer, extracted twice with hexane, and derivatized with N-methyl-N-(trimethylsilyl) trifluoroacetamide. Samples were spiked with 25 μL of an internal standard solution (1-methoxyfluorene 50 μg/L in benzene) prior to injection. Nineteen urinary PAH metabolites were measured: 1-hydroxynaphthalene, 2-hydroxynaphthalene, 2-hydroxyfluorene, 3-hydroxyfluorene, 9-hydroxyfluorene, 1-hydroxyphenanthrene, 2-hydroxyphenanthrene, 3-hydroxyphenanthrene, 4-hydroxyphenanthrene, 9-hydroxyphenanthrene, 3-hydroxyfluoranthene, 1-hydroxypyrene, 1-hydroxybenz[a]anthracene, 3-hydroxybenz[a]anthrancene, 2-hydroxychrysene, 3-hydroxychrysene, 4-hydroxychrysene, 6-hydroxychrysene, and 3-hydroxybenzo[a]pyrene.
Urinary mutagenicity was measured using methodology employed previously (Keir et al. Citation2017). The Ames–Salmonella reverse mutation assay (i.e., the Ames test) was employed using Salmonella typhimurium strain YG1041 provided by Dr. Takehiko Nohmi (NIHS, Tokyo, Japan) in the presence of an exogenous metabolic activation mixture containing Aroclor-induced rat liver extract (Molecular Toxicology Inc., Boone, NC). Urine samples were filtered, enzymatically deconjugated, and concentrated using C18 solid-phase extraction with methanol elution. Five doses were tested; second assays were run with doses in the linear response range for initially positive assays. A simultaneous positive control (i.e., 0.075 μg/plate 2-aminoanthracene; Molecular Toxicology Inc.) and negative solvent control (i.e., dimethyl sulphoxide) were examined to ensure assay performance on each test day. Samples were incubated at 37 °C for 72 hr before the frequency of revertant (rev) colonies was scored. Mutagenic potency was calculated as the slope of the initial linear portion for samples that had a significant concentration–response (p < 0.05). Samples without a significant concentration–response were given a value of zero. Mutagenic potency is expressed as rev/mmoL of solute.
Urinary creatinine and osmolality concentrations were measured to correct for urinary dilution. Creatinine was measured using a Cayman Chemical (Ann Arbor, MI, USA) colorimetric assay kit (No. 500701) and osmolality using a VAPRO Vapor Pressure Osmometer (Model 5600). Osmolality was employed to correct for urinary dilution and kidney function; osmolality-correction has been shown to provide more robust adjustment of hydration compared to creatinine (Middleton et al. Citation2016).
Data analyses
PAHs, or PAH metabolites, with greater than 95% of samples below the detection limit were omitted from analyses and PAH sum determinations. If less than 20% of the values for a PAH were below the detection limit, nondetects were replaced with the limit of detection divided by the square root of 2. If more than 20% of the samples for a particular PAH were below the detection limit, nondetects were replaced with values calculated using Robust regression on order statistics using NDExpo Version 1.0 (http://expostats.ca/site/app-local/NDExpo/). The data were log-transformed where appropriate based on visual inspection of box and Q-Q plots and results of a Shapiro–Wilk test for normality. A paired t test was employed to investigate the effect of fire suppression (i.e., pre- and post-fire) for urinary PAH metabolites, urinary mutagenicity, and skin wipes and the effect of dermal decontamination (i.e., post-fire and post-decontamination differences) for skin wipes. Analysis of variance (ANOVA) with post-hoc multiple range testing (i.e., Duncan method) was used to determine mean exposure differences among the decontamination methods. Data were analyzed using SAS OnDemand for Academics (Release 3.8, SAS Institute, Cary, NC, USA). Differences were considered statistically significant when p < 0.05.
Results
Samples were collected from 88 firefighters during 21 training fires held on 8 days between October 2018 and November 2019. Participants (6 females, 82 males) were, on average, 34.4 years old and had 5.5 years of service. Details of participants’ demographics can be found in .
Table 1. Self-reported participant demographic information (N = 88).
Personal air samples
Twenty-seven personal air samples were collected (i.e., some fires included more than one participant wearing an air sampler). One sample was omitted from analyses due to technical difficulties. Air samples averaged a duration of 43.9 minutes (ranging from 29.7 to 61.7 minutes). The mean of total PAHs in air samples was 759 μg/m3 and ranged from 200 to 3,970 μg/m3. Naphthalene made up the largest proportion of the total PAHs followed by acenaphthylene and phenanthrene (). The average proportion of the known human carcinogen benzo[a]pyrene in air samples was 0.7% (SD = 0.4%, range = 0.2%–1.5%). Probable/possible carcinogenic PAHs (i.e., naphthalene, benz[a]anthracene, chrysene, benzo[b]fluoranthene, benzo[k]fluoranthene, indeno[1,2,3-cd]pyrene, and dibenz[a,h]anthracene) made up on average 51.2% (SD = 22.1%, range = 13.6%–79.2%) of measured PAHs. Results for air sample field and travel blanks can be found in Tables S1 and S2.
Table 2. Concentration of PAHs in personal air samples (N = 27) collected during fire training activities (in μg/m3).
Skin surface samples
Concentrations of PAHs from skin surface samples collected pre-fire, post-fire, and post-decontamination methods, stratified by decontamination method, are reported in Table S3. Total PAHs and high-MW PAH skin exposures significantly increased after fires (1.3- and 2.2-fold increase, p < 0.01 and < 0.0001, respectively). Participants who decontaminated with detergent and water had significantly higher skin exposures after fire exposure (p < 0.05) compared to the other groups (Figure S1). However, no significant differences were observed when naphthalene was removed from the total PAH concentrations. Fold change in PAH skin exposures following decontamination (i.e., concentration after decontamination/skin concentration before decontamination after fire exposure) shows that detergent and water removed the most PAHs, where decreases in all 14 individual PAHs with reportable levels were observed, 10 of them decreasing significantly and 6 significantly lower than the control group (). To determine which decontamination method was most effective, fold-change decreases in PAHs after decontamination (i.e., compared to post-fire) among the intervention groups were also compared. Intervention groups that had fold-change decreases significantly different than the control group are highlighted in . Overall, compared to the control group, detergent and water removed significantly more total PAHs, high-MW PAHs, acenaphthylene, fluoranthene, pyrene, retene, benz[a]anthracene, and chrysene. Wipe B was also significantly better than the control at removing high-MW PAHs and retene. Interestingly, the control had a higher fold-change decreases in naphthalene compared to participants using wipe A; in fact, participants using wipe A had, on average, higher levels of naphthalene on their skin post-decontamination compared to before.
Figure 1. Boxplots illustrating osmolality-corrected urinary concentrations of (A) 1-hydroxypyrene, (B) Ʃhydroxyphenanthrenes, (C) Ʃhydroxyfluorenes, and (D) Ʃhydroxynaphthalenes in firefighters before live fire training (pre-fire) and after live fire training (post-fire). The box limits represent the interquartile range (i.e., 25th to 75th percentile), the diamonds represent the mean value, the solid line represents the group median, and the whiskers extend to the 5th and 95th percentiles. The asterisks (***) indicate a significant difference between pre- and post-fire levels at p < 0.0001 using a paired t test.
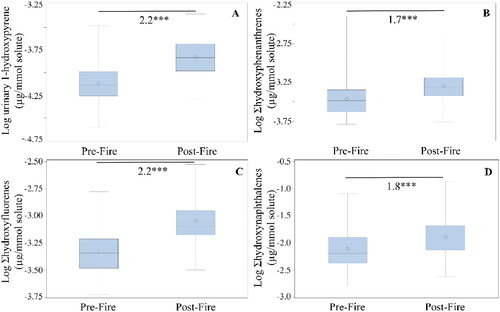
Table 3. Fold-change decreases post-decontamination compared to post-fire in PAHs on skin surface samples (N = 22).
Additional analyses were conducted to investigate the removal of the carcinogenic PAHs. Three possibly carcinogenic PAHs were measured in detectable amounts (i.e., chrysene, benz(a)anthracene, and benzo(b)fluoranthene). The fold change in the sum of the possibly carcinogenic PAHs for post-fire compared to post-decontamination skin wipes were calculated; the results obtained revealed that the three dermal decontamination intervention groups had significantly different fold changes compared to the control (i.e., removed more possible carcinogens compared to the control) (p < 0.05). Additional analyses investigated differences in skin wipe concentrations of the possibly carcinogenic PAHs post-fire compared to post-decontamination. Detergent and water removed a significant amount of possibly carcinogenic PAHs (i.e., mean difference of 0.01 ng/cm2, p < 0.001); wipe A results indicated a weak, significant difference (i.e., mean fold change of 0.007 ng/cm2, p = 0.045).
Urinary PAH metabolites
Eight metabolites had interfering values or were below the detection limit in more than 95% of the samples and were omitted from analyses (i.e., 1- and 3-hydroxybenz[a]anthracene, 2-, 3-, 4-, and 6-hydroxychrysene, 3-hydroxybenzo[a]pyrene, 3-hydroxyfluoranthene). The remaining 11 metabolites were grouped by their parent PAH (e.g., 1- and 2-hydroxynaphthalene reported as Ʃhydroxynaphthalenes). Background PAH metabolite levels (i.e., pre-fire samples) were not significantly different among participants who used different decontamination methods (p > 0.05; data not shown).
Significant increases in osmolality-corrected urinary PAH metabolites post-fire compared with pre-fire were found, ranging from 1.7- to 2.2-fold (p < 0.001) (). Significant increases were also observed in all four PAH metabolite groups for creatinine-corrected values (Table S8). No significant differences in urinary PAH metabolites were found among the three decontamination methods (p > 0.05) both for osmolality-corrected (Table S7) and creatinine-corrected data (Table S8), or between the decontamination methods compared to the control (p > 0.05, data not shown).
Mutagenicity analyses
Three pre-fire urine samples had insufficient sample volume, and one was too cytotoxic for bacteria to grow and was omitted from analyses. Only 29 of the 182 urine samples elicited a significant dose–response relationship. No significant difference in urinary mutagenicity was found overall after fire exposure (n = 172), for samples with a significant dose–response relationship (n = 29), or for paired pre- and post-fire samples with a significant dose–response relationship (n = 6). There were no significant differences in fold changes in urinary mutagenicity (i.e., pre- vs. post-fire) among decontamination methods (data not shown).
Discussion
PAH air concentrations during fires provided a snapshot of the environment firefighters were exposed to during the training exercises. The results herein were similar to previous studies of air PAH concentrations during live fire training or simulated fires, such as Kirk and Logan (Citation2015), that reported concentrations between 430 μg/m3 and 2,700 μg/m3, and such as Fent et al. (Citation2014), that reported concentrations less than 2,000 μg/m3 for one of the two sets of simulated fires they investigated. The other set of fires that Fent et al. (Citation2014) investigated was markedly higher, ranging from around 2,000 to 12,000 μg/m3. Banks et al. (Citation2021a) also reported higher air PAH concentrations than reported here, i.e., from live fire training using particle board where levels ranged from 75,000 to 180,000 μg/m3. Differences in air PAH concentrations among the various studies are likely a result of the fuel of the fires, fire temperatures and ventilation conditions (dependent on firefighting tactics), and role of the participant in fire suppression. Indeed, Banks et al. (Citation2021a) found firefighters’ personal PAH concentrations to differ depending on the fuel of the training fire they attended (i.e., particle board or diesel in a pan). Interestingly, air samples collected herein were notably higher than in a previous study that collected personal air samples at emergency fire events: a mean of 253 μg/m3 (Keir et al. Citation2020). The difference in means may be attributed to sample collection method; in the study of emergency fires, air samples were likely diluted as sample collection encompassed travel to and from the fire event. In contrast, sample collection for this study started immediately before entering the training fire and stopped immediately upon exiting the fire-containing structure. Additionally, participants in the present study were exposed while conducting interior fire suppression operations, whereas the study of emergency fires included firefighters at emergency fire events in multiple roles, both inside and outside structures. Keir et al. (Citation2020) also reported higher proportions of benzo[a]pyrene (1% vs. 0.7%) and possible and probable carcinogenic PAHs (77% vs. 51%). The difference in PAH makeup may be a result of fuel differences: Training fires utilize a single fuel type, whereas emergency fires are composed of multiple fuel types and complex mixtures. This is consistent with a study by Banks et al. (Citation2021a) that presented data indicating higher proportions of possible and probable carcinogenic PAHs in personal air from fires with different fuels (i.e., particleboard fires vs. diesel pan fires), calculated using their reported data.
The results obtained revealed that detergent and water were most effective at removing PAHs from skin compared to two types of wipes or the control (i.e., no dermal cleaning). There are several reasons that may explain why detergent and water were most effective. First, the surfactants and/or other ingredients in detergent and water may provide superior ability to remove PAHs from skin compared to the ingredients in the wipes (e.g., superior surfactants to remove the contaminants and/or the particles the contaminants are adsorbed to). Second, the textured material of the washcloths used in the detergent and water decontamination method may have provided superior removal of PAHs compared to the smooth, flat wipes. Third, although participants in the current study were allowed to use unlimited wipes or were given unlimited access to the bucket of detergent and water, unconscious bias may have occurred between those using wipes and those using detergent and water, leading to less washing in those using wipes. Perhaps the familiarity of a washcloth led to spending more time on dermal decontamination compared with using wipes. Indeed, research staff anecdotally noted that those using a washcloth tended to take longer to perform dermal decontamination compared with those who were given one of the two wipes. More research is required to determine whether there is an optimal wipe formulation for removal of a significant amount of firefighters’ post-fire dermal contamination. This could be useful for situations in which detergent and water dermal decontamination is logistically not possible (e.g., during winter or in remote areas during wildland firefighting).
To the best of our knowledge, this is the first study to comparatively evaluate the ability of several post-fire dermal decontamination methods to remove PAHs from skin and, moreover, to alter internal dose. Fent et al. (Citation2017) authored the only other study to have assessed the ability of post-fire dermal decontamination to remove PAHs from skin (but not internal dose). They found that baby wipes reduced PAHs on the neck of firefighters after firefighting by a median of 54%. Although the findings of Fent et al. (Citation2017) are similar for the detergent and water intervention group in the present study, the two commercial wipes used for the intervention groups in this study reported lower removal rates of PAHs compared to the wipes used in Fent et al. (Citation2017). The differences between the two studies are likely due to differences in the wipes used and the aforementioned reasons behind why various wipes or detergent and water protocols can differ in PAH removal efficiency. It is possible that other methods not yet investigated may be effective at removing dermally deposited PAHs and/or other organic mutagens and should be investigated moving forward.
The lack of difference between urinary PAH metabolite increases between the intervention groups and the control group show that the post-fire dermal cleaning steps did not have a measurable effect on the internal dose of PAHs that firefighters received during the live fire training exercise. It is likely that post-fire dermal decontamination efforts are too late to prevent absorption into the body. This assertion is supported by the dermal absorption fluxes of the parent PAHs of the metabolites measured (i.e., pyrene, phenanthrene, fluorene, and naphthalene). Using post-fire skin PAH concentrations as a proxy for skin PAH concentrations during fire suppression, these fluxes suggest that all bioavailable pyrene, phenanthrene, fluorene, and naphthalene dermally deposited on firefighters in this study would be absorbed within seconds to minutes (). Furthermore, because firefighters experience increased skin temperatures while fighting fires (Horn et al. Citation2018), which has been shown to increase skin permeability (Park et al. Citation2008), the absorption of PAHs during firefighting likely occurs faster than reported flux rates. This suggests that absorption of the parent PAHs would have occurred before the intervention was conducted. During the time prior to absorption, and/or for contaminants that take longer to absorb, dermal cleaning may also increase absorption by hydrating the skin (Maibach Citation2021). This “wash-in” effect, where chemicals are absorbed more readily into the skin with washing, has been reported for different chemicals including benzo[a]pyrene, N,N-diethyl-m-toluamide (DEET), and 1,1,1-trichloro-2,2-bis(4-chlorophenyl)ethane (DDT; Moody et al. Citation1994, Citation1995a, Citation1995b). In fact, Moody and Maibach (Citation2006) reported that the wash-in effect may increase both cutaneous and general systemic toxicity; they recommend caution when practicing skin washing for substance removal. More specifically, the authors recommend understanding the mechanisms of absorption to identify interventions that decrease occupational exposure and, relatedly, exposure-caused occupational disease, so as to avoid a wash-in effect. That being said, post-fire dermal cleaning may be effective at reducing high-MW PAHs (and other slower-absorbing compounds) as they have lower fluxes, taking longer to penetrate the skin and absorb into the body (Silva et al. Citation2021). It is possible that post-fire dermal cleaning reduced absorption and cross-contamination of compounds left on the surface of the skin. However, because high-MW PAHs are excreted via feces rather than urine, any differences in internal dose of high-MW PAHs could not be measured (Motorykin et al. Citation2015). Future work should investigate the influence of dermal cleaning on internal dose of larger PAHs using analyses of blood or fecal samples.
Table 4. Calculation of absorption time for parent PAHs of the urinary metabolites measured.
No significant differences in urinary mutagenicity were seen, contrary to previous studies that found significant increases in urinary mutagenicity for firefighters attending emergency, residential fires, and wildland prescribed burns (Keir et al. Citation2017; Wu et al. Citation2021). The lack of significant change in urinary mutagenicity observed here suggests that the firefighters were not exposed to levels of mutagens that could be detected using the Salmonella reverse mutation test (i.e., Ames test). The lower exposure could be due to different and/or lower amounts of fuel used at the fire (i.e., particle board), temperature differences, shorter duration of fire suppression activity compared to previous studies, and/or their PPE and exposure prevention methods effectively working to reduce exposure to combustion-derived mutagens. It is also possible that exposure to larger mutagens (e.g., benzo(a)pyrene) were not detected due to their excretion through pathways other than urine (e.g., fecal excretion). Future work could scrutinize the lack of detection of mutagens in urine and assess situations where more substantial exposures may occur, such as staged burns with household contents or fires of longer duration, that provide higher exposures to mutagens that could be detected via the Ames test; moreover, any differences related to the use of various dermal decontamination methods could also be examined.
The results of the influence of dermal decontamination on skin surface contamination reported herein and by Fent et al. (Citation2017) focused only on PAHs, yet firefighters can be exposed to hundreds of different chemicals and the efficacy of dermal decontamination can change with different compounds. It is important to utilize exposure biomonitoring proxies that encompass exposure to a range of compounds. Urinary mutagenicity remains a useful tool to determine overall exposure to mutagens without having a priori information on the identity of the combustion-derived mutagens in complex mixtures of combustion emissions and should continue to be utilized. Other exposure biomonitoring proxies that encompass exposure to a range of compounds should also be deployed. For example, Grashow et al. (Citation2020) used untargeted analyses in serum samples from female firefighters and office workers in San Francisco and detected phthalate metabolites, phosphate flame-retardant metabolites, phenols, pesticides, nitro and nitroso compounds, and per- and polyfluoroalkyl substances. Other options for measuring the influence of dermal decontamination on exposure could include use of assays to examine, for example, induced mutations and/or chromosomal damage in blood cells and/or urine-derived epithelial cells. Careful consideration of toxicokinetics would be required to ensure proper timing of sample collection to see any possible influences of dermal decontamination on exposure and/or effect.
Conclusions
It has been suggested that removal of combustion-derived carcinogens from firefighters’ skin might reduce exposure. Of the three on-site decontamination procedures examined in this study, dish detergent and water was much more effective than the two commercially available skin-cleaning wipes and significantly removed approximately half of the PAHs deposited on skin. Despite the effectiveness of the various decontamination methods for removal of PAHs from the skin surface, these differences were not reflected in the measured PAH metabolites in urine. Therefore, the present data indicate that even early attempts to remove PAHs from firefighters’ skin do not reduce the internal dose received, likely because PAHs traverse the skin prior to decontamination efforts. Rather than addressing postexposure efforts, future work should focus on preventing initial exposure through improved compliance in use and quality of PPE, such as novel textiles and/or improved fit of PPE to prevent passage of combustion by-products onto skin.
Supplemental Material
Download MS Word (179.3 KB)Acknowledgements
This study was funded by the Canadian Forces Fire Marshal, the International Association of Fire Fighters, Health Canada, the Ottawa Professional Firefighters Association, the Association des pompiers de Montréal, the Institut de protection contre les incendies du Québec (IPIQ), and the Natural Sciences and Engineering Research Council of Canada (NSERC). In-kind contributions were provided by the Ottawa Fire Services (OFS) and Health Canada. We are grateful to the OFS for their collaboration and participation. We thank Deputy Chief Dave Matschke for help with initial planning of the study, retired Division Chief Peter McBride for helping with acquiring funding and coordination of the study, and Greg Lough and Brad Bignucolo for scheduling and on-scene coordination. This study was approved by the Ethics Reviews Boards at the University of Ottawa and Health Canada.
Data availability statement
Data are not publicly available due to the privacy of the human participants involved.
Disclosure statement
The authors report there are no competing interests to declare.
References
- Banks APW, Thai P, Engelsman M, Wang X, Osorio AF, Mueller JF. 2021a. Characterising the exposure of Australian firefighters to polycyclic aromatic hydrocarbons generated in simulated compartment fires. Int J Hyg Environ Health. 231:113637. doi:10.1016/j.ijheh.2020.113637
- Banks APW, Wang X, Engelsman M, He C, Osorio AF, Mueller JF. 2021b. Assessing decontamination and laundering processes for the removal of polycyclic aromatic hydrocarbons and flame retardants from firefighting uniforms. Environ Res. 194:110616. doi:10.1016/j.envres.2020.110616
- Bolstad-Johnson DM, Burgess JL, Crutchfield CD, Storment S, Gerkin R, Wilson JR. 2000. Characterization of firefighter exposures during fire overhaul. Am Ind Hyg Assoc J. 61(5):636–641. doi:10.1202/0002-8894(2000)061<0636:COFEDF>2.0.CO;2
- Casjens S, Brüning T, Taeger D. 2020. Cancer risks of firefighters: a systematic review and meta-analysis of secular trends and region-specific differences. Int Arch Occup Environ Health. 93(7):839–852. doi:10.1007/s00420-020-01539-0
- Chang SK, Riviere JE. 1991. Percutaneous absorption of parathion in vitro in porcine skin: effects of dose, temperature, humidity, and perfusate composition on absorptive flux. Toxicol Sci. 17(3):494–504. doi:10.1093/toxsci/17.3.494
- Cherry N, Galarneau JM, Kinniburgh D, Quemerais B, Tiu S, Zhang X. 2021. Exposure and absorption of PAHs in wildland firefighters: a field study with pilot interventions. Ann Work Expo Health. 65(2):148–161. doi:10.1093/annweh/wxaa064
- Demers PA, DeMarini DM, Fent KW, Glass DC, Hansen J, Adetona O, Andersen MH, Freeman LEB, Caban-Martinez AJ, Daniels RD, et al. 2022. Carcinogenicity of occupational exposure as a firefighter. Lancet Oncol. 23(8):985–986. doi:10.1016/S1470-2045(22)00390-4
- Fent KW, Alexander B, Roberts J, Robertson S, Toennis C, Sammons D, Bertke S, Kerber S, Smith D, Horn G. 2017. Contamination of firefighter personal protective equipment and skin and the effectiveness of decontamination procedures. J Occup Environ Hyg. 14(10):801–814. doi:10.1080/15459624.2017.1334904
- Fent KW, Eisenberg J, Snawder J, Sammons D, Pleil JD, Stiegel MA, Mueller C, Horn GP, Dalton J. 2014. Systemic exposure to PAHs and benzene in firefighters suppressing controlled structure fires. Ann Occup Hyg. 58(7):830–845. doi:10.1093/annhyg/meu036
- Fent KW, Toennis C, Sammons D, Robertson S, Bertke S, Calafat AM, Pleil JD, Wallace MAG, Kerber S, Smith D, et al. 2020. Firefighters’ absorption of PAHs and VOCs during controlled residential fires by job assignment and fire attack tactic. J Expo Sci Environ Epidemiol. 30(2):338–349. doi:10.1038/s41370-019-0145-2
- Fernando S, Gallea M, VandenEnden L, House R, Verma D, Shaw L, McCarry BE, Shaw D, Britz-McKibbin P. 2016. Evaluation of firefighter exposure to wood smoke during training exercises at burn houses. Environ Sci Technol. 50(3):1536–1543. doi:10.1021/acs.est.5b04752
- Gaudreau É, Bérubé R, Bienvenu J-F, Fleury N. 2016. Stability issues in the determination of 19 urinary (free and conjugated) monohydroxy polycyclic aromatic hydrocarbons. Anal Bioanal Chem. 408(15):4021–4033. doi:10.1007/s00216-016-9491-2
- Golka K, Weistenhöfer W. 2008. Fire fighters, combustion products, and urothelial cancer. J Toxicol Environ Health B Crit Rev. 11(1):32–44. doi:10.1080/10937400701600396
- Grashow R, Bessonneau V, Gerona RR, Wang A, Trowbridge J, Lin T, Buren H, Rudel RA, Morello-Frosch R. 2020. Integrating exposure knowledge and serum suspect screening as a new approach to biomonitoring: an application in firefighters and office workers. Environ Sci Technol. 54(7):4344–4355. doi:10.1021/acs.est.9b04579
- Hoppe-Jones C, Griffin SC, Gulotta JJ, Wallentine DD, Moore PK, Beitel SC, Flahr LM, Zhai J, Zhou JJ, Littau SR, et al. 2021. Evaluation of fireground exposures using urinary PAH metabolites. J Expo Sci Environ Epidemiol. 31(5):913–922. doi:10.1038/s41370-021-00311-x
- Horn GP, Kesler RM, Kerber S, Fent KW, Schroeder TJ, Scott WS, Fehling PC, Fernhall B, Smith DL. 2018. Thermal response to firefighting activities in residential structure fires: impact of job assignment and suppression tactic. Ergonomics. 61(3):404–419. doi:10.1080/00140139.2017.1355072
- IARC. 2010. Some non-heterocyclic polycyclic aromatic hydrocarbons and some related exposures. IARC Monogr Eval Carcinog Risks Hum. 92:1–853.
- Jalilian H, Ziaei M, Weiderpass E, Rueegg CS, Khosravi Y, Kjaerheim K. 2019. Cancer incidence and mortality among firefighters. Int J Cancer. 145(10):2639–2646. doi:10.1002/ijc.32199
- Jones K, Cocker J, Dodd LJL, Fraser I. 2003. Factors affecting the extent of dermal absorption of solvent vapours: a human volunteer study. Ann Occup Hyg. 47(2):145–150.
- Keir JLA, Akhtar U, Matschke DMJ, Kirkham TL, Chan HM, Ayotte P, White PA, Blais JM. 2017. Elevated exposures to polycyclic aromatic hydrocarbons and other organic mutagens in Ottawa firefighters participating in emergency, on-shift fire suppression. Environ Sci Technol. 51(21):12745–12755. doi:10.1021/acs.est.7b02850
- Keir JLA, Akhtar US, Matschke DMJ, White PA, Kirkham TL, Chan HM, Blais JM. 2020. Polycyclic aromatic hydrocarbon (PAH) and metal contamination of air and surfaces exposed to combustion emissions during emergency fire suppression: implications for firefighters’ exposures. Sci Total Environ. 698:134211. doi:10.1016/j.scitotenv.2019.134211
- Kirk KM, Logan MB. 2015. Firefighting instructors’ exposures to polycyclic aromatic hydrocarbons during live fire training scenarios. J Occup Environ Hyg. 12(4):227–234. doi:10.1080/15459624.2014.955184
- Laitinen J, Mäkelä M, Mikkola J, Huttu I. 2012. Firefighters’ multiple exposure assessments in practice. Toxicol Lett. 213(1):129–133. doi:10.1016/j.toxlet.2012.06.005
- Maibach HI. 2021. Dermal decontamination and percutaneous absorption. Boca Raton (FL): CRC Press. p. 315–326. https://www.taylorfrancis.com/books/edit/10.1201/9780429202971/percutaneous-absorption-nina-dragi%C4%87evi%C4%87-howard-maibach?refId=f7bb7121-51d6-455b-98d2-40b7101a7f29&context=ubx
- Middleton DRS, Watts MJ, Lark RM, Milne CJ, Polya DA. 2016. Assessing urinary flow rate, creatinine, osmolality and other hydration adjustment methods for urinary biomonitoring using NHANES arsenic, iodine, lead and cadmium data. Environ Heal. 15:68.
- Moody RP, Maibach HI. 2006. Skin decontamination: importance of the wash-in effect. Food Chem Toxicol. 44(11):1783–1788. doi:10.1016/j.fct.2006.05.020
- Moody RP, Nadeau B, Chu I. 1994. In vitro dermal absorption of pesticides: VI. In vivo and in vitro comparison of the organochlorine insecticide DDT in rat, guinea pig, pig, human and tissue-cultured skin. Toxicol Vitr. 8(6):1225–1232. doi:10.1016/0887-2333(94)90113-9
- Moody RP, Nadeau B, Chu I. 1995a. In vitrodermal absorption of N,N-diethyl-m-toluamide (DEET) in rat, guinea pig, and human skin. Vitr Toxicol J Mol Cell Toxicol. 8:263–275.
- Moody RP, Nadeau B, Chu I. 1995b. In vivo and in vitro dermal absorption of benzo[a]pyrene in rat, guinea pig, human and tissue-cultured skin. J Dermatol Sci. 9(1):48–58.
- Motorykin O, Santiago-Delgado L, Rohlman D, Schrlau JE, Harper B, Harris S, Harding A, Kile ML, Simonich SLM. 2015. Metabolism and excretion rates of parent and hydroxy-PAHs in urine collected after consumption of traditionally smoked salmon for Native American volunteers. Sci Total Environ. 514:170–177. doi:10.1016/j.scitotenv.2015.01.083
- Park JH, Lee JW, Kim YC, Prausnitz MR. 2008. The effect of heat on skin permeability. Int J Pharm. 359(1–2):94–103. doi:10.1016/j.ijpharm.2008.03.032
- Silva J, Marques-da-Silva D, Lagoa R. 2021. Reassessment of the experimental skin permeability coefficients of polycyclic aromatic hydrocarbons and organophosphorus pesticides. Environ Toxicol Pharmacol. 86:103671. doi:10.1016/j.etap.2021.103671
- Soteriades ES, Kim J, Christophi CA, Kales SN. 2019. Cancer incidence and mortality in firefighters: a state-of-the-art review and meta-analysis. Asian Pac J Cancer Prev. 20(11):3221–3231. doi:10.31557/APJCP.2019.20.11.3221
- Wingfors H, Nyholm JR, Magnusson R, Wijkmark CH. 2018. Impact of fire suit ensembles on firefighter PAH exposures as assessed by skin deposition and urinary biomarkers. Ann Work Expo Health. 62(2):221–231. doi:10.1093/annweh/wxx097
- Wu CM, Warren SH, Demarini DM, Song CC, Adetona O. 2021. Urinary mutagenicity and oxidative status of wildland firefighters working at prescribed burns in a Midwestern US forest. Occup Environ Med. 78(5):315–322. doi:10.1136/oemed-2020-106612