Abstract
We discovered a chromosomal locus containing 2 toxin-antitoxin modules (TAs) with an antisense transcriptional organization in the E. faecalis clinical isolate V583. These TAs are homologous to the type I txpA-ratA system and the type II mazEF, respectively. We have shown that the putative MazF is toxic for E. coli and triggers RNA degradation, and its cognate antitoxin MazE counteracts toxicity. The second module, adjacent to mazEF, expresses a toxin predicted to belong to the TxpA type I family found in Firmicutes, and the antisense RNA antidote, RatA. Genomic analysis indicates that the cis-association of mazEF and txpA-ratA modules has been favored during evolution, suggesting a selective advantage for this TA organization in the E. faecalis species. We showed regulatory interplays between the 2 modules, involving transcription control and RNA stability. Remarkably, our data reveal that MazE and MazEF have a dual transcriptional activity: they act as autorepressors and activate ratA transcription, most likely in a direct manner. RatA controls txpA RNA levels through stability. Our data suggest a pivotal role of MazEF in the coordinated expression of mazEF and txpA-ratA modules in V583. To our knowledge, this is the first report describing a crosstalk between type I and II TAs.
Introduction
The Gram positive bacterium Enterococcus faecalis is a natural resident of the gastrointestinal tract that has emerged as a major cause of nosocomial infections over the last 30 years. The dual life style of E. faecalis relies primarily on remarkable capacities to face and survive in harsh environmental conditions.Citation1-3 We previously reported a peculiar chromosomal locus (coined hereafter TAI-II locus) containing 2 adjacent putative toxin-antitoxin (TA) modules, organized in an antisense orientation and predicted to belong to type I (txpA-ratA) and type II (mazEF) families in the clinical E. faecalis isolate V583 ().Citation4 The so-called txpA-ratA module was shown to encode a TA distantly related to txpA-ratA in B. subtilis.Citation5,6 Bacterial TAs are non-essential genetic elements co-expressing a stable toxin protein that inhibits cell growth, and the antidote, an unstable cognate antitoxin. Originally described as systems ensuring inheritance of ‘plasmid addiction’ in the absence of selective pressure, TAs have also been found abundantly in bacterial chromosomes. Remarkably, the number of TAs varies from an isolate to another within the same species, and throughout the bacterial kingdom.Citation5,7-9 Such a variability remains poorly understood, but the impact of TAs on bacterial growth and physiological adjustments in response to environmental changes has been demonstrated, including antibiotic resistance, biofilm development, stress adaptation, virulence and persistence during chronic infections.Citation10-15
Figure 1. Crosstalk regulation at the TAI-II locus between mazEF and txpA-ratA modules. (A). Gene organization and transcripts expressed by the TAI-II locus in the E. faecalis V583 isolate. (B). DNA regions used to overexpress various portions of the TAI-II locus. (C). Northern blots showing steady-state RNA levels expressed by genes mazEF, txpA, and ratA. Each lane indicates the portion of the TAI-II locus present as multicopy in addition to the chromosomal copy, as described in panel B. ‘CS’: V583 strain carrying the empty vector. Transcripts detected are mentioned on the right side of the panel. Exposure times were optimized for each panel for visualization. (D). Diagram showing fold changes of RNA levels normalized to levels in CS considered as equal to 1. p-values from statistical analysis (Student t test) are reported as ‘*’: p ≤ 0.05; ‘**’: p ≤ 0.01; ‘***’: p ≤0 .001. (E). Steady state RNA levels originating from the TAI-II locus in strains carrying an increased copy number of drm sequences.
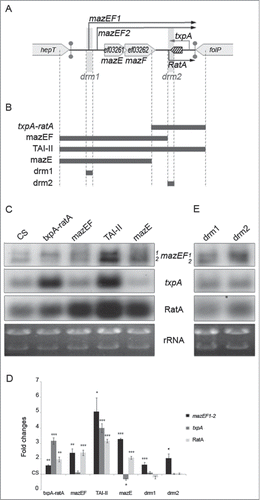
TA modules have been classified in 5 types depending on the nature of the antitoxin and the mechanism used to counteract the toxin effects.Citation16-19 Type I TA (TA-I) comprises an antisense non-coding RNA gene that pairs with the mRNA encoding toxin and prevents translation or activates degradation.Citation5,20-23 TA-I toxins, such as TxpA, are generally small hydrophobic proteins forming pores in membranes.Citation5,24-27 Type II TA systems (TA-II) are usually made up by a bicistronic operon encoding the antitoxin protein that interacts with the toxin and inactivates its deleterious effects. Generally, TA-II toxin and antitoxin repress their own transcription via ‘conditional cooperativity’, a process relying on the DNA-binding properties of the antitoxin, the affinity between the toxin and the antitoxin, and their limited abundance.Citation28-30 Different activities have been characterized among TA-II toxins, and most of them inhibit translation acting as endoribonucleases (endoRNases), including MazF.Citation7,12,31,32 Although TAs are key players in adjusting physiology during adaptation processes, how bacteria coordinate their expression individually and as a whole set remain poorly understood.Citation15,33-37 In particular, a limited number of studies have focused on putative crosstalks between TAs. For instance in E. coli, the TA-II endoribonuclease toxin MsqR was shown to enhance a selective degradation of the ghoST mRNA in favor of the type V TA toxin GhoT, by direct cleavage within the ghoS sequence and, a complex regulatory interplay has been unveiled between several TA-II modules.Citation38,39 These observations suggest that together TAs may mediate concerted physiological responses in the cell.
In this work, we demonstrated that open reading frames at TAI-II locus in the chromosome of E. faecalis V583 strain feature TA-I and TA-II module properties, and characterized the regulatory interplay between mazEF and txpA-ratA modules, where MazEF ensures a pivotal role in the coordinated expression of the 2 modules.
Results
Chromosomal organization of mazEF and txpA-ratA modules within the E. faecalis species
In the E. faecalis V583 isolate, the mazEF/txpA-ratA locus (TAI-II locus) encompasses ∼1,500 nucleotides (nt) on the chromosome and expresses several transcripts ().Citation4 The mazEF module is transcribed from 2 promoters providing mazEF1 (∼1,200 nt) and mazEF2 (˜800 nt) RNAs. The txpA-ratA system consists of the toxin encoding gene, txpA (ef3263 whose transcript was originally named ref40, ∼290 nt), and a non-coding asRNA transcribed in the same direction as mazEF, named RatA (˜300 nt, originally named Ref46). Our previous 3′ RNA end mappings have shown the antisense organization of mRNAs encoding the MazF and TxpA toxins.Citation4,40 Two additional transcripts, Ref5J and Ref45 were previously reported.Citation4 Because none of them was detected by Northern blot in the diverse strains and growth conditions used here, they will be not considered. Moreover, the TAI-II locus features a 167 bp long direct repeat motif, ‘drm1’ and ‘drm2’, flanking the mazEF module. drm1 and drm2 contain the transcription start sites (TSSs), the −10 boxes and extend 5 nucleotides upstream of the mazEF1 and ratA promoters, respectively (; Fig. S1), indicating possible common regulatory elements. Together, antisense organization and drms strongly suggest a regulatory crosstalk between the TA-II (mazEF) and the TA-I (txpA-ratA) modules.
Searches in the V583 genome show that mazEF and txpA sequences are unique at both nucleotide and protein levels. Across the E. faecalis species, the occurrences of each module vary, and no correlation with the phylogeny of strains or virulence traits is observed. Out of 65 assembled genomic sequences from independent isolates, publicly available from the NCBI Genome Database, only 2 strains do not carry homologous sequences to any module indicating a possible selective advantage for the presence of these TA systems (, and data not shown). Half of the strains contain the txpA-ratA system alone and only a few bear mazEF alone (∼8%), suggesting that the presence of mazEF in the absence of txpA-ratA may not be favored (Table 1). About a third of the strains contain both modules, and remarkably both modules always map to the same locus and form a cis organization identical to the one described in the V583 strain, including drm sequences (). This observation suggests a functional advantage provided by the proximity of the modules and reinforces our hypothesis of a crosstalk between mazEF and txpA-ratA.
Table 1. Distribution of mazEF and txpA-ratA modules in E. faecalis species. Numbers provided take into account only the independent isolates available from NCBI database whose genome have been assembled. ‘%’: percentage within the 65 assembled genomes (February 2013). ‘cis’: organization of the TAI-II locus (Fig. 1A). ‘trans’: mazEF and txpA-ratA modules found at different loci
TxpA and MazF of E. faecalis are toxins
First, we show that MazF (121 amino acids) and TxpA (27 aa) are genuine toxins. The corresponding ORFs were expressed in E. coli under control of the inducible promoter PBad. In contrast to TxpA from Bacillus subtilis which is not toxic in E. coli and does not induce lysis,Citation27 the expression of TxpA from E. faecalis V583 provoked cell death without lysis (Fig. S2 and data not shown), showing the toxic effect of the protein. This data fully agrees with the previous report on TxpA from E. faecalis.Citation5 Similarly, the induction of MazF from E. faecalis V583 leads to a total growth inhibition which is reverted when MazE (76 aa) is co-expressed from the inducible promoter Ptac, indicating the toxic effect of MazF and the antitoxin activity of MazE (Fig. S2).
Then, we tested whether MazF from E. faecalis was able to trigger RNA degradation. We analyzed the stability of lpp and ompA transcripts in E. coli in a time course experiment when the toxin was induced (). lpp and ompA RNAs are targets for MazF in E. coli.Citation41 and are commonly used as indicators of the RNase activity of TA-II toxins. MazF from E. coli was used as control for this set of experiments. In comparison to the vector control, both lpp and ompA mRNAs show a decreased abundance during the time course, indicating degradation (). Although both toxins inhibit growth of E. coli similarly (), MazF from E. coli induces degradation more efficiently than MazF from E. faecalis. The consensus sequences recognized by TA encoded endoRNases vary from one species to another, and it may that the abundance of sequences recognized by the 2 toxins in lpp and ompA is different.Citation17 However, it is also possible that the RNA mediated degradation of lpp and ompA by MazF from E. faecalis is indirect and a consequence of the toxic effect of the protein in E. coli.
Figure 2. MazF and TxpA from E. faecalis are toxins. (A). MazF from E. faecalis induces RNA degradation in E. coli. Time after induction is mentioned at the top of each lane. Transcripts probed are indicated on the right side of the panel. (B). Growth curve showing the toxic effect of MazF from E. faecalis when expressed in E. coli. MazF from E. coli was used as control.
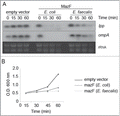
Trans and cis regulatory crosstalks between mazEF and txpA-ratA modules
To investigate potential regulatory interplays between mazEF and txpA-ratA modules, we overexpressed each module separately or together in a cis-association, in addition to the TAI-II chromosomal locus (). As readout, we used RNA levels expressed from each gene, and thereby we investigated: i) the effects of one module cloned on a plasmid (˜10 molcules per cell).Citation42 on the expression of the other present at its chromosomal locus (i.e. trans-effect), and ii) the changes in the expression of one module cloned on a plasmid in comparison to its expression at the chromosomal locus (i.e., cis-effect). We verified that the V583 wild type strain (wt) and the wt strain harboring the empty vector (referred to as control strain (CS)) showed the same abundance for each detected transcript and grew at an equivalent rate (not shown). We also verified by direct extraction that the plasmid copy number was similar for all V583-derivative strains used (not shown).
txpA-ratA activates mazEF
In comparison to CS, the strain harboring the ectopic txpA-ratA module shows an increased abundance of txpA and RatA RNAs, as expected due to higher copy number (, lane ‘txpA-ratA’). However, the magnitude of the increase differs between transcripts: ∼3.2- and ∼2-fold for txpA and RatA, respectively. Such an increase leads to an expression pattern in favor of txpA, in contrast to the reverse pattern observed in CS where the RatA signal is higher than txpA (). This result suggests a regulatory process encoded outside the module that affects the txpA/RatA RNA ratio.
The overexpression of txpA-ratA further causes increased mazEF1 and slightly decreased mazEF2 transcript levels expressed from the chromosome (): globally, the ectopic module trans-activates directly or indirectly mazEF expression (∼1.6-fold, mazEF1 and mazEF2 considered together).
mazEF activates ratA
In comparison to CS, the overexpression of the mazEF module imposes major changes in the RNA pattern (). Beside the expected increase in mazEF RNA levels (∼2.4-fold), remarkably, RatA is also increased (∼2.4-fold). Therefore, mazEF must (directly or indirectly) trans-activate ratA at its chromosomal locus (). In addition and although average values did not show significant variations for the txpA signal in comparison to CS, we did notice a reproducible slight decrease of the toxin RNA in the mazEF strain ().
Crosstalk between modules
To validate the effects of TA modules on each other, we overexpressed the entire TAI-II locus and compared RNA levels to those detected with constructs expressing each module individually. In comparison to the mazEF strain, the presence of txpA-ratA in the TAI-II construct, allows a higher expression of mazEF (∼5-fold relative to CS), (, lanes ‘mazEF’ and ‘TAI-II’). This result further supports the hypothesis that the region encompassing the txpA-ratA module enhances mazEF expression.
In comparison to the txpA-ratA strain, the presence of mazEF in cis mildly increases the txpA level (≤25%) and more significantly RatA (≥50%); in comparison to CS, transcript levels increase ∼4- and ∼3.1-fold, respectively (). Indeed, mazEF restores the RNA pattern observed in the CS strain where the RatA signal is higher than the txpA signal; a reverse pattern to the one observed in the txpA-ratA strain where signals are in favor of the toxin (). This further supports our conclusion that mazEF activates ratA.
MazE and MazEF coordinate gene expression at the TAI-II locus
In E. coli, MazE has been described as a ‘milder’ transcription repressor of the mazEF promoters than MazEF.Citation43 Thus, we hypothesized that MazE and MazEF proteins in E. faecalis would have similar properties as in E. coli. Therewith, the mazEF-dependent activation of ratA could be due to the transcriptional activity of MazE and MazEF, since promoters PmazEF1 and PratA share common drm sequences (; Fig. S1). Based on this hypothesis and in comparison to CS, we predicted that the overexpression of MazE in trans would lead to: i) increased levels of mazEF mRNAs since the competition to bind mazEF DNA promoters between the overexpressed MazE and the chromosomally encoded MazEF would be in favor of MazE, a milder repressor than MazEF, and ii) activation of ratA at its chromosomal locus. To test our predictions, MazE was overexpressed using an identical construct to the one used for MazEF except that approximately 2-third of the C-terminal part of MazF were omitted (, lane ‘mazE’). In this strain, we observed: i) increased levels of mazEF mRNAs expressed from the chromosome (∼3.3-fold), indicating that most likely in E. faecalis and E. coli, MazE and MazEF feature similar transcriptional regulatory properties with respect to mazEF promoters;Citation43 ii) increased RatA abundance expressed from the chromosome (∼2.1-fold), showing that MazE directly or indirectly trans-activates ratA, as previously concluded with the mazEF construct; iii) the increased level of RatA is accompanied by a decreased abundance of txpA (˜0.7-fold), ().
We further confirmed the trans-activation of ratA by the mazEF module by introducing the constructs overexpressing MazEF and MazE into the E. faecalis strain OG1RF, an isolate lacking mazEF, but carrying the txpA-ratA module and a single copy of the drm sequence (Fig. S1).Citation44 In OG1RF, the ectopic expression of MazE and MazEF amplified the effects observed in V583: RatA levels increased more than 20-fold and were accompanied by decreased txpA levels (). This data shows that the txpA pattern follows RatA abundance in an inverse related manner and changes in the expression of the antitoxin RatA affects levels of txpA mRNA (). Although signals for txpA mRNA are not visible in some lanes presented , they were still detected when films were overexposed, suggesting that the abundance of txpA does not depend solely on RatA level (not shown). V583 and OG1RF are 2 distant isolates within the E. faecalis species and share ∼75% of their genome,Citation44 suggesting that the trans-activation of ratA by mazEF may be direct. The efficacy of ratA activation by mazE and mazEF in the 2 E. faecalis strains is different, and differences in drm sequences may partly account for this (Fig. S1).
The direct repeat motifs are DNA regulatory elements
PmazEF1 and PratA share common sequences, drm1 and drm2, respectively, encompassing the −10 box and the 5′ transcribed region (Fig. S1). These data prompted us to test if drm sequences were required for the mazEF-mediated effects. For the experimental strategy, we assumed that TAI-II would conform general features of TA systems, i.e. TAs act in a stochastic manner based on low abundance of the encoded gene products, mRNA and proteins.Citation12,13,45 If so, MazEF protein complexes would be present in limiting amount and the increased copy number of target sequences should titrate away MazEF from chromosomal drms. Each drm was cloned on the multicopy plasmid and transcripts expressed from the chromosomal TAI-II locus were analyzed. In comparison to CS, a higher copy number of drm1 or drm2 increases the amount of mazEF transcripts (1.6- and fold2-, respectively, ). Thus, each drm is able to counteract MazEF autorepression, indicating that this regulatory effect requires the drm and most likely MazEF binds to these DNA sequences. A higher effect was observed for drm2, in comparison to drm1, suggesting a better affinity of MazEF for drm2 (). We also noticed different mazEF RNA levels in strains ‘drm2’ and ‘txpA-ratA’ which also bears drm2 as multicopy (), suggesting that sequences in the vicinity of drm2 may modulate the effect of MazEF. In comparison to CS, no significant variations of RatA and txpA levels were observed, in agreements with the model of MazEF being titrated from PratA on the chromosome by the drm in trans, thereby precluding MazEF-dependent activation of ratA (). This data also indicates that independently of MazEF, PratA endows a sufficient activity to counteract txpA expression as observed when the txpA-ratA module is overexpressed alone (, lane ‘txpA-ratA’).
Hereinabove, we observed the mazEF activation due to the increased copy number txpA-ratA (). To verify whether such an activation could also be due to gene-encoded products from txpA-ratA, in addition to the increased copy number of drm2 sequence (), we abolished the transcription initiation of txpA and ratA genes via mutagenesis of the −10 promoter boxes within the TAI-II construct.Citation46 Two consecutive constructs were made: a simple mutant for the toxin, txpA, and then, a double txpA ratA mutant (). For each construct, RNA levels were determined by Northern blot (). In the simple (‘-10txpA’) and in the double mutant (‘-10txpARatA’), mazEF mRNA levels remain unchanged when compared to the TAI-II strain. This data indicates that neither the expression process (i.e., transcription, per se), nor txpA- nor ratA-encoded products affect mazEF expression, but rather that the DNA sequence of txpA-ratA is responsible for the mazEF activation, most likely the drm2 sequence.
Figure 4. Effects of txpA and ratA expression on mazEF. (A). Single (‘-10txpA’) and double (‘-10txpARatA’) −10 box promoter mutants for txpA and ratA genes. (B). Effects of ‘-10txpA’ and ‘-10txpARatA’ mutations on transcripts expressed at the TAI-II locus. Legend otherwise as in .
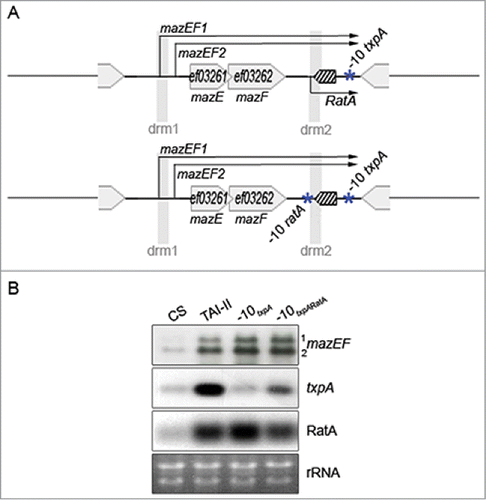
Further, in the simple mutant (‘-10txpA’), txpA level was similar to the CS strain, as expected since the plasmid no longer produces the mRNA encoding toxin (). Remarkably, in that strain RatA abundance is higher than in the TAI-II strain and hence we expect a decreased amount of txpA expressed from the chromosome. However, the txpA amount detected in the simple mutant and in the CS strain are similar (), suggesting that even if RatA and txpA RNAs are fully complementary (), they would not affect the abundance of each other when expressed at different loci. In the double mutant (‘-10txpARatA’), RatA and txpA are both expressed from the chromosome. In comparison to CS, we observed an increased RatA abundance in agreement with our previous conclusion that the increased copy number of mazEF in trans activates ratA expression from the chromosome (). Yet, we observed an increased amount of txpA that we don't fully understand and additional regulatory processes may occur.
All together, these data show that the txpA-ratA module activates mazEF expression via the drm2 sequence encompassing the −10 region of PratA.
TAI-II cross-regulation via RNA synthesis and stability
To evaluate the contribution of transcription and RNA stability to changes observed in RNA levels in strains overexpressing differents parts of the TAI-II locus (), we measured the half-lives of mazEF, RatA and txpA RNAs. Northern blots were performed on total RNAs extracted from bacteria submitted to kinetic experiments after rifampicin treatments; an antibiotic that blocks transcription initiation (; Fig. S3).Citation47 In the CS strain, the 3 transcripts show distinct half-lives: ∼1, ∼2.5 and ∼11 min for mazEF, RatA and txpA, respectively.
Figure 5. Stability measurements of transcripts expressed by E. faecalis V583 strains CS, mazEF, txpA-ratA and TAI-II. Fig.S3 shows a representative experiment of RNA signals detected. For each transcript, half-life was estimated as the time required for 50% decrease of its initial amount.
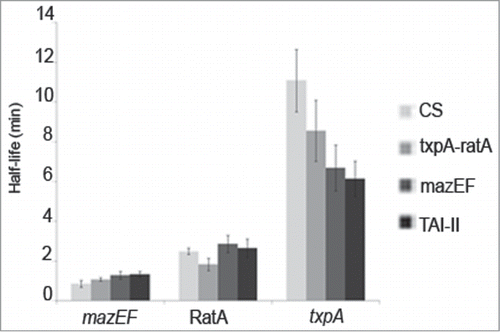
Although mazEF is less stable than RatA and txpA, its stability appears to be independent of the constructs expressed in trans, indicating that variations in RNA levels are mainly due to transcriptional changes ().
RatA shows similar stabilities in CS, mazEF and TAI-II strains (from 2.5 to 2.9 min; ; Fig. S3). In the mazEF strain, where RatA abundance increases compared to CS, RatA is expressed solely from its chromosomal locus, demonstrating that the mazEF-dependent activation of ratA occurs at the transcriptional level (). In contrast, in the txpA-ratA strain and in comparison to the other strains, RatA half-life decreases ∼30% (∼1.8 min; ; Fig. S3) and the ratio of txpA/RatA signals is in favor of txpA. This data indicates that the increased txpA level observed at steady state is accompanied by RatA destabilization ().
txpA stability shows a more complex pattern. In comparison to CS, in strains harboring any module or the entire TAI-II locus, txpA is less stable (). To verify that such an effect was specific to the inserts cloned into the vector, we verified that txpA and RatA RNA levels were equivalent in the CS strain harboring the vector (pJIM2246) and a strain harboring the same vector containing a gene used as negative control (data not shown). The gene used as control was ref25C, encoding an sRNA.Citation4 Thus, this observation indicates that txpA stability is sensitive to the ratio between the txpA RNA and the other encoded products at the TAI-II locus, i.e. RatA and MazEF. When txpA is expressed only from its chromosomal locus, i.e., in CS and mazEF strains, its stability drops near to 2-fold in the later strain (from 11 min in CS to 7 min; ) where RatA level is increased via the mazEF-dependent transcription activation. This data indicates that txpA destabilization is related to increased RatA abundance. When txpA is overexpressed, in strains txpA-ratA and TAI-II, the RNA ratio of txpA/RatA is different: it is in favor of txpA in txpA-ratA strain in comparison to TAI-II strain where RatA is more abundant (). In these backgrounds, txpA half-life decreases by ∼30% (from 8.5 min. to 6 min), while RatA half-life increases by ∼40% (from 1.8 min. to 2.6 min) (; Fig. S3). Thus, stabilities of txpA and RatA RNAs are anti-correlated and modulated by the expression level of the respective other transcript ().
Both mazEF and txpA-ratA are required for H2O2 sensitivity
The systematic cis-association of mazEF and txpA-ratA modules in the E. faecalis species (Table 1) prompted us to test if these TAs could co-exist separately in a ‘trans-association’. We were able to gather the 2 modules cloned in 2 different plasmids in the wild type V583 strain, and all plasmid combinations were viable (not shown). However, empty vectors together were unstable in V583 which didn't allow us to characterize further phenotypes provided by the trans-association of TAs. Yet, this set of constructs strongly suggests that the trans-association of mazEF and txpA-ratA is viable, and most likely that the cis-organization found at the TAI-II locus has been favored during evolution of the E. faecalis species.
Generally, TAs affect cell growth in response to sudden environmental changes that cause an imbalance in favor of the toxin.Citation19 We searched for growth-related phenotypes using the set of V583-derivative strains presented . Several stress conditions were tested, including an array of bacteriostatic and bacteriocidal antibiotics, temperature variations, long term survival (not shown) and an oxidative stress by direct treatment with hydrogen peroxide (H2O2). Among those, only the H2O2 treatment showed a specific effect. As control, we verified that all untreated strains have a comparable growth rate and plating efficiency in the conditions used (, left panel). When cells were treated with H2O2, no significant differences were observed between CS, mazEF and txpA-ratA strains, in contrast the strain overexpressing TAI-II displayed more than 100-fold growth defect (, right panel). Since neither the overexpression of mazEF nor txpA-ratA conferred H2O2 sensitivity, we concluded that both modules, mazEF and txpA-ratA, were required for the phenotype observed indicating that together they may confer particular traits to the strain.
Discussion
Gene products encoded by the TAI-II locus
The TAI-II locus of E. faecalis V583 expresses 4 detectable transcripts, including 3 mRNAs, mazEF1, mazEF2 and txpA, and a non-protein coding sRNA, RatA. We show here that the annotated ORF ef3262 encodes a toxin predicted to belong to the MazF family, and ef3261 encodes a protein that counteracts the deleterious effects of MazF, demonstrating that the so-called MazE is the cognate antitoxin. MazF enhances degradation of lpp and ompA RNAs in E. coli suggesting that it may act as an endoRNase, as described for other members of the MazF family in diverse bacterial species.Citation17 TxpA was previously identified as a putative peptide targeting the membrane and is found in several related Gram positive species. RatA, its cognate antitoxin was characterized as an asRNA.Citation4,5
Regulatory model at the TAI-II locus
The cis-organization of mazEF and txpA-ratA modules, ensures a coordinated expression of the TAI-II locus featuring i) controls previously known for individual TAs in other species,Citation12,48-50 and ii) a regulatory interplay relying on drm sequences and the mazEF-dependent transcriptional activation of ratA (). To our knowledge, this is the first demonstration of a regulatory crosstalk between modules of type-I and -II families.
Figure 7. Regulatory model of the TA crosstalk at the TAI-II locus in E. faecalis V583. The mazEF-encoded product, MazEF, is able to act as an autorepressor and activates ratA transcription by using drm sequences, drm1 and drm2, respectively. MazEF is present in limiting amounts and would act preferentially on drm2, i.e., activates ratA. ratA encodes the antitoxin ncRNA RatA which decreases the stability of the encoding toxin txpA mRNA. By ‘utilizing’ the produced MazEF, indirectly, the txpA-ratA module activates mazEF transcription; a process resulting in novel synthesis of MazEF which then blocks mazEF expression. MazEF proteins and drm sequences at PmazEF1 and PratA promoters are key players of the regulatory crosstalk between the TA-I and TA-II modules at the TAI-II locus. Arrows indicate activation and bars repression processes. Transcriptional and posttranscriptional controls are indicated by full and dashed lines, respectively.
mazEF expression is auto-repressed. Although, we have not confirmed the direct binding of MazE and MazEF to mazEF promoters, our genetic data agree with a model where mazEF is auto-repressedCitation43 and demonstrate that mazE- and mazEF-dependent effects require the drm DNA sequence, including drm2 contained within the ratA promoter region.
txpA transcript levels change in a RatA-dependent manner, and RNA stability is a key factor regulating the expression of the toxin. We hypothesize that txpA and RatA form an RNA duplex substrate for degradation, similarly to the homonymous system in B. subtilis that proved to be recognized and degraded by RNase III, a double-strand-specific RNase.Citation51 However, in E. fecalis, the control of txpA levels is not strictly and only dependent on RatA. The drastically increased abundance of RatA in V583 and OG1RF strains due to the ectopic expression of MazE and MazEF reduces txpA levels, yet some a certain amount of txpA remains detectable, suggesting that a fraction of txpA molecules is refractory to RatA effects. This is consistent with RNA patterns observed in stability measurements where a significant amount of txpA remains after RatA has been wiped out. Indeed, this is further in favor of a txpA/RatA duplex formation. We assume that half-lives determined within the first minutes after rifampicin treatments result from 2 decaying kinetics: an unstable txpA/RatA duplex and a more stable ‘free’ form of txpA. This is also consistent with reported changes of txpA stability in genetic backgrounds that modify the amounts of partners forming the txpA/RatA duplex since they impact the ratio [duplexed to RatA/free form] of txpA. Furthermore and interestingly, in the −10txpA mutant, the txpA level which is solely due to the chromosomal transcription is not affected by the increased RatA level from the vector (i.e. in trans). This indicates that txpA and RatA RNAs most likely interact during their synthesis. Folding constraints may prevent the formation of a duplex efficient for degradation once transcripts have been synthesized. Whether or not the fraction of free txpA mRNA is functional and expresses the TxpA toxin is unknown but translation regulation processes may apply as suggested for other toxins of the TA-I family.Citation21
MazE and the MazEF complex have been described in several bacterial species to bind directly to promoter regions of their encoding genes and repress transcription initiationCitation12 and we have demonstrated here that the mazEF-dependent activation of ratA occurs at the transcriptional level. Although we cannot rule out the possibility that mazEF may repress a transcriptional repressor of ratA, our observations indicate that this activation is most likely direct. In V583, PmazEF1 and PratA share significant homology, drm1 and drm2, and surrounding sequence differences may account for the opposite effect of MazE and MazEF on each promoter. DNA palindromes that encompass the TSS region and the minimal promoter sequence (i.e., −10 and −35 boxes, and the spacer), have been shown to be recognized and bound by MazE and MazEF.Citation43,52-54 We sought for palindromes within promoter sequences of PmazEF1, PmazEF2, PratA, PratA.OG (the ratA promoter in OG1RF) and indeed all 4 promoters feature major inverted repeats flanking TSSs (Fig. S4), suggesting that these DNA elements could be targeted by the MazEF complex. However, that PmazEF1 and PratA.OG have identical sequences within the portion containing the inverted repeats, thus these structural elements alone cannot be responsible for the positive and negative MazEF-dependent transcriptional effects observed on PmazEF1, PratA and PratA.OG. In addition, both drm1 and drm2 overcome mazEF-dependent effects on mazEF repression and ratA activation. This demonstrates that mazEF-mediated control requires drm sequences to function, which supports the hypothesis of a limited number of active MazE and MazEF complexes in the cell. This agrees with oscillatory properties of TA-II loci that enable bistable genetic switches.Citation12,45,55,56 Recent studies have demonstrated that TA-II systems can affect positively or negatively the expression of other TA-II modules.Citation39,57,58 However in these studies, only changes in steady-state RNA levels have been shown, and RNA synthesis (transcription) and RNA stability effects cannot be distinguished. In our experiments, direct RNA stability measurements rule out stability effects of MazE and MazEF on RatA, since the RatA half-life is independent of MazE and MazEF levels.
Thus, our current regulatory model of TAI-II is based on drm sequences recognized by MazEF, which is present in limiting amounts and with a dual transcriptional activity. Our data indicate that MazEF may act preferentially on drm2 (within PratA) as compared to drm1 (within PmazEF1). If so, the MazEF-dependent activation of ratA prevents mazEF-autorepression and reduces txpA RNA stability. This ‘unconventional’ use of MazEF as activator of ratA, enables on its turn MazEF synthesis which results in mazEF transcriptional repression. Indeed, the crosstalk between mazEF and txpA-ratA modules coordinates their negative autoregulation (). As consequence, such a regulatory loop enables higher steady state RNA levels to be expressed and maintained for each module in comparison to a ‘standard’ isolated autorepression. This is substantiated by the significant difference of txpA and RatA RNA levels observed in V583 and OG1RF isolates, and supports the hypothesis that if individually mazEF and txpA-ratA modules offer selective advantages, their regulatory crosstalk can provide strains with novel fitness capacities. A few reports show that TA-II modules coordinate their expression by acting on their respective RNA levels or by direct interactions between non-cognate toxins and antitoxins.Citation39,58 Modeling such interactions suggests that interplays between TAs offer better chances to create heterogeneous populations.Citation59 Thus, it is tempting to speculate that functional and regulatory crosstalks between TAs, associated to genomic plasticity may provide the E. faecalis species with capacities to conquer habitats and adapt life styles.
Materials and Methods
Strains, plasmids and primers
Bacterial strains, plasmids and primers used in this study are listed Table S1. Parental E. coli K12 strains were DJ624Δara,Citation60 MG1655Δ10,Citation61 and DH5α (Invitrogen), and E. faecalis strains were V583, and OG1RF.Citation44,62 Plasmid pCRII-TOPO (Invitrogen) was used for direct cloning of amplicons. Clones were screened with oligos FR.T7 and FR.SP6 (Table S1). Sequences of inserts were verified and cloned into the shuttle vector pJIM2246 at the EcoRI restriction site.Citation42
Direct repeated sequences, drm1 and drm2, were specifically amplified from plasmid constructs harboring mazEF and txpA-ratA modules alone, and then cloned into the vector pJIM2246. Punctual mutants of the −10 promoter boxes for ratA and txpA were obtained directly from the plasmid bearing TAI-II () and using the ‘Q5-Site-Directed mutagenesis kit’ following the manufacturer's protocol (New England Biolabs). The simple mutant ‘-10txpA’ corresponds to changes of the −10 box TATACT of the txpA promoter (PtxpA) into CCCGAC. The double mutant ‘−10txpAratA’ was obtained by using the ‘−10txpA’ simple mutant construct as template and changing the −10 sequence TATAAT of the ratA promoter (PratA) into CCCGAC. Constructs were verified by sequencing the entire TAI-II locus. For each mutant, 2 independent plasmids were used to transform E. faecalis V583 and to perform independent experiments.
Proteins encoded by open reading frames (ORFs) annotated in the E. faecalis V583 chromosome as ef3261, ef3262, ef3263, re-named in this study mazE, mazF and txpA, respectively, were expressed under control of inducible promoters in the K12 E. coli strain DJ624Δara. ef3262 and ef3263 were cloned into pBAD33 under control of PBAD, an arabinose-inducible promoter, and ef3261 was cloned into pKK223-3 under control of Ptac, an IPTG-inducible promoter (Table S1).
Bacterial growth
E. coli derivative strains were grown in Luria Broth medium (LB, Invitrogen) at 37°C in aerated conditions at 160 rpm. To test the toxicity of MazF (Ef3262) and TxpA (Ef3263) in E. coli, pBAD33 derivative plasmids were transformed into the DJ624Δara strain. TxpA was used as a control. Colonies of derivative strains were diluted in 10−2 M MgSO4 and survival was estimated by spotting 10 µl of serial dilutions from 1 to 10−6 on solid M9 minimum medium containing the appropriate antibiotics and inducers.
To assay the anti-MazF activity of MazE, the pKK223-3-MazE construct was introduced into the strain harboring the plasmid pBAD33-MazF and induced by 10 µM IPTG. Plates were incubated at 37°C for 16 hours before reading. To avoid interference, the endoribonuclease activity of MazF from E. faecalis V583 was evaluated in the MG1655Δ10 E. coli strain, a K12 strain deleted for 10 TA systems encoding endoRNases, MG1655Δ10.Citation61 Total RNA was recovered after 0, 15, 30 and 60 minutes of induction of the toxin.
E. faecalis strains were grown in brain heart infusion medium (BHI, Difco) at 37°C in static conditions as previously described.Citation4 Assays using hydrogen peroxide (H2O2) treatments were performed as follows. Bacteria were grown to an OD600 ranged from 0.5 to 0.55 and recovered by 3 min centrifugation at 13 000 rpm, and then resuspended in the same volume of 0.9% NaCl solution with and without (negative control) 50 mM hydrogen peroxide (H2O2). Cells were kept at room temperature for 1 hour, washed with 1 volume 0.9% NaCl and recovered in 1 volume 0.9% NaCl. Bacterial survival was estimated by spotting 4 µl of serial dilutions from 1 to 10−7 on BHI plates. Plates were incubated at 37°C for 16 hours before reading.
When required, for E. faecalis V583 and OG1RF derivative strains, chloramphenicol and tetracycline were added to BHI media at final concentration of 10 and 5 µg/ml, respectively. For E. coli recipient strains, ampicillin, chloramphenicol and tetracycline were added to media when required at final concentrations of 100 µg/ml, 20 µg/ml and 12.5 µg/ml, respectively.
RNA preparation, Northern blots and signal quantification
Preparation of total RNA from E. faecalis strains and Northern blots were performed as previously described.Citation63 Specific DNA oligonucleotides utilized as probe are listed Table S1. Northern blots shown are representative data obtained with at least 3 independent RNA preparations. For all figures shown, 5 µg of total RNA were loaded per lane. To measure RNA stability, bacteria were grown as described above, and at an OD600 ranged between 0.50 and 0.55, they were treated by rifampin at 300 µg/ml as previously described.Citation64 Sensitivity to rifampin was confirmed by monitoring growth. Values provided are averages obtained from at least 4 independent RNA preparations tested. Half-lifes were calculated as the time necessary to obtain half of the amount detected at 0 min.
Signals were quantified using the ImageJ program.65 Variations of RNA levels between strains were estimated by taking ‘pixel values’ of the control strain (‘CS’) as reference, i.e. equal to 1. For each transcript, gel exposures were adapted to visualize signals in each strain. Although, statistical values show the significance of quantifications, strong signals were ‘overexposed’ and corresponding pixel values were possibly underestimated.
BLAST searches
Searches for highly homologous sequences to mazE, mazF, ratA and txpA at DNA and proteins levels within assembled E. faecalis genomes were performed on February 2013.
Disclosure of Potential Conflicts of Interest
No potential conflicts of interest were disclosed.
Supplemental Material
Supplemental data for this article can be accessed on the publisher's website.
1084465_supplemental_files.zip
Download Zip (772.7 KB)Acknowledgments
We acknowledge Tatiana Rochat for comments on the manuscript and our colleagues C. Archambaud, S. Furlan, S Gaubert and L. Rigottier-Gois for constructive comments and materials.
Additional information
Funding
References
- Gilmore MS, Lebreton F, van Schaik W. Genomic transition of enterococci from gut commensals to leading causes of multidrug-resistant hospital infection in the antibiotic era. Curr Opin Microbiol 2013; 16:10-6; PMID:23395351; http://dx.doi.org/10.1016/j.mib.2013.01.006
- Hancock LE, Gilmore MS. Pathogenicity of Enterococci. In: Fischette VA, et al., ed. Gram Positive Pathogens, 2nd Edition. Washington, DC: ASM Press, 2006:299-311
- Arias CA, Murray BE. The rise of the Enterococcus: beyond vancomycin resistance. Nat Rev Microbiol 2012; 10:266-78; PMID:22421879; http://dx.doi.org/10.1038/nrmicro2761
- Fouquier d'Herouel A, Wessner F, Halpern D, Ly-Vu J, Kennedy SP, Serror P, Aurell E, Repoila F. A simple and efficient method to search for selected primary transcripts: non-coding and antisense RNAs in the human pathogen Enterococcus faecalis. Nucleic Acids Res 2011; 39:e46; PMID:21266481; http://dx.doi.org/10.1093/nar/gkr012
- Fozo EM, Makarova KS, Shabalina SA, Yutin N, Koonin EV, Storz G. Abundance of type I toxin-antitoxin systems in bacteria: searches for new candidates and discovery of novel families. Nucleic Acids Res 2010; 38:3743-59; PMID:20156992; http://dx.doi.org/10.1093/nar/gkq054
- Silvaggi JM, Perkins JB, Losick R. Genes for small, noncoding RNAs under sporulation control in Bacillus subtilis. J Bacteriol 2006; 188:532-41; PMID:16385044; http://dx.doi.org/10.1128/JB.188.2.532-541.2006
- Leplae R, Geeraerts D, Hallez R, Guglielmini J, Dreze P, Van Melderen L. Diversity of bacterial type II toxin-antitoxin systems: a comprehensive search and functional analysis of novel families. Nucleic Acids Res 2011; 39:5513-25; PMID:21422074; http://dx.doi.org/10.1093/nar/gkr131
- Makarova KS, Wolf YI, Koonin EV. Comprehensive comparative-genomic analysis of type 2 toxin-antitoxin systems and related mobile stress response systems in prokaryotes. Biol Direct 2009; 4:19; PMID:19493340; http://dx.doi.org/10.1186/1745-6150-4-19
- Goeders N, Van Melderen L. Toxin-antitoxin systems as multilevel interaction systems. Toxins 2014; 6:304-24; PMID:24434905; http://dx.doi.org/10.3390/toxins6010304
- De la Cruz MA, Zhao W, Farenc C, Gimenez G, Raoult D, Cambillau C, Gorvel JP, Méresse S. A toxin-antitoxin module of Salmonella promotes virulence in mice. PLoS Pathog 2013; 9:e1003827; PMID:24385907; http://dx.doi.org/10.1371/journal.ppat.1003827
- Georgiades K, Raoult D. Genomes of the most dangerous epidemic bacteria have a virulence repertoire characterized by fewer genes but more toxin-antitoxin modules. PLoS One 2011; 6:e17962; PMID:21437250; http://dx.doi.org/10.1371/journal.pone.0017962
- Gerdes K, Maisonneuve E. Bacterial persistence and toxin-antitoxin loci. Annu Rev Microbiol 2012; 66:103-23; PMID:22994490; http://dx.doi.org/10.1146/annurev-micro-092611-150159
- Lewis K. Persister cells. Annu Rev Microbiol 2010; 64:357-72; PMID:20528688; http://dx.doi.org/10.1146/annurev.micro.112408.134306
- Moll I, Engelberg-Kulka H. Selective translation during stress in Escherichia coli. Trends Biochem Sci 2012; 37:493-8; PMID:22939840; http://dx.doi.org/10.1016/j.tibs.2012.07.007
- Norton JP, Mulvey MA. Toxin-antitoxin systems are important for niche-specific colonization and stress resistance of uropathogenic Escherichia coli. PLoS Pathog 2012; 8:e1002954; PMID:23055930; http://dx.doi.org/10.1371/journal.ppat.1002954
- Blower TR, Salmond GP, Luisi BF. Balancing at survival's edge: the structure and adaptive benefits of prokaryotic toxin-antitoxin partners. Curr Opin Struct Biol 2011; 21:109-18; PMID:21315267; http://dx.doi.org/10.1016/j.sbi.2010.10.009
- Cook GM, Robson JR, Frampton RA, McKenzie J, Przybilski R, Fineran PC, Arcus VL. Ribonucleases in bacterial toxin-antitoxin systems. Biochim Biophys Acta 2013; 1829:523-31; PMID:23454553; http://dx.doi.org/10.1016/j.bbagrm.2013.02.007
- Hu MX, Zhang X, Li EL, Feng YJ. Recent advancements in toxin and antitoxin systems involved in bacterial programmed cell death. Int J Microbiol 2010; 2010:781430; PMID:21253538; http://dx.doi.org/10.1155/2010/781430
- Schuster CF, Bertram R. Toxin-antitoxin systems are ubiquitous and versatile modulators of prokaryotic cell fate. FEMS Microbiol Lett 2013; 340:73-85; PMID:23289536; http://dx.doi.org/10.1111/1574-6968.12074
- Darfeuille F, Unoson C, Vogel J, Wagner EG. An antisense RNA inhibits translation by competing with standby ribosomes. Mol Cell 2007; 26:381-92; PMID:17499044; http://dx.doi.org/10.1016/j.molcel.2007.04.003
- Durand S, Jahn N, Condon C, Brantl S. Type I toxin-antitoxin systems in Bacillus subtilis. RNA Biol 2012; 9:1491-7; PMID:23059907; http://dx.doi.org/10.4161/rna.22358
- Fozo EM, Kawano M, Fontaine F, Kaya Y, Mendieta KS, Jones KL, Ocampo A, Rudd KE, Storz G. Repression of small toxic protein synthesis by the Sib and OhsC small RNAs. Mol Microbiol 2008; 70:1076-93; PMID:18710431; http://dx.doi.org/10.1111/j.1365-2958.2008.06394.x
- Vogel J, Argaman L, Wagner EG, Altuvia S. The small RNA IstR inhibits synthesis of an SOS-induced toxic peptide. Curr Biol 2004; 14:2271-6; PMID:15620655; http://dx.doi.org/10.1016/j.cub.2004.12.003
- Jahn N, Preis H, Wiedemann C, Brantl S. BsrG/SR4 from Bacillus subtilis-the first temperature-dependent type I toxin-antitoxin system. Mol Microbiol 2012; 83:579-98; PMID:22229825; http://dx.doi.org/10.1111/j.1365-2958.2011.07952.x
- Unoson C, Wagner EG. A small SOS-induced toxin is targeted against the inner membrane in Escherichia coli. Mol Microbiol 2008; 70:258-70; PMID:18761622; http://dx.doi.org/10.1111/j.1365-2958.2008.06416.x
- Sayed N, Nonin-Lecomte S, Rety S, Felden B. Functional and structural insights of a Staphylococcus aureus apoptotic-like membrane peptide from a toxin-antitoxin module. J Biol Chem 2012; 287:43454-63; PMID:23129767; http://dx.doi.org/10.1074/jbc.M112.402693
- Silvaggi JM, Perkins JB, Losick R. Small untranslated RNA antitoxin in Bacillus subtilis. J Bacteriol 2005; 187:6641-50; PMID:16166525; http://dx.doi.org/10.1128/JB.187.19.6641-6650.2005
- Cataudella I, Trusina A, Sneppen K, Gerdes K, Mitarai N. Conditional cooperativity in toxin-antitoxin regulation prevents random toxin activation and promotes fast translational recovery. Nucleic Acids Res 2012; 40:6424-34; PMID:22495927; http://dx.doi.org/10.1093/nar/gks297
- Garcia-Pino A, Balasubramanian S, Wyns L, Gazit E, De Greve H, Magnuson RD, Charlier D, van Nuland NA, Loris R. Allostery and intrinsic disorder mediate transcription regulation by conditional cooperativity. Cell 2010; 142:101-11; PMID:20603017; http://dx.doi.org/10.1016/j.cell.2010.05.039
- Zorzini V, Buts L, Schrank E, Sterckx YG, Respondek M, Engelberg-Kulka H, Loris R, Zangger K, van Nuland NA. Escherichia coli antitoxin MazE as transcription factor: insights into MazE-DNA binding. Nucleic Acids Res 2015; 43:1241-56; PMID:25564525; http://dx.doi.org/10.1093/nar/gku1352
- Van Melderen L. Toxin-antitoxin systems: why so many, what for? Curr Opin Microbiol 2010; 13:781-5; PMID:21041110; http://dx.doi.org/10.1016/j.mib.2010.10.006
- Mutschler H, Gebhardt M, Shoeman RL, Meinhart A. A novel mechanism of programmed cell death in bacteria by toxin-antitoxin systems corrupts peptidoglycan synthesis. PLoS Biol 2011; 9:e1001033; PMID:21445328; http://dx.doi.org/10.1371/journal.pbio.1001033
- Frampton R, Aggio RB, Villas-Boas SG, Arcus VL, Cook GM. Toxin-antitoxin systems of Mycobacterium smegmatis are essential for cell survival. J Biol Chem 2012; 287:5340-56; PMID:22199354; http://dx.doi.org/10.1074/jbc.M111.286856
- Maisonneuve E, Shakespeare LJ, Jorgensen MG, Gerdes K. Bacterial persistence by RNA endonucleases. Proc Natl Acad Sci U S A 2011; 108:13206-11; PMID:21788497; http://dx.doi.org/10.1073/pnas.1100186108
- Schifano JM, Edifor R, Sharp JD, Ouyang M, Konkimalla A, Husson RN, Woychik NA. Mycobacterial toxin MazF-mt6 inhibits translation through cleavage of 23S rRNA at the ribosomal A site. Proc Natl Acad Sci U S A 2013; 110:8501-6; PMID:23650345; http://dx.doi.org/10.1073/pnas.1222031110
- Tsilibaris V, Maenhaut-Michel G, Mine N, Van Melderen L. What is the benefit to Escherichia coli of having multiple toxin-antitoxin systems in its genome? J Bacteriol 2007; 189:6101-8; PMID:17513477; http://dx.doi.org/10.1128/JB.00527-07
- Vesper O, Amitai S, Belitsky M, Byrgazov K, Kaberdina AC, Engelberg-Kulka H, Moll I. Selective Translation of Leaderless mRNAs by Specialized Ribosomes Generated by MazF in Escherichia coli. Cell 2011; 147:147-57; PMID:21944167; http://dx.doi.org/10.1016/j.cell.2011.07.047
- Wang X, Lord DM, Hong SH, Peti W, Benedik MJ, Page R, Wood TK. Type II toxin/antitoxin MqsR/MqsA controls type V toxin/antitoxin GhoT/GhoS. Environ Microbiol 2013; 15:1734-44; PMID:23289863; http://dx.doi.org/10.1111/1462-2920.12063
- Kasari V, Mets T, Tenson T, Kaldalu N. Transcriptional cross-activation between toxin-antitoxin systems of Escherichia coli. BMC Microbiol 2013; 13:45; PMID:23432955; http://dx.doi.org/10.1186/1471-2180-13-45
- Innocenti N, Golumbeanu M, Fouquier d'Herouel A, Lacoux C, Bonnin RA, Kennedy SP, Wessner F, Serror P, Bouloc P, Repoila F, et al. Whole-genome mapping of 5′ RNA ends in bacteria by tagged sequencing: a comprehensive view in Enterococcus faecalis. RNA 2015; 21:1018-30; PMID:25737579; http://dx.doi.org/10.1261/rna.048470.114
- Zhang Y, Zhang J, Hoeflich KP, Ikura M, Qing G, Inouye M. MazF cleaves cellular mRNAs specifically at ACA to block protein synthesis in Escherichia coli. Mol Cell 2003; 12:913-23; PMID:14580342; http://dx.doi.org/10.1016/S1097-2765(03)00402-7
- Renault P, Corthier G, Goupil N, Delorme C, Ehrlich SD. Plasmid vectors for gram-positive bacteria switching from high to low copy number. Gene 1996; 183:175-82; PMID:8996104; http://dx.doi.org/10.1016/S0378-1119(96)00554-9
- Marianovsky I, Aizenman E, Engelberg-Kulka H, Glaser G. The regulation of the Escherichia coli mazEF promoter involves an unusual alternating palindrome. J Biol Chem 2001; 276:5975-84; PMID:11071896; http://dx.doi.org/10.1074/jbc.M008832200
- Bourgogne A, Garsin DA, Qin X, Singh KV, Sillanpaa J, Yerrapragada S, Ding Y, Dugan-Rocha S, Buhay C, Shen H, et al. Large scale variation in Enterococcus faecalis illustrated by the genome analysis of strain OG1RF. Genome Biol 2008; 9:R110; PMID:18611278; http://dx.doi.org/10.1186/gb-2008-9-7-r110
- Kaern M, Elston TC, Blake WJ, Collins JJ. Stochasticity in gene expression: from theories to phenotypes. Nat Rev Genet 2005; 6:451-64; PMID:15883588; http://dx.doi.org/10.1038/nrg1615
- Mekler V, Pavlova O, Severinov K. Interaction of Escherichia coli RNA polymerase sigma70 subunit with promoter elements in the context of free sigma70, RNA polymerase holoenzyme, and the β'-sigma70 complex. J Biol Chem 2011; 286:270-9; PMID:20952386; http://dx.doi.org/10.1074/jbc.M110.174102
- Mosteller RD, Yanofsky C. Transcription of the tryptophan operon in Escherichia coli: rifampicin as an inhibitor of initiation. J Mol Biol 1970; 48:525-31; PMID:4986890; http://dx.doi.org/10.1016/0022-2836(70)90064-1
- Brantl S. Bacterial type I toxin-antitoxin systems. RNA Biol 2012; 9:1488-90; PMID:23324552; http://dx.doi.org/10.4161/rna.23045
- Brantl S, Bruckner R. Small regulatory RNAs from low-GC Gram-positive bacteria. RNA Biol 2014; 11:443-56; PMID:24576839; http://dx.doi.org/10.4161/rna.28036
- Wen J, Fozo EM. sRNA antitoxins: more than one way to repress a toxin. Toxins 2014; 6:2310-35; PMID:25093388; http://dx.doi.org/10.3390/toxins6082310
- Durand S, Gilet L, Condon C. The Essential Function of B. subtilis RNase III Is to Silence Foreign Toxin Genes. PLoS Genet 2012; 8:e1003181; PMID:23300471; http://dx.doi.org/10.1371/journal.pgen.1003181
- Donegan NP, Cheung AL. Regulation of the mazEF toxin-antitoxin module in Staphylococcus aureus and its impact on sigB expression. J Bacteriol 2009; 191:2795-805; PMID:19181798; http://dx.doi.org/10.1128/JB.01713-08
- Rothenbacher FP, Suzuki M, Hurley JM, Montville TJ, Kirn TJ, Ouyang M, Woychik NA. Clostridium difficile MazF toxin exhibits selective, not global, mRNA cleavage. J Bacteriol 2012; 194:3464-74; PMID:22544268; http://dx.doi.org/10.1128/JB.00217-12
- Zhang J, Zhang Y, Inouye M. Characterization of the interactions within the mazEF addiction module of Escherichia coli. J Biol Chem 2003; 278:32300-6; PMID:12810711; http://dx.doi.org/10.1074/jbc.M304767200
- Kruse K, Julicher F. Oscillations in cell biology. Curr Opin Cell Biol 2005; 17:20-6; PMID:15661515; http://dx.doi.org/10.1016/j.ceb.2004.12.007
- Veening JW, Smits WK, Kuipers OP. Bistability, epigenetics, and bet-hedging in bacteria. Annu Rev Microbiol 2008; 62:193-210; PMID:18537474; http://dx.doi.org/10.1146/annurev.micro.62.081307.163002
- Yang M, Gao C, Wang Y, Zhang H, He ZG. Characterization of the interaction and cross-regulation of three Mycobacterium tuberculosis RelBE modules. PLoS One 2010; 5:e10672; PMID:20498855; http://dx.doi.org/10.1371/journal.pone.0010672
- Zhu L, Sharp JD, Kobayashi H, Woychik NA, Inouye M. Noncognate Mycobacterium tuberculosis toxin-antitoxins can physically and functionally interact. J Biol Chem 2010; 285:39732-8; PMID:20876537; http://dx.doi.org/10.1074/jbc.M110.163105
- Fasani RA, Savageau MA. Molecular mechanisms of multiple toxin-antitoxin systems are coordinated to govern the persister phenotype. Proc Natl Acad Sci U S A 2013; 110:E2528-37; PMID:23781105; http://dx.doi.org/10.1073/pnas.1301023110
- Hallez R, Geeraerts D, Sterckx Y, Mine N, Loris R, Van Melderen L. New toxins homologous to ParE belonging to three-component toxin-antitoxin systems in Escherichia coli O157:H7. Mol Microbiol 2010; 76:719-32; PMID:20345661; http://dx.doi.org/10.1111/j.1365-2958.2010.07129.x
- Goeders N, Dreze PL, Van Melderen L. Relaxed cleavage specificity within the RelE toxin family. J Bacteriol 2013; 195:2541-9; PMID:23543711; http://dx.doi.org/10.1128/JB.02266-12
- Paulsen IT, Banerjei L, Myers GS, Nelson KE, Seshadri R, Read TD, Fouts DE, Eisen JA, Gill SR, Heidelberg JF, et al. Role of mobile DNA in the evolution of vancomycin-resistant Enterococcus faecalis. Science 2003; 299:2071-4; PMID:12663927; http://dx.doi.org/10.1126/science.1080613
- Mandin P, Repoila F, Vergassola M, Geissmann T, Cossart P. Identification of new noncoding RNAs in Listeria monocytogenes and prediction of mRNA targets. Nucleic Acids Res 2007; 35:962-74; PMID:17259222; http://dx.doi.org/10.1093/nar/gkl1096
- Repoila F, Gottesman S. Signal transduction cascade for regulation of RpoS: temperature regulation of DsrA. J Bacteriol 2001; 183:4012-23; PMID:11395466; http://dx.doi.org/10.1128/JB.183.13.4012-4023.2001
- Abramoff MD, Magalhaes PJ, Ram SJ. Image Processing with ImageJ. Biophotonics Int 2004; 11:36-42.