Abstract
Translation of mRNAs is the primary function of the ribosomal machinery. Although cells allow for a certain level of translational errors/mistranslation (which may well be a strategic need), maintenance of the fidelity of translation is vital for the cellular function and fitness. The P-site bound initiator tRNA selects the start codon in an mRNA and specifies the reading frame. A direct P-site binding of the initiator tRNA is a function of its special structural features, ribosomal elements, and the initiation factors. A highly conserved feature of the 3 consecutive G:C base pairs (3GC pairs) in the anticodon stem of the initiator tRNAs is vital in directing it to the P-site. Mutations in the 3GC pairs diminish/abolish initiation under normal physiological conditions. Using molecular genetics approaches, we have identified conditions that allow initiation with the mutant tRNAs in Escherichia coli. During our studies, we have uncovered a novel phenomenon of in vivo initiation by elongator tRNAs. Here, we recapitulate how the cellular abundance of the initiator tRNA, and nucleoside modifications in rRNA are connected with the tRNA selection in the P-site. We then discuss our recent finding of how a conserved feature in the mRNA, the Shine-Dalgarno sequence, influences tRNA selection in the P-site.
Introduction
Translation of an mRNA is a highly regulated process. It is the cellular mechanism to generate proteome diversity without affecting the basic repertoire of the genetic information. Prokaryotes devote more than 30% of their dry mass and ˜95% of the total RNA for the translation machinery itself to ensure accurate and efficient translation.Citation1,2 Translation of mRNA occurs in the steps of initiation, elongation and termination followed by ribosome recycling for another round of initiation. Initiation is thought to be the most rate-limiting step in translation.Citation3 In eubacteria, the canonical pathway of initiation begins with the formation of a 30S pre-initiation complex (PIC) involving the small subunit of the ribosome (30S), mRNA, initiation factors (IF1, IF2 and IF3) and the initiator tRNA (tRNAfMet). The tRNAfMet plays an important role in selecting the start codon. Any failures at this step could lead to mRNA translation in incorrect reading frames and production of mis-translated peptides. Docking of the large subunit of the ribosome (50S) converts the 30S PIC into the 70S initiation complex (IC). In both complexes, tRNAfMet is present in a tilted conformation between the P/E and P/P state, the P/I state.Citation4,5 Upon GTP hydrolysis (mediated by IF2), the ribosome undergoes transitions, which locate tRNAfMet in the P/P state and result in release of the initiation factors (). At this point the 70S complex is ready to accept elongator tRNAs in its A-site.Citation5 The selection of tRNAfMet in the P-site is assisted by the ribosome bound initiation factors, the elements in the P-site and the distinct structural features in tRNAfMet.Citation6-17 We have been interested in understanding the mechanism of initiation from a ‘tRNAfMet-centric' view using an in vivo assay system we developed nearly 3 decades ago.Citation18 In this short article, we summarize our findings toward understanding the mechanism of initiation in E. coli. To lead up to these findings, we first discuss the salient structural features of the initiator tRNA and the in vivo assay system central to our studies.
Figure 1. Translation initiation in eubacteria. The formation of 30S pre-initiation complex (PIC) involves binding of all the 3 initiation factors IF1, IF2 and IF3 along with tRNAfMet and mRNA. The tRNAfMet in this stage is slightly tilted away from the P-site, called P/I state. This complex recruits 50S subunit with the help of IF2 to form 70S IC with the tRNAfMet in P/I state. This IC undergoes a rotational movement upon GTP hydrolysis to give rise to a 70S complex with the tRNAfMet in P/P state. This rotation also leads to release of initiation factors from the 70S to convert into an elongation competent 70S.
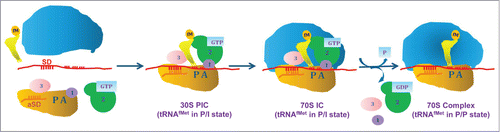
Initiator tRNA: Structural Features and Cross Talk with the Ribosomal Elements
The prokaryotic tRNAfMet are characterized by at least 3 special features (). Firstly, they possess a non-Watson-Crick pair (C1 × A72 in E. coli) at the top of the acceptor stem. This feature is an important determinant for, (a) formylation of the amino acid attached to tRNAfMet, (b) avoidance of binding of the aminoacylated tRNAfMet to EF-Tu, and (c) prevention of hydrolysis of the formyl-aminoacyl-tRNAfMet by peptidyl-tRNA hydrolase (Pth), an essential enzyme which recycles tRNAs from the peptidyl-tRNAs that fall off the translating ribosomes.Citation17,19,20 Secondly, at position 11:24 (in DHU stem), tRNAfMet possesses a purine:pyrimidine pair in contrast to the other tRNAs, which possess a pyrimidine:purine pair at this position. A known function of this feature is to contribute to the formylation efficiency of the tRNA.Citation19 Thirdly, a feature that is highly conserved in initiator tRNAs from all domains of life, is the presence of 3 consecutive G:C base pairs (GC/GC/GC or 3GC pairs) in the anticodon stem at positions 29:41, 30:40 and 31:39. The primary function of this feature is to facilitate preferential binding of the initiator tRNA in the ribosomal P-site.Citation21-23
Figure 2. Cloverleaf structure of E. coli tRNAfMet2 (metY). Sequences important for its special properties are shown in colored box. CxA mismatch at 1×72 position (shown in orange color) together with the base pairs at 2:71, 3:70 is a major determinant for formylation of aminoacid attached to tRNAfMet. The 11:24 position (shown in yellow) also affects the efficiency of formylation. The 3 consecutive GC base pairs in anticodon stem (in green box) are important for the ribosomal P- site targeting of the initiator tRNA.
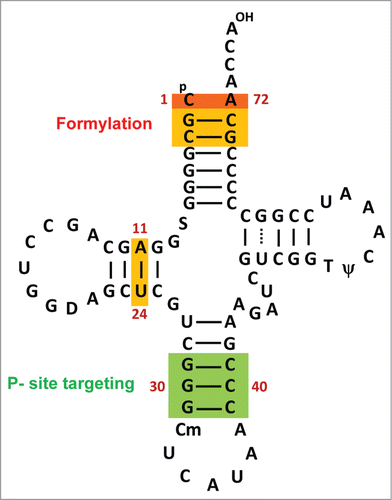
Of the 3 distinctive features in tRNAfMet, 2 i. e., the presence of a non-Watson-Crick pair at the top of the acceptor stem and the 3GC pairs in the anticodon stem are the most crucial. When incorporated into an elongator tRNA, they allow it to function as an initiator.Citation24 The property of formylation of tRNAfMet increases its affinity to IF2.Citation22,25 However, unlike formylation which occurs only in eubacteria, 3GC pairs are almost universally conserved in the initiator tRNAs emphasizing their functional significance.Citation21,26 Mutational analyses have shown that of the 3GC pairs, the middle GC pair is the most critical one. Interestingly, even though tRNAfMet retaining just the middle GC pair (and mimicking the unusual tRNAfMet from some of the mycoplasmas or rhizobiaCitation27) functions in initiation, the naturally occurring elongator tRNAs with even 2 GC base pairs (including the middle GC pair) do not. Thus, it appears that the context of the 3GC pairs vis a vis rest of the tRNA may also be important. However, it should also be said that at least in yeast, the requirement of the 3GC pairs in the initiator tRNA seems more flexible as changing the middle GC pair to CG pair could still sustain its growth.Citation28 These observations suggest that the precise mechanistic role of the 3GC pairs in initiation is still an open question. How the 3GC pairs help tRNAfMet in its function of P-site binding/initiation has been the focus of our studies.
The ribosome bound initiation factors,Citation6-8 the elements in the P-site such as the 16S rRNA nucleotides G1338 and A1339 (known to interact with the 3GC pairs)Citation29,30; methylated nucleotides m2G966 and m5C967Citation31,32 and the tails of the ribosomal proteins S9 and S13Citation31,33,34 etc. are also known to contribute to tRNAfMet selection in the P-site. Besides, there is evidence that methylations at other positions in 16S rRNA play a role in tRNAfMet selection.Citation35-37 The possibility that the rRNA methylations impact the affinity between the 50S and 30S subunitsCitation38 needs further investigation. It has been suggested that a rapid rate of 50S subunit docking onto the 30S PIC may adversely impact the fidelity of tRNAfMet selection in the initiation complex.Citation7,8
In vivo Assay System, Mutations in the 3GC Base Pairs in tRNAfMet and Isolation of Suppressors
Our in vivo assay is a plasmid-based system, which employs chloramphenicol (Cm) acetyltransferase (CAT) reporter where the AUG start codon has been mutated to an amber codon (UAG). This reporter (CATam1) mRNA fails to confer Cm resistance to E. coli because the cellular tRNAfMet (with CAU anticodon) does not initiate from the ‘UAG' start codon (). However, when the cells are supplied with the tRNAfMet whose anticodon is mutated to CUA (from CAU), initiation from the UAG start codon produces CAT protein conferring CmR to the cell.Citation18,22,39 The tRNAfMet with CUA anticodon is aminoacylated with Gln by GlnRS.Citation18 When the 3GC pairs in the anticodon stem of the tRNAfMet are mutated to those found in the elongator tRNAMet (UA/CG/AΨ, called 3GC mutant tRNAfMet), the mutant tRNA loses its competence to initiate even though it is efficiently aminoacylated and formylated, rendering the host Cm sensitive (CmS). We used this phenotype of CmS of the host in a suppressor analysis to characterize mutations in the E. coli chromosome [introduced by N-methyl-N'-nitro-N-nitrosoguanidine, (MNNG) treatment] that conferred CmR to the host by enabling the 3GC mutant tRNAfMet to initiate from the CATam1 reporter.Citation36 Identification of the chromosomal mutations in the suppressor strains () discussed below resulted in characterization of mechanisms that contribute to tRNAfMet selection in the P-site, and in the fidelity of initiation.
Figure 3. In vivo assay system to investigate the role of 3GC pairs in tRNAfMet. The plasmid based assay system employs CATam1 reporter with amber start codon together with a tRNAfMet containing CUA anticodon (derived from metYCUA gene). The metYCUA encoded tRNA is aminoacylated with Gln and then formylated by 10-formyltetrahydrofolate:L-methionyl-tRNAfMetN-formyltransferase (Fmt)Citation18 to produce fGln-tRNAfMet,CUA. The UAG start codon of CATam1 is recognized by metYCUA encoded tRNA to produce CAT protein, which confers chloramphenicol resistance (CmR) to the cell (A). When the 3GC base pairs in the metYCUA encoded tRNA are changed to UA/CG/AΨ pairs (3GC mutation), the mutant tRNA does not function in initiation (even though it is efficiently aminoacylated and formylated) rendering the cell CmS (B). Extragenic mutations that rescue the initiation defect of the 3GC mutant tRNA allow initiation to occur from the UAG start codon of CATam1 mRNA to produce CT and confer CmR (C).
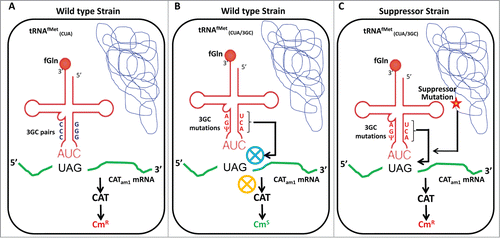
Contribution of Cellular Initiator tRNA Levels
In E. coli, tRNAfMet is encoded by 2 loci, metZWV at 63.5 min and metY at 71.5 min. The metZWV locus possesses 3 genes in an operon encoding tRNAfMet1 and the metY locus possesses a single tRNAfMet gene (as a first gene of the metY-nusA-infB operon) encoding tRNAfMet2.Citation40 In E. coli B strains, both the loci encode identical tRNAfMet.Citation41 However, in E. coli K strains, the metY encoded tRNAfMet differs in that it possesses an A (instead of m7G) at position 46Citation41 with no apparent functional differences. The metZWV encoded tRNAfMet contributes to about 75% of the total tRNAfMet in E. coli. In an earlier work, one class of the suppressor strains which fostered initiation with the 3GC mutant tRNAfMet, we observed mutations in the metZWV promoter which led to a severe down-regulation of the chromosomally encoded tRNAfMet.Citation42 Further, deletion of the metZWV locus also allowed initiation with the 3GC mutant tRNAfMet suggesting that 3GC pairs offer a competitive advantage to tRNAfMet to bind the P-site. In the ΔmetZWV strain we observed that not only the unusual tRNAfMet of the mycoplasma and rhizobia origin (lacking either the first, the third or both the first and the third GC pairs) but also the elongator tRNAs initiated protein synthesis.Citation42,43 In fact, initiation by the unusual tRNAfMet sustained growth of E. coli in the absence of all 4 of the tRNAfMet genes.Citation27 Normally these unusual tRNAs fail to initiate in E. coli to any significant level. Thus, although a higher number of initiator tRNA genes has been attributed to overcome the rate limiting step of initiation, our studies suggest that higher cellular abundance of tRNAfMet makes a definite contribution to the fidelity of initiation by preventing initiation with elongator tRNAs. The relative levels of tRNAfMet and elongator tRNAs and their aminoacylated forms are known to vary under stress.Citation44-47 At least in E. coli, ppGpp as well as cAMP-CAP regulate transcription of tRNAfMet genes.Citation48,44 Thus, it is possible that under the stressful conditions, initiation (from noncanonical positions) by elongator tRNAs generates novel polypeptides by translation in alternate or the original reading frames. Physiological relevance of any such novel polypeptides is unknown. However, the potential to initiate with elongator tRNAs may serve as a cellular strategy to generate proteome diversity which in turn may rescue cells from stress. Interesting as the observation of in vivo initiation with elongator tRNAs is, it raises several questions. How are these tRNAs recruited to the ribosome? Also, as initiation with elongator tRNAs is competed out by tRNAfMet, the elongators must initiate from the P-site. If so, is the relative affinity of tRNAs for EF-Tu and IF2 somehow influenced? Could an elongator tRNA (in its EF-Tu bound form) reposition itself into the P-site for initiation? Given that E. coli can grow in the absence of formylationCitation49 and that an EF-Tu bound aminoacyl-tRNA may be re-sampledCitation50 by other proteins in cell, answers to these questions may not be improbable.
Contribution of Shine-Dalgarno (SD): anti-SD (aSD) Interaction
Recently, we observed that in yet another suppressor strain, a mutation (C1536T) in the aSD sequence of a 16S rRNA gene which resulted in an extended pairing of 8 (from the pre-existing 6) base pairs with the SD sequence of the CATam1 mRNA enabled initiation with the 3GC mutant tRNAfMet.Citation51 Similarly, a mutation in the SD sequence of the CATam1 reporter mRNA resulting in its extended interaction of 8 base pairs in the same region of aSD of the wild-type 16S rRNA also resulted in initiation with the 3GC mutant tRNAfMet. While the exact mechanism remains to be investigated, we observed that the extended SD:aSD interaction resulted in an increased occupancy of the 3GC mutant tRNAfMet onto the elongation competent 70S ribosomes suggesting that at least one of the roles of the 3GC pairs in the native tRNAfMet is to facilitate its transition from 30S PIC to the 70S complex. The 3GC pairs may be ‘licensing' the tRNAfMet to pass through the multistep process of the scrutiny by initiation factors (e. g. IF3Citation6,7,29) and/or the conformational changes the ribosome undergoes (e. g. upon GTP hydrolysisCitation5) to yield an elongation competent 70S (). A systematic mutational analysis of the 3GC base pairs in tRNAfMet might offer further insights into these processes. For example, in our earlier studies, we showed that overproduction of the 3GC mutant tRNAfMet could also lead to a minor increase in initiation, an observation consistent with the role of 3GC base pairs in the direct binding of the tRNAfMet to the 30S ribosome.Citation42,43 It is also known that an extended SD stabilizes the 70S complex.Citation52,53 Consistent with this, we observed that kasugamycin treatment (which is known to allow 70S mode of initiation in leaderless mRNAsCitation54–56) offered a selective advantage to initiation with the 3GC mutant tRNAfMet from the CATam1 mRNA with the extended SD sequence. Other conditions such as increased IF2 levels or decreased RRF activity or decreased IF3 activity (conditions that increase 70S population in cell) also facilitate initiation with the 3GC mutant tRNAfMet. These observations suggest that the requirement of the 3GC pairs is more acute for the canonical mode of initiation from 30S ribosome. The wild-type tRNAfMet is known to facilitate recruitment of a leaderless mRNA for translation in the 70S mode of initiation.Citation57 Conversely, could a stably bound mRNA in 70S ribosome facilitate P-site recruitment of a tRNA via codon:anticodon interaction?
G1338 and A1339, 2 of the highly conserved residues of 16S rRNA, have been shown to form type II and type I A-minor interactions with the first and the middle GC base pairs of the 3 GC pairs, respectively.Citation33 However, as the absence of any of the GC pairs affects the efficiency of initiation, it may well be that during the various stages (transitions) of the initiation process, G1338/A1339 make dynamic interactions with all the 3 of the GC base pairs to offer discrimination between tRNAfMet and the elongator tRNAs.Citation29,58,59 Thus, any displacements in the locations of G1338 and A1339 may impact the selection/retention of a tRNA in the P-site. In this context, it has been suggestedCitation60 that the h26 and h28 in 30S move apart by ∼2Å upon interaction between SD and aSD sequences resulting in a displacement of G1338 and A1339 by ˜13 Å compared with their location in the empty ribosomes (without mRNA or tRNA). In the absence of availability of ribosome structures that directly address these changes, it is unclear how such differences might impact the scrutiny of the 3GC pairs. However, among the 3 initiation factors, IF3 plays an important role in selection of 3GC pairsCitation6,61 through G1338 and A1339.Citation29 A displacement of these residues due to an extended SD:aSD interaction might attenuate the IF3 mediated scrutiny of the 3GC pairs, and allow initiation with 3GC mutant tRNAs. At least, from the perspective of the genetic analyses, an extended SD:aSD interaction seems to compensate for the conformational changes that might be brought about by the interaction of the G1338 and A1339 with the 3GC pairs of the tRNAfMet to allow initiation to proceed even in the absence of the 3GC pairs.
Outlook
Using 3GC mutant tRNAfMet in our genetic analyses, we have explored cellular mechanisms that contribute to the maintenance of fidelity of translation initiation, especially in the selection of tRNAfMet in the P-site. Interestingly, these studies have also uncovered cellular strategies that generate heterogeneity in the ribosomal poolCitation36,38 (for example, a combinatorial effect of lesser than quantitative modifications of the known nucleoside positions would generate an enormous diversity/heterogeneity of arrangements of rRNA modifications available in the individual ribosomes), which may allow selective/preferential translation of mRNAs in cell to generate proteome diversity/plasticity. Earlier studies have shown heterogeneity in the ribosomal pool that arose as a consequence of various environmental cues or changes in the toxin-antitoxin systems.Citation62,63 Cellular abundance of initiator tRNA has been thought to be important in regulating the rate-limiting step of initiation. Revelation that the potential of elongator tRNAs to initiate is unleashed by decreasing pools of initiator tRNA, and given that relative levels of initiator/elongator tRNAs in the cell do change,Citation48 it seems that alternative sites of initiation determined by the elongator tRNAs may generate novel polypeptides to create another level of proteome diversity/plasticity in the cell. Such diversity may be crucial for the cell to re-program cellular physiology to tide over the stressful conditions. There are circumstances where initiator tRNA levels are transcriptionally down-regulatedCitation44,48 or diminished by ribonucleases such as VapC.Citation64 Furthermore, although not yet experimentally tested, our recent finding that extended SD:aSD interaction allows for initiation with an initiator tRNA lacking the highly conserved feature of the 3GC pairs suggests that the natural occurrences of SD sequences in translation initiation regions or at internal positions could also impact the extent and/or sites of initiation by elongator tRNAs. It is known that there are various mRNAs with extended SD sequences in the upstream or within the coding regions which lead to pausing of ribosomes.Citation65 Preference for SD sequence uses has already been shown to vary with temperature.Citation66 It is also of interest to note that the 70S ribosomes from E. coli with low levels of tRNAfMet contained 15% less S1 protein.Citation43 Earlier studies have shown that S1 depleted ribosomes use 70S mode of initiation in leaderless mRNAs.Citation55,57 Thus, it seems that cells may exploit a number of strategies available to it to generate proteome diversity. However, a challenge that these observations pose is to design methods to detect and identify minor populations of peptides/proteins, which are to likely arise by initiation from the alternative sites. Clearly, more sensitive genetic and biochemical assays would need to be developed to investigate into the possibility of the impact of the alternative means of initiation by elongator tRNAs.
Disclosure of Potential Conflicts of Interest
No potential conflicts of interest were disclosed.
Funding
The work in the investigator's laboratory is supported by funds from the Department of Science and Technology (DST), Department of Biotechnology (DBT), and the Council of Scientific and Industrial Research (CSIR), New Delhi. U.V. is a J.C. Bose fellow of DST. S.S. is supported by a Shyama Prasad Mukherjee SRF of CSIR. S.B. was supported by an SRF of CSIR.
References
- Nierhaus KH. The assembly of prokaryotic ribosomes. Biochimie 1991; 73:739-55; PMID:1764520; http://dx.doi.org/10.1016/0300-9084(91)90054-5
- Neidhardt FC, Umbarger HE. in Escherichia coli and Salmonella. ASM Press, Washington, DC.; 1996; 13-16
- Gold L. Posttranscriptional regulatory mechanisms in Escherichia coli. Annu Rev Biochem 1988; 57:199-233; PMID:3052271; http://dx.doi.org/10.1146/annurev.bi.57.070188.001215
- Julian P, Milon P, Agirrezabala X, Lasso G, Gil D, Rodnina MV, Valle M. The Cryo-EM structure of a complete 30S translation initiation complex from Escherichia coli. PLoS Biol 2011; 9:e1001095; PMID:21750663; http://dx.doi.org/10.1371/journal.pbio.1001095
- Marshall RA, Aitken CE, Puglisi JD. GTP hydrolysis by IF2 guides progression of the ribosome into elongation. Mol Cell 2009; 35:37-47; PMID:19595714; http://dx.doi.org/10.1016/j.molcel.2009.06.008
- Hartz D, McPheeters DS, Gold L. Selection of the initiator tRNA by Escherichia coli initiation factors. Genes Dev 1989; 3:1899-912; PMID:2695390; http://dx.doi.org/10.1101/gad.3.12a.1899
- Antoun A, Pavlov MY, Lovmar M, Ehrenberg M. How initiation factors maximize the accuracy of tRNA selection in initiation of bacterial protein synthesis. Mol Cell 2006; 23:183-93; PMID:16857585; http://dx.doi.org/10.1016/j.molcel.2006.05.030
- Antoun A, Pavlov MY, Lovmar M, Ehrenberg M. How initiation factors tune the rate of initiation of protein synthesis in bacteria. EMBO J 2006; 25:2539-50; PMID:16724118; http://dx.doi.org/10.1038/sj.emboj.7601140
- Canonaco MA, Calogero RA, Gualerzi CO. Mechanism of translational initiation in prokaryotes. Evidence for a direct effect of IF2 on the activity of the 30 S ribosomal subunit. FEBS Lett 1986; 207:198-204; PMID:3533628; http://dx.doi.org/10.1016/0014-5793(86)81488-0
- Gualerzi CO, Brandi L, Caserta E, Garofalo C, Lammi M, La Teana A, Petrelli D, Spurio R, Tomsic J, Pon CL. Initiation factors in the early events of mRNA translation in bacteria. Cold Spring Harb Symp Quant Biol 2001; 66:363-76; PMID:12762039; http://dx.doi.org/10.1101/sqb.2001.66.363
- Gualerzi CO, Pon CL. Initiation of mRNA translation in prokaryotes. Biochemistry 1990; 29:5881-89; PMID:2200518; http://dx.doi.org/10.1021/bi00477a001
- La Teana A, Pon CL, Gualerzi CO. Late events in translation initiation. Adjustment of fMet-tRNA in the ribosomal P-site. J Mol Biol 1996; 256:667-75; PMID:8642589; http://dx.doi.org/10.1006/jmbi.1996.0116
- Milon P, Konevega AL, Gualerzi CO, Rodnina MV. Kinetic checkpoint at a late step in translation initiation. Mol Cell 2008; 30:712-20; PMID:18570874; http://dx.doi.org/10.1016/j.molcel.2008.04.014
- Tomsic J, Vitali LA, Daviter T, Savelsbergh A, Spurio R, Striebeck P, Wintermeyer W, Rodnina MV, Gualerzi CO. Late events of translation initiation in bacteria: a kinetic analysis. EMBO J 2000; 19:2127-36; PMID:10790378; http://dx.doi.org/10.1093/emboj/19.9.2127
- Dyson MR, Mandal N, RajBhandary UL. Relationship between the structure and function of Escherichia coli initiator tRNA. Biochimie 1993; 75:1051-60; PMID:7515283; http://dx.doi.org/10.1016/0300-9084(93)90004-C
- Mayer C, Stortchevoi A, Kohrer C, Varshney U, RajBhandary UL. Initiator tRNA and its role in initiation of protein synthesis. Cold Spring Harb Symp Quant Biol 2001; 66:195-206; PMID:12762022; http://dx.doi.org/10.1101/sqb.2001.66.195
- RajBhandary UL. Initiator transfer RNAs. J Bacteriol 1994; 176:547-52; PMID:7507918
- Varshney U, RajBhandary UL. Initiation of protein synthesis from a termination codon. Proc Natl Acad Sci U S A 1990; 87:1586-90; PMID:2406724; http://dx.doi.org/10.1073/pnas.87.4.1586
- Lee CP, Seong BL, RajBhandary UL. Structural and sequence elements important for recognition of Escherichia coli formylmethionine tRNA by methionyl-tRNA transformylase are clustered in the acceptor stem. J Biol Chem 1991; 266:18012-7; PMID:1917939
- Thanedar S, Kumar NV, Varshney U. The fate of the initiator tRNAs is sensitive to the critical balance between interacting proteins. J Biol Chem 2000; 275:20361-7; PMID:10748005; http://dx.doi.org/10.1074/jbc.M001238200
- Seong BL, RajBhandary UL. Escherichia coli formylmethionine tRNA: mutations in GGGCCC sequence conserved in anticodon stem of initiator tRNAs affect initiation of protein synthesis and conformation of anticodon loop. Proc Natl Acad Sci U S A 1987; 84:334-8; PMID:3540960; http://dx.doi.org/10.1073/pnas.84.2.334
- Varshney U, Lee CP, Seong BL, RajBhandary UL. Mutants of initiator tRNA that function both as initiators and elongators. J Biol Chem 1991; 266:18018-24; PMID:1917940
- Mandal N, Mangroo D, Dalluge JJ, McCloskey JA, Rajbhandary UL. Role of the three consecutive G:C base pairs conserved in the anticodon stem of initiator tRNAs in initiation of protein synthesis in Escherichia coli. RNA 1996; 2:473-82; PMID:8665414
- Varshney U, Lee CP, RajBhandary UL. From elongator tRNA to initiator tRNA. Proc Natl Acad Sci U S A 1993; 90:2305-9; PMID:8460138; http://dx.doi.org/10.1073/pnas.90.6.2305
- Seong BL, RajBhandary UL. Mutants of Escherichia coli formylmethionine tRNA: a single base change enables initiator tRNA to act as an elongator in vitro. Proc Natl Acad Sci U S A 1987; 84:8859-63; PMID:3321059; http://dx.doi.org/10.1073/pnas.84.24.8859
- Sprinzl M, Hartmann T, Weber J, Blank J, Zeidler R. Compilation of tRNA sequences and sequences of tRNA genes. Nucleic Acids Res 1989; 17 Suppl:r1-172; PMID:2470031; http://dx.doi.org/10.1093/nar/17.suppl.r1
- Samhita L, Shetty S, Varshney U. Unconventional initiator tRNAs sustain Escherichia coli. Proc Natl Acad Sci U S A 2012; 109:13058-63; PMID:22829667; http://dx.doi.org/10.1073/pnas.1207868109
- Dong J, Munoz A, Kolitz SE, Saini AK, Chiu WL, Rahman H, Lorsch JR, Hinnebusch AG. Conserved residues in yeast initiator tRNA calibrate initiation accuracy by regulating preinitiation complex stability at the start codon. Genes Dev 2014; 28:502-20; PMID:24589778; http://dx.doi.org/10.1101/gad.236547.113
- Lancaster L, Noller HF. Involvement of 16S rRNA nucleotides G1338 and A1339 in discrimination of initiator tRNA. Mol Cell 2005; 20:623-32; PMID:16307925; http://dx.doi.org/10.1016/j.molcel.2005.10.006
- Dallas A, Noller HF. Interaction of translation initiation factor 3 with the 30S ribosomal subunit. Mol Cell 2001; 8:855-64; PMID:11684020; http://dx.doi.org/10.1016/S1097-2765(01)00356-2
- Arora S, Bhamidimarri SP, Bhattacharyya M, Govindan A, Weber MH, Vishveshwara S, Varshney U. Distinctive contributions of the ribosomal P-site elements m2G966, m5C967 and the C-terminal tail of the S9 protein in the fidelity of initiation of translation in Escherichia coli. Nucleic Acids Res 2013; 41:4963-75; PMID:23530111; http://dx.doi.org/10.1093/nar/gkt175
- Burakovsky DE, Prokhorova IV, Sergiev PV, Milon P, Sergeeva OV, Bogdanov AA, Rodnina MV, Dontsova OA. Impact of methylations of m2G966/m5C967 in 16S rRNA on bacterial fitness and translation initiation. Nucleic Acids Res 2012; 40:7885-95; PMID:22649054; http://dx.doi.org/10.1093/nar/gks508
- Selmer M, Dunham CM, Murphy FVt, Weixlbaumer A, Petry S, Kelley AC, Weir JR, Ramakrishnan V. Structure of the 70S ribosome complexed with mRNA and tRNA. Science 2006; 313:1935-42; PMID:16959973; http://dx.doi.org/10.1126/science.1131127
- Hoang L, Fredrick K, Noller HF. Creating ribosomes with an all-RNA 30S subunit P site. Proc Natl Acad Sci U S A 2004; 101:12439-43; PMID:15308780; http://dx.doi.org/10.1073/pnas.0405227101
- Kimura S, Suzuki T. Fine-tuning of the ribosomal decoding center by conserved methyl-modifications in the Escherichia coli 16S rRNA. Nucleic Acids Res 2010; 38:1341-52; PMID:19965768; http://dx.doi.org/10.1093/nar/gkp1073
- Das G, Thotala DK, Kapoor S, Karunanithi S, Thakur SS, Singh NS, Varshney U. Role of 16S ribosomal RNA methylations in translation initiation in Escherichia coli. EMBO J 2008; 27:840-51; PMID:18288206; http://dx.doi.org/10.1038/emboj.2008.20
- Brimacombe R, Mitchell P, Osswald M, Stade K, Bochkariov D. Clustering of modified nucleotides at the functional center of bacterial ribosomal RNA. FASEB J 1993; 7:161-7; PMID:8422963
- Seshadri A, Dubey B, Weber MH, Varshney U. Impact of rRNA methylations on ribosome recycling and fidelity of initiation in Escherichia coli. Mol Microbiol 2009; 72:795-808; PMID:19400784; http://dx.doi.org/10.1111/j.1365-2958.2009.06685.x
- Das G, Dineshkumar TK, Thanedar S, Varshney U. Acquisition of a stable mutation in metY allows efficient initiation from an amber codon in Escherichia coli. Microbiology 2005; 151:1741-50; PMID:15941983; http://dx.doi.org/10.1099/mic.0.27915-0
- Ishii S, Kuroki K, Imamoto F. tRNAMetf2 gene in the leader region of the nusA operon in Escherichia coli. Proc Natl Acad Sci U S A 1984; 81:409-413; PMID:6364142; http://dx.doi.org/10.1073/pnas.81.2.409
- Mandal N, RajBhandary UL. Escherichia coli B lacks one of the two initiator tRNA species present in E. coli K-12. J Bacteriol 1992; 174:7827-30; PMID:1447149
- Kapoor S, Das G, Varshney U. Crucial contribution of the multiple copies of the initiator tRNA genes in the fidelity of tRNA(fMet) selection on the ribosomal P-site in Escherichia coli. Nucleic Acids Res 2011; 39:202-12; PMID:20798174; http://dx.doi.org/10.1093/nar/gkq760
- Samhita L, Virumae K, Remme J, Varshney U. Initiation with elongator tRNAs. J Bacteriol 2013; 195:4202-9; PMID:23852868; http://dx.doi.org/10.1128/JB.00637-13
- Krin E, Laurent-Winter C, Bertin PN, Danchin A, Kolb A. Transcription regulation coupling of the divergent argG and metY promoters in Escherichia coli K-12. J Bacteriol 2003; 185:3139-46; PMID:12730174; http://dx.doi.org/10.1128/JB.185.10.3139-3146.2003
- Kanduc D. Changes of tRNA population during compensatory cell proliferation: differential expression of methionine-tRNA species. Arch Biochem Biophys 1997; 342:1-5; PMID:9185607; http://dx.doi.org/10.1006/abbi.1996.9869
- Dittmar KA, Sorensen MA, Elf J, Ehrenberg M, Pan T. Selective charging of tRNA isoacceptors induced by amino-acid starvation. EMBO Rep 2005; 6:151-7; PMID:15678157; http://dx.doi.org/10.1038/sj.embor.7400341
- Conesa C, Ruotolo R, Soularue P, Simms TA, Donze D, Sentenac A, Dieci G. Modulation of yeast genome expression in response to defective RNA polymerase III-dependent transcription. Mol Cell Biol 2005; 25:8631-42; PMID:16166643; http://dx.doi.org/10.1128/MCB.25.19.8631-8642.2005
- Nagase T, Ishii S, Imamoto F. Differential transcriptional control of the two tRNA(fMet) genes of Escherichia coli K-12. Gene 1988; 67:49-57; PMID:2843439; http://dx.doi.org/10.1016/0378-1119(88)90007-8
- Guillon JM, Mechulam Y, Schmitter JM, Blanquet S, Fayat G. Disruption of the gene for Met-tRNA(fMet) formyltransferase severely impairs growth of Escherichia coli. J Bacteriol 1992; 174:4294-301; PMID:1624424
- Ling J, So BR, Yadavalli SS, Roy H, Shoji S, Fredrick K, Musier-Forsyth K, Ibba M. Resampling and editing of mischarged tRNA prior to translation elongation. Mol Cell 2009; 33:654-60; PMID:19285947; http://dx.doi.org/10.1016/j.molcel.2009.01.031
- Shetty S, Nadimpalli H, Shah RA, Arora S, Das G, Varshney U. An extended Shine-Dalgarno sequence in mRNA functionally bypasses a vital defect in initiator tRNA. Proc Natl Acad Sci U S A 2014; 111:E4224-33; PMID:25246575; http://dx.doi.org/10.1073/pnas.1411637111
- Mawn MV, Fournier MJ, Tirrell DA, Mason TL. Depletion of free 30S ribosomal subunits in Escherichia coli by expression of RNA containing Shine-Dalgarno-like sequences. J Bacteriol 2002; 184:494-502; PMID:11751827; http://dx.doi.org/10.1128/JB.184.2.494-502.2002
- Masuda T, Petrov AN, Iizuka R, Funatsu T, Puglisi JD, Uemura S. Initiation factor 2, tRNA, and 50S subunits cooperatively stabilize mRNAs on the ribosome during initiation. Proc Natl Acad Sci U S A 2012; 109:4881-5; PMID:22411833; http://dx.doi.org/10.1073/pnas.1118452109
- Moll I, Blasi U. Differential inhibition of 30S and 70S translation initiation complexes on leaderless mRNA by kasugamycin. Biochem Biophys Res Commun 2002; 297:1021-6; PMID:12359258; http://dx.doi.org/10.1016/S0006-291X(02)02333-1
- Kaberdina AC, Szaflarski W, Nierhaus KH, Moll I. An unexpected type of ribosomes induced by kasugamycin: a look into ancestral times of protein synthesis? Mol Cell 2009; 33:227-36; PMID:19187763; http://dx.doi.org/10.1016/j.molcel.2008.12.014
- Poldermans B, Goosen N, Van Knippenberg PH. Studies on the function of two adjacent N6,N6-dimethyladenosines near the 3' end of 16 S ribosomal RNA of Escherichia coli. I. The effect of kasugamycin on initiation of protein synthesis. J Biol Chem 1979; 254:9085-9; PMID:383710
- Moll I, Hirokawa G, Kiel MC, Kaji A, Blasi U. Translation initiation with 70S ribosomes: an alternative pathway for leaderless mRNAs. Nucleic Acids Res 2004; 32:3354-63; PMID:15215335; http://dx.doi.org/10.1093/nar/gkh663
- Abdi NM, Fredrick K. Contribution of 16S rRNA nucleotides forming the 30S subunit A and P sites to translation in Escherichia coli. RNA 2005; 11:1624-32; PMID:16177132; http://dx.doi.org/10.1261/rna.2118105
- Qin D, Abdi NM, Fredrick K. Characterization of 16S rRNA mutations that decrease the fidelity of translation initiation. RNA 2007; 13:2348-55; PMID:17942743; http://dx.doi.org/10.1261/rna.715307
- Korostelev A, Trakhanov S, Asahara H, Laurberg M, Lancaster L, Noller HF. Interactions and dynamics of the Shine Dalgarno helix in the 70S ribosome. Proc Natl Acad Sci U S A 2007; 104:16840-3; PMID:17940016; http://dx.doi.org/10.1073/pnas.0707850104
- Hartz D, Binkley J, Hollingsworth T, Gold L. Domains of initiator tRNA and initiation codon crucial for initiator tRNA selection by Escherichia coli IF3. Genes Dev 1990; 4:1790-800; PMID:1701151; http://dx.doi.org/10.1101/gad.4.10.1790
- Sauert M, Temmel H, Moll I. Heterogeneity of the translational machinery: Variations on a common theme. Biochimie 2014; PMID:25542647
- Vesper O, Amitai S, Belitsky M, Byrgazov K, Kaberdina AC, Engelberg-Kulka H, Moll I. Selective translation of leaderless mRNAs by specialized ribosomes generated by MazF in Escherichia coli. Cell 2011; 147:147-57; PMID:21944167; http://dx.doi.org/10.1016/j.cell.2011.07.047
- Winther KS, Gerdes K. Enteric virulence associated protein VapC inhibits translation by cleavage of initiator tRNA. Proc Natl Acad Sci U S A 2011; 108:7403-7; PMID:21502523; http://dx.doi.org/10.1073/pnas.1019587108
- Li GW, Oh E, Weissman JS. The anti-Shine-Dalgarno sequence drives translational pausing and codon choice in bacteria. Nature 2012; 484:538-41; PMID:22456704; http://dx.doi.org/10.1038/nature10965
- Vimberg V, Tats A, Remm M, Tenson T. Translation initiation region sequence preferences in Escherichia coli. BMC Mol Biol 2007; 8:100; PMID:17973990; http://dx.doi.org/10.1186/1471-2199-8-100