ABSTRACT
Although adipose-derived stem cells (ADSCs) have demonstrated a promising potential for the applications of cell-based therapy and regenerative medicine, excessive reactive oxygen species (ROS) are harmful to ADSCs cell survival and proliferation. Vitamin C is an important antioxidant, and is often added into culture media as an essential micronutrient. However, its roles on the proliferation of human ADSCs have not been studied. Therefore, in this study, human ADSCs were isolated, and detected by flow cytometry for the analysis of their cell surface antigens. Cell proliferation and cell cycle progression were measured with cell counting kit-8 assay and flow cytometry, respectively. Western blotting was used to detect the expression levels of cyclin E1, p53, p21, and CDK2 proteins. The effect of vitamin C pretreatment on the production of hydrogen peroxide (H2O2)-mediated ROS in the ADSCs was evaluated by flow cytometry. Our results indicated that vitamin C treatment significantly increased cell proliferation, and changed the cell cycle distribution of ADSCs by decreasing the percentage of G1 phase, and concurrently increased the percentage of S and G2/M phase. Western blot analysis indicated that vitamin C treatment up-regulated the expression levels of cyclin E1 and CDK2, but down-regulated p53 and p21 proteins expression, which contributed to cell proliferation and cell cycle progression. Vitamin C pretreatment significantly reduced the production of H2O2-induced ROS in the ADSCs. These findings suggest that vitamin C can promote the proliferation and cell cycle progression in the ADSCs possibly through regulation of p53-p21 signal pathway.
INTRODUCTION
Since adipose-derived stem cells (ADSCs) were successfully isolated from human adipose tissue,Citation1,2 increasing studies have indicated that ADSCs become a promising potential for regenerative medicine due to its easy harvest from donors, and strong differentiation capacity into various cell lineages such as cartilage, bone, fat, skeletal muscle, tendon, and ligament in the appropriate microenvironment.Citation3-5 The in vitro expansion of ADSCs in culture medium is an important approach to obtain substantial cells before cell transplantation. However, it was reported that the in vitro cultured cells produced more reactive oxygen species (ROS) when compared to the in vivo conditions.Citation6 Additionally, high levels of ROS can damage cell membrane, and result in DNA fragmentation and cell injury or death in vitro.Citation7,8 Accordingly, it is necessary to reduce excessive ROS production, promote cell proliferation and growth in vitro, and enhance the expansion of stem cells.
Vitamin C (ascorbate) is a well-known antioxidant that can scavenge highly reactive free radicals generated constantly by the cellular machinery,Citation9 and it is often supplemented into culture media to ensure appropriate ROS level for cell growth.Citation10 Vitamin C is also an important cofactor in several enzymatic reactions such as histone demethylases and prolylhydroxylases.Citation11 Recent studies have demonstrated that vitamin C supplementation produces important effects on stem cell generation, proliferation and differentiation.Citation12 It was reported that the supplementation of vitamin C with an appropriate dose in culture medium could increase the efficiency of induced pluripotent stem cell generation from human somatic cells,Citation13 enhance the expansion and osteogenic differentiation of bone marrow-derived mesenchymal stem cells.Citation14 However, the effect of vitamin C treatment on the proliferation of human ADSCs has not been fully elucidated. Accordingly, in this study, we tried to investigate the effect of vitamin C supplementation in culture medium on the proliferation of human ADSCs.
RESULTS
Cell surface antigens of the isolated ADSCs
Human ADSCs were isolated from the subcutaneous adipose tissues by adhering to the culture flasks, and their spindle-shape appearance was observed after grown for 2 passages (). Additionally, the results of flow cytometry analysis demonstrated that the isolated ADSCs highly expressed CD29 (98.62%), CD44 (99.91%), CD90 (99.95%), and CD105 (99.32%), which were considered as the markers for mesenchymal stem cells,Citation2 but exhibited very weak expression of haematopoietic lineage markers CD31(0.19%), CD34 (0.38%), and CD45 (0.06%). In addition, high expression of CD13 (99.79%) and negligible expression of CD14 (0.05%) and CD106 (0.02%) were also observed in these cells at passage 3 (), which was similar to previous reports.Citation15
FIGURE 1. Detection of cell surface markers. (a-b) Spindle-shape morphological feature of the ADSCs isolated from human adipose tissue.(c) The cell surface markers of ADSCs at passage 3 were detected by flow cytometry, and nonspecific IgG was served as the control of background fluorescence. The positive percentage of cell surface markers was exhibited in the histograms.
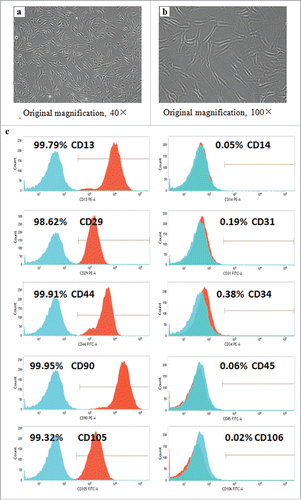
Effect of vitamin C treatment on cell proliferation of ADSCs
CCK-8 assay indicated that 30, 60, and 90 µg/ml of vitamin C treatment all significantly promoted the proliferation of ADSCs 24 h after incubation, but with the continuous extension of time, we found that the best suitable concentration of vitamin C the proliferation of ADSCs is 30 µg/ml, compared to 60 or 90 µg/ml of vitamin C ().
FIGURE 2. The effect of vitamin C treatment on cell proliferation of ADSCs. The effect of vitamin C on the cell proliferation of ADSCs was evaluated by CCK-8 assay after treatment with various concentrations (0, 30, 60, and 90 µg/ml) of vitamin C for 24, 48, and 72 h. The values were expressed as mean ± SD, n = 3, *P< 0.05, compared with control (0 µg/ml of vitamin C).
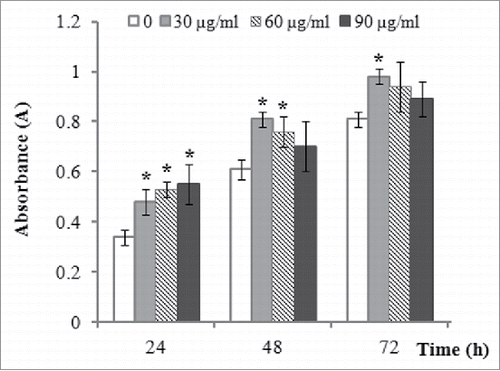
Vitamin C changes cell cycle progression
Flow cytometry analysis indicated that vitamin C could promote cell cycle progression after the ADSCs were treated by 30 µg/ml of vitamin C for 24 and 48 h, and significantly decreased the percentage of G1 phase, and a parallel increase of S and G2/M phase was markedly observed after the treatment of vitamin C ().
FIGURE 3. The effect of vitamin C treatment on cell cycle progression. Cell cycle distribution was determined by flow cytometry after the ADSCs were incubated in the absence or the presence of 30 µg/ml vitamin C for 24 and 48 h. The data denoted as mean ± SD, *P< 0.05, compared with control.
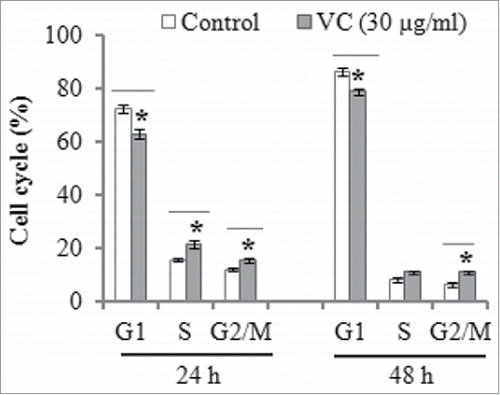
Additionally, we further detected the expression level of cell cycle-associated proteins. The results of western blotting demonstrated that the expression of cyclin E1 and CDK2 was upregulated, but p53 and p21 proteins were both downregulated after 24 h treatment with 30 µg/ml of vitamin C (), which contributed to cell cycle progression.
FIGURE 4. The effects of vitamin C on the expression levels of cyclin E1, CDK2, p53, and p21 proteins in ADSCs. Cell lysates were prepared from the ADSCs treated with 30 µg/ml of vitamin C or equal volume of culture medium (control) for 24 h, and protein gel blotting was used to detected the expression levels of cell cycle-associated proteins. (a) Representative western blot images for detecting the expression levels of cyclin E1, CDK2, p53, and p21 proteins. β-Tubulin was used as the loading control. (b) Relative expression levels of cyclin E1, CDK2, p53, and p21 proteins from 3 independent experiments based on (A) as quantified by densitometry of above proteins/β-Tubulin.
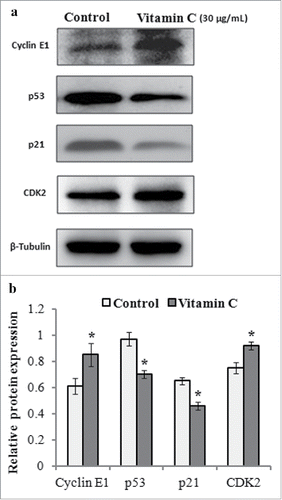
Vitamin C decreases H2O2-mediated ROS production in ADSCs
We evaluated the effect of vitamin C pretreatment on H2O2-induced ROS production in the ADSCs that were exposed to 0.25 or 0.35 nM H2O2 for 2 h. The results of flow cytometry measurement indicated that the fluorescence intensity of DCFDA, an intracellular ROS indicator, was significantly decreased in the ADSCs pretreated with vitamin C ().
FIGURE 5. The effects of vitamin C on ROS production in ADSCs. ADSCs were pretreated with 30 µg/ml of vitamin C for 20 min, and exposed to 0.25 or 0.35 nM H2O2 for 2 h, and intracellular ROS level was measured by flow cytometry. Cells, which were incubated with only culture, were used as the negative control. (a) Representative images of ROS detection by flow cytometry. (b) The relative levels of ROS as measured by fluorescence intensity of DCFDA compared to negative control (NC). The values were expressed as mean ± SD, n = 3, *P< 0.05.
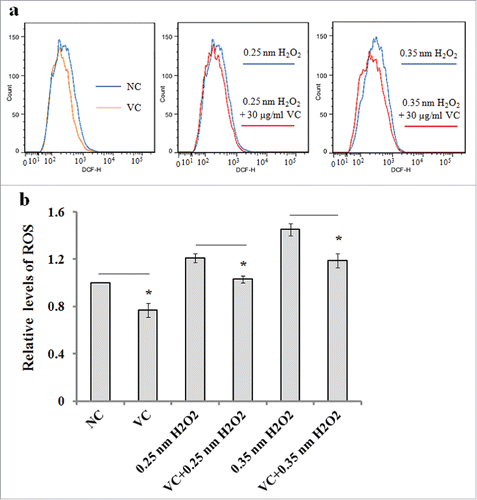
DISCUSSION
Increasing evidence has indicated that ADSCs have a promising potential for stem cell-based tissue engineering and regenerative medicine due to their strong capacity to differentiate into multiple cell lineages including adipocytes, osteoblasts, and chondroblasts under proper induction environment.Citation2,16 As an essential micronutrient, vitamin C is often added to culture medium for cell growth. In the present study, we found that the supplementation of vitamin C at an optimal concentration in culture medium was beneficial to the proliferation of human ADSCs by regulating the p53-p21 pathway and related cell cycle progression.
In our experiment, human ADSCs were first isolated from adipose tissue by using the method that we described previously.Citation17 Next, we detected the cell surface antigens of these cells. We found that these obtained cells expressed highly surface markers of CD29, CD44, CD90, CD13, and CD105 and lacked the expression of CD31, CD34, CD45, CD14, and CD106. These data were in agreement with the previously reported property of mesenchymal stem cells,Citation15,17 and implied that these isolated ADSCs had relatively high purity.
The treatment of vitamin C may promote cell proliferation was an initial concern since various doses probably result in diverse effects on stem cells. We found that 30 µg/ml of vitamin C had a better effect on the proliferation of ADSCs than 60 and 90 µg/ml of vitamin C. The ADSCs treated with 30 µg/ml of vitamin C have demonstrated the best cell proliferation activity, which was similar to previous reports about the concentration-dependent effect of vitamin C on cell proliferation.Citation18-20 In addition, our findings also indicated that cell cycle progression was promoted, and the decreased percentage of G1 phase was obviously observed after incubation with 30 µg/ml of vitamin C for 24 and 48 h, and meanwhile, the increased percentage of the cells at S and G2/M phase was also observed. To further investigate the mechanism by which vitamin C increased cell proliferation and promoted cell cycle progression, we detected the cell cycle-associated proteins including cyclin E1, p53, p21, and CDK2. Cyclin E1 belongs to the highly conserved cyclin family, which is required for cell cycle G1/S transition.Citation21 It has been reported that cyclin E1 can interact with CDK2 and activate CDK2 at the G1/S transition of the cell cycle.Citation22,23 In the present study, protein gel blotting analysis indicated that the expression levels of cyclin E1 and CDK2 proteins were up-regulated after 12 h of incubation with vitamin C, which suggests that cyclin E1 and CDK2 may involved in the transition of cell cycle from G1 to S phase in the ADSCs. The p53 protein is an important regulative factor of many processes necessary for the proper cellular function, and is also responsible for the regulation of cell cycle.Citation24 Additionally, it was reported that the proliferation rate of mesenchymal stem cells (MSCs) derived from p53 knockout mice was significantly increased, compared to the MSCs derived from wild-type animals.Citation25 In this study, our results demonstrated that p53 protein and its downstream target, p21, were decreased after vitamin C treatment, which was consistent with previous reports that suppression of p53-p21 pathway was beneficial to the proliferation of cells.Citation26,27 Additionally, we also evaluated the effect of vitamin C pretreatment on the H2O2-induced ROS production in the ADSCs. The results demonstrated that vitamin C pretreatment could significantly reduce ROS level in the ADSCs. Recent studies indicate that ROS at low levels may play an important role as a second messenger and activate normal cellular processes,Citation28,29 but excessive accumulation of ROS production to has the potential to damage cells, resulting in cell aging and apoptosis.Citation8 In fact, whether ROS is beneficial or harmful is primarily a question of dosage. That is why that the effects of ROS generation on the proliferation of ADSCs were found to be quite different.Citation30-32 Therefore, a precise control of intracellular ROS production probably plays a key determining role in cell proliferation.
Taken together, our data indicate that vitamin C addition in culture medium significantly augment the proliferation of ADSCs, and promoted cell cycle progression in the ADSCs. Vitamin C treatment resulted in the up-regulation of cyclin E1 and CDK2 proteins, and the down-regulation of p53 and p21 proteins in ADSCs, which probably contributed to cell cycle progression. Additionally, vitamin C pretreatment significantly decreased the H2O2-induced ROS levels in the ADSCs. These findings suggest that vitamin C could be used as a supplement in the culture medium for the culture of ADSCs.
MATERIALS AND METHODS
Chemical and reagents
Vitamin C were purchased from Sigma-Aldrich Company (St. Louis, MO, USA), and its purity was more than 98% detected by HPLC. A stock solution was first prepared through dissolution of vitamin C in DMEM medium (Gibco, USA) to a concentration of 5 mg/ml, and stored at −20°C before use. The finally used concentration of vitamin C was made by further dilution with DMEM medium. Cell counting kit-8 was obtained from Beyotime Biotechnology company (China). DMEM medium and fetal bovine serum (FBS) were purchased from Gibco company. The primary antibodies against cyclin E1, p53, p21, CDK2, and β-actin were purchased from Cell Signal Technology (Beverly, MA, USA).
Isolation and verification of human ADSCs
The isolation and culture of human ADSCs were performed as we described previously.Citation17 Cell surface antigens including CD45, CD105, CD44, CD29, CD90, CD34, CD31, CD106, and CD31 (BD, Bioscience) of ADSCs at passage 3 were determined by flow cytometry (Becton-Dickinson) according to our described previously methods.Citation17 The ADSCs at passage 4–6 were used for the following experiments in this study.
Cell proliferation assay
ADSCs at passage 4 were seeded in 96-well microplates at a density of 3000 cells per well. After 24 h of inoculation, the cells were treated by vitamin C of various concentrations (0, 30, 60, and 90 µg/ml) for 24, 48, and 72 h, and followed by removing culture medium. 10 µl of cell counting kit-8 solution (CCK-8) in 100 µl fresh culture medium were added to each well of 96-well microplates, and incubated for 1 h at 37°C. The absorbance value of each well was then determined at 450 nm with automated spectrophotometric plate reader (PerkinElmer, USA). All experiments were performed for duplicate at least 3 times.
Vitamin C affects cell cycle distribution
The effects of vitamin C treatment on cell cycle distribution of ADSCs were performed by flow cytometer. ADSCs at passage 5 were seeded in 6-well plates at a density of 1 × 105 cells/well for 12 h incubation. Then, the cells were treated with 0 or 30 µg/ml vitamin C for 24 and 48 h, and followed by digestion with 0.25% trypsin containing 0.02% EDTA. Subsequently, cells were fixed with 75% ethanol for 1 h, and then incubated in propidium iodide (50 µg/ml) solution for 30 minutes in the dark at room temperature. Cell cycle distribution was measured with flow cytometry (Becton Dickinson, NJ, USA). The experiments were performed for duplicate at 3 times.
Western blotting
The expression levels of cell cycle-associated proteins including cyclin E1, p53, p21, and CDK2 were detected by western blotting after ADSCs were treated by 0 and 30 µg/ml of vitamin C for 24 h. The cells were treated with cell lysis buffer and cell lysates were collected. Total protein concentration was measured with BCA assay (Beyotime, China), and 20 µg of total proteins were then separated by electrophoresis on 10% SDS-PAGE, and transferred to polyvinylidene difluoride (PVDF) membranes. Subsequently, the membranes were incubated in a blocking buffer for 1 h. After blocking, the membrane was probed with the primary antibodies against cyclin E1, p53, p21, and CDK2 overnight at 4°C, and followed by washing 3 times with 1× TBST, and incubated with the corresponding horseradish peroxidase-conjugated secondary antibodies for 1 h. The expression levels of proteins were detected using an enhanced chemiluminescence reagent and system (Amersham Biosciences Corp., NJ, USA). β-actin was used as a loading control.
Measurement of intracellular reactive oxygen species (ROS)
Intracellular ROS level was determined with fluorescent probe 2′,7′-dichlorofluorescein diacetate (DCFDA; Molecular Probes, OR, USA). Briefly, ADSCs (approximately 1 × 105 cells) were seeded in 60-mm culture dish and incubation for 24 h, and then pretreated with 30 µg/ml of vitamin C for 20 min, and followed by treatment with 0.25 or 0.35 nM H2O2 for 2 h. The cells were incubated in serum-free culture medium containing 10 µM DCFDA at 37°C in a humidified incubator for 20 min. After washing 3 times, fluorescence intensity of DCFDA was detected using flow cytometry (Becton Dickinson, NJ, USA).
Statistical analyses
All data were presented as mean values ± standard deviation, and statistical significance was evaluated by a 2-tailed Student's t-test by using a SPSS 13.0 statistical package, and the P < 0.05 was defined statistically significant.
DISCLOSURE OF POTENTIAL CONFLICTS OF INTEREST
No potential conflicts of interest were disclosed.
Funding
This work was supported by the Scientific Research Starting Foundation for the Doctors of Guangdong Medical University (grant number: BJ201510), and National Natural Science Foundation of China (81372511 to Xudong Tang), and Natural Science Foundation of Guangdong Province (grant number: 2014A030313535), Zhanjiang Municipal Governmental Specific Financial Fund Allocated for Competitive Scientific &Technological Projects (No. 2014C01022), and Scientific Research Fund of Guangdong Medical University (grant number: M2014042).
REFERENCES
- Zuk PA, Zhu M, Mizuno H, Huang J, Futrell JW, Katz AJ, Benhaim P, Lorenz HP, Hedrick MH. Multilineage cells from human adipose tissue: implications for cell-based therapies. Tissue Eng 2001; 7:211-28; PMID:11304456; http://dx.doi.org/10.1089/107632701300062859
- Zuk PA, Zhu M, Ashjian P, De Ugarte DA, Huang JI, Mizuno H, Alfonso ZC, Fraser JK, Benhaim P, Hedrick MH. Human adipose tissue is a source of multipotent stem cells. Mol Biol Cell 2002; 13:4279-95; PMID:12475952; http://dx.doi.org/10.1091/mbc.E02-02-0105
- Kim JH, Jung M, Kim HS, Kim YM, Choi EH. Adipose-derived stem cells as a new therapeutic modality for ageing skin. Exp Dermatol 2011; 20:383-7; PMID:21355887; http://dx.doi.org/10.1111/j.1600-0625.2010.01221.x
- Mizuno H, Tobita M, Uysal AC. Concise review: Adipose-derived stem cells as a novel tool for future regenerative medicine. Stem Cells 2012; 30:804-10; PMID:22415904; http://dx.doi.org/10.1002/stem.1076
- Catalano E, Cochis A, Varoni E, Rimondini L, Carrassi A, Azzimonti B. Adipose-derived adult stem cells: available technologies for potential clinical regenerative applications in dentistry. Crit Rev Biomed Eng 2013; 41:483-93; PMID:24940661
- Goto Y, Noda Y, Mori T, Nakano M. Increased generation of reactive oxygen species in embryos cultured in vitro. Free Radic Biol Med 1993; 15:69-75; PMID:8359711; http://dx.doi.org/10.1016/0891-5849(93)90126-F
- Cramer C, Freisinger E, Jones RK, Slakey DP, Dupin CL, Newsome ER, Alt EU, Izadpanah R. Persistent high glucose concentrations alter the regenerative potential of mesenchymal stem cells. Stem Cells Dev 2010; 19:1875-84; PMID:20380516; http://dx.doi.org/10.1089/scd.2010.0009
- Park JY, Kim MJ, Kim YK, Woo JS. Ceramide induces apoptosis via caspase-dependent and caspase-independent pathways in mesenchymal stem cells derived from human adipose tissue. Arch Toxicol 2011; 85:1057-65; PMID:21259059; http://dx.doi.org/10.1007/s00204-011-0645-x
- Harrison FE, May JM. Vitamin C function in the brain: vital role of the ascorbate transporter SVCT2. Free Radic Biol Med 2009; 46:719-30; PMID:19162177; http://dx.doi.org/10.1016/j.freeradbiomed.2008.12.018
- Hu J, Cheng D, Gao X, Bao J, Ma X, Wang H. Vitamin C enhances the in vitro development of porcine pre-implantation embryos by reducing oxidative stress. Reprod Domest Anim 2012; 47:873-9; PMID:22239270; http://dx.doi.org/10.1111/j.1439-0531.2011.01982.x
- Shi Y. Histone lysine demethylases: Emerging roles in development, physiology and disease. Nat Rev Genet 2007; 8:829-33; PMID:17909537; http://dx.doi.org/10.1038/nrg2218
- Nualart F, Salazar K, Oyarce K, Cisternas P, Jara N, Silva-Álvarez C, Pastor P, Martínez F, García A, García-Robles Mde L, et al. Typical and atypical stem cells in the brain, vitamin C effect and neuropathology. Biol Res 2012; 45:243-56; PMID:23283434; http://dx.doi.org/10.4067/S0716-97602012000300006
- Esteban MA, Wang T, Qin B, Yang J, Qin D, Cai J, Li W. Vitamin C enhances the generation of mouse and human induced pluripotent stem cells. Cell Stem Cell 2010; 6:71-9; PMID:20036631; http://dx.doi.org/10.1016/j.stem.2009.12.001
- Wang Y, Singh A, Xu P, Pindrus MA, Blasioli DJ, Kaplan DL. Expansion and osteogenic differentiation of bone marrow-derived mesenchymal stem cells on a vitamin C functionalized polymer. Biomaterials 2006; 27:3265-73; PMID:16494940; http://dx.doi.org/10.1016/j.biomaterials.2006.01.036
- Yu G, Floyd ZE, Wu X, Halvorsen YD, Gimble JM. Isolation of human adipose-derived stem cells from lipoaspirates. Methods Mol Biol 2011; 702:17-27; PMID:21082391; http://dx.doi.org/10.1007/978-1-61737-960-4_2
- Gimble JM, Bunnell BA, Frazier T, Rowan B, Shah F, Thomas-Porch C, Wu X. Adipose-derived stromal/stem cells: a primer. Organogenesis 2013; 9:3-10; PMID:23538753; http://dx.doi.org/10.4161/org.24279
- Zeng G, Lai K, Li J, Zou Y, Huang H, Liang J, Tang X, Wei J, Zhang P. A rapid and efficient method for primary culture of human adipose-derived stem cells. Organogenesis 2013; 9:287-95; PMID:24280895; http://dx.doi.org/10.4161/org.27153
- Chepda T, Cadau M, Girin P, Frey J, Chamson A. Monitoring of ascorbate at a constant rate in cell culture: effect on cell growth. In Vitro Cell Dev Biol Anim 2011; 37:26-30; http://dx.doi.org/10.1290/1071-2690(2001)037%3c0026:MOAAAC%3e2.0.CO;2
- Osiecki M, Ghanavi P, Atkinson K, Nielsen LK, Doran MR. The ascorbic acid paradox. Biochem Biophys Res Commun 2010; 400:466-70; PMID:20732307; http://dx.doi.org/10.1016/j.bbrc.2010.08.052
- Huang Y, Tang X, Xie W, Zhou Y, Li D, Zhou Y, Zhu J, Yuan T, Lai L, Pang D, et al. Vitamin C enhances in vitro and in vivo development of porcine somatic cell nuclear transfer embryos. Biochem Biophys Res Commun 2011; 411:397-401; PMID:21749856; http://dx.doi.org/10.1016/j.bbrc.2011.06.160
- Gourguechon S, Savich JM, Wang CC. The multiple roles of cyclin E1 in controlling cell cycle progression and cellular morphology of Trypanosoma brucei. J Mol Biol 2007; 368:939-50; PMID:17376478; http://dx.doi.org/10.1016/j.jmb.2007.02.050
- Nevzorova YA, Bangen JM, Hu W, Haas U, Weiskirchen R, Gassler N, Huss S, Tacke F, Sicinski P, Trautwein C, et al. Cyclin E1 controls proliferation of hepatic stellate cells and is essential for liver fibrogenesis in mice. Hepatology 2012; 56:1140-9; PMID:22454377; http://dx.doi.org/10.1002/hep.25736
- Jayapal SR, Kaldis P. Cyclin E1 regulates hematopoietic stem cell quiescence. Cell Cycle 2013; 12:3588; PMID:24189528; http://dx.doi.org/10.4161/cc.26974
- Wawryk-Gawda E, Chylińska-Wrzos P, Lis-Sochocka M, Chłapek K, Bulak K, Jędrych M, Jodłowska-Jędrych B. P53 protein in proliferation, repair and apoptosis of cells. Protoplasma 2014; 251:525-33; PMID:24043441; http://dx.doi.org/10.1007/s00709-013-0548-1
- Armesilla-Diaz A, Elvira G, Silva A. p53 regulates the proliferation, differentiation and spontaneous transformation of mesenchymal stem cells. Exp Cell Res 2009; 315:3598-610; PMID:19686735; http://dx.doi.org/10.1016/j.yexcr.2009.08.004
- Esteban MA, Pei D. Vitamin C improves the quality of somatic cell reprogramming. Nat Genet 2012; 44:366-7; PMID:22456737; http://dx.doi.org/10.1038/ng.2222
- Yi C, Wang Q, Wang L, Huang Y, Li L, Liu L, Zhou X, Xie G, Kang T, Wang H, et al. MiR-663, a microRNA targeting p21 (WAF1/CIP1), promotes the proliferation and tumorigenesis of nasopharyngealcarcinoma. Oncogene 2012; 31:4421-33; PMID:22249270; http://dx.doi.org/10.1038/onc.2011.629
- Kim JH, Song SY, Park SG, Song SU, Xia Y, Sung JH. Primary involvement of NADPH oxidase 4 in hypoxia-induced generation of reactive oxygen species in adipose-derived stem cells. Stem Cells Dev 2012; 21:2212-21; PMID:22181007; http://dx.doi.org/10.1089/scd.2011.0561
- Kim JH, Park SG, Song SY, Kim JK, Sung JH. Reactive oxygen species-responsive miR-210 regulates proliferation and migration of adipose-derived stem cells via PTPN2. Cell Death Dis 2013; 4:e588; PMID:23579275; http://dx.doi.org/10.1038/cddis.2013.117
- Lee EY, Xia Y, Kim WS, Kim MH, Kim TH, Kim KJ, Park BS, Sung JH. Hypoxia-enhanced wound-healing function of adipose-derived stem cells: increase in stem cell proliferation and up-regulation of VEGF and bFGF. Wound Repair Regen 2009; 17:540-7; PMID:19614919; http://dx.doi.org/10.1111/j.1524-475X.2009.00499.x
- Jee MK, Kim JH, Han YM, Jung SJ, Kang KS, Kim DW, Kang SK. DHP-derivative and low oxygen tension effectively induces human adipose stromal cell reprogramming. PLOS One 2010; 5:e9026; PMID:20161735; http://dx.doi.org/10.1371/journal.pone.0009026
- Song JS, Cho HH, Lee BJ, Bae YC, Jung JS. Role of thioredoxin 1 and thioredoxin 2 on proliferation of human adipose tissue-derived mesenchymal stem cells. Stem Cells Dev 2011; 20:1529-37; PMID:21158569; http://dx.doi.org/10.1089/scd.2010.0364