Abstract
Regulation of small RNAs by other non-coding RNAs is a ubiquitous feature of gene regulatory systems that can be exploited by viruses. Examples of this have been described in 3 different herpesviruses, where viral non-coding RNAs bind to highly abundant cellular (miRNAs), mediating their degradation: miR-27 is targeted by both murine cytomegalovirus and herpesvirus saimiri, while the miR-17 family is targeted by human cytomegalovirus. We review what is known about RNA-mediated regulation of miRNA stability and propose 3 potential roles that viral non-coding RNAs might assume to initiate the destruction of a miRNA, acting as “recruiters,” “localizers” or “exposers.” Whereas the miRNAs (miR-17 and miR-27) appear to be ancient and pre-date the common ancestor of all mammalian herpesviruses, comparative analyses of herpesvirus genomes indicate that the 3 known viral regulators of miRNA each evolved independently, and much more recently. Noting that the anti-viral activity of miRNAs might be countered by a variety of mechanisms, we propose that (i) there has been continual turnover of these mechanisms during herpesvirus evolution, and (ii) there may be many other, as yet undescribed, anti-miRNA activities encoded by other herpesviruses and indeed by viruses from other families.
Introduction
Viruses use diverse mechanisms to manipulate cellular gene expression toward conditions that are conducive to replication or persistence and this is known to include, in some viral families, the use of viral-encoded microRNAs (miRNAs) or viral-encoded miRNA inhibitors.Citation1 miRNAs are a class of small non-coding RNA that post-transcriptionally regulate gene expression. They derive from stem loop structures generally contained within Pol II transcripts, which are recognized and processed by RNase III enzymes in the nucleus (Drosha) and the cytoplasm (Dicer), yielding a short double-stranded RNA duplex, on average 22 nt long. The duplex RNA is transferred to an Argonaute (Ago) protein in the RNA-induced silencing complex (RISC) and one strand, the functional miRNA, is selectively maintained whereas the other “passenger” strand dissociates and is degraded (reviewed inCitation2). The mature miRNA then guides RISC to specific mRNAs through base pair recognition, resulting in inhibited translation and/or accelerated de-adenylation of the mRNA.Citation3 Specificity is largely dictated by perfect complementarity between the target and nucleotides 2–8 of the miRNA, termed the “seed site.”Citation4 An individual miRNA can therefore interact with hundreds of different mRNA targets and indeed the majority of human protein-coding genes contain miRNA-binding sites retained under selective pressure.Citation5 Some viral transcripts also contain functional binding sites for host or virus-derived miRNAs. Therefore four different classes of miRNA-target interaction can impact the outcome of an infection: host-host, host-virus, virus-host or virus-virus. There is extensive diversity in the functions of interactions in each class, depending on the miRNA, the target, the cell type, and the stage in the viral life cycle – reviewed and referenced in depth elsewhere.Citation6-8 Here our aim is to consider the mechanism and potential breadth of one specific mode of host miRNA-virus interaction, where targeting works in the opposite direction and the viral RNA causes the degradation of the cellular miRNA to which it binds.
Widespread RNA-mediated regulation of small RNAs
Regulation of miRNAs by other RNAs was first seen and coined “target mimicry” in plants, where a non-coding RNA induced during phosphate starvation binds to miR-399 and relieves the repression of its target, a phosphate storage regulator.Citation9 A similar phenomenon occurs in bacteria where mRNAs and non-coding RNAs induced in response to environmental cues bind to, and inhibit, small RNAs that regulate sugar metabolism.Citation10,11 Seitz postulated that competition between miRNA targets likely occurs naturally in cells and might explain why some targets do not appear important in physiological assays: they have evolved miRNA-binding sites so that they can regulate the miRNA, rather than to be regulated by the miRNA.Citation12 This concept was developed as the “cellular endogenous RNA” (ceRNA) hypothesis to coincide with additional studies confirming that mRNAs, pseudogenes and various non-coding RNAs including circular RNAs can serve roles as competitive inhibitors of a miRNA, reviewed further inCitation13. Further studies suggest competition may only occur in a very narrow range of miRNA and target concentrations, limiting the extent to which this may be relevant under physiological conditionsCitation14,15. Nonetheless this phenomena can be exploited to artificially inhibit miRNAs in animal cells: transgenes with multiple miRNA binding sites (termed “miRNA sponges”) were developed several years ago to sequester miRNAs from binding to their normal targets.Citation9,16
Targeted host microRNA degradation by herpesviruses
Three evolutionarily distinct cases of miRNA degradation by non-coding RNAs in herpesviruses have been described. In 2010, we reported that miR-27 is rapidly down-regulated in mouse cells infected with murine cytomegalovirus (MCMV), resulting in >90% reduction by 24 hours post-infection.Citation17 This observation was intriguing because the half-lives of most miRNAs are reported to be in the range of daysCitation18. We and others subsequently identified an MCMV transcript that directly associates with mature miR-27 and mediates its degradation.Citation19,20 The miR-27 binding site is within the 3′ UTR of the m169 gene and mutation of this binding site results in virus attenuation following in vivo infection.Citation20 Also in 2010, a distantly related herpesvirus from squirrel monkeys, Herpesvirus saimiri (HVS), was shown to produce a small non-coding RNA, termed HSUR-1, which binds to the same cellular miRNA, and also mediates its degradation;Citation21 this is thought to be involved in activation and potentially transformation of T-cells. Intriguingly, HSUR-1 and the m169 3′ UTR share no sequence similarity, except in the short miR-27 binding site. More recently, human cytomegalovirus (HCMV) has been shown to produce a non-coding RNA that binds to members of a different miRNA family, miR-17, causing their degradation. Five of the miR-17 family members (with conserved seed sites and near identical 3′end sequences) are all downregulated during infection: miR-17, miR-20a, miR-20b, miR-93 and miR-106b. The downregulation of these miRNAs was found to accelerate virus production during lytic infection.Citation22 HCMV and MCMV are both members of the β subfamily of herpesviruses, but their anti-miRNA activities share no obvious common ancestry since their sequences are quite different and the adjacent genes share no similarity. In none of these cases has the actual degradation mechanism yet been characterized, but it seems likely that herpesviruses tap into, and exploit, a host miRNA turnover pathway.
Destructive regulators of miRNAs: recruiters, localizers or exposers?
Relatively little is known about miRNA turnover in mammalian systems, including the identity of exonucleases required, as reviewed elsewhere.Citation23-25 Here we focus on the potential role of RNAs in mediating turnover and propose that there are 2 classes of regulator: those that induce degradation of the miRNA and those that do not. Most known targets of miRNAs are considered to be non-destructive but could out-compete other targets depending on concentration, number of binding sites and affinity, according to studies of ceRNAs.Citation26–28 On the other hand, it is not clear what factors make some RNAs destructive. From a theoretical standpoint, there are several modes by which RNAs could mediate degradation of a miRNA: 1) they could specifically recruit proteins to the RNA that are required for turnover, including exonucleases; 2) they could mediate localization of the miRNA to sites associated with turnover or export; or 3) they could induce a conformational change in the miRNA-RISC complex that exposes the miRNA, making it susceptible to modification and degradation by proteins. These modes of regulation are not mutually exclusive, however it is envisioned that specific RNA motifs outside the miRNA binding site would define a “recruiter” or “localizer.” The concept of a recruiter seems logical given the presence of motifs in mRNAs that are associated with mRNA stability and HSUR-1 is degraded by an AU-rich element (ARE)-dependent pathway.Citation29 However, to date, there are no reports pinning these motifs to miRNA degradation. Little is known about the localization of miRNA turnover, however components of RISC have been shown to be associated with multivesicular bodies (MVB), which have been linked to gene silencing and RISC turnover.Citation30,31 Could miRNA localization at membranes be required for turnover and could this be regulated by the RNA to which a miRNA binds? This concept is intriguing based on the precedent that some RNAs can mediate sub-cellular localization, for example localizing to the endoplasmic reticulum independently of translation.Citation32 However there is no evidence to date that RNA-mediated changes in miRNA localization are involved in turnover. An alternative hypothesis is that RNAs regulate the movement of miRNAs outside of cells, for example in vesicles. However a recent publication suggests the opposite may be true as raising the level of the targets of a miRNA can relocate them from the MVB/biogenesis pathway to P bodies.Citation33 There is no strong evidence that any motifs outside the binding site exist in the miRNA regulators in herpesviruses. Lee et al. (2013) suggested that, in HCMV, in addition to the miRNA binding site an additional 20 nt sequence may be important for turnover. However this region is not conserved in related viruses (see below) and the evidence that it is required for turnover is limited. Motifs outside of the miRNA binding site have not been found to be involved in turnover of miR-27 by HSUR-1 or m169. Furthermore comparison of these RNAs reveals little in common: HSUR-1 is a small nuclear RNA (snRNA) of 143 nt whereas m169 is a ∼1.7 kb spliced mRNA transcript that localizes predominantly to the cytoplasm.Citation19
Tailing and trimming of miRNAs: inside or outside of RISC?
The concept of RNA “exposers” is the only supported mode of RNA-based miRNA regulation at present. This model was first described by Ameres et al. who reported the tailing and trimming of miRNAs when incubated with complementary targets in flies and human cell lines.Citation34 Specifically, they observed the addition of non-templated nucleotides to the 3′ end of miRNAs in the presence of targets with complementarity at the 3′ end and demonstrated that this tailing correlated with trimming of the miRNA and a reduction in its abundance.Citation34 Consistent with this Baccarini et al. showed a target-induced decrease in miR-223 abundance in HEK293 cells that was based on accelerated decay of the miRNA and correlated with an increased proportion of non-templated uridines and adenosines at the 3′ end.Citation35 One model to fit these studies is that pairing to the 3′end of a miRNA makes it susceptible to tailing, which is required for, and precedes, degradation. Along these lines Marcinowski et al. reported that during MCMV infection miR-27 had an increase in 3′end modifications as well as an increase in shorter (trimmed) sequences and that the tailed forms were more prevalent in total RNA compared to RNA isolated from Ago2 immuno-precipitation.Citation20 This model would be consistent with structural studies showing that pairing of a target to the 3′end of a DNA guide within bacterial Ago causes exposure of the guide strandCitation36 and pairing of a target at the 3′end of a miRNA increases its off rate from mammalian Ago2.Citation37 However Flores et al. recently examined miRNA content inside and outside of RISC in mammalian cell lines and found that RISC-associated miRNAs were slightly more likely to have 3′end modifications.Citation38 Indeed without understanding the degradation mechanism(s) the relationship between tailing, RISC occupancy and turnover is still unclear. It is rather striking that recent reports suggests a very large proportion of miRNAs within a cell are not in fact associated with any Ago protein.Citation39,40 Despite extensive research in this area we still understand little regarding the organization of miRNAs within a cell and the turnover pathways involved in their regulation.
Evolution of viral regulators of miRNAs
Even in the absence of mechanistic data, the presence of miRNA regulators in viral genomes suggests that individual miRNAs play important roles during infection. Furthermore the studies cited above suggest the anti-miRNA activities of the herpesvirus transcripts confer an advantage on these viruses. However their distribution is puzzling. miR-27 is thought to have originated prior to the last common ancestor of the vertebrates,Citation41 more than half a billion years ago. In contrast, both m169 and HSUR-1 have evolved quite recently. While the m169 gene is present, and the miR-27 binding site is conserved, among all known strains of MCMV, this locus is not found in 2 divergent forms of rat cytomegalovirus (RCMV), the closest known relatives of MCMV. Many mammalian herpesviruses show phylogenetic relationships mirroring those of their hosts, suggesting that their ancestors infected and co-diverged with the ancestors of their hosts.Citation42,43 Thus, the miR-27 binding site in MCMV appears to have arisen since the common ancestor of mouse and rat, about 12 Myr ago.Citation44 HSUR-1 in HVS may be a little older. A homologous site within the same type of RNA element (HAUR-1) is found in a closely related rhadinovirus from spider monkeys, Herpesvirus ateles (HVA),Citation45 but not in other rhadinoviruses infecting Old World monkeys and apes. Squirrel monkeys and spider monkeys are both New world monkeys, and are thought to have shared a common ancestor around 20 Myr ago.Citation46 Thus, the approximate points when these 2 miR-27 degrading activities arose in the ancestry of MCVM and HVS can be mapped onto the herpesvirus phylogeny (). It is clear that ancestral herpesviruses, infecting mammals and their ancestors, may have been exposed to miR-27 for hundreds of millions of years, but the 2 known miR-27 antagonists have only developed much more recently; and, of course, in the β and gamma subfamilies of herpesviruses this has been achieved by 2 completely different routes.
Figure 1. Phylogenetic relationships among representative mammalian herpesviruses, showing the branches on which 3 distinct miRNA regulators appear to have evolved (circles), and 4 occasions where IL10 genes have been acquired (triangles). Ancestral branches leading to the α, β and gamma subfamilies of herpesviruses are indicated. Virus acronyms are explained, and sequence sources are given, in Supplementary Table 1.
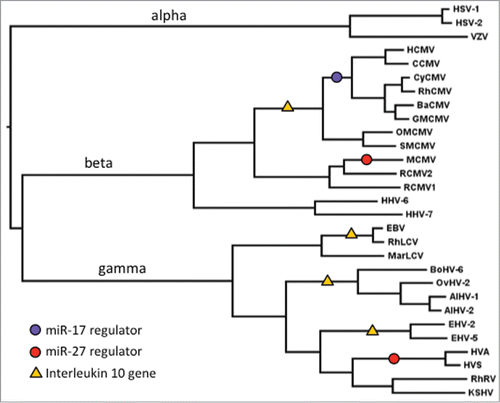
This raises a number of questions. First, has miR-27 always played an anti-viral role? In the case of HVS infection of T cells, Guo et al. identified and validated miR-27 targets that are involved in T cell receptor (TCR) signaling,Citation45 a pathway which is activated in HVS-transformed human T cells.Citation47 While this particular pathway is unlikely to be relevant to MCMV, which does not infect T cells, it was also shown that miR-27 suppressed mitogen-activated protein kinase (MAPK) signaling and effector cytokines (IFN-γ, IL-10) that are part of the cellular response to many viruses including MCMV. Thus, while it seems unlikely that miR-27 evolved specifically as a host defense mechanism, it is possible that suppressing miR-27 could be advantageous to a broad range of viruses, including the ancient ancestors of today's herpesviruses.
A second question, then, is why are miR-27 antagonists not more widespread among herpesviruses? An alternative strategy for coping with miR-27 activity has been suggested. Guo et al. (2014) noted that some gamma-herpesviruses have acquired copies of cellular genes that are targets of miR-27. For example, ovine herpesvirus 2 (OvHV-2) has genes encoding the cellular miR-27 targets interleukin-10 (IL10), ATF3 and SEMA7A, and these are located within the same region upstream of ORF3 where HSUR-1 and HAUR-1 lie in the New World monkey rhadinovirus genomes. Guo et al. (2014) found that the v-IL10 gene is not present in alcelaphine herpesvirus 1 (AlHV-1), a close relative of OvHV-2, but this appears to reflect recent gene loss, since there is a copy in the AlHV-2 genome, and a fragment of the v-IL10 gene appears in the more distantly related bovine herpesvirus 6 (BoHV-6).Citation48 In fact, many different β and gamma herpesviruses have IL10 homologues, resulting from at least 4 independent gene acquisitions of host genes (). The immunosuppressive properties of IL-10 are known to be beneficial to herpesviruses in a range of contextsCitation49 and it is assumed (though this has not been tested) that cellular IL-10 is increased as a result of degradation of miR-27 by the RNA regulators. While numerous herpesviruses have either an IL10 gene or an miR-27 antagonist, none are known to have both (). The large number of independent, evolutionarily recent events leading to the acquisition of either an miR-27 antagonist or an IL10 gene () might reflect a continual process of turnover in the mechanisms by which herpesviruses manipulate their hosts. That is, there may have been a large number of more ancient events, generating anti-miRNA activities for which the evidence has now been lost. The loss of the v-IL10 gene in AlHV-1 is perhaps one example of such a process of turnover and demonstrates that this class of viral gene can indeed fall out of existence.
MiR-27 is not the only miRNA targeted by a virus. As described above, a clinical strain of HCMV was shown to degrade members of the miR-17 family through a non-coding RNA within the region between the UL144 and UL145 genes, described as a “miRNA decay element” (miRDE). Homologues of UL144 and UL145 are found in chimpanzee CMV as well as cytomegaloviruses infecting Old World monkeys. Although the UL144-UL145 intergenic region is extremely divergent among these viruses, the sites complementary to both the 5′ seed and 3′ end of the miR-17 family are perfectly conserved (). The absence of a UL144 homolog, and lack of any obvious miR-17 binding site, in related New World monkey viruses pinpoints the origin of this miRDE (). Assuming co-divergence of primate cytomegaloviruses with their hosts, this element appears to have originated subsequent to the divergence of New and Old World monkeys, but prior to the common ancestor of apes and Old World monkeys, perhaps 25–30 Mya.Citation44 However, miR-17 is thought to be as old as miR-27, predating the diversification of the vertebrates.Citation41 Since different miRNAs can target the same genes and pathways it is possible there is functional overlap between these anti-miR activities in MCMV and HCMV, but this has not been investigated. Given the diversity of miRNA functions inside cells, it is also possible that the anti-miR-17 activity serves a different purpose. Indeed we have probably only scratched the surface in finding miRNA regulators in herpesviruses. Furthermore these could be missed in some studies as the anti-miR-17 activities in HCMV are not present in some lab-adapted strains.
Figure 2. miRNA binding sites in herpesviruses. Sites within viral RNAs that are complementary to miRNAs are shown in red; black lines (or dots for G-U) show sites where potential binding is conserved. Conserved sequences in the miR-17 family members are denoted with a black line. Virus acronyms are explained, and sequence sources are given, in Supplementary Table 1.
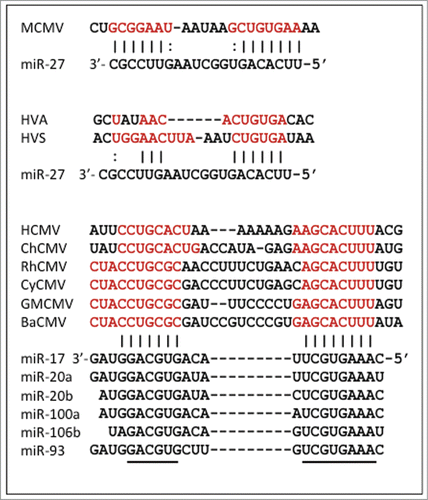
Outlook: beyond herpesvirsues
The miRNA regulators are another example of the versatile mechanisms herpesviruses can use to manipulate their hosts. Do we expect to see more of these viral non-coding RNAs in other herpesviruses, or other viral families? How costly is this mechanism to viruses in relation to the benefit? Clearly the need for miRNA regulators will depend on the diverse requirements of each DNA (or RNA) virus to achieve acute, chronic or latent infection within the host. From what is known regarding m169 and HSUR-1 we expect a miRNA regulator must be highly expressed, presumably at least as abundant as the miRNA it is targeting. Indeed the m169 mRNA is the most abundant transcript in lytic infection.Citation50 We expect that the miRNA regulator does not need to be very big (HSUR-1 is <150 nt) but we do not yet know if the regulator is degraded in parallel with the miRNA.
It seems likely that miRNA regulators would exist in other virus families, particularly where it is known that individual miRNAs impair viral replication or persistance. Although the miRNA regulators have only been found in herpesviruses to date, there is a precedent for RNA viruses to evolve bonafide miRNA binding sites in their genomes.Citation51,52 For example, hepatitis C virus (HCV) directly binds to host miR-122 to stabilize the viral genome, stimulate viral translation within the liver and prevent the induction of innate immune responses.Citation51,53-55. A recent report suggests this interaction may also inhibit the natural targets of miR-122 in the cell, though this is not expected to involve miRNA degradation.Citation56. The majority of RNA viruses cause acute infections and it has been hypothesized that miRNAs lack the ability to regulate the host transcriptome in the time frame of most infectionsCitation57 since miRNA-mediated reductions in protein expression are contingent on protein turnover. Nevertheless, a recent study demonstrates that eastern equine encephalitis virus utilizes a host myeloid specific miRNA binding site in its genome to limit replication and thus innate immune induction in myeloid cells.Citation52 It does not seem far-fetched that miRNA binding sites could also be used for miRNA inhibition and we continue to learn about the kinetics of miRNA regulation and function. Specific miRNAs are rapidly regulated in response to growth factor stimulation, altering targets that are immediate early genes and eliciting rapid physiological changes in the cell.Citation58 They are also dynamically regulated during the cell cycleCitation59 and in neurons, reviewed inCitation24. Additionally non-canonical functions of miRNAs have been reported that might require rapid miRNA regulation, including direct stimulation of toll like receptorsCitation60 and sequence-dependent sequestration of RNA binding proteins.Citation61 A recent paper suggests that miRNAs, when not bound to Argonaute proteins, can interact with the HIV-1 Gag protein and disrupt viral particle production, blocking budding at the plasma membrane.Citation62 As our knowledge of the function of miRNAs continues to expand it is worth keeping an open mind to the wide range of possibilities for how and why viruses can interact with, and seek to inhibit, individual members of this class of small RNA.
Disclosure of Potential Conflicts of Interest
No potential conflicts of interest were disclosed.
Authors' Contributions
AB drafted the manuscript, PS contributed evolutionary analyses and all authors contributed ideas and text.
Supplemental_Table.xlsx
Download MS Excel (11.3 KB)Acknowledgments
We thank F.Gray and D.Obbard for critical reading of the manuscript and helpful discussions.
Funding
Research in Buck's lab is supported by Wellcome Trust RCDF (WT097394A1A) and BBSRC (BB/J001279). The authors were also supported by funding from the Wellcome Trust funded Centre for Immunity, Infection and Evolution (095831/Z/11/Z).
Supplemental Material
Supplemental data for this article can be accessed on the publisher's website.
References
- Guo YE, Steitz JA. Virus meets host microRNA: the destroyer, the booster, the hijacker. Mol Cell Biol 2014; 34:3780-7; PMID:25047834; http://dx.doi.org/10.1128/MCB.00871-14
- Kim VN, Han J, Siomi MC. Biogenesis of small RNAs in animals. Nat Rev Mol Cell Biol 2009; 10:126-39; PMID:19165215; http://dx.doi.org/10.1038/nrm2632
- Fabian MR, Sonenberg N. The mechanics of miRNA-mediated gene silencing: a look under the hood of miRISC. Nat Struct Mol Biol 2012; 19:586-93; PMID:22664986; http://dx.doi.org/10.1038/nsmb.2296
- Bartel DP. MicroRNAs: target recognition and regulatory functions. Cell 2009; 136:215-33; PMID:19167326; http://dx.doi.org/10.1016/j.cell.2009.01.002
- Friedman RC, Farh KK, Burge CB, Bartel DP. Most mammalian mRNAs are conserved targets of microRNAs. Genome Res 2009; 19:92-105; PMID:18955434; http://dx.doi.org/10.1101/gr.082701.108
- Grundhoff A, Sullivan CS. Virus-encoded microRNAs. Virology 2011; 411:325-43; PMID:21277611; http://dx.doi.org/10.1016/j.virol.2011.01.002
- Skalsky RL, Cullen BR. Viruses, microRNAs, and host interactions. Annu Rev Microbiol 2010; 64:123-41; PMID:20477536; http://dx.doi.org/10.1146/annurev.micro.112408.134243
- Cullen BR. Viral and cellular messenger RNA targets of viral microRNAs. Nature 2009; 457:421-5; PMID:19158788; http://dx.doi.org/10.1038/nature07757
- Franco-Zorrilla JM, Valli A, Todesco M, Mateos I, Puga MI, Rubio-Somoza I, Leyva A, Weigel D, García JA, Paz-Ares J. Target mimicry provides a new mechanism for regulation of microRNA activity. Nat Genet 2007; 39:1033-7; PMID:17643101; http://dx.doi.org/10.1038/ng2079
- Figueroa-Bossi N, Valentini M, Malleret L, Fiorini F, Bossi L. Caught at its own game: regulatory small RNA inactivated by an inducible transcript mimicking its target. Genes Dev 2009; 23:2004-15; PMID:19638370; http://dx.doi.org/10.1101/gad.541609
- Overgaard M, Johansen J, Moller-Jensen J, Valentin-Hansen P. Switching off small RNA regulation with trap-mRNA. Mol Microbiol 2009; 73:790-800; PMID:19682266; http://dx.doi.org/10.1111/j.1365-2958.2009.06807.x
- Seitz H. Redefining microRNA targets. Curr Biol 2009; 19:870-3; PMID:19375315; http://dx.doi.org/10.1016/j.cub.2009.03.059
- Tay Y, Rinn J, Pandolfi PP. The multilayered complexity of ceRNA crosstalk and competition. Nature 2014; 505:344-52; PMID:24429633; http://dx.doi.org/10.1038/nature12986
- Denzler R, Agarwal V, Stefano J, Bartel DP, Stoffel M. Assessing the ceRNA hypothesis with quantitative measurements of miRNA and target abundance. Mol Cell 2014; 54:766-76; PMID:24793693; http://dx.doi.org/10.1016/j.molcel.2014.03.045
- Broderick JA, Zamore PD. Competitive endogenous RNAs cannot alter microRNA function in vivo. Mol Cell 2014; 54:711-3; PMID:24905003; http://dx.doi.org/10.1016/j.molcel.2014.05.023
- Ebert MS, Neilson JR, Sharp PA. MicroRNA sponges: competitive inhibitors of small RNAs in mammalian cells. Nat Methods 2007; 4:721-6; PMID:17694064; http://dx.doi.org/10.1038/nmeth1079
- Buck AH, Perot J, Chisholm MA, Kumar DS, Tuddenham L, Cognat V, Marcinowski L, Dölken L, Pfeffer S. Post-transcriptional regulation of miR-27 in murine cytomegalovirus infection. RNA 2010; 16:307-15; PMID:20047990; http://dx.doi.org/10.1261/rna.1819210
- Bail S, Swerdel M, Liu H, Jiao X, Goff LA, Hart RP, Kiledjian M. Differential regulation of microRNA stability. Rna 2010; 16:1032-9; PMID:20348442; http://dx.doi.org/10.1261/rna.1851510
- Libri V, Helwak A, Miesen P, Santhakumar D, Borger JG, Kudla G, Grey F, Tollervey D, Buck AH. Murine cytomegalovirus encodes a miR-27 inhibitor disguised as a target. Proc Natl Acad Sci U S A 2012; 109:279-84; PMID:22184245; http://dx.doi.org/10.1073/pnas.1114204109
- Marcinowski L, Tanguy M, Krmpotic A, Radle B, Lisnic VJ, Tuddenham L, Chane-Woon-Ming B, Ruzsics Z, Erhard F, Benkartek C, et al. Degradation of cellular miR-27 by a novel, highly abundant viral transcript is important for efficient virus replication in vivo. PLoS Pathog 2012; 8:e1002510; PMID:22346748
- Cazalla D, Yario T, Steitz JA. Down-regulation of a host microRNA by a Herpesvirus saimiri noncoding RNA. Science 2010; 328:1563-6; PMID:20558719; http://dx.doi.org/10.1126/science.1187197
- Lee S, Song J, Kim S, Kim J, Hong Y, Kim Y, Kim D, Baek D, Ahn K. Selective degradation of host MicroRNAs by an intergenic HCMV noncoding RNA accelerates virus production. Cell Host Microbe 2013; 13:678-90; PMID:23768492; http://dx.doi.org/10.1016/j.chom.2013.05.007
- Zhang Z, Qin YW, Brewer G, Jing Q. MicroRNA degradation and turnover: regulating the regulators. Wiley Interdiscip Rev RNA 2012; 3:593-600; PMID:22461385; http://dx.doi.org/10.1002/wrna.1114
- Ruegger S, Grosshans H. MicroRNA turnover: when, how, and why. Trends Biochem Sci 2012; 37:436-46; PMID:22921610; http://dx.doi.org/10.1016/j.tibs.2012.07.002
- Kai ZS, Pasquinelli AE. MicroRNA assassins: factors that regulate the disappearance of miRNAs. Nat Struct Mol Biol 2010; 17:5-10; PMID:20051982; http://dx.doi.org/10.1038/nsmb.1762
- Arvey A, Larsson E, Sander C, Leslie CS, Marks DS. Target mRNA abundance dilutes microRNA and siRNA activity. Mol Syst Biol 2010; 6:363; PMID:20404830; http://dx.doi.org/10.1038/msb.2010.24
- Tay Y, Kats L, Salmena L, Weiss D, Tan SM, Ala U, Karreth F, Poliseno L, Provero P, Di Cunto F, et al. Coding-independent regulation of the tumor suppressor PTEN by competing endogenous mRNAs. Cell 2011; 147:344-57; PMID:22000013; http://dx.doi.org/10.1016/j.cell.2011.09.029
- Sumazin P, Yang X, Chiu HS, Chung WJ, Iyer A, Llobet-Navas D, Rajbhandari P, Bansal M, Guarnieri P, Silva J, et al. An extensive microRNA-mediated network of RNA-RNA interactions regulates established oncogenic pathways in glioblastoma. Cell 2011; 147:370-81; PMID:22000015; http://dx.doi.org/10.1016/j.cell.2011.09.041
- Cook HL, Mischo HE, Steitz JA. The Herpesvirus saimiri small nuclear RNAs recruit AU-rich element-binding proteins but do not alter host AU-rich element-containing mRNA levels in virally transformed T cells. Mol Cell Biol 2004; 24:4522-33; PMID:15121869; http://dx.doi.org/10.1128/MCB.24.10.4522-4533.2004
- Lee YS, Pressman S, Andress AP, Kim K, White JL, Cassidy JJ, Li X, Lubell K, Lim do H, Cho IS, et al. Silencing by small RNAs is linked to endosomal trafficking. Nat Cell Biol 2009; 11:1150-6; PMID:19684574; http://dx.doi.org/10.1038/ncb1930
- Gibbings DJ, Ciaudo C, Erhardt M, Voinnet O. Multivesicular bodies associate with components of miRNA effector complexes and modulate miRNA activity. Nat Cell Biol 2009; 11:1143-9; PMID:19684575; http://dx.doi.org/10.1038/ncb1929
- Pyhtila B, Zheng T, Lager PJ, Keene JD, Reedy MC, Nicchitta CV. Signal sequence- and translation-independent mRNA localization to the endoplasmic reticulum. Rna 2008; 14:445-53; PMID:18192611; http://dx.doi.org/10.1261/rna.721108
- Squadrito ML, Baer C, Burdet F, Maderna C, Gilfillan GD, Lyle R, Ibberson M, De Palma M. Endogenous RNAs modulate microRNA sorting to exosomes and transfer to acceptor cells. Cell Rep 2014; 8:1432-46; PMID:25159140; http://dx.doi.org/10.1016/j.celrep.2014.07.035
- Ameres SL, Horwich MD, Hung JH, Xu J, Ghildiyal M, Weng Z, Zamore PD. Target RNA-directed trimming and tailing of small silencing RNAs. Science 2010; 328:1534-9; PMID:20558712; http://dx.doi.org/10.1126/science.1187058
- Baccarini A, Chauhan H, Gardner TJ, Jayaprakash AD, Sachidanandam R, Brown BD. Kinetic analysis reveals the fate of a microRNA following target regulation in mammalian cells. Curr Biol 2011; 21:369-76; PMID:21353554; http://dx.doi.org/10.1016/j.cub.2011.01.067
- Wang YL, Juranek S, Li HT, Sheng G, Wardle GS, Tuschl T, Patel DJ. Nucleation, propagation and cleavage of target RNAs in Ago silencing complexes. Nature 2009; 461:754-U3; PMID:19812667; http://dx.doi.org/10.1038/nature08434
- De N, Young L, Lau PW, Meisner NC, Morrissey DV, MacRae IJ. Highly complementary target RNAs promote release of guide RNAs from human Argonaute2. Mol Cell 2013; 50:344-55; PMID:23664376; http://dx.doi.org/10.1016/j.molcel.2013.04.001
- Flores O, Kennedy EM, Skalsky RL, Cullen BR. Differential RISC association of endogenous human microRNAs predicts their inhibitory potential. Nucleic Acids Res 2014; 42:4629-39; PMID:24464996; http://dx.doi.org/10.1093/nar/gkt1393
- Stalder L, Heusermann W, Sokol L, Trojer D, Wirz J, Hean J, Fritzsche A, Aeschimann F, Pfanzagl V, Basselet P, et al. The rough endoplasmatic reticulum is a central nucleation site of siRNA-mediated RNA silencing. Embo J 2013; 32:1115-27; PMID:23511973; http://dx.doi.org/10.1038/emboj.2013.52
- Janas MM, Wang B, Harris AS, Aguiar M, Shaffer JM, Subrahmanyam YV, Behlke MA, Wucherpfennig KW, Gygi SP, Gagnon E, et al. Alternative RISC assembly: binding and repression of microRNA-mRNA duplexes by human Ago proteins. Rna 2012; 18:2041-55; PMID:23019594; http://dx.doi.org/10.1261/rna.035675.112
- Heimberg AM, Cowper-Sal-lari R, Semon M, Donoghue PC, Peterson KJ. microRNAs reveal the interrelationships of hagfish, lampreys, and gnathostomes and the nature of the ancestral vertebrate. Proc Natl Acad Sci U S A 2010; 107:19379-83; PMID:20959416; http://dx.doi.org/10.1073/pnas.1010350107
- Sharp PM. Origins of human virus diversity. Cell 2002; 108:305-12; PMID:11853665; http://dx.doi.org/10.1016/S0092-8674(02)00639-6
- McGeoch DJ, Dolan A, Ralph AC. Toward a comprehensive phylogeny for mammalian and avian herpesviruses. J Virol 2000; 74:10401-6; PMID:11044084; http://dx.doi.org/10.1128/JVI.74.22.10401-10406.2000
- Benton MJ, Donoghue PC. Paleontological evidence to date the tree of life. Mol Biol Evol 2007; 24:26-53; PMID:17047029; http://dx.doi.org/10.1093/molbev/msl150
- Guo YE, Riley KJ, Iwasaki A, Steitz JA. Alternative capture of noncoding RNAs or protein-coding genes by herpesviruses to alter host T cell function. Mol Cell 2014; 54:67-79; PMID:24725595; http://dx.doi.org/10.1016/j.molcel.2014.03.025
- Sallam HM, Seiffert ER, Steiper ME, Simons EL. Fossil and molecular evidence constrain scenarios for the early evolutionary and biogeographic history of hystricognathous rodents. Proc Natl Acad Sci U S A 2009; 106:16722-7; PMID:19805363; http://dx.doi.org/10.1073/pnas.0908702106
- Noraz N, Saha K, Ottones F, Smith S, Taylor N. Constitutive activation of TCR signaling molecules in IL-2-independent Herpesvirus saimiri-transformed T cells. J Immunol 1998; 160:2042-5; PMID:9498738
- Jia J, Delhon G, Tulman ER, Diel DG, Osorio FA, Wen X, Kutish GF, Rock DL. Novel gammaherpesvirus functions encoded by bovine herpesvirus 6 (bovine lymphotropic virus). J Gen Virol 2014; 95:1790-8; PMID:24836671; http://dx.doi.org/10.1099/vir.0.066951-0
- Ouyang P, Rakus K, van Beurden SJ, Westphal AH, Davison AJ, Gatherer D, Vanderplasschen AF. IL-10 encoded by viruses: a remarkable example of independent acquisition of a cellular gene by viruses and its subsequent evolution in the viral genome. J Gen Virol 2014; 95:245-62; PMID:24225498; http://dx.doi.org/10.1099/vir.0.058966-0
- Juranic Lisnic V, Babic Cac M, Lisnic B, Trsan T, Mefferd A, Das Mukhopadhyay C, Cook CH, Jonjic S, Trgovcich J. Dual analysis of the murine cytomegalovirus and host cell transcriptomes reveal new aspects of the virus-host cell interface. PLoS Pathog 2013; 9:e1003611; PMID:24086132
- Jopling CL, Yi M, Lancaster AM, Lemon SM, Sarnow P. Modulation of hepatitis C virus RNA abundance by a liver-specific microRNA. Science 2005; 309:1577-81; PMID:16141076; http://dx.doi.org/10.1126/science.1113329
- Trobaugh DW, Gardner CL, Sun C, Haddow AD, Wang E, Chapnik E, Mildner A, Weaver SC, Ryman KD, Klimstra WB. RNA viruses can hijack vertebrate microRNAs to suppress innate immunity. Nature 2014; 506:245-8; PMID:24352241; http://dx.doi.org/10.1038/nature12869
- Lanford RE, Hildebrandt-Eriksen ES, Petri A, Persson R, Lindow M, Munk ME, Kauppinen S, Ørum H. Therapeutic silencing of microRNA-122 in primates with chronic hepatitis C virus infection. Science 2010; 327:198-201; PMID:19965718; http://dx.doi.org/10.1126/science.1178178
- Machlin ES, Sarnow P, Sagan SM. Masking the 5′ terminal nucleotides of the hepatitis C virus genome by an unconventional microRNA-target RNA complex. Proc Natl Acad Sci U S A 2011; 108:3193-8; PMID:21220300; http://dx.doi.org/10.1073/pnas.1012464108
- Jopling CL, Schutz S, Sarnow P. Position-dependent function for a tandem microRNA miR-122-binding site located in the hepatitis C virus RNA genome. Cell Host Microbe 2008; 4:77-85; PMID:18621012; http://dx.doi.org/10.1016/j.chom.2008.05.013
- Luna JM, Scheel TK, Danino T, Shaw KS, Mele A, Fak JJ, Nishiuchi E, Takacs CN, Catanese MT, de Jong YP, et al. Hepatitis C virus RNA functionally sequesters miR-122. Cell 2015; 160:1099-110; PMID:25768906; http://dx.doi.org/10.1016/j.cell.2015.02.025
- tenOever BR. RNA viruses and the host microRNA machinery. Nat Rev Microbiol 2013; 11:169-80; PMID:23411862; http://dx.doi.org/10.1038/nrmicro2971
- Avraham R, Sas-Chen A, Manor O, Steinfeld I, Shalgi R, Tarcic G, Bossel N, Zeisel A, Amit I, Zwang Y, et al. EGF decreases the abundance of microRNAs that restrain oncogenic transcription factors. Sci Signal 2010; 3:ra43; PMID:20516477
- Rissland OS, Hong SJ, Bartel DP. MicroRNA destabilization enables dynamic regulation of the miR-16 family in response to cell-cycle changes. Mol Cell 2011; 43:993-1004; PMID:21925387; http://dx.doi.org/10.1016/j.molcel.2011.08.021
- Fabbri M, Paone A, Calore F, Galli R, Gaudio E, Santhanam R, Lovat F, Fadda P, Mao C, Nuovo GJ, et al. MicroRNAs bind to Toll-like receptors to induce prometastatic inflammatory response. Proc Natl Acad Sci U S A 2012; 109:E2110-6; PMID:22753494; http://dx.doi.org/10.1073/pnas.1209414109
- Eiring AM, Harb JG, Neviani P, Garton C, Oaks JJ, Spizzo R, Liu S, Schwind S, Santhanam R, Hickey CJ, et al. miR-328 functions as an RNA decoy to modulate hnRNP E2 regulation of mRNA translation in leukemic blasts. Cell 2010; 140:652-65; PMID:20211135; http://dx.doi.org/10.1016/j.cell.2010.01.007
- Chen AK, Sengupta P, Waki K, Van Engelenburg SB, Ochiya T, Ablan SD, Freed EO, Lippincott-Schwartz J. MicroRNA binding to the HIV-1 Gag protein inhibits Gag assembly and virus production. Proc Natl Acad Sci U S A 2014; 111:E2676-83; PMID:24938790; http://dx.doi.org/10.1073/pnas.1408037111