ABSTRACT
Antisense Noncoding RNA in the INK4 Locus (ANRIL) is the prime candidate gene at Chr9p21, the well-defined genetic risk locus associated with multiple human diseases including coronary artery disease (CAD), while little is known regarding its role in the pathological processes. Endothelial dysfunction triggers atherosclerotic processes that are causatively linked to CAD. To evaluate the function of ANRIL in human endothelial cells (ECs), we examined ANRIL expression under pathological stimuli and found ANRIL was markedly induced by pro-inflammatory factors. Loss-of-function and chromatin immunoprecipitation approaches revealed that NF-κB mediates TNF-α induced ANRIL expression. RNA sequencing revealed that ANRIL silencing dysregulated expression of inflammatory genes including IL6 and IL8 under TNF-α treatment. We explored the regulatory mechanism of ANRIL on IL6/8 and found that Yin Yang 1 (YY1), an ANRIL binding transcriptional factor revealed by RNA immunoprecipitation, was required for IL6/8 expression under TNF-α treatment. YY1 was enriched at promoter loci of IL6/8 and ANRIL silencing impaired the enrichment, indicating a cooperation between ANRIL and YY1 in the regulation of inflammatory genes. For the first time, we establish the connection between ANRIL and NF-κB pathway and show that ANRIL regulates inflammatory responses through binding with YY1. The newly identified TNF-α-NF-κB-ANRIL/YY1-IL6/8 pathway enhances understanding of the etiology of CAD and provides potential therapeutic target for treatment of CAD.
Abbreviations
lncRNA | = | long non-coding RNA |
ANRIL | = | Antisense Noncoding RNA in the INK4 Locus |
CAD | = | coronary artery disease |
EC | = | endothelial cell |
HUVEC | = | human umbilical vein endothelial cell |
YY1 | = | Yin Yang 1 |
SNP | = | single nucleotide polymorphisms |
PRC | = | Polycomb repressive complex |
CHIP | = | Chromatin Immunoprecipitation |
RIP | = | RNA immunoprecipitation |
CHIRP | = | Chromatin Isolation by RNA Purification. |
Introduction
Large-scale sequencing of mammalian transcriptomes enables the discovery of a huge number of previously uncharacterized non-coding RNA molecules.Citation1,2 Long non-coding RNAs (lncRNAs) are arbitrarily defined as transcripts longer than 200 nucleotides, with no protein coding capacity.Citation3 Recently, functional lncRNAs are recognized as critical players of gene regulation in multiple biological processes such as cell growth, development, angiogenesis and inflammation.Citation4–7 LncRNAs are also associated with many human diseases such as cancers, Alzheimer disease and cardiovascular diseases.Citation3,8–10 The functional characterization of lncRNAs is however much more difficult than expected due to their poor conservation and complex mechanisms. The biological functions of lncRNAs associated with human diseases remain largely unrevealed.
ANRIL (Antisense Noncoding RNA in the INK4 Locus) is a 3.8k nt long non-coding RNA transcribed from chromosome 9p21, the best implicated genetic susceptibility locus for coronary artery disease (CAD). Citation11,12 ANRIL gene is transcribed and spliced in a complex pattern. Multiple isoforms of ANRIL have been identified Citation13,14 and they are mainly grouped into 2 categories including short forms terminating with exon 13 (short ANRIL isoforms, SANRIL) and long forms which lack exon 13 and terminate with exon 19 and exon 20 (long ANRIL isoforms, LANRIL). The chromosome 9p21 CAD risk haplotype containing multiple single nucleotide polymorphisms (SNP) in tight linkage disequilibrium resides at the 3′ end of ANRIL gene and exhibits a clear association with ANRIL expression. Citation15–19 Based on these genetics studies, ANRIL is currently considered as a prime candidate gene for the chromosome 9p21 CAD locus. Subsequently ANRIL is found to be associated with periodontitis, diabetes, open angle glaucoma and various cancers.Citation20 Previous studies in prostate tissue and fibroblast cells revealed that ANRIL epigenetically represses its neighboring CDKN2A/B genes in INK4 locus, which encode for cell cycle regulators p16INK4A and p15INK4B Citation21 by recruitment of Polycomb repressive complex 1 (PRC1) and PRC2 proteins to their genomic regions.Citation22,23 ANRIL has also been documented to bind with PRC-associated protein YY1,Citation24 which is a transcriptional factor and has been shown to induce or repress gene expression in inflammatory processes.Citation25,26,27 YY1 was proposed as an RNA-binding protein that bridges regulatory RNA and chromatin targets.Citation28 Nevertheless, little is known about how ANRIL is regulated in pathological process of CAD.
CAD is regarded as a progressive inflammatory process.Citation29 It arises from endothelial dysfunction in which distinct but overlapping pathways of pathogenesis are involved. In initial phase of CAD development, vascular endothelial cells (ECs) could sense pathological stimuli in blood and initiate atherosclerosis events by secreting inflammatory factors.Citation30 It is appealing to us to explore whether ANRIL plays a role in this initial inflammation phase in order to find clues for ANRIL contribution to CAD. In the present study, functions of ANRIL are explored in a pathological context in vascular ECs. We reveal that ANRIL is markedly induced by activation of NF-κB pathway, and elevated ANRIL show a regulatory effect on expression of inflammatory genes downstream of NF-κB. ANRIL forms a functional complex with transcriptional factor Yin Yang 1 (YY1) to exert transcriptional regulation on inflammatory genes IL6 and IL8. Our findings thus provide a mechanistic insight into how ANRIL functions in the intricate gene expression regulation network in inflammation process in vascular endothelium and extend the NF-κB pathway further into the long non-coding RNA area.
Results
ANRIL is markedly induced by pro-inflammatory factor TNF-α in ECs
Functions of ANRIL under physiological conditions in human umbilical vein endothelial cells (HUVECs) were explored by loss-of-function experiments. ANRIL was silenced (Fig. S1A) and expression of CDKN2A/B genes was assessed. RNA levels of p16INK4A and p15INK4B were up-regulated (Fig. S1B) and cell growth was arrested upon ANRIL knockdown (Fig. S1C and S1D). These results are in line with previous reports that ANRIL is required for regulation of cell proliferation. To explore the functions of ANRIL in vascular ECs under pathological conditions, we measured ANRIL expression in response to pro-inflammatory cytokine TNF-α and growth factors VEGF165, PDGF-BB by RT-qPCR in HUVECs. IFN-γ, a known cytokine that regulates ANRIL expression, Citation31 was incorporated as a control. No significant changes of ANRIL expression were found toward the potent proliferative growth factors (). ANRIL showed a remarkable increase (∼5 fold) by TNF-α stimulation, which was much higher than that by IFN-γ (). TNF-α induced up-regulation of ANRIL was further confirmed by the time- and dose-dependent effects (). In addition, both short (SANRIL, ) and long (LANRIL, ) ANRIL isoforms were upregulated by TNF-α in a similar time-dependent pattern, indicating a shared regulation mechanism by TNF-α for ANRIL isoforms. Moreover, ANRIL was identified in cell nucleus, and the nuclear ANRIL was significantly induced under TNF-α treatment (), while cytoplasmic 7S RNA (Fig. S2A) and nucleic U6 snRNA (Fig. S2B) showed no expression change upon TNF-α treatment. These results indicate that ANRIL is regulated by TNF-α signaling pathway in vascular ECs.
Figure 1. ANRIL expression is induced by pro-inflammatory factors in ECs. (A) RT-qPCR analysis of ANRIL expression in HUVECs treated with TNF-α (25 ng/mL), IFN-γ (100 ng/mL), PDGF-BB (10 ng/mL) or VEGF165 (50 ng/mL) for 24 hours. (**p < 0.01, ***p < 0.005, n.s., non-significant vs. control). (B) RT-qPCR showing that ANRIL levels were induced by TNF-α (25 ng/mL) in a time-dependent manner. (**p < 0.01, ***p < 0.005 vs. control). (C) RT-qPCR showing that ANRIL levels were induced by TNF-α (24h) in a dose-dependent manner. (*p < 0.05, **p < 0.01 vs. control). (D and E) Both short (D) and long (E) ANRIL isoforms were upregulated upon TNF-α treatment (25 ng/mL) at indicated time points by RT-qPCR. (**p < 0.01, ***p < 0.005 vs. control). (F) Cell fractionation and RT-qPCR analysis in HUVECs showing that ANRIL was mainly distributed in nucleus upon control or TNF-α (25 ng/mL) treatment. (*p < 0.05). All results are presented as mean ± SEM from at least 3 independent biological experiments.
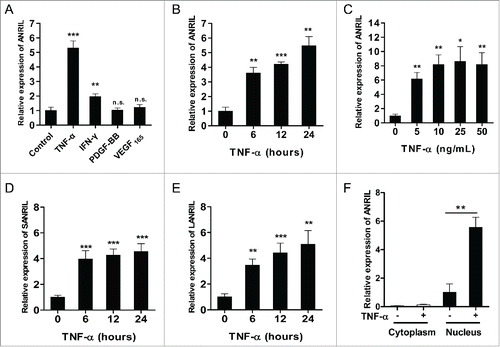
TNF-α induced ANRIL expression is mediated by NF-κB
In order to find out the regulatory mechanism for ANRIL induction by TNF-α, we analyzed ANRIL promoter sequence (1kb upstream of TSS) and found a putative NF-κB binding site (-656 to-665 relative to TSS) (). To test the hypothesis that NF-κB mediates TNF-α induced ANRIL expression, we silenced the key subunit of NF-κB, p65 by siRNAs (), and measured ANRIL expression in HUVECs with or without TNF-α treatment. It was found that ANRIL induction by TNF-α was attenuated when NF-κB was silenced by siRNAs (). The direct interaction between NF-κB and ANRIL promoter was determined by CHIP assay. IL8, a known NF-κB target, was taken as a positive control and GAPDH was taken as a negative control. An induced elevation of NF-κB binding to ANRIL promoter was shown upon TNF-α treatment (). Thus we concluded that NF-κB mediates ANRIL gene expression induction upon TNF-α activation.
Figure 2. TNF-α induced ANRIL expression is mediated by NF-κB activation. (A) Schematic representation of ANRIL promoter region and the predicted NF-κB binding site. TSS means transcriptional start site. (B and C) NF-κB p65 (B) and ANRIL (C) RNA levels in HUVECs transfected with scramble or 2 sets of NF-κB p65 siRNAs (sip65–1 and sip65–2, 24h) upon TNF-α treatment (25 ng/mL, 12h). (*p < 0.05, **p < 0.01, ***p < 0.005). (D) CHIP analysis showing the increased binding of p65 to ANRIL promoter in HUVECs upon TNF-α treatment (25 ng/mL, 6h). Binding of p65 to IL8 promoter was used as a positive control, and binding of p65 to GAPDH promoter was a negative control. (*p < 0.05, n.s. means non-significant). (E) ANRIL levels in HUVECs treated with TNF-α (25 ng/mL), IL-1β (10 ng/mL), LPS (1 μg/mL), IFN-γ (100 ng/mL), EGF (10 ng/mL), PDGF-BB (10 ng/mL), VEGF165 (50 ng/mL) and insulin (100 nmol/L) for 24 hours. (*p < 0.05, ***p < 0.005 vs. control). (F) Representative immunoblot showing the p65 phosphorylation (ser536) in HUVECs treated with factors mentioned in (E) for 30 min. β-tubulin was used as an internal control. (G) Histogram showing pooled quantification data of p65 phosphorylation from immunoblot experiments in (F) (*p < 0.05 vs. control). All results are presented as mean ± SEM from at least 3 independent biological experiments.
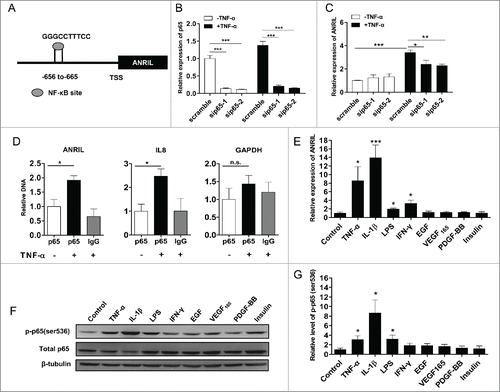
In addition to TNF-α, NF-κB is activated by series of other pathological factors including cytokines and LPS. To determine the specificity of ANRIL induction by NF-κB, we further examined ANRIL expression in HUVECs, which were treated with NF-κB activators including TNF-α, IL-1β and LPS, by RT-qPCR. Growth factors and insulin, which are unrelated with NF-κB activation, were used as controls (). IL-1β was found to induce ANRIL expression dramatically (∼13.9 fold). A moderate but significant elevation of the expression of ANRIL was also seen in LPS treated cells (∼1.97 fold). In contrast, ANRIL expression was not affected by growth factors and insulin. Meanwhile, NF-κB activation was determined by western-blot using phosphorylated p65 (ser536) antibody () and the relative p65 phosphorylation (ser536) was quantified by scanning the bands (). A significant positive correlation (r = 0.9165, p < 0.005) was found between NF-κB activation in and ANRIL expression in . It is observed that the response of ANRIL to IFN-γ does not depend on NF-κB activation. These data provide supportive evidence that ANRIL expression depends on NF-κB activation under pathological context.
ANRIL is required to regulate inflammatory genes downstream of TNF-α
Given that ANRIL is a known regulatory lncRNA and a novel target of NF-κB signaling, we wondered whether ANRIL executes its regulatory effects on other inflammation-associated genes downstream of NF-κB signaling. ANRIL has multiple isoforms, and we decided to explore the overall functions of ANRIL isoforms in vascular inflammation. To determine this, we transfected HUVECs with scramble or ANRIL siRNA targeting exon 1, which is a common exon shared by all known ANRIL isoforms. HUVECs were then treated with TNF-α and high throughput RNA sequencing was performed. Differential gene expression analysis revealed that 1026 genes were affected by ANRIL knockdown in which 473 genes were up-regulated and 553 genes were down-regulated (fold change > 2, p < 0.001) (Table S1). Three hundred and 7 genes were mapped to KEGG pathways and results revealed that a large portion of ANRIL regulated genes (67 genes) were enriched in the inflammation related pathways including cytokine-cytokine receptor signaling, Toll like receptor pathway, cell adhesion molecules and ECM-receptor interaction (p < 0.05) (, Table S2). IL6 and IL8, the most well-established pro-inflammatory factors triggered by TNF-α, were selected for further validation. We used 2 siRNAs separately targeting exon 1 and exon 6, both of which are common exons shared by all known isoforms of ANRIL, for RT-qPCR and ELISA experiments. Results confirmed that when ANRIL was knocked down (), TNF-α induced expression of IL6 and IL8 were significantly suppressed at RNA () and protein levels (). To further ascertain the effects of ANRIL on endothelial inflammation in response to TNF-α, the monocyte adhesion assay was carried out. It was found that TNF-α treatment increased the number of U937 cells bound to HUVECs, while ANRIL knockdown suppressed TNF-α induced binding of U937 cells to HUVECs (). ANRIL isoforms were silenced by specific siRNA targeting exon 13 and exon 19 separately. Results showed that silencing the short () and long () ANRIL isoforms inhibited IL6 () and IL8 () expression levels under TNF-α. Moreover, we investigated the role of ANRIL in human coronary endothelial cells (HCAECs) to validate the association between TNF-α/ANRIL/IL-6 we found in HUVECs. Upon TNF-α treatment, ANRIL expression was significantly upregulated in HCAECs (Fig. S3A). Knockdown of overall ANRIL isoforms (Fig. S3B) inhibited TNF-α induced IL6 and IL8 expression (Fig. S3C and S3D), which is consistent with the findings in HUVECs. Knockdown of the short (Fig. S3E) and long (Fig. S3F) ANRIL isoforms also inhibited IL6 (Fig. S3G) and IL8 (Fig. S3H) expression levels induced by TNF-α. These consistent data indicate that the ANRIL is involved in TNF-α-NF-κB signaling to regulate inflammatory response in endothelial cells.
Figure 3. ANRIL regulates inflammatory genes expression downstream of TNF-α signaling pathway. (A) KEGG pathway analysis (DAVID Functional Annotation Bioinformatics Microarray Analysis) of differentially expressed genes in RNA sequencing data from HUVECs treated with scramble or ANRIL siRNA (24h) upon TNF-α treatment (25 ng/mL, 24h). (p < 0.05). (B) RT-qPCR analysis showing that ANRIL was significantly knocked down by 2 sets of siRNAs (siANRIL-1 and siANRIL-2, 24h) targeting common sequences of ANRIL isoforms upon TNF-α treatment (25 ng/mL, 24h). (*p < 0.05). (C and D) RT-qPCR (C) and ELISA assay (D) of IL6 and IL8 levels in HUVECs treated with scramble or 2 sets of ANRIL siRNAs (siANRIL-1 and siANRIL-2, 24h) upon TNF-α treatment (25 ng/mL, 24h). (*p < 0.05, **p < 0.01, ***p < 0.005). (E) Monocyte adhesion assay showing that ANRIL knockdown decreased the adhesion of U937 monocytes to HUVECs. HUVECs were treated with scramble or 2 sets of ANRIL siRNAs (siANRIL-1 and siANRIL-2). Twenty-four hours later, HUVECs were treated with TNF-α (25 ng/mL) for 6 h and incubated with U937 cells for another 1 h. After washing, attached U937 cells were photographed (×200) and quantified (right panel). (*p < 0.05, **p < 0.01). (F-I) RT-qPCR of RNA levels of short (SANRIL) (F), long (LANRIL) (G) ANRIL isoforms and IL6 (H), IL8 (I) in HUVECs treated with scramble or specific siRNAs (siSANRIL and siLANRIL, 24h) upon TNF-α treatment (25 ng/mL, 24h). (*p < 0.05, **p < 0.01, ***p < 0.005). All results are presented as mean ± SEM from at least 3 independent biological experiments.
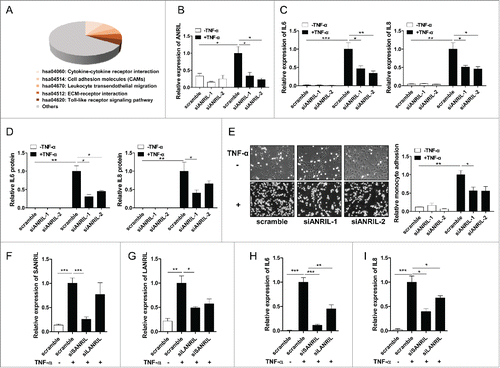
ANRIL interacts with transcriptional factor YY1 and is required for YY1 binding to IL6 and IL8 promoter loci
Publicly available CHIP-sequencing dataCitation32–34 showed YY1 binding signals in promoter loci of the majority of ANRIL regulated inflammatory genes (50 out of 67) including IL6 and IL8 (, Table S3). YY1 was shown to be an RNA-binding protein mediating RNA and chromatin regions of target genes. We thereby hypothesized that YY1 is the responsible factor to mediate ANRIL regulation on IL6 and IL8. To test this, we silenced YY1 with 2 sets of siRNAs (). Results showed that both IL6 and IL8 genes expression under TNF-α was repressed by YY1 knockdown (). Meanwhile, ANRIL expression was not affected by YY1 knockdown (Fig. S4). These data indicates YY1 is essential for TNF-α induction of IL6 and IL8 genes expression.
Figure 4. ANRIL binds with YY1 to cooperatively activate IL6 and IL8 expression upon TNF-α treatment. (A) YY1 binding sites in promoter regions of human IL6 and IL8 genes according to UCSC Genome Browser (http://genome.ucsc.edu). TSS means transcriptional start site. (B and C) YY1 (B) and IL6, IL8 (C) levels in HUVECs transfected with scramble or 2 sets of YY1 siRNAs (siYY1–1 and siYY1–2, 24h) upon TNF-α treatment (25 ng/mL, 24h). (*p < 0.05, **p < 0.01, ***p < 0.005). (D) RIP analysis showing YY1 binding with ANRIL upon TNF-α treatment (25 ng/mL, 6h). GAPDH RNA was used as a control. (*p < 0.05, n.s. means non-significant). (E) CHIP assay showing that ANRIL knockdown by siRNA transfection (24h) impaired YY1 binding to IL6, IL8 and CXCR4 promoter regions upon TNF-α treatment (25 ng/mL, 6h). GAPDH DNA was used as a control. (*p < 0.05, **p < 0.01, n.s. means non-significant). (F) CHIRP assay showing that ANRIL bound to IL6, IL8 and CXCR4 promoter regions upon TNF-α treatment (25 ng/mL, 6h). ANRIL probes were used and NC probe was used as negative probe control. TERC DNA was used as a control. (*p < 0.05, n.s. means non-significant). All results are presented as mean ± SEM from at least 3 independent biological experiments.
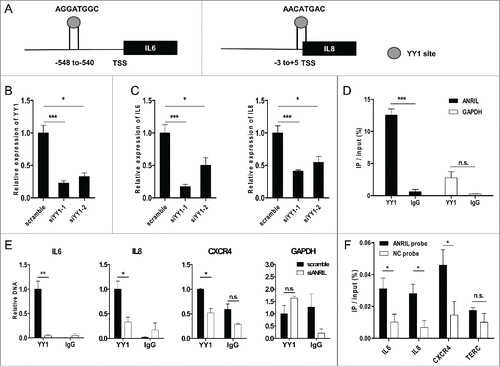
To elucidate the molecular mechanisms, we performed immunoprecipitation assays to find out whether ANRIL directly interacts with YY1 to cooperatively target and activate IL6 and IL8 expression in TNF-α activated endothelial cells. Initially, RNA-immunoprecipitation (RIP) analysis demonstrated the binding between ANRIL and YY1 in TNF-α activated HUVECs (). Next, YY1 was found to be enriched in promoter loci of IL6 and IL8 genes in scramble siRNA treated HUVECs, as confirmed by chromatin-immunoprecipitation (CHIP) assay (). CXCR4, which is known to be suppressed by YY1 through directly binding to its promoter, Citation25,35 is incorporated as a positive control (). ANRIL knockdown led to decreased binding between YY1 and the promoters of IL6 and IL8 (). YY1 binding to CXCR4 promoter was also significantly impaired upon ANRIL knockdown (), indicating that ANRIL silencing may increase CXCR4 expression, which is consistent with the RNA-seq results that ANRIL knockdown caused increased (∼1.7 fold) CXCR4 expression. These results indicate that YY1 regulates inflammatory genes expression by binding to their promoters and ANRIL facilitates such binding. Moreover, chromatin isolation by RNA purification (CHIRP) with ANRIL probes (Fig. S5) was employed to examine the binding of ANRIL to genomic regions of target genes. Results showed a direct binding of ANRIL to the promoter loci of IL6 and IL8 (). ANRIL probe also enriched more CXCR4 promoter than the NC probe (). Taken together, our findings show that ANRIL forms a functional complex with YY1 to cooperatively activate IL6 and IL8 expression by binding to their promoter loci.
ANRIL associates with TNF-α/IL6/IL8 in CAD patients
To determine the clinical significance of inflammation associated TNF-α-ANRIL-IL6/IL8 axis in vivo, we measured serum TNF-α, IL6 and IL8 levels in 61 CAD patients and attempted to search for their correlations. ANRIL, IL6 and IL8 expression levels in PBMCs from CAD patients were also monitored by RT-qPCR. Serum TNF-α is detectable in 28 patients with an average level of 6.53 pg/mL, but below the limit of detection in the other 33 patients. Serum TNF-α did not have a significant correlation with ANRIL in PBMCs from the 28 patients. Similarly, significant correlations between TNF-α in serum and IL6/8 expressions in PBMCs were not observed. Within such a background, we divided the CAD patients into “High TNF-α” and “Low TNF-α” groups according to the detection threshold and compared ANRIL, IL6 or IL8 levels between the 2 groups. Patients´ information was listed in . ANRIL () expression in PBMCs and IL6 () or IL8 () protein levels in serum were significantly higher in the High TNF-α group, which is consistent with that TNF-α induces ANRIL. In addition, we analyzed the correlations between ANRIL and IL6/IL8 expression in PBMCs from the 61 CAD patients. ANRIL expression exhibited a significant correlation with IL6 (, r = 0.3955, p < 0.05) and IL8 (, r = 0.3412, p < 0.05), supporting that ANRIL-IL6/IL8 axis exists under CAD pathological context. Collectively, the association between ANRIL and IL6/IL8 in CAD patients provides supportive evidence for ANRIL functions in inflammation.
Figure 5. ANRIL, IL6 and IL8 levels are increased in CAD patients with high level of serum TNF-α. (A) RT-qPCR showing ANRIL levels in PBMCs from 2 groups of CAD patients. (*p < 0.05). (B and C) Luminex-detection showing serum IL6 (B) and IL8 (C) levels in 2 groups of CAD patients. (*p < 0.05, **p < 0.01). (D and E) Scatter plots showing the correlation between ANRIL and IL6 (r=0.3955, p < 0.05) (D), ANRIL and IL8 (r=0.3412, p < 0.05) (E) in PBMCs in the enrolled patients (n=61). Linear regression line for the association is shown as a solid line with the 95% confidence intervals shown as dotted lines.
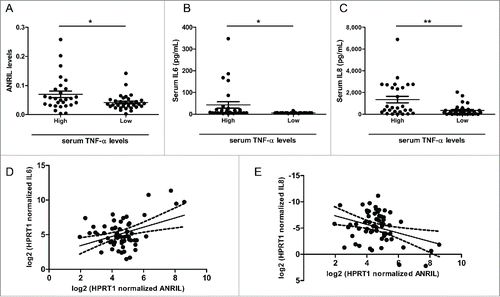
Table 1. Clinical characteristics of the CAD patients.
Discussion
ANRIL is a well-known functional lncRNA associated with multiple human diseases, especially CAD. It is proposed that ANRIL is involved in the proliferation process by epigenetic regulation on neighboring CDKN2A/B genes. Citation22,23 There have been multiple reports linking CAD to inflammation.Citation29,36,37 In our study, we showed that under pathological conditions, ANRIL was significantly induced upon inflammatory factors and regulate genes expression downstream of NF-kB signaling. ANRIL appeared to be the regulatory lncRNA that links the 2 pathological processes together. A working model for the function and mechanism of ANRIL in regulating inflammatory responses was shown as .
Figure 6. A working model for ANRIL to regulate inflammation responses in human vascular ECs. Under pathological conditions, inflammatory stimuli such as TNF-α triggers NF-κB activation, which induces ANRIL transcription. Then ANRIL interacts with transcriptional factor YY1 to form a functional complex, cooperatively binds to and regulates expression of target genes.
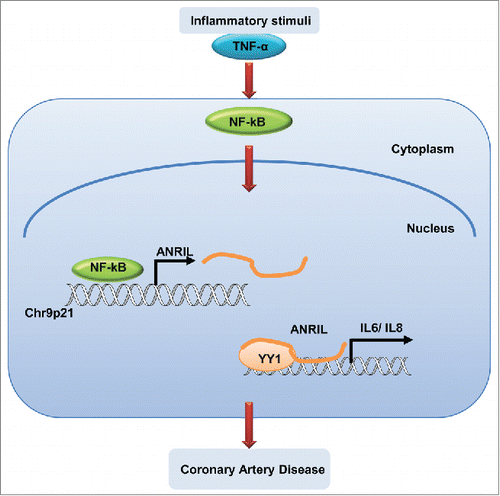
As an emerging group of regulatory molecules, lncRNAs have recently been indicated as the critical layer in modulating human immune response.Citation38,39 NF-κB pathway is the central mediator and a key participant of the inflammatory processes. Citation40 NF-κB is also a converged molecule acting as both a responder and regulator of the inflammatory factors linked to endothelial activation and atherosclerosis.Citation41,42 Recently characterized lncRNAs including Lethe and lincRNA-Cox2 are all linked to regulation of NF-κB activity Citation43,44 and manipulation of inflammatory signaling. Data presented in this study explicitly demonstrate that ANRIL is not only a novel downstream target of NF-κB in vascular ECs, but also a modulator of other genes downstream of NF-κB in addition to other known components of this pathway.
We further demonstrated that ANRIL exerted this regulatory role by interaction with an RNA binding protein. YY1, which is a multifunctional transcriptional factor with both inhibitory and activating effects, is capable of binding both RNA and DNA through different motifs, bridging the interaction between lncRNA and chromosome.Citation28 YY1 exhibits cell type and context-dependent regulatory propertiesCitation45 and is also found to be involved in inflammatory processes by activating COX2 and interleukin 4 genes in immune cells.Citation26,27 Moreover, YY1 activity is shown to be regulated by NF-κB in skeletal myogenesis and cancers.Citation46–48 Existing evidence already suggested that YY1 might bind ANRIL in cells.Citation24 In this work we demonstrated the biological implications of the binding between ANRIL and YY1 in the control of inflammatory genes downstream of NF-κB. Tagging to proteins appeared to be a typical mode of action for lncRNAs in gene regulation. For instance, THRIL from activated human macrophages is required for TNF-α expression by binding with hnRNPL toCitation7 LincRNA-Cox2 identified in TLR signaling modulates both the activation and repression of immune response genes through its interaction with hnRNPA/B and A2/B1.Citation44
During CAD pathogenesis, a wide range of chemokines, cytokines, and growth factors are produced in different stages of the process, triggering pathological inflammation events.Citation49 ANRIL is found to be a novel member of TNF-α-NF-κB pathway to activate inflammatory factors under pathological stresses. For the first time, we provided the clinical evidence that ANRIL level is associated with IL6/IL8 levels in blood, which further confirmed the pathological role of ANRIL relevant to CAD etiology.Citation50 Among the patients with detectable TNF-α, the significant correlation between TNF-α in serum and ANRIL/IL6/IL8 in PBMCs was not observed, in contrary to the grouping approach we described in . We reasoned that ANRIL/IL6/IL8 expression in PBMCs might be subjected to regulation by multiple serum factors in addition to TNF-α, thus the expression change could be consequential of these mixed stimulatory factors in serum. We also found that ANRIL levels in PBMCs did not have significant correlations with serum IL6/IL8 levels although such a correlation is indeed observed within the PBMC cells. Since PBMCs might not be the only source of the serum IL6/IL8,Citation51–53 thus it is reasonable that serum levels of these 2 cytokines may not well reflect changes of ANRIL in PBMCs. The ANRIL modulated fluctuation of IL6/IL8 might actually become functionally relevant either within these cells, or when the PMBCs are present in certain more enclosed spaces where PMBCs become the major source of IL6/IL8.
In summary, our data demonstrate that under pathological stresses, ANRIL is activated by NF-κB pathway and is required for the expression of a subset of pro-inflammatory genes controlled by NF-κB. It should be further realized that besides CAD, other human diseases associated with Chr9p21 including cancers and periodontitis are also complex chronic immune disorders with aberrated NF-κB signalingCitation54 and the current finding might shed light on the illustration of ANRIL/NF-κB function in these diseases as well. Since blocking NF-κB signaling in endothelial cells has been shown to significantly protect against atherosclerosis,Citation42 ANRIL as a downstream modulator of NF-κB might be explored to establish whether it can act as a more specific target for drug development.
Methods
Reagents
Recombinant human TNF-α (300–01A), IFN-γ (300–02), IL-1β (200–01B), EGF (AF-100–15), VEGF165 (100–20) and PDGF-BB (100–14B) were from Peprotech. LPS and insulin were from Sigma-Aldrich. RNA oligonucleotides were from GenePharma and RiboBio. DNA oligonucleotides were from Life Technologies. Anti-NF-κB p65 antibody (ab7970), YY1 antibody (ab12132) and normal rabbit IgG (ab46540) were from Abcam Biotechnology. Antibody to phospho-p65 (Ser536) (AN371) was from Beyotime. Antibody to β-tubulin was from EASYBIO.
Cell culture
HUVECs and HCAECs were cultured in Endothelial Cell Medium (ScienCell) supplemented with 5% (vol/vol) FBS, 1% Endothelial Cell Growth Supplement and 1% penicillin/streptomycin. HUVECs and HCAECs within 3–7 passages were used in this study.
RNA isolation and RT-PCR analysis
Total RNA was isolated with TRIzol Reagent (Invitrogen) and then used for cDNA synthesis with TransScript® Reverse Transcriptase (TransGen) and HiScript® II Q RT SuperMix for qPCR (+gDNA wiper) (Vazyme). Quantitative PCR was performed to measure RNA levels of target genes by using SYBR Green I dye (Invitrogen). Glyceraldehyde 3-phosphate dehydrogenase (GAPDH) was used as an internal control. PCR primers were listed in Table S4.
For ANRIL, IL6 and IL8 expression measurement in PBMCs from CAD samples, Hypoxanthine phosphoribosyl-transferase 1 (HPRT1) was used as an internal control according to the reference.Citation55
For nuclear and cytoplasmic fraction isolation, PARIS™ kit (Ambion) was used according to the manufacturer´s instructions.
SiRNA transfection
The siRNA sequences were listed in Table S4. Sequence of siANRIL-1 was from previous report,Citation23 and siANRIL-2 was from siRNA screening. SiRNAs were transfected into HUVECs and HCAECs using lipofectamine™ 2000 (Invitrogen) at final concentration of 70nM. SiRNAs targeting NF-κB p65 and YY1 were used at final concentration of 50nM.
RNA sequencing
HUVECs treated with scramble or ANRIL targeting siRNA were serum-starved for 12 hours, followed with 25 ng/mL TNF-α stimulation for 24h before total RNA was isolated. RNA was extracted, sent for library preparation and sequencing on the Illumina HiSeq2000 by Biopic (Peking University). Between 47.1 and 40.5 million reads per sample were generated with a unique mapping rate of more than 85%. Differential gene expression was calculated by DEGseq.Citation56 P-values were determined using Fisher's exact tests implemented in the software. P-values are adjusted to Q-values for multiple testing corrections by 2 alternative strategies.Citation57,58
Monocyte adhesion assay
Monocyte adhesion assay was carried out as described previously with some modifications.Citation59 HUVECs in 12-well plates were treated with scramble or ANRIL siRNA for 24 h, followed by incubation with or without TNF-α (25 ng/mL) for another 6 h. U937 cells were then added for 1 h. Unbound cells were removed by gentle washing, and the number of U937 cells attached to HUVECs was counted under a phase-contrast microscope (Olympus).
CHIP analysis
HUVECs treated with or without TNF-α (25 ng/mL) for 6 hours were collected. Chromatins were then formaldehyde cross-linked and sonicated to ∼200–1000 bp using the sonicator (SONICS Uibra Cell) for 8 sets of 10-second pulses on wet ice. Total of 25–30 µg chromatin was immunoprecipitated with p65 (4µg) antibody, YY1 antibody (2ug) or equal amount of normal rabbit IgG followed by incubation with protein A-sepharose/salmon sperm DNA. DNA was isolated from immunoprecipitated chromatin by chelex-100 based method, and used as templates for quantitative PCR analysis. Citation60 Primers for CHIP were listed in Table S4.
RNA immunoprecipitation
RNA immunoprecipitation (RIP) assays were carried out as described previously Citation61,62 with some modifications. HUVECs were treated with TNF-α (25 ng/mL) for 6 hours and then harvested by trypsinization and resuspended in 2 mL PBS, 2mL nuclear isolation buffer (1.28 M sucrose; 40 mM Tris-HCl ph 7.5; 20 mM MgCl2; 4% Triton X-100) and 6 mL water on ice for 20 min. Pelleted Nuclei were resuspended in 1 mL RIP buffer [150 mM KCl, 25 mM Tris pH 7.4, 5 mM EDTA, 0.5 mM DTT, 0.5% NP40, 100 U/mL RNasin (Tiangen), protease inhibitor cocktail] and lysed by sonication. Nuclear membrane and debris were pelleted by centrifugation at 16,000 g for 10 min. Antibody to rabbit IgG and YY1 (Abcam) were added to supernatant (3 μg each) and incubated overnight at 4°C with gentle rotation. Protein A beads were added for additional 1 hour incubation. The beads are washed 5 times with 1 mL of highstringency RIPA buffer (50 mM Tris-Cl, pH 7.5, 1% NP-40, 1% sodium deoxycholate, 0.1% sodium dodecyl sulfate (SDS), 1 mM EDTA, 1 M NaCl, 1–4 M urea, and 0.2 mM phenylmethylsulfonyl fluoride (PMSF) by 10 min rotation at room temperature. The beads containing the immunoprecipitated samples are collected and resuspended in 100 uL of 50 mM Tris-Cl, pH 7.0, 5 mM EDTA, 10 mM dithiothreitol (DTT) and 1% SDS and incubated at 70°C for 45 min to reverse the crosslinks. Co-precipitated RNAs were isolated by phenol-chloroform and RT-qPCR for GAPDH or ANRIL were performed. Primers for RIP were listed in Table S4.
Chromatin Isolation by RNA Purification
Chromatin Isolation by RNA Purification (CHRIP) assays were carried out and ANRIL probes were designed according to the protocolsCitation63,64 with some modifications. NC probe was from previous report.Citation65 Probe oligos were 5´-biotinylated. HUVECs were treated with TNF-α (25 ng/mL) for 6 hours and then harvested by trypsinization. Cells were cross-linked with 2 mM DSG at room temperature for 45 minutes and 3% formaldehyde at 37°C for additional 10 min.Citation64 Chromatins were sonicated to ∼100–500 bp and hybridized with ANRIL or NC probes. DNA fraction was isolated by phenol-chloroform from ChIRP samples and subjected to quantitative PCR analysis. Primers for CHIRP and probe sequences were listed in Table S4.
ELISA assay and western blot
Culture medium from different groups was collected and protein levels of IL6 and IL8 were determined by ELISA kits under the instructions of the manufacturer (kit. No. 12-1060-096 and 12-1080-096, DAKEWE).
Total proteins were extracted by cell lysis buffer (50 mM Tris HCl, pH 7.4, 150 mM NaCl, 1 mM EDTA, 1% TRITON X-100), and equal amounts of protein from each lysate was separated by SDS-PAGE and transferred onto a nitrocellulose filter membrane (Millipore). The membrane was blocked and incubated at 4°C overnight with primary antibodies described above. Then the membrane was washed and incubated at room temperature with secondary antibodies. Proteins were detected by ECL kit (CWBIO) and visualized on an imaging system (Bio-Rad).
Study subjects
Sixty and one CAD patients were recruited at the Affiliated Hospital of Jining Medical University, Shandong province, China. Blood sample (2–3ml) was obtained from each individual. Peripheral blood cells were isolated for ANRIL/IL6/IL8 detection and serum was used for cytokine measurement. The study has received ethical approval by the local hospital ethics committees, which is in accordance with the Helsinki Declaration. All the subjects gave their written and informed consent before accepting study participation.
Serological detection
The levels of TNF-α, IL6 and IL8 in serum were measured by using Luminex-detection technology (Beijing 4A Biotech Co., Ltd) according to manufacture protocol.
Statistical analysis
All data are processed using GraphPad Prism 5.0. Data are expressed as the means ± standard error (SEM). Pairwise comparison of means was performed using Student´s t-test. The Pearson correlation coefficient (Pearson´s r) was used to evaluate the correlation of the 2 variables. P value < 0.05 was considered to be statistically significant (*p < 0.05, **p < 0.01, ***p < 0.005).
Disclosure of potential conflicts of interest
No potential conflict of interest were disclosed
KRNB_A_1122164_Supplementary_Materials.doc
Download MS Word (1.6 MB)Acknowledgments
This study was supported by the National Natural Science Foundation of China (81570327 and 81273422). The work received financial support from the Sahlgrenska University Hospital research fund (ALF/LUA) and unrestricted research grant from AstraZeneca R&D.
References
- Griffiths-Jones S. Annotating noncoding RNA genes. Annu Rev Genomics Hum Genet 2007; 8:279–98; PMID:17506659; http://dx.doi.org/10.1146/annurev.genom.8.080706.092419
- Kapranov P, Cheng J, Dike S, Nix DA, Duttagupta R, Willingham AT, Stadler PF, Hertel J, Hackermuller J, Hofacker IL, et al. RNA maps reveal new RNA classes and a possible function for pervasive transcription. Science 2007; 316:1484–8; PMID:17510325; http://dx.doi.org/10.1126/science.1138341
- Yang L, Froberg JE, Lee JT. Long noncoding RNAs: fresh perspectives into the RNA world. Trends Biochem Sci 2014; 39:35–43; PMID:24290031; http://dx.doi.org/10.1016/j.tibs.2013.10.002
- Ghazal S, McKinnon B, Zhou J, Mueller M, Men Y, Yang L, Mueller M, Flannery C, Huang Y, Taylor HS. H19 lncRNA alters stromal cell growth via IGF signaling in the endometrium of women with endometriosis. EMBO Mol Med 2015; 7:996–1003; PMID:26089099; http://dx.doi.org/10.15252/emmm.201505245
- Michalik KM, You X, Manavski Y, Doddaballapur A, Zornig M, Braun T, John D, Ponomareva Y, Chen W, Uchida S, et al. Long noncoding RNA MALAT1 regulates endothelial cell function and vessel growth. Circ Res 2014; 114:1389–97; PMID:24602777; http://dx.doi.org/10.1161/CIRCRESAHA.114.303265
- Ng SY, Lin L, Soh BS, Stanton LW. Long noncoding RNAs in development and disease of the central nervous system. Trends Genet 2013; 29:461–8; PMID:23562612; http://dx.doi.org/10.1016/j.tig.2013.03.002
- Li Z, Chao TC, Chang KY, Lin N, Patil VS, Shimizu C, Head SR, Burns JC, Rana TM. The long noncoding RNA THRIL regulates TNFalpha expression through its interaction with hnRNPL. Proc Natl Acad Sci U S A 2014; 111:1002–7; PMID:24371310; http://dx.doi.org/10.1073/pnas.1313768111
- Wapinski O, Chang HY. Long noncoding RNAs and human disease. Trends Cell Biol 2011; 21:354–61; PMID:21550244; http://dx.doi.org/10.1016/j.tcb.2011.04.001
- Kataoka M, Wang DZ. Non-Coding RNAs Including miRNAs and lncRNAs in Cardiovascular Biology and Disease. Cells 2014; 3:883–98; PMID:25153164; http://dx.doi.org/10.3390/cells3030883
- Wilusz JE, Sunwoo H, Spector DL. Long noncoding RNAs: functional surprises from the RNA world. Genes Dev 2009; 23:1494–504; PMID:19571179; http://dx.doi.org/10.1101/gad.1800909
- McPherson R, Pertsemlidis A, Kavaslar N, Stewart A, Roberts R, Cox DR, Hinds DA, Pennacchio LA, Tybjaerg-Hansen A, Folsom AR, et al. A common allele on chromosome 9 associated with coronary heart disease. Science 2007; 316:1488–91; PMID:17478681; http://dx.doi.org/10.1126/science.1142447
- Helgadottir A, Thorleifsson G, Manolescu A, Gretarsdottir S, Blondal T, Jonasdottir A, Sigurdsson A, Baker A, Palsson A, Masson G, et al. A common variant on chromosome 9p21 affects the risk of myocardial infarction. Science 2007; 316:1491–3; PMID:17478679; http://dx.doi.org/10.1126/science.1142842
- Folkersen L, Kyriakou T, Goel A, Peden J, Malarstig A, Paulsson-Berne G, Hamsten A, Hugh W, Franco-Cereceda A, Gabrielsen A, et al. Relationship between CAD risk genotype in the chromosome 9p21 locus and gene expression. Identification of eight new ANRIL splice variants. PLoS One 2009; 4:e7677; PMID:19888323; http://dx.doi.org/10.1371/journal.pone.0007677
- Burd CE, Jeck WR, Liu Y, Sanoff HK, Wang Z, Sharpless NE. Expression of linear and novel circular forms of an INK4/ARF-associated non-coding RNA correlates with atherosclerosis risk. PLoS genetics 2010; 6:e1001233; PMID:21151960; http://dx.doi.org/10.1371/journal.pgen.1001233
- Broadbent HM, Peden JF, Lorkowski S, Goel A, Ongen H, Green F, Clarke R, Collins R, Franzosi MG, Tognoni G, et al. Susceptibility to coronary artery disease and diabetes is encoded by distinct, tightly linked SNPs in the ANRIL locus on chromosome 9p. Hum Mol Genet 2008; 17:806–14; PMID:18048406; http://dx.doi.org/10.1093/hmg/ddm352
- Jarinova O, Stewart AF, Roberts R, Wells G, Lau P, Naing T, Buerki C, McLean BW, Cook RC, Parker JS, et al. Functional analysis of the chromosome 9p21.3 coronary artery disease risk locus. Arterioscler Thromb Vasc Biol 2009; 29:1671–7; PMID:19592466; http://dx.doi.org/10.1161/ATVBAHA.109.189522
- Liu Y, Sanoff HK, Cho H, Burd CE, Torrice C, Mohlke KL, Ibrahim JG, Thomas NE, Sharpless NE. INK4/ARF transcript expression is associated with chromosome 9p21 variants linked to atherosclerosis. PLoS One 2009; 4:e5027; PMID:19343170; http://dx.doi.org/10.1371/journal.pone.0005027
- Holdt LM, Beutner F, Scholz M, Gielen S, Gabel G, Bergert H, Schuler G, Thiery J, Teupser D. ANRIL expression is associated with atherosclerosis risk at chromosome 9p21. Arterioscler Thromb Vasc Biol 2010; 30:620–7; PMID:20056914; http://dx.doi.org/10.1161/ATVBAHA.109.196832
- Cunnington MS, Santibanez Koref M, Mayosi BM, Burn J, Keavney B. Chromosome 9p21 SNPs Associated with Multiple Disease Phenotypes Correlate with ANRIL Expression. PLoS genetics 2010; 6:e1000899; PMID:20386740; http://dx.doi.org/10.1371/journal.pgen.1000899
- Congrains A, Kamide K, Ohishi M, Rakugi H. ANRIL: Molecular Mechanisms and Implications in Human Health. Int J Mol Sci 2013; 14:1278–92; PMID:23306151; http://dx.doi.org/10.3390/ijms14011278
- Roussel MF. The INK4 family of cell cycle inhibitors in cancer. Oncogene 1999; 18:5311–7; PMID:10498883; http://dx.doi.org/10.1038/sj.onc.1202998
- Yap KL, Li S, Munoz-Cabello AM, Raguz S, Zeng L, Mujtaba S, Gil J, Walsh MJ, Zhou MM. Molecular interplay of the noncoding RNA ANRIL and methylated histone H3 lysine 27 by polycomb CBX7 in transcriptional silencing of INK4a. Mol Cell 2010; 38:662–74; PMID:20541999; http://dx.doi.org/10.1016/j.molcel.2010.03.021
- Kotake Y, Nakagawa T, Kitagawa K, Suzuki S, Liu N, Kitagawa M, Xiong Y. Long non-coding RNA ANRIL is required for the PRC2 recruitment to and silencing of p15(INK4B) tumor suppressor gene. Oncogene 2011; 30:1956–62; PMID:21151178; http://dx.doi.org/10.1038/onc.2010.568
- Holdt LM, Hoffmann S, Sass K, Langenberger D, Scholz M, Krohn K, Finstermeier K, Stahringer A, Wilfert W, Beutner F, et al. Alu elements in ANRIL non-coding RNA at chromosome 9p21 modulate atherogenic cell functions through trans-regulation of gene networks. PLoS genetics 2013; 9:e1003588; PMID:23861667; http://dx.doi.org/10.1371/journal.pgen.1003588
- Hasegawa A, Yasukawa M, Sakai I, Fujita S. Transcriptional down-regulation of CXC chemokine receptor 4 induced by impaired association of transcription regulator YY1 with c-Myc in human herpesvirus 6-infected cells. J Immunol 2001; 166:1125–31; PMID:11145693; http://dx.doi.org/10.4049/jimmunol.166.2.1125
- Joo M, Wright JG, Hu NN, Sadikot RT, Park GY, Blackwell TS, Christman JW. Yin Yang 1 enhances cyclooxygenase-2 gene expression in macrophages. Am J Physiol Lung Cell Mol Physiol 2007; 292:L1219–26; PMID:17220375; http://dx.doi.org/10.1152/ajplung.00474.2006
- Guo J, Casolaro V, Seto E, Yang WM, Chang C, Seminario MC, Keen J, Georas SN. Yin-Yang 1 activates interleukin-4 gene expression in T cells. J Biol Chem 2001; 276:48871–8; PMID:11687571; http://dx.doi.org/10.1074/jbc.M101592200
- Jeon Y, Lee JT. YY1 tethers Xist RNA to the inactive X nucleation center. Cell 2011; 146:119–33; PMID:21729784; http://dx.doi.org/10.1016/j.cell.2011.06.026
- Gutierrez E, Flammer AJ, Lerman LO, Elizaga J, Lerman A, Fernandez-Aviles F. Endothelial dysfunction over the course of coronary artery disease. Eur Heart J 2013; 34:3175–81; PMID:24014385; http://dx.doi.org/10.1093/eurheartj/eht351
- Pate M, Damarla V, Chi DS, Negi S, Krishnaswamy G. Endothelial cell biology: role in the inflammatory response. Adv Clin Chem 2010; 52:109–30; PMID:21275341; http://dx.doi.org/10.1016/S0065-2423(10)52004-3
- Harismendy O, Notani D, Song X, Rahim NG, Tanasa B, Heintzman N, Ren B, Fu XD, Topol EJ, Rosenfeld MG, et al. 9p21 DNA variants associated with coronary artery disease impair interferon-gamma signalling response. Nature 2011; 470:264–8; PMID:21307941; http://dx.doi.org/10.1038/nature09753
- Wang J, Zhuang J, Iyer S, Lin X, Whitfield TW, Greven MC, Pierce BG, Dong X, Kundaje A, Cheng Y, et al. Sequence features and chromatin structure around the genomic regions bound by 119 human transcription factors. Genome Res 2012; 22:1798–812; PMID:22955990; http://dx.doi.org/10.1101/gr.139105.112
- Wang J, Zhuang J, Iyer S, Lin XY, Greven MC, Kim BH, Moore J, Pierce BG, Dong X, Virgil D, et al. Factorbook.org: a Wiki-based database for transcription factor-binding data generated by the ENCODE consortium. Nucleic Acids Res 2013; 41:D171–6; PMID:23203885; http://dx.doi.org/10.1093/nar/gks1221
- Gerstein MB, Kundaje A, Hariharan M, Landt SG, Yan KK, Cheng C, Mu XJ, Khurana E, Rozowsky J, Alexander R, et al. Architecture of the human regulatory network derived from ENCODE data. Nature 2012; 489:91–100; PMID:22955619; http://dx.doi.org/10.1038/nature11245
- Moriuchi M, Moriuchi H, Margolis DM, Fauci AS. USF/c-Myc enhances, while Yin-Yang 1 suppresses, the promoter activity of CXCR4, a coreceptor for HIV-1 entry. J Immunol 1999; 162:5986–92; PMID:10229837
- Khan R, Spagnoli V, Tardif JC, L'Allier PL. Novel anti-inflammatory therapies for the treatment of atherosclerosis. Atherosclerosis 2015; 240:497–509; PMID:25917947; http://dx.doi.org/10.1016/j.atherosclerosis.2015.04.783
- Libby P, Ridker PM, Maseri A. Inflammation and atherosclerosis. Circulation 2002; 105:1135–43; PMID:11877368; http://dx.doi.org/10.1161/hc0902.104353
- Li Z, Rana TM. Decoding the noncoding: Prospective of lncRNA-mediated innate immune regulation. RNA Biol 2014; 11:979–85; PMID:25482890; http://dx.doi.org/10.4161/rna.29937
- Fitzgerald KA, Caffrey DR. Long noncoding RNAs in innate and adaptive immunity. Curr Opin Immunol 2014; 26:140–6; PMID:24556411; http://dx.doi.org/10.1016/j.coi.2013.12.001
- Vallabhapurapu S, Karin M. Regulation and function of NF-kappaB transcription factors in the immune system. Annu Rev Immunol 2009; 27:693–733; PMID:19302050; http://dx.doi.org/10.1146/annurev.immunol.021908.132641
- de Winther MP, Kanters E, Kraal G, Hofker MH. Nuclear factor kappaB signaling in atherogenesis. Arteriosclerosis, thrombosis, and vascular biology 2005; 25:904–14; PMID:15731497; http://dx.doi.org/10.1161/01.ATV.0000160340.72641.87
- Gareus R, Kotsaki E, Xanthoulea S, van der Made I, Gijbels MJ, Kardakaris R, Polykratis A, Kollias G, de Winther MP, Pasparakis M. Endothelial cell-specific NF-kappaB inhibition protects mice from atherosclerosis. Cell Metab 2008; 8:372–83; PMID:19046569; http://dx.doi.org/10.1016/j.cmet.2008.08.016
- Rapicavoli NA, Qu K, Zhang J, Mikhail M, Laberge RM, Chang HY. A mammalian pseudogene lncRNA at the interface of inflammation and anti-inflammatory therapeutics. Elife 2013; 2:e00762; PMID:23898399; http://dx.doi.org/10.7554/eLife.00762
- Carpenter S, Aiello D, Atianand MK, Ricci EP, Gandhi P, Hall LL, Byron M, Monks B, Henry-Bezy M, Lawrence JB, et al. A long noncoding RNA mediates both activation and repression of immune response genes. Science 2013; 341:789–92; PMID:23907535; http://dx.doi.org/10.1126/science.1240925
- Gordon S, Akopyan G, Garban H, Bonavida B. Transcription factor YY1: structure, function, and therapeutic implications in cancer biology. Oncogene 2006; 25:1125–42; PMID:16314846; http://dx.doi.org/10.1038/sj.onc.1209080
- Wang H, Garzon R, Sun H, Ladner KJ, Singh R, Dahlman J, Cheng A, Hall BM, Qualman SJ, Chandler DS, et al. NF-kappaB-YY1-miR-29 regulatory circuitry in skeletal myogenesis and rhabdomyosarcoma. Cancer Cell 2008; 14:369–81; PMID:18977326; http://dx.doi.org/10.1016/j.ccr.2008.10.006
- Huerta-Yepez S, Vega M, Garban H, Bonavida B. Involvement of the TNF-alpha autocrine-paracrine loop, via NF-kappaB and YY1, in the regulation of tumor cell resistance to Fas-induced apoptosis. Clin Immunol 2006; 120:297–309; PMID:16784892; http://dx.doi.org/10.1016/j.clim.2006.03.015
- Siednienko J, Maratha A, Yang S, Mitkiewicz M, Miggin SM, Moynagh PN. Nuclear factor kappaB subunits RelB and cRel negatively regulate Toll-like receptor 3-mediated beta-interferon production via induction of transcriptional repressor protein YY1. J Biol Chem 2011; 286:44750–63; PMID:22065573; http://dx.doi.org/10.1074/jbc.M111.250894
- Hansson GK, Libby P. The immune response in atherosclerosis: a double-edged sword. Nat Rev Immunol 2006; 6:508–19; PMID:16778830; http://dx.doi.org/10.1038/nri1882
- Vausort M, Wagner DR, Devaux Y. Long Noncoding RNAs in Patients With Acute Myocardial Infarction. Circulation Research 2014; 115:668–U180; PMID:25035150; http://dx.doi.org/10.1161/CIRCRESAHA.115.303836
- Zeuke S, Ulmer AJ, Kusumoto S, Katus HA, Heine H. TLR4-mediated inflammatory activation of human coronary artery endothelial cells by LPS. Cardiovasc Res 2002; 56:126–34; PMID:12237173; http://dx.doi.org/10.1016/S0008-6363(02)00512-6
- Song C, Hsu K, Yamen E, Yan W, Fock J, Witting PK, Geczy CL, Freedman SB. Serum amyloid A induction of cytokines in monocytes/macrophages and lymphocytes. Atherosclerosis 2009; 207:374–83; PMID:19535079; http://dx.doi.org/10.1016/j.atherosclerosis.2009.05.007
- Kaplanski G, Marin V, Montero-Julian F, Mantovani A, Farnarier C. IL-6: a regulator of the transition from neutrophil to monocyte recruitment during inflammation. Trends Immunol 2003; 24:25–9; PMID:12495721; http://dx.doi.org/10.1016/S1471-4906(02)00013-3
- Barnes PJ, Karin M. Nuclear factor-kappaB: a pivotal transcription factor in chronic inflammatory diseases. N Engl J Med 1997; 336:1066–71; PMID:9091804; http://dx.doi.org/10.1056/NEJM199704103361506
- Zhang X, Ding L, Sandford AJ. Selection of reference genes for gene expression studies in human neutrophils by real-time PCR. BMC Mol Biol 2005; 6:4; PMID:15720708; http://dx.doi.org/10.1186/1471-2199-6-4
- Wang L, Feng Z, Wang X, Wang X, Zhang X. DEGseq: an R package for identifying differentially expressed genes from RNA-seq data. Bioinformatics 2010; 26:136–8; PMID:19855105; http://dx.doi.org/10.1093/bioinformatics/btp612
- Klipper-Aurbach Y, Wasserman M, Braunspiegel-Weintrob N, Borstein D, Peleg S, Assa S, Karp M, Benjamini Y, Hochberg Y, Laron Z. Mathematical formulae for the prediction of the residual beta cell function during the first two years of disease in children and adolescents with insulin-dependent diabetes mellitus. Med Hypotheses 1995; 45:486–90; PMID:8748093; http://dx.doi.org/10.1016/0306-9877(95)90228-7
- Storey JD, Tibshirani R. Statistical significance for genomewide studies. Proc Natl Acad Sci U S A 2003; 100:9440–5; PMID:12883005; http://dx.doi.org/10.1073/pnas.1530509100
- Hu FY, Wu C, Li Y, Xu K, Wang WJ, Cao HQ, Tian XL. AGGF1 is a novel anti-inflammatory factor associated with TNF-alpha-induced endothelial activation. Cellular Signalling 2013; 25:1645–53; PMID:23628701; http://dx.doi.org/10.1016/j.cellsig.2013.04.007
- Nelson JD, Denisenko O, Sova P, Bomsztyk K. Fast chromatin immunoprecipitation assay. Nucleic Acids Res 2006; 34:e2; PMID:16397291; http://dx.doi.org/10.1093/nar/gnj004
- Rinn JL, Kertesz M, Wang JK, Squazzo SL, Xu X, Brugmann SA, Goodnough LH, Helms JA, Farnham PJ, Segal E, et al. Functional demarcation of active and silent chromatin domains in human HOX loci by noncoding RNAs. Cell 2007; 129:1311–23; PMID:17604720; http://dx.doi.org/10.1016/j.cell.2007.05.022
- Niranjanakumari S, Lasda E, Brazas R, Garcia-Blanco MA. Reversible cross-linking combined with immunoprecipitation to study RNA-protein interactions in vivo. Methods 2002; 26:182–90; PMID:12054895; http://dx.doi.org/10.1016/S1046-2023(02)00021-X
- Chu C, Quinn J, Chang HY. Chromatin isolation by RNA purification (ChIRP). J Vis Exp 2012
- Engreitz J, Lander ES, Guttman M. RNA antisense purification (RAP) for mapping RNA interactions with chromatin. Methods Mol Biol 2015; 1262:183–97; PMID:25555582; http://dx.doi.org/10.1007/978-1-4939-2253-6_11
- Kaida D, Berg MG, Younis I, Kasim M, Singh LN, Wan L, Dreyfuss G. U1 snRNP protects pre-mRNAs from premature cleavage and polyadenylation. Nature 2010; 468:664–8; PMID:20881964; http://dx.doi.org/10.1038/nature09479