abstract
A persistent clinical demand exists for a suitable arterial prosthesis. In this study, a vascular conduit mimicking the native 3-layered artery, and constructed from the extracellular matrix proteins type I collagen and elastin, was evaluated for its performance as a blood vessel equivalent.
A tubular 3-layered graft (elastin-collagen-collagen) was prepared using highly purified type I collagen fibrils and elastin fibers, resembling the 3-layered native blood vessel architecture. The vascular graft was crosslinked and heparinised (37 ± 4 μg heparin/mg graft), and evaluated as a vascular graft using a porcine bilateral iliac artery model. An intra-animal comparison with clinically-used heparinised ePTFE (Propaten®) was made. Analyses included biochemical characterization, duplex scanning, (immuno)histochemistry and scanning electron microscopy.
The tubular graft was easy to handle with adequate suturability. Implantation resulted in pulsating grafts without leakage. One week after implantation, both ePTFE and the natural acellular graft had 100% patencies on duplex scanning. Grafts were partially endothelialised (Von Willebrand-positive endothelium with a laminin-positive basal membrane layer). After one month, layered thrombi were found in the natural (4/4) and ePTFE graft (1/4), resulting in occlusion which in case of the natural graft is likely due to the porosity of the inner elastin layer. In vivo application of a molecularly-defined tubular graft, based on nature's matrix proteins, for vascular surgery is feasible.
Introduction
Vascular diseases, and atherosclerosis in particular, are a major cause of mortality in Western society.Citation1 Therefore, vascular grafts from synthetic polymers have been introduced, including expanded polytetrafluoroethylene (ePTFE) and Dacron (polyethylene terephthalate).Citation2 Application of these polymers as small diameter grafts proves challenging due to potential occlusion in the long term,Citation3,4 and limited tissue response e.g. in the case of endothelialisation possibly due to the graft's porosity and (ultra)structural appearances.Citation5 As a result, research has turned to the field of regenerative medicine including the application of natural molecules.Citation6
The main components of the native vascular wall include the extracellular matrix proteins elastin and type I collagen, which supply elasticity and strength, respectively. Elastic fibers are prominently present in arteries to facilitate blood flow and propagation of pressure waves. Elastin is primarily found in the intima and media, whereas type I collagen is most prominent in the media and adventitia.Citation7 Current examples of clinically applied collagenous natural vascular grafts include, but are not limited to, Omniflow®Citation8 and Artegraft.Citation9 The latter consists of a chemically processed and dialdehyde treated bovine carotid artery.Citation10 Omniflow® consists of a synthetic polymer mesh enwrapped with ovine collagen, which was obtained after mesh implantation in sheep followed by explantation, decellularization and glutaraldehyde tanning.Citation8 Applications of such natural vascular grafts are as peripheral bypass, in vascular access/repair, and for arteriovenous shunting in hemodialysis.Citation11 Next to fibrillar structures as components of grafts, glycosaminoglycans such as heparin/heparan sulfate have been applied. Glycosaminoglycan-based grafts, such as hyaluronan-based tubular constructs, have been used for grafting in rat aorta and porcine carotid arteries,Citation12,13 resulting in deposition of circumferentially organized elastic fibers.Citation13 The glycosaminoglycan heparin, a well-known anti-coagulant, has been coated on synthetic grafts which are used in clinical practice.Citation3,14,15 An example of such a heparinised ePTFE graft is Propaten® (GoreTex), indicated for (below-knee) peripheral surgery when autologous vein is unavailable.Citation16 Another component, elastin, has been indicated as a nonthrombogenic biomaterialCitation17,18 and has also been used in the preparation of vascular natural grafts.Citation19-22 Bi-, tri, and multilayered grafts,Citation23-28 generally obtained by electrospinning or casting/molding techniques, have been proposed to mimic the native arterial architecture, and grafts incorporating soluble collagen, elastin, synthetic polymers and/or growth factors, collagen-mimeticsCitation29 or elastin/collagen analogsCitation30 have been prepared. However, in multiple reported cases, the electrospun materials' small pores and dense structure may have limited cell ingrowth and thus tissue remodelling.Citation24,25,31
Previously, we reported on the controlled construction of an elastin and collagen-based conduit from scratch, containing highly-purified fibrillar type I collagen and elastin fibers.Citation32 This work demonstrated the compatibility of the constructed natural graft from a biochemical/biophysical, mechanical and haemocompatible point of view, with mechanical properties comparable to human tissue and not inducing platelet aggregation. Only few fibrillar natural grafts have been constructed containing purified insoluble elastin fibers on the luminal side. Berglund et al. Citation20 made construct-sleeve hybrids from arterial elastin and constructed a collagen support sleeve for improved strength in order to sustain arterial pressures of the blood flow. Hinds et al.Citation21 tested grafts from purified arterial elastin and acellular small intestinal submucosa. In their study the natural grafts showed improved patency in a porcine interposition model compared to ePTFE. The objective of the presented study was to evaluate the feasibility of this conduit in a porcine model of interposition grafting. Numerous animal models have been used in the field of cardiovascular tissue engineering.Citation33 We have chosen the porcine arterial system as it is highly comparable to the human situation.Citation34 The graft was evaluated using biochemical characterization, duplex scanning, (immuno)histological examination and scanning electron microscopy. Intra-animal comparison was made to Propaten®, a heparin containing ePTFE graft. Despite recent progress in this area, limited long-term experimental (large) animal data is available with respect to vascular grafts composed of natural components only. In addition, most grafts are prepared by decellularisation and/or washing procedures of native vessels, and may harbour contaminating components precluding the study of the effect of single components. Batch-to-batch variation in the isolation/purification of the used natural raw materials (i.e. collagen and elastin) may be less in comparison to a decellularisation procedure because of e.g., the high degree of standardization of washings and pulverization of the starting material. Besides, decellularization may leave chemical remnants deep within the preserved native vascular construction. Therefore, the chosen approach, i.e., the construction of a layered elastin-collagen graft from scratch, provides a measure of controllability and tunability with regards to the graft's composition, thickness and length.
Materials and Methods
Unless stated otherwise, all chemicals were purchased from Merck Chemicals (Darmstadt, Germany).
Vascular graft construction
Elastin fibers were purified from pulverised equine ligamentum nuchae using extractions with solutions of salt, organic solvents, 8% CNBr in formic acid, 2-mercaptoethanol and trypsin, as described previously.Citation35 Type I collagen fibrils were obtained from pulverised bovine Achilles tendon by extractions using solutions of salt, urea, acetic acid, acetone and demineralised water, as described previously.Citation36
Tubular three-layered vascular conduits (elastin-collagen-collagen) were constructed from these natural materials as previously described.Citation32 Briefly, elastin fibers and type I collagen were applied in layers onto 4 mm poly-ether-ether-ketone mandrels by a tubular sequential molding, freezing and freeze-drying process. An elastin luminal layer was cast and lyophilized first, then a single collagen layer was cast and dried, followed by another third collagen layer and subsequent lyophilization. Grafts were chemically crosslinked with 1-ethyl-3-dimethyl aminopropyl carbodiimide (EDC) and N-hydroxysuccinimide (NHS) in the presence of 0.25% heparin (Diosynth, Akzo Nobel, Oss, The Netherlands). Grafts with 4 mm inner diameter were cut to length (3.5 cm) and disinfected by 4 washings with 70% ethanol (for one hour each) followed by washings with sterile phosphate buffered saline (pH 7.4).Heparin-coated, 4 mm diameter ePTFE grafts (Propaten, Gore-Tex, Flagstaff, Arizona, USA), from here on referred to as ePTFE, were disinfected similarly.
Biochemical characterization
Scaffolds were biochemically characterized for crosslinking by determining the amount of primary amine groups using 2,4,6-trinitrobenzene sulfonic acid (TNBS) assays.Citation37 The amount of heparin bound to the scaffold was determined by hexosamine analysis.Citation36
Animal welfare and ethics statement
The animal experiment was approved by the Ethics Committee of the Radboud University Medical Center (RUMC), the Netherlands (permit number 20091 DEC 2008–021 / CDL 080171). Animal welfare and care were in accordance to the NIH guidelines for the care and use of laboratory animals (NIH publication 85–23 Rev. 1985), and was carried out by the Central Animal Laboratory, RUMC. Animals were housed together, on straw and/or hay, and kept at a standard day and night light regime. The animals were fed a standard diet and water ad libitum, and were regularly observed by biotechnicians for appetite, wound healing and mobility. Directly after surgery, animals were housed separately as their mobility was low due to surgery. After one week mobility improved and animals were housed in groups.
Surgical procedure and implantation
Six pigs (57 ± 7 kg, female, Dutch Landrace) were housed in the laboratory at least one day prior to bilateral iliac artery interposition. All animals (6 in total) were tested negative for methicillin resistant Staphylococcus aureus. One day before surgery, animals were orally medicated with Plavix (clopidogrel, 1.5 mg/kg, Sanofi-Avents, Paris, France) and Ascal (carbasalate calcium, 4 mg/kg, Meda Pharma, Amstelveen, the Netherlands). Both Plavix (0.75 mg/kg) and Ascal (2 mg/kg) were also administered before surgery (day 0) and 2 d after. From day 3 to 28, animals received Plavix daily (1.5 mg/kg).
At the day of surgery, animals were sedated intramuscularly with ketamine (10 mg/kg)(Eurovet Animal Health BV, Bladel, The Netherlands), atropine (50 µg/kg)(Pharmachemie BV, Haarlem, The Netherlands) and midazolam (1 mg/kg)(Roche, Woerden, The Netherlands). After intravenous administration of propofol (2–3 mg/kg)(B. Braun Melsungen AG, Melsungen, Germany), animals were intubated, placed upon a heating pad in the supine position, and connected to a respiration machine (Ohmeda Modilus 2 plus, Ohmeda, Hoevelaken, the Netherlands). Further anesthesia was performed with 0.5% isoflurane (Baxter International, Deerfield, IL, USA) and an intravenous bolus administration of sufentanil (5 µg/kg)(Janssen-Cilag BV, Tilburg, The Netherlands) and vecuronium (0.2 mg/kg)(Norcuron, Organon, Oss, The Netherlands). Anesthesia was maintained during surgery with midazolam (0.6 mg/kg/h), sufentanil (10 µg/kg/h), atropine (25 µg/kg) and vecuronium (0.4 mg/kg/h) (i.v.). Continuous monitoring included electrocardiography, pulse oximetry, and measurements for CO2 and temperature. Bypass surgery was performed by experienced vascular surgeons (AK and JvdV). The right iliac artery was freed from surrounding tissue (); papaverine was used to prevent spasm of the artery. After heparinisation (10 000 U i.v.), the artery was clamped proximally and distally over a length of approximately 5 cm. The artery was removed over a length of 3.5 cm for interposition of the natural graft or ePTFE. Proximal and distal anastomoses were made with running sutures (CV-7, Gore-Tex) (). After testing the anastomoses for possible leakage (by clamp loosening), clamps were removed, the blood flow resumed, and the graft was wrapped with haemostatic material (oxidised regenerated cellulose, Surgicel®, Johnson & Johnson Medical Ltd, Gargrave, North Yorkshire, UK) (), most commonly occurring in case of suturing ePTFE. Next, a second and separate inguinal incision was made to expose the contralateral or left iliac artery and the procedure was repeated using ePFTE (Propaten) as the interposition conduit (). After closure, Fraxiparine® was administered intramuscularly (5 700 IE anti Xa, GlaxoSmithKline, Zeist, The Netherlands). The muscle layer and skin were closed with 2.0 Vicryl sutures.
Figure 1. Macroscopic view and scanning electron micrographs of the triple-layered natural graft and the control graft Propaten (ePTFE). (A) macroscopic view. (B-C) cross-sections, (D-E) luminal surface morphology. The natural graft displays a 3 layered architecture with an inner elastin layer (B-1), an intermediate porous/lamellar type I collagen film for strength (B-2) and a porous outer type I collagen layer (B-3). In the PTFE graft the wall (C-1) and the supporting ring (C-2) are seen. Scanning electron microscopy reveals differences in wall thicknesses and porosity between both grafts. Luminal lining of the natural graft consists of elastin fibers (D), whereas the ePTFE graft displays lamellae with perpendicular struts (E). Bars represent 100 µm (B-C) and 10 µm (D-E). L: lumen.
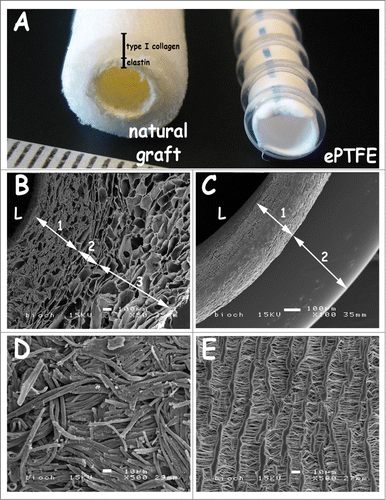
Figure 2. Overview of the surgical procedure. (A) The iliac artery freed from adherent tissue. (B) Suturing of the natural graft proximally with running sutures. (C) Anastomised graft with running sutures. (D) Interpositioned graft after clamp removal, and Surgicel® placement. Please note resumed blood flow in supplemental movie. (E) Interpositioned ePTFE (Propaten) used as control graft. p: proximal, d: distal.
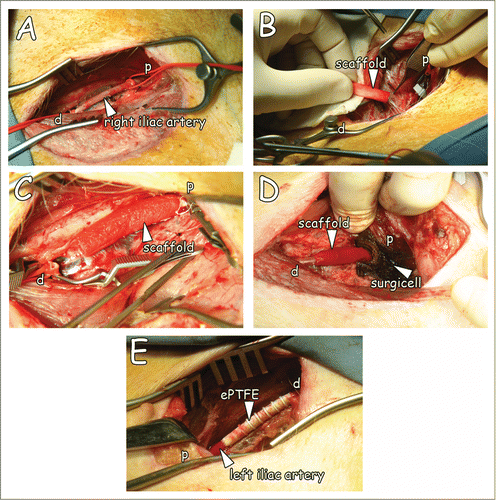
Duplex scanning
Duplex scannings (IU22, Philips, Eindhoven, the Netherlands) were performed directly after surgery (day 0) and at day 7 and 28. Animals were sedated by intramuscular administration of ketamine (10 mg/kg), atropine (50 µg/kg) and midazolam (1 mg/kg), and placed upon a heating pad in the supine position. Graft patencies were determined by measuring peak systolic velocities (PSVs) proximally, within and distally to the graft and in both anastomoses. When flow velocities could not be measured accurately as was the case when severe turbulence occurred, the diameter ratio of graft and artery were used to determine the percentage of narrowing.
Tissue explantation and processing
Seven or 28 d after conduit implantation, animals were sedated with ketamine (10 mg/kg), atropine (50 µg/kg) and midazolam (1 mg/kg), and placed in the supine position. Heparinization (10 000 U) preceded sacrifice by an overdose of barbiturate to prevent post mortem blood coagulation. Both grafts were excised with adherent proximal and distal arteries. Then, grafts were cut open longitudinally and divided into samples for later analyses. One longitudinal piece was divided in 2, fixed in 4% paraformaldehyde for 16 h and embedded in paraffin. The other longitudinal piece was divided; anastomoses were snap frozen with liquid nitrogen cooled isopentane, and central pieces were fixed with 2% glutaraldehyde (16 h, 4°C) for scanning electron microscopy.
(Immuno)Histological examination
Paraffin sections were cut (5 µm) and haematoxylin and eosin staining (H&E) was used to evaluate general histology. Elastin von Gieson staining (EVG) was used to visualize collagen (pink) and elastin (black). Elastin Masson Trichrome staining was applied to visualize collagen (green), elastin (black) and cells (red). Presence of calcified deposits was analyzed by alizarin red staining.Citation38
Immunohistochemistry was performed for smooth muscle actin (SMA), factor VIII, laminin and von Willebrand factor (VWF). In brief, paraffin sections were deparaffinised in xylene and hydrated in a degrading series of ethanol. Antibody retrieval was performed by treatment with 10 mM Tris/1 mM EDTA pH 9.0 for 40 min (95°C)(SMA), or 0.1% pronase in PBS pH 7.4 at 37°C for 10 min (factor VIII) or 30 min (laminin). For VWF staining no pre-treatment was performed. After blocking endogenous peroxidase activity with 0.3% H2O2, sections were incubated for 16 h at 4°C with polyclonal rabbit anti-human VWF (1:100, DAKO, Glostrup, Denmark), monoclonal mouse anti-human α-smooth muscle actin (1:400, Sigma-Aldrich, Steinheim, Germany), polyclonal rabbit anti-human factor VIII (1:1200, Sanquin, Amsterdam, the Netherlands) or polyclonal rabbit anti-mouse laminin (1:50, Sigma). After PBS washings, sections were incubated for 1 h with horse anti-mouse biotinylated secondary antibody (1: 400, Vectastain Elite ABC-kit, Vectorlabs, Burlingame, CA, USA) or donkey anti-rabbit biotinylated secondary antibody (1:200, Jackson ImmunoResearch Inc.., West Grove, PA, USA). After PBS washings, sections were developed with 3,3′-diaminobenzidine (DAB) as a chromogen. In the case of factor VIII, sections were developed using power DAB (Immunologic, Duiven, the Netherlands). Sections were dehydrated in an increasing series of ethanol and mounted with entellan. (Immuno)histochemical sections were analyzed using a Zeiss Axioscop (Oberkochen, Germany).
Scanning electron microscopy (SEM)
SEM was used to analyze the morphology and (ultra)structure of the grafts. Glutaraldehyde-fixed samples were post-fixed with osmium tetroxide (1 h), washed for 3 times with 0.1 M phosphate buffer (pH 7.4), and subsequently taken through a series of 30%, 50%, 70% and 100% ethanol washings. Next, samples were critically point dried and fixed on a stub with double-sided carbon tape and sputtered with an ultrathin gold layer (using Polaron E5100 SEM Coating System, East Sussex, UK). Examination was performed in a JEOL SEM 6310 (Tokyo, Japan) at an accelerating voltage of 15 kV.
Results
Vascular graft construction
Macroscopic and scanning electron microscopic images of the grafts are shown in . Wall thicknesses of the natural triple-layered and of the ePTFE grafts were approximately 1.5 and 0.25 mm, respectively (). The natural graft harboured a 3-layered architecture with an inner layer of elastin (), an intermediate layer of porous/lamellar type I collagen film () and an outer layer of a porous type I collagen scaffold ().Citation32 ePTFE grafts contained supporting rings (0.4 mm in thickness) . The luminal lining of the natural graft consisted of elastin fibers (diameter ∼5 µm, ). ePTFE grafts contained fibrils and nodes with lamellae on the luminal side ().
Figure 3. Representative duplex scannings from day 0, week 1 and week 4 of the natural and ePTFE graft (left and right column, respectively). Both grafts were open 1 week after surgery (A-D). The natural grafts (4/4) and one ePTFE (1/4) were occluded 4 weeks after surgery (E-F, white arrow, occluded natural graft and open ePTFE). In some cases, the view at day 0 was blocked due to placement of Surgicel®, recognized by black shading (B, white arrow). Measurements were performed proximally (A, B), within the graft (C, D) and distally (E, F). Quantitative values are listed in .
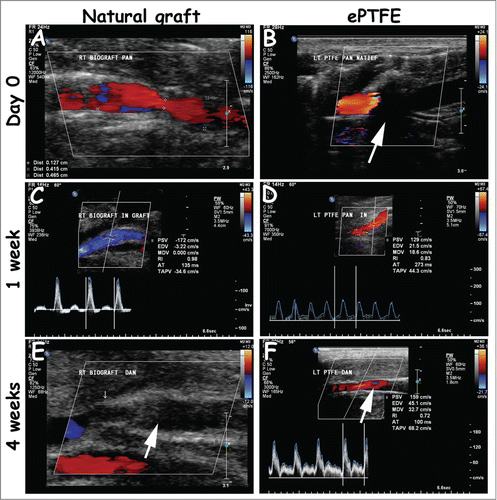
Table 1. Duplex scanning measurements of blood velocities in natural graft and ePTFE interposed in iliac arteries. The constructed natural graft was placed in the right iliac artery and the control graft Propaten (ePTFE) in the left iliac artery. Peak systolic velocities (PSV) were measured (cm/s) in the native proximal and distal artery. Additionally, velocity was measured in both anastomoses and within the graft itself. In some cases, velocity measurements performed directly after surgery (day 0) were hindered by the placement of Surgicel® and were scored n.d. for not determined. Normal PSV amounts to 100 cm/s.
Biochemical characterization
Natural grafts were crosslinked resulting in a 56% decrease in primary amine groups. The amount of covalently-bound heparin to the natural graft was 37 ± 4 µg/mg graft (n=3, mean ± s.d.).
Surgical procedure and implantation
Iliac arteries were exposed and interposed with either the natural graft or ePTFE (). The natural graft was easy to handle by the vascular surgeons and its suturability was adequate. After incision, tissue was cauterised to expose the iliac arteries. In all cases, surgery resulted in transient post-operative formation of pockets containing lymphatic fluid (lymphoceles) which is a common phenomenon. Lymphatic fluid was not observed after 4 weeks, which is clinically more than acceptable. In one animal, a diameter mismatch was encountered, the diameter of the native iliac arteries being larger than 4 mm, but this animal was still operated on. Clamping times of iliac arteries were 33 ± 8 min and 23 ± 4 min for implantation of the natural and ePTFE graft, respectively. After implantation, the natural graft pulsated according to the blood flow, and no leakage was observed (See movie ‘pulsating_naturalgraft.wmv’). In one case (animal 6), the natural graft occluded immediately after surgery, without an obvious cause. This graft was replaced by a new natural graft without any complications. Surgicel® was topically applied to prevent excessive suture hole bleeding, especially in the case of ePTFE. The natural and ePTFE graft were comparable in handling during surgical procedures.
Duplex scanning
represents duplex scanning measurements, whereas represents duplex scanning images. Occasionally, velocity measurements at day 0 were hindered by the presence of Surgicel®. All grafts were patent at week 1. Tortuosity of native arteries (6/12 cases, both natural and ePTFE grafts) did not lead to turbulent flow. Turbulence was seen at sites of anastomoses. The PSV ratio, and in the case of severe turbulence the ratio in lumen diameter of graft and artery, was used to determine the degree of narrowing. After 4 weeks, 4/4 natural grafts were occluded vs. 1/4 ePTFE graft. In the case of occlusion, undulating flow resulted into preocclusive signals. Retrograde flow was then seen in distal arteries, implicating formation of collaterals due to blood pressure differences. In animal 4, an infection caused a tandem aneurysm at 4 weeks in the natural graft.
Tissue explantation
Both natural and ePTFE graft were excised with surrounding tissue. Lumens from both grafts were free for passage at one week ().
(Immuno)histological examination
and 6 display histological images 1 and 4 weeks after surgery, respectively, whereas shows immunohistology. Anastomotic sites between artery and graft were clearly observed (, dotted lines), as well as places of suturing (). The elastic layer of the natural graft closely linked to and interacted with the artery's natural elastin, forming a continuum with the elastin of the native artery. The outer collagenous porous layer facilitated ingrowth from the surrounding connective tissue (). The natural graft's elastin layering (black/blue) was clearly seen in Elastin von Gieson (EvG) and Elastin Masson Trichrome (EMT) staining (). Elastin Masson Trichrome staining showed collagen lamellae of the natural graft in green.
Figure 5. Histological images from distal anastomoses 1 week after surgery. Distinct anastomotic sites and patent grafts are visible. The natural graft's elastin layer is shown in black/blue (C, E). Please note continuity between elastin layering of the natural graft and elastin of the native vessel (E). Lamellae of the type I collagen film are seen in pink (A, C) and green (E), and indicated by asterisks (*). (A-B) H&E staining. (C-D) Elastin von Gieson staining (collagen: pink, elastin: blue/black). (E-F) Elastin Masson Trichrome staining (collagen: green, elastin: black, red: fibrin, cells). Dotted lines indicate anastomoses. Bar represents 200 µm.
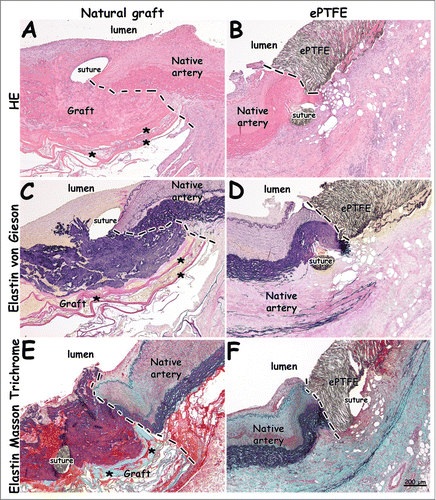
Figure 6. Histological images 4 weeks after surgery. Natural grafts show occlusion with layered thrombi characterized by lines of Zahn (A, C). One ePTFE graft was found to be occluded (B). Calcification was seen in the natural graft located in the elastin layer (C, D, alizarin red staining). Bar represents 200 µm.
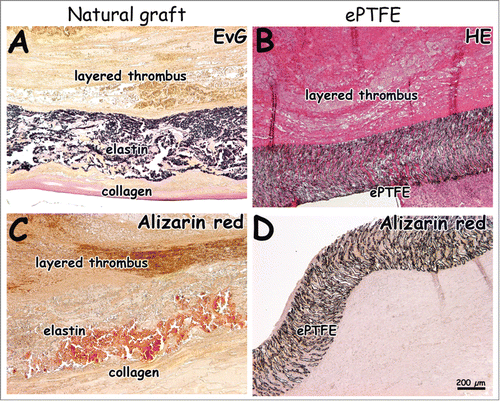
Figure 7. Immunohistochemical images of explants 1 week after surgery (patent lumens). Staining for smooth muscle actin (A, B), laminin (C, D), factor VIII (E, F) and VWF (G, H). Surrounding tissue has invaded the outer collagen layer of the natural graft (A). Endothelial cells on a laminin-positive layer (C, D). Endothelial cells positive for VWF and factor VIII (E-H). VWF-rich depots in the collagen porous film (insert E). Arrows indicate positive staining (C-E), and dotted lines indicate boundary between collagen scaffold and surrounding tissue (A), and anastomosis (B, distal). Bars present 200 µm (A-F) and 50 µm (G, H, and insert of E).
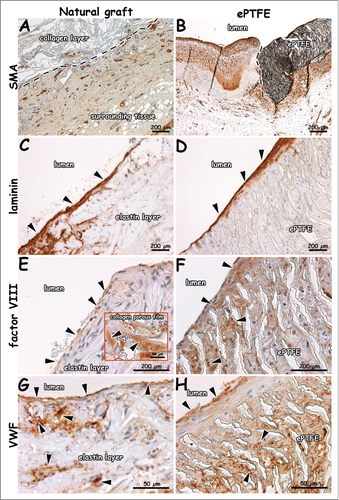
Fibrin was found in between the elastin fibers (yellow in EvG, red in EMT) and in surrounding tissue for both the natural and synthetic graft (). At 4 weeks, elastin fibers in the luminal layer were dislodged, and a fibrin layer was found in between the elastin and intermediate type I collagen film layer (). Layered thrombi were found in all natural grafts and in one ePTFE graft. Organized thrombi contained macrophages. Layered thrombi were recognized by the typical lines of Zahn. For the natural graft, more calcification (alizarin red staining) was found within the elastin layer at 4 weeks () than at 1 week. displays immunohistochemical results of tissue collected at 1 week. Tissue with SMA-positive blood vessels had invaded the outer scaffold layer of the natural graft (). In surrounding native tissue, larger blood and lymphatic vessels were found. A laminin-positive layer (basement membrane) with new endothelium was observed at the anastomotic sites. Cells on elastin fibers and ePTFE stained positive for factor VIII and VWF (). In the natural and ePTFE graft, staining for factor VIII was observed within the graft's wall. In the natural graft, factor VIII-positive areas of plasma were observed.
Scanning electron microscopy
Luminal deposition of (fibrin) matrix and blood-derived cells was observed. Elastin fibers were still recognizable. The ePTFE grafts showed a similar result.
Discussion
This feasibility study demonstrates the surgical application of a multi-layered heparinised elastin-collagen vascular graft, constructed from elastin fibers and type I collagen fibrils, using a porcine model of interposition grafting. This vascular graft mimics the natural blood vessel in architecture and molecular composition by its 3-layered composition of elastin and type I collagen (elastin-collagen-collagen). The vascular graft was chosen to be porous in nature, because this may increase patency and tissue take, as has been demonstrated for ePTFE grafts.Citation39 Observed heparin levels in the constructed triple-layered natural graft correspond to approximately 50 nmol/cm2, whereas this value is 15–25 pmol/cm2 in commercial ePTFE grafts.Citation40 Qualitatively, more heparin is bound to collagen (fibrils) than to elastin (fibers ) as the latter has fewer amine groups available for crosslinking.
Currently, synthetic polymers are widely applied as small-diameter grafts but may result in (re)stenosis in the long termCitation3,4 and limited integration with host tissue.Citation5 Heparinised ePTFE is currently the preferred alternative in peripheral surgery when autologous vein is unavailable. The performance of the layered elastin-collagen graft was therefore compared to that of heparinised ePTFE (Propaten®).
The feasibility of the use of layered elastin-collagen grafts was demonstrated by their one week patency, however, longer-term patency was less than ePTFE. During surgery, the iliac arteries were easy to access and clamping/disclamping of arteries was well within time limits. Surgicel® was topically applied to prevent excessive suture hole bleeding, especially in the case of ePTFE; this may be prevented by using smaller sutures. The natural grafts had thicker walls in comparison to ePTFE. During suturing, this may have resulted in entangling the wall into the blood stream with anastomotic narrowing as a result. In some animals, grafts were undersized in comparison to the exposed artery. This should be avoided, e.g. by duplex scanning before surgery, since it has been shown -at least in the case of ePTFE- that diameter match between artery and ePTFE results in improved neoendothelialisation.Citation41
At surgery, duplex scannings revealed some tortuosity in native arteries for both the natural and ePTFE graft (6/12). This did not relate to the turbulent flows observed. It may merely result in a higher flow at the outer curvature of an artery (thus a lower flow at the inner curvature). Relative narrowing at the anastomotic sites was the main cause of observed turbulence. The resulting high flow and twirling may cause vacuum, and thus sedimentation.
Generally, vascular surgeons hesitate to clinically apply constructed natural grafts because of the risk of early graft degradation resulting in aneurysm formation.Citation26 In this study, no aneurysm formation was observed at any time point, except for one graft, due to infection. Histology showed patent grafts at 1 week of implantation. Endothelialisation started from native arteries into the natural graft and ePTFE, but was not yet confluent. SMA–positive vessels were found in tissue invading the outer type I collagen scaffold layer. These vessels were either VWF-positive or -negative, identifying arteries and lymphatic vessels, respectively. For the natural graft, more calcification (alizarin red staining) was found within the elastin layer at 4 weeks in comparison to 1 week.
The occlusion for natural grafts and ePTFE graft histologically displayed layered thrombi and their characteristic lines of Zahn. This may be caused by the relatively large surface area available for platelet aggregation and by the relative narrowing caused by anastomotic suturing. In addition, increased blood shear stresses through the porous elastin layer may have contributed in subsequent occlusion as well. Either way, a decrease in luminal surface area may improve patency rates for the natural graft, e.g., by changing the porosity or by creating a smoother luminal layer. The intermediate type I collagen porous film did provide additional strength to the natural graft. Fibrin was found in surrounding tissue, which may indicate leakage of blood plasma through the porous collagen film. Fibrin deposition may be a good template for the observed (myo)fibroblasts as it has been widely investigated for scaffolding in vascular tissue engineering.Citation42
Future research may elaborate on the results in this study and may include the (in vitro) preparation and testing of a range of grafts with different pore sizes, thinner walls and/or incorporation of a synthetic polymer mesh or support film.Citation24,25 Improvements to reduce the wall thickness may include performing controlled compression of the graft's porous wall during construction.
In compliance with the ARRIVE guidelinesCitation43 and golden standard publication checklist,Citation44 we reported the animal experiment as detailed as possible, including description of the surgical procedure, medication, nutrition and outcome measurements (i.e. duplex scanning and histology). This may contribute to the implementation of the Three Rs in animal studies and facilitate future systematic reviews on animal trials.Citation44
Conclusion
The surgical application of molecularly-defined heparinised vascular grafts, made from scratch using type I collagen fibrils and elastin fibers, and resembling the native arterial architecture, is feasible. Patency was achieved for at least one week and results match those of the clinically applied ePTFE grafts (1 week). At 4 weeks, however, occlusion was observed. Wall adaptation of the graft may result in long-term patencies. From a fundamental point of view, the use of grafts made from molecularly-defined building blocks may facilitate research to the effect of single graft components.
Disclosure of Potential Conflicts of Interest
No potential conflicts of interest were disclosed.
Acknowledgments
Wilma Janssen-Kessels and Marieke Keizer from the Central Animal Facility (RUMC) are acknowledged for their biotechnical assistance during the surgical procedure. Dr. Paul Jap is appreciated for his histopathological expertise and advice. The microscopical imaging center (MIC, RIMLS, RUMC) is acknowledged for facilitating electron microscopy services.
Funding
This study was financially supported by the Dutch Program for Tissue Engineering (DPTE 6735) and the Foundation for Surgical Research, Department of Surgery, RUMC. Additionally, submission of this study was financed by the ZonMw program MKMD ‘More Knowledge with Fewer Animals’ project number 114024008. The funders had no role in study design, data collection and analysis, decision to publish, or preparation of the manuscript.
References
- Gotlieb AI. Atherosclerosis and acute coronary syndromes. Cardiovasc Pathol 2005;14(4):181-4. Epub 2005/07/13; PMID:16009315; http://dx.doi.org/10.1016/j.carpath.2005.03.007.
- Kapadia MR, Popowich DA, Kibbe MR. Modified prosthetic vascular conduits. Circulation 2008;117(14):1873-82. Epub 2008/04/09; PMID:18391121; http://dx.doi.org/10.1161/CIRCULATIONAHA.107.714170.
- Devine C, McCollum C, North West Femoro-Popliteal Trial P. Heparin-bonded Dacron or polytetrafluorethylene for femoropopliteal bypass: five-year results of a prospective randomized multicenter clinical trial. J Vasc Surg. 2004;40(5):924-31. Epub 2004/11/24; PMID:15557906; http://dx.doi.org/10.1016/j.jvs.2004.08.033.
- Greenwald SE, Berry CL. Improving vascular grafts: the importance of mechanical and haemodynamic properties. J Pathol. 2000;190(3):292-9. Epub 2000/02/24; PMID:10685063; http://dx.doi.org/10.1002/(SICI)1096-9896(200002)190:3%3c292::AID-PATH528%3e3.0.CO;2-S.
- Zilla P, Bezuidenhout D, Human P. Prosthetic vascular grafts: wrong models, wrong questions and no healing. Biomaterials 2007;28(34):5009-27. Epub 2007/08/11; PMID:17688939; http://dx.doi.org/10.1016/j.biomaterials.2007.07.017.
- Piterina AV, Cloonan AJ, Meaney CL, Davis LM, Callanan A, Walsh MT, McGloughlin TM. ECM-based materials in cardiovascular applications: Inherent healing potential and augmentation of native regenerative processes. Int J Mol Sci 2009;10(10):4375-417. Epub 2010/01/09; PMID:20057951; http://dx.doi.org/10.3390/ijms10104375.
- Junqueira JCCJ. Basic histology: text and atlas. Eleven ed: McGraw Hill; 2005.
- Ketharanathan V, Christie BA. Glutaraldehyde tanned ovine collagen compared with polytetrafluoroethylene (GORE-TEX) as a conduit for small calibre artery substitution; an experimental study in dogs. 21 Jan 2008; http://dx.doi.org/10.1111/j.1445-2197.1981.tb05254.x
- Kennealey PT, Elias N, Hertl M, Ko DS, Saidi RF, Markmann JF, Smoot EE, Schoenfeld DA, Kawai T. A prospective, randomized comparison of bovine carotid artery and expanded polytetrafluoroethylene for permanent hemodialysis vascular access. J Vasc Surg 2011;53(6):1640-8. Epub 2011/05/26; PMID:21609797; http://dx.doi.org/10.1016/j.jvs.2011.02.008.
- Gibson R, Potter La, Horn NJ, VanderVeer C. Artegraft. [cited 2013 2nd Octobre 2013]; Available from: www.artegraft.com/bios.htm.
- Palumbo R, Niscola P, Calabria S. Long-term favorable results by arteriovenous graft with Omniflow II prosthesis for hemodialysis. Nephron Clin Pract 2009;113:c76-c80; PMID:19602902; http://dx.doi.org/10.1159/000228538.
- Lepidi S, Grego F, Vindigni V, Zavan B, Tonello C, Deriu GP, Abatangelo G, Cortivo R. Hyaluronan biodegradable scaffold for small-caliber artery grafting: preliminary results in an animal model. Eur J Vasc Endovasc Surg 2006;32(4):411-7. Epub 2006/05/09; PMID:16678455.
- Zavan B, Vindigni V, Lepidi S, Iacopetti I, Avruscio G, Abatangelo G, Cortivo R. Neoarteries grown in vivo using a tissue-engineered hyaluronan-based scaffold. FASEB J. 2008;22(8):2853-61. Epub 2008/04/04; PMID:18385214.
- Bosiers M, Deloose K, Verbist J, Schroe H, Lauwers G, Lansink W, Peeters P. Heparin-bonded expanded polytetrafluoroethylene vascular graft for femoropopliteal and femorocrural bypass grafting: 1-year results. J Vasc Surg. 2006;43(2):313-8; discussion 8–9. Epub 2006/02/16; PMID:16476607; http://dx.doi.org/10.1016/j.jvs.2005.10.037.
- Scharn DM, Dirven M, Barendregt WB, Boll AP, Roelofs D, van der Vliet JA. Human umbilical vein versus heparin-bonded polyester for femoro-popliteal bypass: 5-year results of a prospective randomized multicentre trial. Eur J Vasc Endovasc Surg. 2008;35(1):61-7. Epub 2007/10/16; PMID:17936036.
- Hugl B, Nevelsteen A, Daenens K, Perez MA, Heider P, Railo M, Schelzig H, Gluecklich B, Balzer K, Vermassen F, et al. PEPE II–a multicenter study with an end-point heparin-bonded expanded polytetrafluoroethylene vascular graft for above and below knee bypass surgery: determinants of patency. J Cardiovasc Surg. 2009;50(2):195-203. Epub 2009/03/31; PMID:19329916.
- Waterhouse A, Wise SG, Ng MK, Weiss AS. Elastin as a nonthrombogenic biomaterial. Tissue Eng Part B, Rev. 2011;17(2):93-9. Epub 2010/12/21; PMID:21166482.
- Jordan SW, Haller CA, Sallach RE, Apkarian RP, Hanson SR, Chaikof EL. The effect of a recombinant elastin-mimetic coating of an ePTFE prosthesis on acute thrombogenicity in a baboon arteriovenous shunt. Biomaterials 2007;28(6):1191-7. Epub 2006/11/08; PMID:17087991; http://dx.doi.org/10.1016/j.biomaterials.2006.09.048.
- Lamprou D, Zhdan P, Labeed F, Lekakou C. Gelatine and gelatine/elastin nanocomposites for vascular grafts: processing and characterization. J Biomater Appl. 2011;26(2):209-26. Epub 2010/06/23; PMID:20566658; http://dx.doi.org/10.1177/0885328210364429.
- Berglund JD, Nerem RM, Sambanis A. Incorporation of intact elastin scaffolds in tissue-engineered collagen-based vascular grafts. Tissue Eng. 2004;10(9–10):1526-35. Epub 2004/12/14; PMID:15588412; http://dx.doi.org/10.1089/ten.2004.10.1526.
- Hinds MT, Rowe RC, Ren Z, Teach J, Wu PC, Kirkpatrick SJ, Breneman KD, Gregory KW, Courtman DW. Development of a reinforced porcine elastin composite vascular scaffold. J Biomed Mater Res A 2006;77(3):458-69. Epub 2006/02/03; PMID:16453334; http://dx.doi.org/10.1002/jbm.a.30571.
- McKenna KA, Hinds MT, Sarao RC, Wu PC, Maslen CL, Glanville RW, Babcock D, Gregory KW. Mechanical property characterization of electrospun recombinant human tropoelastin for vascular graft biomaterials. Acta Biomater. 2012;8(1):225-33. Epub 2011/08/19; PMID:21846510; http://dx.doi.org/10.1016/j.actbio.2011.08.001.
- McClure MJ, Simpson DG, Bowlin GL. Tri-layered vascular grafts composed of polycaprolactone, elastin, collagen, and silk: Optimization of graft properties. J Mech Behav Biomed Mater. 2012;10:48-61. Epub 2012/04/24; PMID:22520418; http://dx.doi.org/10.1016/j.jmbbm.2012.02.026.
- Zhang H, Jia X, Han F, Zhao J, Zhao Y, Fan Y, Yuan X. Dual-delivery of VEGF and PDGF by double-layered electrospun membranes for blood vessel regeneration. Biomaterials 2013;34(9):2202-12. Epub 2013/01/08; PMID:23290468; http://dx.doi.org/10.1016/j.biomaterials.2012.12.005.
- Han F, Jia X, Dai D, Yang X, Zhao J, Zhao Y, Fan Y, Yuan X. Performance of a multilayered small-diameter vascular scaffold dual-loaded with VEGF and PDGF. Biomaterials 2013;34(30):7302-13. Epub 2013/07/09; PMID:23830580; http://dx.doi.org/10.1016/j.biomaterials.2013.06.006.
- Wise SG, Byrom MJ, Waterhouse A, Bannon PG, Weiss AS, Ng MK. A multilayered synthetic human elastin/polycaprolactone hybrid vascular graft with tailored mechanical properties. Acta Biomater. 2011;7(1):295-303. Epub 2010/07/27; PMID:20656079; http://dx.doi.org/10.1016/j.actbio.2010.07.022.
- McClure MJ, Sell SA, Simpson DG, Walpoth BH, Bowlin GL. A three-layered electrospun matrix to mimic native arterial architecture using polycaprolactone, elastin, and collagen: a preliminary study. Acta Biomater. 2010;6(7):2422-33. Epub 2010/01/12; PMID:20060934; http://dx.doi.org/10.1016/j.actbio.2009.12.029.
- de Valence S, Tille JC, Giliberto JP, Mrowczynski W, Gurny R, Walpoth BH, Möller M. Advantages of bilayered vascular grafts for surgical applicability and tissue regeneration. Acta Biomater 2012;8(11):3914-20. Epub 2012/07/10; PMID:22771455; http://dx.doi.org/10.1016/j.actbio.2012.06.035.
- Browning MB, Dempsey D, Guiza V, Becerra S, Rivera J, Russell B, Höök M, Clubb F, Miller M, Fossum T, et al. Multilayer vascular grafts based on collagen-mimetic proteins. Acta Biomater 2012;8(3):1010-21. Epub 2011/12/07; PMID:22142564; http://dx.doi.org/10.1016/j.actbio.2011.11.015.
- Kumar VA, Caves JM, Haller CA, Dai E, Liu L, Grainger S, Chaikof EL. Acellular vascular grafts generated from collagen and elastin analogs. Acta Biomater 2013;9(9):8067-74. Epub 2013/06/08; PMID:23743129; http://dx.doi.org/10.1016/j.actbio.2013.05.024.
- McClure MJ, Wolfe PS, Simpson DG, Sell SA, Bowlin GL. The use of air-flow impedance to control fiber deposition patterns during electrospinning. Biomaterials 2012;33(3):771-9. Epub 2011/11/08; PMID:22054536.
- Koens MJ, Faraj KA, Wismans RG, van der Vliet JA, Krasznai AG, Cuijpers VM, Jansen JA, Daamen WF, van Kuppevelt TH. Controlled fabrication of triple layered and molecularly defined collagen/elastin vascular grafts resembling the native blood vessel. Acta Biomater 2010;6(12):4666-74. Epub 2010/07/14; PMID:20619367; http://dx.doi.org/10.1016/j.actbio.2010.06.038.
- Swartz DD, Andreadis ST. Animal models for vascular tissue-engineering. Curr Opin Biotechnol 2013; 24(5):916-25. Epub 2013/06/19.
- Geutjes PJ, van der Vliet JA, Faraj KA, Vries N, van Moerkerk HT, Wismans RG, Hendriks T, Daamen WF, van Kuppevelt TH. An animal model for femoral artery pseudoaneurysms. J Vasc Interv Radiol 2010;21(7):1078-83. Epub 2010/04/13; PMID:20382547.
- Daamen WF, Hafmans T, Veerkamp JH, van Kuppevelt TH. Isolation of intact elastin fibers devoid of microfibrils. Tissue Eng 2005;11(7–8):1168-76. Epub 2005/09/08; PMID:16144453; http://dx.doi.org/10.1089/ten.2005.11.1168.
- Pieper JS, Hafmans T, Veerkamp JH, van Kuppevelt TH. Development of tailor-made collagen-glycosaminoglycan matrices: EDC/NHS crosslinking, and ultrastructural aspects. Biomaterials 2000;21(6):581-93. Epub 2000/03/04; PMID:10701459.
- Buttafoco L, Engbers-Buijtenhuijs P, Poot AA, Dijkstra PJ, Daamen WF, van Kuppevelt TH, Vermes I, Feijen J. First steps towards tissue engineering of small-diameter blood vessels: preparation of flat scaffolds of collagen and elastin by means of freeze drying. J Biomed Mater Res B Appl Biomater 2006;77(2):357-68. Epub 2005/12/20; PMID:16362956.
- Bancroft JD SA. Theory and Practice of Histological Techniques: Churchill Livingstone; 1996 1996.
- Chlupac J, Filova E, Bacakova L. Blood vessel replacement: 50 years of development and tissue engineering paradigms in vascular surgery. Physiol Res 2009; 58 Suppl 2:S119-39. Epub 2009/01/01; PMID:20131930.
- Begovac PC, Thomson RC, Fisher JL, Hughson A, Gallhagen A. Improvements in GORE-TEX vascular graft performance by Carmeda BioActive surface heparin immobilization. Eur J Vasc Endovasc Surg 2003;25(5):432-7. Epub 2003/04/26; PMID:12713782.
- Cikirikcioglu M, Pektok E, Cikirikcioglu YB, Osorio-da Cruz S, Tille JC, Kalangos A, Walpoth BH. Matching the diameter of ePTFE bypass prosthesis with a native artery improves neoendothelialization. Eur Surg Res 2008;40(4):333-40. Epub 2008/02/28; PMID:18303269; http://dx.doi.org/10.1159/000118029.
- Brown AC, Barker TH. Fibrin-based biomaterials: Modulation of macroscopic properties through rational design at the molecular level. Acta Biomater 2013; 10(4):1502-14. Epub 2013/09/24.
- Kilkenny C, Browne WJ, Cuthill IC, Emerson M, Altman DG. Improving bioscience research reporting: the ARRIVE guidelines for reporting animal research. PLoS Biol 2010;8(6):e1000412. Epub 2010/07/09; PMID:20613859; http://dx.doi.org/10.1371/journal.pbio.1000412.
- Hooijmans CR, Leenaars M, Ritskes-Hoitinga M. A gold standard publication checklist to improve the quality of animal studies, to fully integrate the Three Rs, and to make systematic reviews more feasible. Altern Lab Anim 2010;38(2):167-82. Epub 2010/05/29; PMID:20507187.