ABSTRACT
Hard-to-heal wounds can be detrimental to patients’ quality of life. Currently, there is scarcity of therapeutic alternatives to mainstay surgical treatment, which uses the principles of tissue debridement, temporary wound coverage, and subsequent tissue reconstruction. Here, a new approach is proposed that harnesses the regenerative power of autologous peripheral blood, through a process termed hypoxia-adjusted in vitro preconditioning. The effectiveness of this method is demonstrated with six cases of surgical wounds, including two cases of large full-thickness dermal wounds that developed as a result of skin necrosis following abdominoplasty and buttock-lift procedures in heavy smokers, as well as a case of extensive inflammatory tissue damage that occurred following breast surgery. While these wounds differed in size (4–160 cm2), geometry and location, all of them could be managed conservatively with topical application of growth factor-enriched hypoxia preconditioned serum derived from the patient’s own peripheral blood. This treatment led to complete wound closure by latest 135 days. The finding of complete skin regeneration even in large (>10 cm2), full-thickness wounds, where initially no dermal tissue was available in the wound bed, strongly suggests that the treatment targeted key cellular regenerative mechanisms, including differentiation, angiogenesis, granulation tissue induction, contraction and epithelialization. The method is readily clinically applicable, cost effective, and overcomes limitations of the classic reconstructive approach.
Introduction
One of the main areas of focus in regenerative medicine research is that of dermal tissue repair, especially the treatment of chronic wounds.Citation1,Citation2 Despite recent advances, however, there is still lack of effective therapeutic tools that can be readily applied in the clinical setting.Citation3,Citation4 As a result, for the treatment of large wounds most clinicians rely on the long-standing and proven invasive surgical principles of debridement, temporary wound coverage and subsequent tissue reconstruction through skin transplantation or local/free (microsurgical) tissue transfer.Citation5–8 This approach faces, however, certain important limitationsCitation9: 1. Temporary wound coverage and wound preconditioning via negative-pressure wound therapy (NPWT)/vacuum-assisted closure (V.A.C.)), for prolonged periods of time until the wound bed is healthy enough for surgical reconstruction, requires surgical intervention and prolonged hospitalization, both of which can be costly, while it also significantly limits the patient’s mobility by interfering with daily activities. 2. Skin transplantation and local or free tissue transfer procedures are accompanied by risk of graft-take failure, donor site morbidity, and are also burdened by high cost. 3. The final appearance of the grafted site may significantly differ from that of surrounding native tissue, providing inferior aesthetic outcomes, while in cases where the wound is grafted with weak tissue (e.g. split-thickness skin grafts) or left to heal by secondary intention, the resulting scar contracture may limit the range of motion.
Regenerative therapy approaches toward dermal tissue repair have been attempted to remedy some of these limitations. A large body of work has focused on the utilization of bioactive scaffolds that can structurally fill the defect, while also acting as carriers to cells and growth factors that can directly promote tissue regeneration.Citation10–14 In order to overcome issues relating to cell-biomaterial interaction and foreign body reaction, mesenchymal stem cells (MSCs) have been employed, which have the ability to specifically adapt their fate according to the surrounding microenvironment.Citation10, Citation11, Citation15–17 While these strategies are indeed very promising for developing effective tools to deal with tissue damage and necrosis, most of them are complex and currently at an experimental stage.Citation3,Citation4 This might indeed explain the increasing interest toward harnessing the body’s own resources by using more simple approaches to enhance regeneration.Citation18 One of the few clinically available autologous therapies for treating chronic wounds through the stimulation of bioactive mechanisms is platelet-rich plasma (PRP).Citation19,Citation20 The PRP method utilizes a blood-derived growth factor product, obtained following peripheral blood centrifugation and platelet concentration.Citation18,Citation21 It is based on the broadly accepted notion that platelets are the key cellular players that orchestrate wound repair following trauma, by releasing a wide range of pre-stored regenerative growth factors. These include vascular endothelial growth factor (VEGF), platelet-derived growth factor (PDGF), fibroblast growth factor (FGF), EGF (epidermal growth factor), IGF-1 (insulin-like growth factor-1), bFGF (basic fibroblast growth factor) and TGF-beta1 (transforming growth factor beta-1).Citation22–25 However, the fact that platelets are also the primary source of strong inhibitors of angiogenesis (development of new blood vessels), such as platelet factor 4 (PF-4) and thrombospondin 1 (TSP-1), has been largely neglected.Citation26–29 Furthermore, from a chronological perspective, platelets primarily participate in the early wound healing phase, which starts immediately after vascular trauma and blood clotting, but since they lack nuclear DNA they cannot participate in new growth factor production.Citation30 Therefore, their conducting function is later on taken over by leukocytes (neutrophils, macrophages) as the main drivers of the inflammatory and proliferative phase.Citation31,Citation32 These crucial gaps in our current understanding of the multidimensional wound healing mechanisms might appear trivial, but in practice they may well limit our success when trying to implement an approach, such as PRP, that in retrospect appears incomplete. This could explain the lack of global recognition for PRP as a gold-standard regenerative treatment, despite many years of research and clinical application.
Through simple deterministic thinking, it is not difficult to see how peripheral blood might indeed hold the key to wound healing and dermal tissue regeneration, as this is effectively the main source of new repair signals (nutrients, cells, growth factors) following trauma.Citation33,Citation34 By acknowledging this key idea, we have studied over the past decade the hypothesis that the complete growth factor signaling component of the regenerative program could be derived through the extracorporeal exposure of peripheral blood cells (PBCs) to those very same conditions that are normally encountered within the wound microenvironment, i.e. physiological temperature and hypoxia, rather than blood centrifugation and platelet concentration.Citation33–37 A major finding that facilitates this approach has been the realization that local, i.e. pericellular hypoxia can readily be achieved in situ, by adjusting the PBC concentration (i.e. the PBC seeding density) within the blood incubation chamber, and therefore cellular oxygen consumption.Citation26,Citation33,Citation36 As such, we have found that conditioning peripheral venous blood within a conventional syringe at a blood volume per unit cross-sectional area (BVUA) of ≥1 mL/cm2 is sufficient for generating prolonged (7+ days) pericellular hypoxia (≤1% O2). This is important, since it effectively overcomes the need for creation of environmental hypoxia within (costly) hypoxic incubators, while it also offers a more physiological (i.e. cell-regulated) microenvironment for conditioning PBCs that can easily be achieved within a simple temperature-control incubator ().
Figure 1. Preparation of hypoxia preconditioned serum (HPS) using the method of hypoxia-adjusted in vitro preconditioning. Peripheral venous blood is allowed to coagulate within the blood-sampling syringe, once this is placed upright in the temperature-control incubator. Sedimentation passively separates serum at the top, from the fibrin clot in the middle layer and red blood cells (RBC) at the bottom. Peripheral blood cells (within the clot layer) are conditioned under physiological temperature (37oC) and local-pericellular (i.e. surrounding the cellular-clot layer) hypoxia (~1%O2, this is automatically generated within the ‘bioreactor’ syringe as a result of cellular O2 consumption) for a defined period of time, typically 4–7 days, leading to production/secretion of cell-derived protein factors into the serum. Growth factor-rich HPS is extracted at the end of the incubation period through sterile filtration to remove cellular debris. BVUA refers to the blood volume per unit cross-sectional area of the blood-containing syringe.
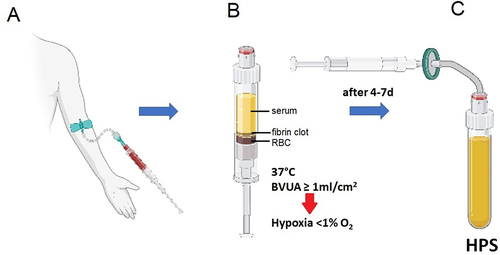
Since the concept of utilizing hypoxia preconditioned plasma as a tool for promoting tissue regeneration was first proposed,Citation33 we have shown that blood-derived hypoxia preconditioned secretomes (plasma or serum) are rich in protein growth factors (e.g. VEGF, PDGF, EGF, FGF) that can stimulate endothelial cell microvessel formation, angiogenic and lymphangiogenic sprouting and dermal fibroblast migration and proliferation in vitro,Citation28, Citation36, Citation37 as well as osteoblast proliferation, migration and matrix deposition.Citation38 Hypoxia preconditioned serum (HPS) has also recently been found effective at accelerating wound healing in mice.Citation39 Importantly, these secretomes appear to retain their bioactivity when derived from blood that is obtained from diabetic patients,Citation40 while at certain concentrations they also seem to have a significantly greater angiogenic potency than PRP when compared in vitro.Citation29 Furthermore, we could show that they can be extremely effective toward the treatment of small (<10 cm2) lower extremity venous ulcers.Citation37 Nonetheless, the effectiveness of this strategy as a treatment for large (>10 cm2) full-thickness wounds, where no healthy dermal tissue is available within the wound bed, has yet to be determined.
Of the various risk factors contributing to post-operative wound healing complications, cigarette smoking significantly increases risk. This is especially true when high skin tension is applied (e.g. after body-lift surgery) or skin perfusion is impaired by incision (e.g. flap plasties), due to the fact that nicotine promotes vasoconstriction, thus restricting blood supply to healing tissue.Citation41,Citation42 Such complications can be detrimental to patients’ psychological well-being and significantly limit their quality of life, both in the short-term due to prolonged hospitalization, as well as in the long-term as a result of bad scarring.Citation43–45 In this study, we present 6 cases of post-surgical wound-healing complications, including two severe cases in smokers after body-lift procedures, namely abdominoplasty and buttock-lift surgery, and a case of a wound healing complication following breast reduction surgery in the context of inflammatory pathology (pyoderma gangrenosum). In all cases topical application of peripheral blood-derived HPS growth factors, obtained through the method of hypoxia-adjusted in vitro preconditioning (), was tried as a last resort treatment due to lack of viable alternatives. The wounds in three of these cases had a large surface area (24–160 cm2), and developed after full-thickness skin necrosis, including the deep dermis, such that direct re-epithelialisation from adjacent areas was not possible. Also, since the wounds were located at points of maximum skin tension (all flexible local tissue appropriate for local wound closure was removed during the skin-tightening procedure), local tissue mobilization was initially not feasible. Complete wound closure could, nonetheless, be achieved using this conservative treatment approach by 60–135 days, without need for skin grafts, local/regional flaps or microsurgical free-tissue reconstruction. These results, in the context of the severity of these cases, suggest that this simple method is effective at promoting complete tissue repair, even in large full-thickness dermal wounds, most probably by targeting multiple cell-driven regenerative mechanisms. The findings of this pilot study will be informative toward designing a formal clinical trial for testing this approach against standard treatment controls.
Results
Small dermal wounds (surface area <10 cm2) − 3 cases
Three patients that had undergone body-lift procedures for aesthetic indications, namely vertical thigh-lift, full abdominoplasty and breast-lift surgery, developed wound healing complications in the areas of maximum tissue tension, i.e. in the (right) upper thigh, the central lower abdomen and in the (right) nipple-areolar complex, respectively. Wound surface area varied from 4 to 9 cm2. All wounds could be managed conservatively with mild debridement and daily local application of hypoxia preconditioned serum (10% HPS/hyaluronic acid-based ointment) obtained from the patient’s peripheral venous blood. Time to closure with complete wound epithelialization was 60 days for all patients ().
Figure 2. Treatment of small dermal wounds with topical application of hypoxia preconditioned serum (HPS) obtained from the patient’s peripheral blood. (a) Image panel showing a wound healing complication in the right inner thigh after a vertical thigh-lift procedure in a 30-year-old female smoker. Initial wound surface area was 6 cm2. Complete wound closure was achieved at 60 days post-debridement and initiation of treatment with daily application of an emulsion containing 10% HPS. (b) Image panel showing a wound healing complication at the lower central abdomen after an abdominoplasty procedure in a 40-year-old female smoker. Initial wound surface area was 4 cm2, while complete wound closure was achieved at 60 days post-debridement and initiation of treatment with daily application of an emulsion containing 10% HPS. (c) Image panel showing a wound healing complication (nipple-areola complex necrosis) in the right breast following a breast-lift procedure in a 22-year-old female patient. Initial wound surface area was 9 cm2. Complete wound closure was achieved at 60 days post-debridement and initiation of treatment with daily application of an emulsion containing 10% HPS. Debridement refers to surgical excision of necrotic tissue deep-down to well-perfused layers. The number of days indicated is counted from the time point of first treatment application. Bars = 1 cm.
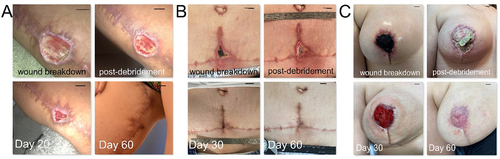
Large dermal wounds (surface area >10 cm2)
Case 1 – Ventral waistline full-thickness dermal wound
A 29-year-old female patient underwent a full lipo-abdominoplasty procedure (with re-insertion of the umbilicus) using a high-superior tension technique,Citation46 for lipodystrophy and lower abdominal skin laxity, following 30 Kg weight loss, and after having achieved a stable weight for the past 6 months. Since the patient was a heavy smoker (>10 pack years), she had been thoroughly informed about the risks of cigarette smoking with regards to wound healing complications post-abdominoplasty surgery and was advised to quit smoking for at least 30 days prior to and after the procedure. Despite this, the patient continued to smoke up until the day of the operation, as well as in the immediate post-operative period, without having informed her surgeon. Given the patient’s smoking habit, every care had been taken during the procedure to avoid applying high skin tension on the final closure. Nonetheless, full-thickness tissue necrosis, including the deep dermis and subcutaneous tissue to the level of the Scarpa’s fascia/anterior rectus sheath, developed in the vertical component of the scar (lower central abdomen), which appeared as extensive wound breakdown 2 weeks post-operatively (). The wound underwent radical surgical debridement to remove all necrotic tissue, followed by initiation of treatment with daily topical application of hypoxia preconditioned serum (10% HPS/hyaluronic acid-based ointment) obtained from the patient’s peripheral venous blood. Initial wound surface area immediately after debridement was 40 cm2, which in the subsequent 4 days had enlarged to 64 cm2, as the edema subsided. Granulation tissue formation within the wound bed was clearly first observed at 7 days after initiation of treatment, continuing its development up to 36 days. The wound surface area contracted by approx. 30% in the first 12 days post-initiation of treatment, thereafter following a more gradual reduction. Complete wound closure with full epithelialization was achieved by 135 days post-initiation of treatment (). A secondary corrective procedure was carried out for aesthetic reasons at this point, in order to excise the remaining scar tissue plate (surface area approx. 4 cm2) and achieve horizontal wound closure via local flap tissue transposition. The patient was satisfied with the aesthetic outcome of the final scar position and appearance.
Figure 3. Treatment of a large full-thickness wound in the ventral waistline with topical application of hypoxia preconditioned serum (HPS) obtained from the patient’s peripheral blood. (a) Image panel showing the progression of a wound healing complication in the lower abdomen following an abdominoplasty procedure in a 29-year-old female smoker. Initial wound surface area was 40 cm2. Complete wound closure was achieved at 135 days post-debridement and initiation of treatment with daily application of an emulsion containing 10% HPS. Debridement refers to surgical excision of necrotic tissue deep-down to well-perfused layers. The number of days indicated is counted from the time point of first treatment application. Bars= 1 cm. (b) Plot showing the wound surface area, measured by image analysis, vs. time (days post-initiation of treatment).
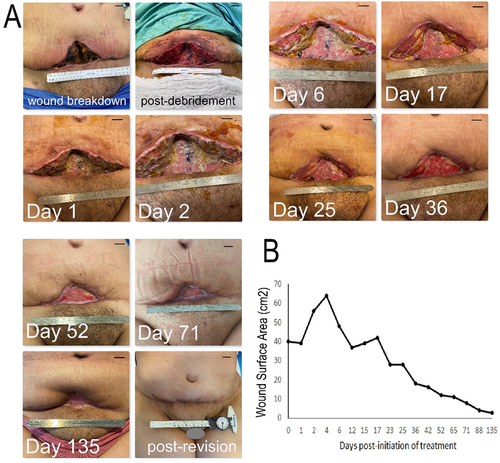
Case 2 – Dorsal waistline full-thickness dermal wound
A 49-year-old female heavy smoker underwent a lower body-lift (buttock-lift) procedure using the dermal fat flap (LP-flap) techniqueCitation47 for lipodystrophy and buttock ptosis. Despite medical advice, the patient continued to smoke in the pre-and post-operative period, and while an effort was made to avoid applying high skin tension, full-thickness tissue necrosis, including the deep dermis to the level of the fascial layer, developed across the full length of the dorsal skin closure, which appeared as extensive wound breakdown 7 days post-operatively (). The wound underwent aggressive surgical debridement to remove all necrotic tissue, together with bilateral full-thickness skin transplantation, using the lower abdomen as donor site. Seven days after the second procedure, it became evident that transplanted skin survival was partial and limited to the right side of the defect, while complete failure of graft take was visible on the left side, due to friction and sheer stress placed on the graft (and possibly continued nicotine use). Following removal of the non-viable skin graft, wound treatment with daily topical application of hypoxia preconditioned serum (10% HPS/hyaluronic acid-based ointment) obtained from the patient’s peripheral venous blood was initiated. Initial total wound surface area immediately after debridement was 160 cm2. Granulation tissue formation was clearly first observed 7 days after initiation of treatment, while the first visible signs of epithelialization appeared at 35 days. Wound contraction was rapid in the first 35 days post-treatment, reaching approx. 50% of initial surface area, thereafter becoming more gradual. Complete wound closure with full epithelialization was achieved by 98 days, with maturation of the epithelium by 210 days (). Interestingly, the final scar width on the graft-free left side appeared to be significantly smaller than the mean scar width on the right side (p < 0.05), where partial skin-graft take had occurred (). The patient was satisfied with the aesthetic outcome of the final scar, which could be further improved with fractional Erbium-Glass and Erbium-YAG laser resurfacing and medical tattooing.
Figure 4. Treatment of a large full-thickness wound in the dorsal waistline with topical application of hypoxia preconditioned serum (HPS) obtained from the patient’s peripheral blood. (a) Image panel showing the progression of a wound healing complication in the dorsal waistline following a buttock-lift procedure in a 49-year-old female smoker. Initial wound surface area was 160 cm2. Complete wound closure was achieved at 98 days post-debridement and initiation of treatment with daily application of an emulsion containing 10% HPS. The appearance of the mature scar following laser-resurfacing is shown at 210 days, with visible difference in mean scar width between left and right side. Debridement refers to surgical excision of necrotic tissue deep-down to well-perfused layers. The number of days indicated is counted from the time point of first treatment application. Bars= 1 cm. (b) Plot showing the wound surface area, measured by image analysis, vs. time (days post-initiation of treatment). (c) Plot comparing the mean width of the final scar that formed on the graft-free left side vs. that of the skin-grafted right side. Error bars refer to standard deviation of the mean, *p<0.05.
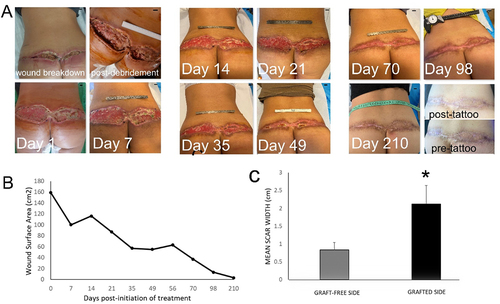
Case 3 – Breast full-thickness dermal wound
A 27-year-old nonsmoker female patient underwent a bilateral breast reduction procedure (using a superomedial pedicle with circumvertical scar)Citation48 for hypermastia and associated shoulder/back pain. At the time of surgery, no pathologies influencing wound healing were known (the patient did not inform her treating physician of her family history of inflammatory disease, including Crohn’s disease). Within the first week post-operatively, the wound edges appeared increasingly erythematous () and were significantly tender to touch bilaterally, while the patient complained of episodes of recuring pyrexia. Despite negative blood cultures, oral antibiotic treatment was initiated but with no improvement. At 10 days, a decision was made for an explorative procedure, at which the wound edges where carefully debrided. Wound swabs obtained were negative for bacterial growth. Following this procedure, and despite broad-spectrum intravenous antibiotic treatment, the wound edges maintained their inflamed appearance. At this point, a presumptive diagnosis was made for post-surgical pyoderma gangrenosum (PG), which while rare, does have a high association with breast surgery.Citation49–51 A tissue biopsy was obtained which revealed ulcerated skin with acute and chronic granulomatous necrotizing inflammation, characteristic of PG. Based on this, high-dose intravenous steroid treatment was initiated, with a positive response within 24 h seen as lack of fever and cessation of cellulitis progression. However, full-thickness wound breakdown, including the deep dermis and subcutaneous tissue, became evident bilaterally at multiple points along the vertical and horizontal suture lines at 24 h after the revision procedure. These ulcers enlarged up until 7 days and only stabilized in size at 10 days post-operatively (). At this point, treatment with daily topical application of hypoxia preconditioned serum (10% HPS/hyaluronic acid-based ointment) obtained from the patient’s peripheral venous blood was initiated. Initial wound surface area was 24 cm2 in the right breast and 23 cm2 in the left breast. Granulation tissue formation within the wound bed was clearly first observed at 8 days after initiation of treatment, continuing its development up to 34 days. The wound surface area contracted by approx. 30% in the right breast and 50% in the left breast within first 17 days post-initiation of treatment, respectively, thereafter following a more gradual reduction. Complete wound closure with full epithelialization was achieved by 80 days post-initiation of treatment, with maturation of the epithelium by 120 days (). The patient remained on oral steroid treatment tapered over 2 months, and was satisfied with the aesthetic outcome of the final scar position and appearance.
Figure 5. Treatment of large full-thickness wounds in the breast with topical application of hypoxia preconditioned serum (HPS) obtained from the patient’s peripheral blood. (a) Image panel showing the progression of a wound healing complication in the left and right breast that developed as a result of an inflammatory reaction following a breast reduction procedure in a 27-year-old female patient. Initial wound surface area was 23 and 24 cm2 in the left and right breast, respectively. Complete wound closure was achieved at 80 days post-debridement and initiation of treatment with daily application of an emulsion containing 10% HPS. Debridement refers to surgical excision of necrotic tissue deep-down to well-perfused layers. The number of days indicated is counted from the time point of first treatment application. Bars= 1 cm. (b) Plot showing the wound surface area, measured by image analysis, vs. time (days post-initiation of treatment).
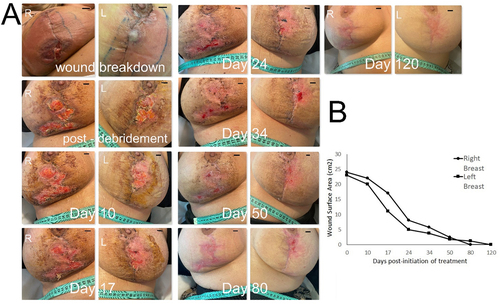
Discussion
Dermal tissue damage can occur as a result of mechanical trauma, burn injury (thermal/chemical), increased pressure (e.g. decubitus ulcers) and vasculopathy (e.g. diabetic ulcers),Citation31,Citation52 as well as autoimmune/inflammatory disease.Citation53 Post-surgical wound healing complications can arise when increased skin tension is applied, a situation aggravated by cigarette smoking due to nicotine-induced vasoconstriction and endothelial dysfunction.Citation41,Citation42,Citation54 Regardless of cause, skin necrosis removes the protective epidermal boundary which exposes the patient to infection. Thus, clinical difficulties increase significantly with wound surface area. In contrast to partial-thickness wounds, where the deep dermis remains intact and regeneration is possible from viable dermal appendages, full-thickness wounds present a much greater challenge since no dermal tissue is available from which repair can be initiated. Therefore, the mainstay treatment is aggressive surgical wound debridement to remove all necrotic tissue (up to well-perfused tissue layers), temporary wound coverage with application of vacuum-assisted closure (which aids wound contraction), and subsequent tissue reconstruction via skin grafting or local/free (microsurgical) tissue transfer.Citation5,Citation8,Citation55
Beyond the multiple issues relating to donor site morbidity, the classical tissue reconstruction strategy is burdened by high cost, prolonged patient hospitalization, as well as the risk of graft-take failure.Citation56 The latter is particularly relevant to transplanted body areas where increased pressure and/or shear stress is constantly present, such as in the second case (dorsal waistline wound) of this report. The severity of the presented cases, characterized by the large size of wounds at locations of high skin-tension, would typically demand repair through reconstructive micro-surgery. The ability to promote tissue regeneration by stimulating the natural repair mechanisms opens a new avenue for treatment of such problematic wounds. Furthermore, our findings suggest that under certain circumstances, reconstructive surgery may even limit new tissue synthesis and wound contraction by placing an inert “graft-spacer” between the healing wound edges that serves more like a block than being biologically “registered” by the body as a regenerative template (). Skin regeneration via “biological tissue expansion” essentially mimics the effects of surgical tissue expansion using an expander, while (theoretically) maintaining tissue thickness and strength (note; artificially expanded tissue shows dermal thinning due to pressure forces on skin,Citation57), as well as avoiding infection risk and other surgery-related complications. As such, classical tissue reconstruction might not only be unnecessary, but possibly even be counterproductive to the regenerative process, when applying this method. This was well evident in the second case presented here, where the final scar width on the successfully transplanted site was significantly larger than on the transplant-free site where graft-take failure occurred (), suggesting that skin grafting had limited the degree of wound contraction/skin regeneration. In this context, it is conceivable that naturally regenerated dermal-subdermal tissue may be more robust than split-thickness or even full-thickness skin grafts that, respectively, lack deep-dermal and subdermal support, and eventually undergo themselves contraction, rather than promote peripheral wound contraction (). The recent finding that epidermal-mesenchymal-transition (EMT) of keratinocytes can be triggered by cell stretching through Piezo1 signalingCitation58 may help to explain these findings. As the grafted part of the wound stays thinner, it is subject to more stretching than the intact surrounding skin, even at normal forces (this is exaggerated by graft contraction). This may lead to uncontrolled EMT of both keratinocytes from the wound edge and keratinocytes on the graft, which would lead to more scarring than full thickness regeneration, where first the dermis is restored to its full thickness and thereby the stretch-related EMT mechanics are also normalized.
Figure 6. Comparison of the tissue reconstruction vs. tissue regeneration principles. In surgical tissue reconstruction of a partial-thickness dermal wound (A) a graft is placed and fixed in the defect for replacement of damaged dermal tissue (B). While grafts immediately provide adequate wound coverage, they also act as ‘spacers’ that obstruct tissue growth from the free wound edges, thus preventing the dermis from growing back to its original height (blue arrows), which leaves the grafted area thinner. This thinner part will be mechanically weaker and therefore more stretched than the surrounding intact tissue (red arrows) under normal loading, with the risk of overstretching. Following full graft take and integration, grafts undergo themselves contraction, which can further reduce tissue elasticity and range of motion. C) a regenerative therapy aims at supporting/stimulating new tissue growth which first restores the full height of the dermis and then epidermal healing from the free wound edges, additionally to uninhibited wound contraction.
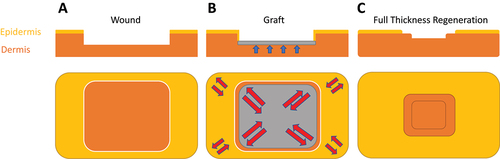
Our bodies’ inherent ability to spontaneously heal small wounds quite efficiently, generates the question, why does the wound healing program stall when the wound surface area increases beyond a certain limit. While this still remains unclear, we had previously hypothesized that cellular adaptation to stress, specifically hypoxia (the key trigger for angiogenesis) may hamper cellular function.Citation10,Citation35,Citation36,Citation59 The spatial component of a wound is intrinsically interlinked with its temporal component, as wound healing normally progresses at a defined rate; wound healing in large wounds may progress at a slow pace relative to the spatial distance that needs to be covered (from the oxygenated free wound edge to the hypoxic wound center), which may result in cellular habituation to hypoxic stress, consequently altering the cell-driven angiogenic response. Without adequate vascular (i.e. nutrient/oxygen) supply, the regenerative cellular processes cannot operate optimally; therefore, impaired angiogenesis is likely the main cause for impaired cell differentiation, migration, proliferation and tissue remodeling. This could explain the ineffectiveness of passive wound healing treatments (e.g. dressings, vacuum-assisted closure, hydrating ointments etc.) to promote the repair of large wounds, despite the fact that they are beneficial for small wounds,Citation60 since they lack the ability to provide bioactive support to the key angiogenic and contractile mechanisms.
Hypoxia preconditioned blood-derived secretomes comprise the complete regenerative growth factor signaling that naturally drives angiogenesis and wound healing in the body.Citation28,Citation29,Citation36,Citation37,Citation39,Citation59 We previously demonstrated in multiple studies the superiority of hypoxia preconditioned plasma and serum to their non-preconditioned counterparts, in terms of their ability to promote angiogenesis and lymphangiogenesis.Citation28,Citation29 The proposed method of hypoxia-adjusted in vitro preconditioning makes it possible to harvest these secretomes on demand, and apply them strategically, i.e. repeatedly to the wound, thus overcoming the limit of gradual cellular habituation to hypoxic stress. This approach effectively “converts” a large wound into a series of smaller wounds that sequentially heal from its dynamic edge toward the center, which was evident here by the successful healing of wounds at various body locations, having different sizes and topographies, over comparable time-frames. Interestingly, and in agreement with our previous data, high dilutions of the native secretome growth factor concentration (e.g. 1:5 to 1:10, as used here) seem to be effective at accelerating wound healing,Citation29,Citation37,Citation39 which emphasizes the tremendous regenerative power that can be harnessed from a small volume of peripheral blood. Provided that there is significant variation in terms of HPS growth factor concentrations between individuals,Citation37,Citation40 it is reasonable to argue that utilization of an autologous approach is not only safe, but also guarantees an “automatic” match between secretome composition and biological requirements, since it appears that the precise relative stoichiometry of growth factors is more important than their absolute concentrations. Even more impressive seems to be the ability of these secretomes to promote wound healing in heavy smokers (shown in cases 1 & 2), where tissue perfusion is restricted,Citation41,Citation42 as well as in a setting of inflammatory-autoimmune pathology (shown in case 3), which highlights their ability to improve/regulate cellular responses by resetting physiological homeostasis.
Importantly, the findings of the cases presented demonstrate that this type of signaling has the capacity to promote tissue regeneration in large full-thickness wounds, and not merely the generation of scar tissue, despite the fact that the wound edges were far apart (which prevented wound closure simply by contraction), and that no or little dermal tissue was available in the wound bed from which outgrowth could have initially occurred. As previously shown by our in vitro and in vivo data,Citation26,Citation28,Citation29,Citation36,Citation37,Citation39 this was likely made possible through stimulation of multiple cellular processes, including angiogenesis (seen here as early granulation tissue formation), lymphangiogenesis (as evident by the reduction of edema in the wound edges and eventual cessation of wound secretions), cell differentiation, migration and proliferation (which are prerequisites for covering such large distances/surface areas), tissue remodelling/contraction (evident as reduction in wound surface area) and epithelialization (creation of an intact, mature epidermal barrier). What is perhaps unexpected is that these processes appear to have the capacity to progress at a variable pace, depending upon the exogenous bioactive support provided, rather than at a predefined (i.e. self-limiting) speed, as suggested by current wound healing theory. As such, it seems possible that this approach may not only be supportive to the wound healing program at multiple stages, but it could also reactivate any particular phase when this has come to a complete halt, with significant implications for the treatment of chronic wounds.
The method described here is simple enough to be applied clinically, while the treatment itself can be carried out by patients (or carer givers) at home, without the need for prolonged hospitalization. The only piece of equipment required is a temperature-control incubator, which is generally affordable. This therapy could be of great value in the treatment of chronic (e.g. diabetic) wounds and wound healing complications following aesthetic procedures, by offering a true “like-with-like” tissue replacement, instead of tissue substitution via transplantation, while also avoiding the creation of donor sites. While its application in settings of acute trauma may be complementary to first-line approaches offering rapid wound closure (when urgency of infection prevention is greater than the quality of cover), it could also find utility in high-risk scenarios, where blood could be pre-sampled from personnel (e.g. fire fighters, rescue personnel, soldiers) in anticipation of traumatic injury. Beyond offering a useful therapeutic tool, the utility of this approach as a preventive treatment (via injectable application) in cases where there is high risk of wound healing complications due to inadequate tissue perfusion (e.g. in smokers, diabetics, high skin-tension surgical sites) may also be worth exploring. This strategy may also lay a platform for an idea that has long been proposed, yet to this day remains only theoretical, namely that of “in vivo tissue engineering.” Indeed, the ability to generate new tissue, on demand, by actively supporting key cellular processes holds great potential for developing regenerative therapies to repair damaged tissue of dermal or other origin in the future. Finally, the results of this pilot study will help to design a formal clinical trial for examining the effect of this approach against conventional treatment controls.
Methods
Ethical approval
This study protocol was formulated in accordance with the requirements of the Declaration of Helsinki of the World Medical Association. All patients undergoing treatment provided written informed consent prior to participation as directed by the ethics committee of the Lefkos Stavros Hospital Athens, Greece (protocol number 1186 35/08042021) where the primary surgical procedures had been carried out.
Patient selection
The patients presented in this case series underwent thigh-lift, abdominoplasty, buttock-lift and breast-lift/reduction procedures for aesthetic indications. The primary operations were carried out at the Lefkos Stavros Hospital (Athens, Greece) by the primary author. The patients that underwent thigh-lift, abdominoplasty and buttock-lift procedures were heavy smokers (>10 pack years), who despite medical advice, continued to heavily smoke up until prior to and after their surgery, without having informed their surgeon. The patient that underwent breast reduction was a nonsmoker who had a family history of inflammatory disease but failed to inform the treating physicians of this prior to surgery. Exclusion criteria were presence of type II diabetes mellitus, cardiovascular pathology and treatment with anti-inflammatory medication prior to initiation of treatment. In all patients involved, tissue necrosis was already fully demarcated at the first follow up, when treatment options were examined. In all cases topical application of peripheral blood-derived HPS growth factors was tried as a last resort treatment due to lack of viable alternatives. All patients had declined conventional surgical treatment of their wound healing complications with vacuum-assisted closure and subsequent tissue reconstruction using local/free flap.
Preparation of hypoxia preconditioned serum (HPS) from peripheral blood
Hypoxia preconditioned serum (HPS) was prepared as previously described.Citation37 Briefly, peripheral venous blood (15 mL) was collected from each patient into a 20 ml polypropelene syringe (OmnifixⓇ, B Braun AG, Melsungen, Germany) four days prior to beginning of the treatment. For initial blood-gas equilibration, 5 ml of air were drawn into the syringe through a 0.2 µm filter (SterifixⓇ, B Braun AG, Melsungen, Germany), with the plunger fully retracted. Subsequently, the syringe was placed upright in the incubator (37°C) and incubated for 4 days, effectively using the syringe as bioreactor. Pericellular (local) hypoxia (~1% O2) was generated in situ (within the syringe) through peripheral blood cell (PBC)-mediated O2 consumption, by adjustment of the blood volume per unit cross-sectional area (BVUA ≥1 mL/cm2) within the blood-containing syringe, and consequently, the PBC seeding density.Citation26,Citation33 After incubation, the blood was separated through sedimentation into three layers (from top to bottom: serum, fibrin clot, red blood cell component, see ), so that the top layer (HPS) could be filtered (SterifixⓇ, B Braun AG, Melsungen, Germany) into a new syringe, removing cells/cellular debris.
Preparation of HPS-containing topical product
As a vehicle for controlled delivery of the HPS protein factors, sterile-filtered HPS was mixed into a hydrophilic hyaluronic acid-based ointment (Pharmathink P.C, Greece) at a concentration of 10%, using a sterile wooden spatula. Specifically, 5 mL HPS obtained from 15 mL peripheral blood was mixed into 45 ml ointment base, to a final volume of 50 mL. This preparation was stored at +5°C and was brought to room temperature only during use. The maximum duration of use of each 50 mL preparation was four weeks.
Wound treatment with topical HPS application
The wounds initially underwent formal surgical debridement under local anesthesia and patient sedation in order to remove all necrotic tissue and achieve a well-perfused wound bed. The wound was cleaned daily with a 10% iodine solution (BetadineⓇ, Avrio Health L.P, USA), followed by rinsing with normal saline, and let to air-dry for 15 min. After this, the HPS-containing ointment preparation was applied to the wound (wound bed and side walls) through light massage using sterile gloves. Five minutes were allowed for adsorption, before the wound was covered with sterile paraffin-gauze (Jelonet, Smith+Nephew, USA) and sterile cotton gauzes. The bandage was fixed with paper tape. Wound treatment, including sterile bandage change was carried out once daily, by trained nursing stuff for the first two weeks, and later on by the patient at home, until full wound closure was achieved.
Image analysis
Digital photographs of the wounds were taken using a Nikon DSLR D780 camera (Nikon Corporation, USA) on the day of first identification of the post-operative wound complication, followed by the time point of surgical debridement and then daily from the beginning of treatment. Time to closure was defined as the time at which the wound bed was completely epithelialized, clinically confirmed by the absence of wound secretion. Wound area was analyzed by tracing the wound margin and calculating the area (in cm2 using ImageJ software Images (NIH, Bethesda, MD, USA, version 1.53)Citation61) by a blinded observer.
Statistical analysis
Data sets were analyzed by paired t-test. All values are expressed as means ± standard deviation of the mean. A value of p < 0.05 was considered statistically significant (* p < 0.05).
Author contributions
Conceptualization: HE, SFA; methodology: HE, DU; software: MP, SFA validation: SFA, MP, JJ; formal analysis: HE, SFA, MP; resources: HE, MHG; data curation: HE, SFA; writing – original draft preparation: HE, SFA, DU; writing – review and editing: HE, SFA, DU, JJ, MP, MHG; visualization: HE, SFA; supervision: HE, SFA, MHG; project administration: HE, MHG; funding acquisition: HE, MHG. All authors have read and agreed to the published version of the manuscript.
Acknowledgments
The patients presented in this work were treated pro bono. The authors thank Dr. Kai Böker for help with generating Figure 1 created with Biorender.com. Special thanks also go to Mrs Mia Chramas for her kind assistance with medical tattooing in case 2.
Disclosure statement
MHG holds a patent entitled “DEVICE-BASED METHODS FOR LOCALISED DELIVERY OF CELL-FREE CARRIERS WITH STRESS-INDUCED CELLULAR FACTORS” (WO2013113821, Inventors: SCHILLING, Arndt, HADJIPANAYI, Ektoras, MACHENS, Hans-Günther) associated with the cell-free delivery of stress-induced cellular growth factors.
Additional information
Funding
References
- Gurtner GC, Chapman MR. Regenerative medicine: charting a new course in wound healing. Adv Wound Care (New Rochelle). 2016;5(7):314–15. doi:10.1089/wound.2015.0663.
- Owlarn S, Klenner F, Schmidt D, Rabert F, Tomasso A, Reuter H, Mulaw MA, Moritz S, Gentile L, Weidinger G, et al. Generic wound signals initiate regeneration in missing-tissue contexts. Nat Commun. 2017;8(1):2282. doi:10.1038/s41467-017-02338-x.
- Veith AP, Henderson K, Spencer A, Sligar AD, Baker AB. Therapeutic strategies for enhancing angiogenesis in wound healing. Adv Drug Deliv Rev. 2019;146:97–125. doi:10.1016/j.addr.2018.09.010.
- Dehkordi NA, Babaheydari MF, Chehelgerdi M, Dehkordi SR. Skin tissue engineering: wound healing based on stem-cell-based therapeutic strategies. Stem Cell Res Ther. 2019;10:111. doi:10.1186/s13287-019-1212-2.
- Levin S. Principles of definitive soft tissue coverage with flaps. J Orthop Trauma. 2008;22(10 Suppl):161–66. doi:10.1097/BOT.0b013e318188e2ed.
- Leypold T, Schäfer B, Boos AM, Beier JP. Plastic surgery reconstruction of chronic/non-healing wounds. Surg Technol Int. 2020;38:65–71. doi:10.52198/21.STI.38.WH1371.
- Kozusko SD, Liu X, Riccio CA, Chang J, Boyd LC, Kokkalis Z, Konofaos P. Selecting a free flap for soft tissue coverage in lower extremity reconstruction. Injury. 2019;50(5):32–S39. doi:10.1016/j.injury.2019.10.045.
- Moog P, Jensch M, Betzl J, Bauer A-T, Cerny MK, Schmauss D, Kükrek H, Erne H, Machens H-G, Megerle K, et al. Bacterial bioburden of wounds: influence of debridement and negative-pressure wound therapy (NPWT). J Wound Care. 2021;30(8):604–11. doi:10.12968/jowc.2021.30.8.604.
- Frykberg RG, Banks J. Challenges in the treatment of chronic wounds. Adv Wound Care (New Rochelle). 2015;4(9):560–82. doi:10.1089/wound.2015.0635.
- Hadjipanayi E, Cheema U, Mudera V, Deng D, Liu W, Brown RA. First implantable device for hypoxia-mediated angiogenic induction. J Control Release. 2011;153(3):217–24. doi:10.1016/j.jconrel.2011.03.029.
- Hadjipanayi E, Cheema U, Hopfner U, Bauer A, Machens HG, Schilling AF. Injectable system for spatio-temporally controlled delivery of hypoxia-induced angiogenic signalling. J Control Release. 2012;161(3):852–60. doi:10.1016/j.jconrel.2012.04.048.
- Ishihara M, Kishimoto S, Takikawa M, Hattori H, Nakamura S, Shimizu M. Biomedical application of low molecular weight heparin/protamine nano/micro particles as cell- and growth factor-carriers and coating matrix. Int J Mol Sci. 2015;16(12):11785–803. doi:10.3390/ijms160511785.
- Tomblyn S, Pettit, kneller EL., Walker, SJ, Ellenburg, MD, Kowalczewski, CJ, Van Dyke, M, Burnett, L, Saul, JM, et al. Keratin hydrogel carrier system for simultaneous delivery of exogenous growth factors and muscle progenitor cells. J Biomed Mater Res B Appl Biomater. 2016;104(5):864–79. doi:10.1002/jbm.b.33438.
- Alarçin E, Lee TY, Karuthedom S, Mohammadi M, Brennan MA, Lee DH, Marrella A, Zhang J, Syla D, Zhang YS, et al. Injectable shear-thinning hydrogels for delivering osteogenic and angiogenic cells and growth factors. Biomater Sci. 2018;6(6):1604–15. doi:10.1039/C8BM00293B.
- Sorrell JM, Caplan A. Topical delivery of mesenchymal stem cells and their function in wounds. Stem Cell Res Ther. 2010;1(4):30. doi:10.1186/scrt30.
- Hassan WU, Greiser U, Wang W. Role of adipose-derived stem cells in wound healing. Wound Repair Regen. 2014;22(3):313–25. doi:10.1111/wrr.12173.
- Maranda EL, Rodriguez-Menocal L, Badiavas EV. Role of mesenchymal stem cells in dermal repair in burns and diabetic wounds. Curr Stem Cell Res Ther. 2017;12(1):61–70. doi:10.2174/1574888X11666160714115926.
- Laschke MW, Menger MD. The simpler, the better: tissue vascularization using the body’s own resources. Trends Biotechnol. 2022;40(3):281–90. doi:10.1016/j.tibtech.2021.07.002.
- Chicharro-Alcántara D, Rubio-Zaragoza M, Damiá-Giménez E, Carrillo-Poveda J, Cuervo-Serrato B, Peláez-Gorrea P, Sopena-Juncosa J. Platelet rich plasma: new insights for cutaneous wound healing management. J Funct Biomater. 2018;9(1):10. doi:10.3390/jfb9010010.
- Carter MJ, Fylling CP, Parnell LK. Use of platelet rich plasma gel on wound healing: a systematic review and meta-analysis. Eplasty. 2011;11:38.
- Everts P, Onishi K, Jayaram P, Lana JF, Mautner K. Platelet-rich plasma: new performance understandings and therapeutic considerations in 2020. Int J Mol Sci. 2020;21(20):7794. doi:10.3390/ijms21207794.
- Marx RE. Platelet- Rich Plasma (Prp): what is PRP and what is not PRP? Implant Dent. 2001;10(4):225–28. doi:10.1097/00008505-200110000-00002.
- Sclafani AP. Applications of platelet-rich fibrin matrix in facial plastic surgery. Facial Plast Surg. 2009;25(4):270–76. doi:10.1055/s-0029-1242033.
- Van Den Dolder J, Mooren R, Vloon APG, Stoelinga PJW, Jansen JA. Platelet-rich plasma: quantification of growth factor levels and the effect on growth and differentiation of rat bone marrow cells. Tissue Eng. 2006;12(11):3067–73. doi:10.1089/ten.2006.12.3067.
- Aghaloo TL, Moy P, Freymiller DEG. Investigation of platelet-rich plasma in rabbit cranial defects: a pilot study. Oral Maxillofac Surg. 2002;60(10):1176–81. doi:10.1053/joms.2002.34994.
- Hadjipanayi E, Kuhn P-H, Moog P, Bauer A-T, Kuekrek H, Mirzoyan L, Hummel A, Kirchhoff K, Salgin B, Isenburg S, et al. The fibrin matrix regulates angiogenic responses within the hemostatic microenvironment through biochemical control. PLoS One. 2015;10(8):1–20. doi:10.1371/journal.pone.0135618.
- Martinez-Zapata MJ, Martí-Carvajal AJ, Solà I, Expósito JA, Bolíbar I, Rodríguez L, Garcia J, Zaror C. Autologous platelet-rich plasma for treating chronic wounds. AR Cochrane Database Syst Rev. 2016;25(5):1–56. doi:10.1002/14651858.CD006899.pub3.
- Moog P, Schams R, Schneidinger A, Schilling AF, Machens H-G, Hadjipanayi E, Dornseifer U. Effect of hypoxia preconditioned secretomes on lymphangiogenic and angiogenic sprouting: an in vitro analysis. Biomedicines. 2020;8(9):365. doi:10.3390/biomedicines8090365.
- Moog P, Kirchhoff K, Bekeran S, Bauer A-T, von Isenburg S, Dornseifer U, Machens H-G, Schilling AF, Hadjipanayi E. Comparative evaluation of the angiogenic potential of hypoxia preconditioned blood-derived secretomes and platelet-Rich Plasma: an in vitro analysis. Biomedicines. 2020;8(1):16. doi:10.3390/biomedicines8010016.
- Melchinger H, Jain K, Tyagi T, Hwa J. Role of platelet mitochondria: life in a nucleus-free zone. Front Cardiovasc Med. 2019;29(6):153. doi:10.3389/fcvm.2019.00153.
- Wilkinson HN, Hardman M. Wound healing: cellular mechanisms and pathological outcomes. Open Biol. 2020;10(9):200–23. doi:10.1098/rsob.200223.
- Reinke JM, Sorg H. Wound repair and regeneration. Eur Surg Res. 2012;49(1):35–43. doi:10.1159/000339613.
- Hadjipanayi E, Schilling AF. Regeneration through autologous hypoxia preconditioned plasma. Organogenesis. 2014;10(2):164–69. doi:10.4161/org.29208.
- Hadjipanayi H, Bekeran S, Moog P. Extracorporeal wound simulation as a foundation for tissue repair und regeneration therapies. Int J Transplant & Plastic Surg. 2016;2:1–10.
- Hadjipanayi E, Brown RA, Mudera V, Deng D, Liu W, Cheema U. Controlling physiological angiogenesis by hypoxia-induced signaling. J Control Release. 2010;146(3):309–17. doi:10.1016/j.jconrel.2010.05.037.
- Hadjipanayi E, Bauer AT, Moog P, Salgin B, Kuekrek H, Fersch B, Hopfner U, Meissner T, Schlüter A, Ninkovic M, et al. Cell-free carrier system for localised delivery of peripheral blood cell-derived engineered factor signaling: towards development of a one-step device for autologous angiogenic therapy. J Control Release. 2013;169(1–2):91–102. doi:10.1016/j.jconrel.2013.04.008.
- Hadjipanayi E, Moog P, Bekeran S, Kirchhoff K, Berezhnoi A, Aguirre J, Bauer A-T, Kükrek H, Schmauss D, Hopfner U, et al. In vitro characterization of Hypoxia Preconditioned Serum (HPS)—fibrin hydrogels: basis for an injectable biomimetic tissue regeneration therapy. J Funct Biomater. 2019;10(2):1–28. doi:10.3390/jfb10020022.
- Jiang J, Röper L, Alageel S, Dornseifer U, Schilling AF, Hadjipanayi E, Machens H-G, Moog P. Hypoxia Preconditioned Serum (HPS) promotes osteoblast proliferation, migration and matrix deposition. Biomedicines. 2022;10(7):1631. doi:10.3390/biomedicines10071631.
- Jiang J, Kraneburg U, Dornseifer U, Schilling AF, Hadjipanayi E, Machens H-G, Moog P. Hypoxia Preconditioned Serum (HPS)-hydrogel can accelerate dermal wound healing in mice—an in vivo pilot study. Biomedicines. 2022;10:176. doi:10.3390/biomedicines10010176.
- Moog P, Jensch M, Hughes J, Salgin B, Dornseifer U, Machens H-G, Schilling AF, Hadjipanayi E. Use of oral anticoagulation and diabetes do not inhibit the angiogenic potential of hypoxia preconditioned blood-derived secretomes. Biomedicines. 2020;8(8):283. doi:10.3390/biomedicines8080283.
- Rinker B. The evils of nicotine: an evidence-based guide to smoking and plastic surgery. Ann Plast Surg. 2013;70(5):599–605. doi:10.1097/SAP.0b013e3182764fcd.
- Xu Z, Norwich-Cavanaugh A, Hsia HC. Can nicotine replacement therapy decrease complications in plastic surgery? Ann Plast Surg. 2019;83(1 Suppl):55–58. doi:10.1097/SAP.0000000000002095.
- Monstrey S, Middelkoop E, Vranckx JJ, Bassetto F, Ziegler UE, Meaume S, Téot L. Updated scar management practical guidelines: noninvasive and invasive measures. J Plast Reconstr Aesthet Surg. 2014;67(8):1017–25. doi:10.1016/j.bjps.2014.04.011.
- Lipman K, Wang M, Berthiaume E, Holloway J, Da Lio A, Ting K, Soo C, Zheng Z. Evaluating current scar assessment methods. Ann Plast Surg. 2020;84(2):222–31. doi:10.1097/SAP.0000000000002029.
- Ogawa R. The most current algorithms for the treatment and prevention of hypertrophic scars and keloids: a 2020 update of the algorithms published 10 years ago. Plast Reconstr Surg. 2022;149(1):79–94. doi:10.1097/PRS.0000000000008667.
- Le Louarn C, Pascal JF. The high-superior-tension technique: evolution of lipoabdominoplasty. Aesthetic Plast Surg. 2010;34(6):773–81. doi:10.1007/s00266-010-9551-5.
- Pascal JF, Le Louarn C. Remodeling bodylift with high lateral tension. Aesthetic Plast Surg. 2002;26(3):223–30. doi:10.1007/s00266-002-1478-z.
- Serra MP, Longhi P, Sinha M. Breast reduction with a superomedial pedicle and a vertical scar (Hall-Findlay’s technique): experience with 210 consecutive patients. Ann Plast Surg. 2010;64(3):275–78. doi:10.1097/SAP.0b013e3181b0a611.
- Ehrl DC, Heidekrueger PI, Broer PN. Pyoderma gangrenosum after breast surgery: a systematic review. J Plast Reconstr Aesthet Surg. 2018;71(7):1023–32. doi:10.1016/j.bjps.2018.03.013.
- Simon AM, Khuthailia D, Hammond DC, Andres A. Pyoderma gangrenosum following reduction mammaplasty. Can J Plast Surg. 2006;14(1):37–40. doi:10.1177/229255030601400109.
- Doren EL, Aya-Ay M. Pyoderma gangrenosum following breast reduction: treatment with topical tacrolimus and steroids. Aesthet Surg J. 2014;34(3):394–99. doi:10.1177/1090820X13520448.
- Gurtner GC, Werner S, Barrandon Y, Longaker MT. Wound repair and regeneration. Nature. 2008;453(7193):314–21. doi:10.1038/nature07039.
- Kirloskar KM, Dekker PK, Kiene J, Zhou S, Bekeny JC, Rogers A, Zolper EG, Fan KL, Evans KK, Benedict CD, et al. The relationship between autoimmune disease and disease-modifying antirheumatic drugs on wound healing. Adv Wound Care (New Rochelle). 2022;11(12):650–56. doi:10.1089/wound.2021.0150.
- Zheng YL, Wang WD, Li MM, Lin S, Lin HL. Updated role of neuropeptide Y in nicotine-induced endothelial dysfunction and atherosclerosis. Front Cardiovasc Med. 2021;23(8):630968. doi:10.3389/fcvm.2021.630968.
- Lutz DA, Hallock GG. Microsurgical transfer of vascularized tissue to close problem wounds. Aorn J. 1995;62(2):234-238, 240, 242–233. doi:10.1016/S0001-2092(06)63655-7.
- Kozak GM HJ, RB B, Broach RB, Shakir S, Calvert C, Stranix JT, Messa C, Levin LS, Serletti JM, Kovach SJ, et al. Comparative effectiveness analysis of complex lower extremity reconstruction: outcomes and costs for biologically based, local tissue rearrangement, and free flap reconstruction. Plast Reconstr Surg. 2020;145(3):608–16. doi:10.1097/PRS.0000000000006589.
- Johnson PE, Kerahan D, Bauer BS. Dermal and epidermal response to soft-tissue expansion in the pig. Plast Reconstr Surg. 1998;81(3);390–97.
- He J, Shan S, Li Q, Fang B, Xie Y. Mechanical stretch triggers epithelial-mesenchymal transition in keratinocytes through piezo1 channel. Front Physiol. 2022;13:745572. doi:10.3389/fphys.2022.745572.
- Hadjipanayi E, Schilling AF. Hypoxia- based strategies for angiogenic induction. Organogenesis Landes Bioscience. 2013;9(4):1–12. doi:10.4161/org.25970.
- Pappalardo V, Frattini F, Ardita V, Rausei S. Negative Pressure Therapy (NPWT) for management of surgical wounds: effects on wound healing and analysis of devices evolution. Surg Technol Int. 2019;34:56–67.
- Schneider CA, Rasband WS, Eliceiri KW. NIH Image to ImageJ: 25 years of image analysis. Nat Methods. 2012;9(7):671–75. doi:10.1038/nmeth.2089.