ABSTRACT
The addition of telomeric DNA to chromosome ends is an essential cellular activity that compensates for the loss of genomic DNA that is due to the inability of the conventional DNA replication apparatus to duplicate the entire chromosome. The telomerase reverse transcriptase and its associated RNA bind to the very end of the telomere via a sequence in the RNA and specific protein-protein interactions. Telomerase RNA also provides the template for addition of new telomeric repeats by the reverse-transcriptase protein subunit. In addition to the template, there are 3 other conserved regions in telomerase RNA that are essential for normal telomerase activity. Here we briefly review the conserved core regions of telomerase RNA and then focus on a recent study in fission yeast that determined the function of another conserved region in telomerase RNA called the Stem Terminus Element (STE).Citation1 The STE is distant from the templating core of telomerase in both the linear and RNA secondary structure, but, nonetheless, affects the fidelity of telomere sequence addition and, in turn, the ability of telomere binding proteins to bind and protect chromosome ends. We will discuss possible mechanisms of STE action and the suitability of the STE as an anti-cancer target.
Introduction
The passage of intact genetic information to the next generation is essential for life to continue. In addition to environmental assaults, genome integrity is threatened by the process of DNA replication itself. Duplication of the very ends of chromosomes creates unique problems for the DNA replication machinery. Because DNA polymerases work only in the 5′ to 3′ direction and require a primer, usually a short stretch of RNA, to begin replication, the lagging strand cannot be duplicated fully by the conventional replication apparatus.Citation2 This “end replication problem” is solved in almost all eukaryotes by a unique ribonucleoprotein particle (RNP) called telomerase. Telomerase counteracts DNA loss due to conventional replication by adding short non-coding repetitive sequences called telomeric DNA, to the 3′ ends of chromosomes.
Telomerase is only active in the subset of human cells that are highly proliferative, such as stem cells. However, telomerase is reactivated in about 85% of tumors and is a target of anti-cancer drug strategies (Reviewed in Citation3). For in vitro activity, the essential components of the telomerase RNP are the catalytic protein subunit, which is a specialized reverse transcriptase, and the highly structured non-coding telomerase RNA. Although the names given to these subunits differ in different organisms, for simplicity we use TERT (Telomerase reverse transcriptase) and TER (Telomerase RNA) when referring to these subunits. The prototypical function of TER is to provide the template for the telomeric repeats that are added by the catalytic activity of TERT. In this article, we briefly summarize the properties of the 3 conserved regions of the TER core and then review a recent paper Citation1 that sheds light on the function of a conserved non-templating TER region called the STE (Stem terminus element) that nonetheless affects the fidelity of fission yeast telomerase templating activity (The vertebrate STE was formerly referred to as CR4/CR5Citation4).
TER
In addition to the TERT and TER subunits, telomerase holoenzymes contain species-specific accessory proteins (). For example, in Saccharomyces cerevisiae and fission yeast, the accessory factor Est1 is part of the holoenzyme, H/ACA interacting proteins, such as Dyskerin, are a part of the human RNP and p65 is an integral component of ciliate telomerase.Citation5-8 Species-specific holoenzyme components bind differently, but each molecule interacts with the RNP via TER. This scaffolding function may account for the large differences in the size of TER,Citation9,10 which vary in length from ∼150 nucleotides (nts) in Tetrahymena thermophila Citation11 to an inferred ∼2200 nts in Plasmodium falciparum.Citation13 The scaffolding interactions influence protein-protein interactions within the telomerase holoenzyme, subcellular localization of the complex, RNP biogenesis and stability and interactions of the holoenzyme with telomeres (reviewed in Citation14,15). While different telomerase RNA motifs and different telomerase accessory proteins RNA binding domains are used in different species to generate mature telomerase RNPs, 4 RNA regions that affect catalytic activity are present in nearly every telomerase RNA. The template, the TBE (Template boundary element) and the pseudoknot are close together in the catalytic core of the telomerase RNP. However, the STE is far from the core in both the linear distance and secondary structure ().
Figure 1. Schematic of evolutionarily conserved telomerase RNA (TER) regions (based primarily on ciliate, vertebrate and fungal sequences; adapted from Citation15). Conserved Template, Pseudoknot, TBE and STE regions are depicted as black lines. In some vertebrates, the “core enclosing helix” functions as the TBE. Stem Loop IV is the ciliate STE (see text for details) similar to echinoderms.Citation59 TER regions of interaction with TERT are depicted as a large oval. Unnamed species-specific telomerase components, which are depicted as dark gray circles, bind to TER regions (dashed gray lines) separate from the template, pseudoknot, TBE and STE.
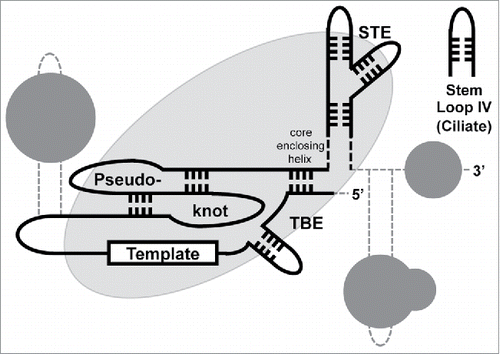
TER template
The TER template is at the center of telomerase catalysis (). In most organisms, including ciliates,Citation11 fission yeast Citation16,17 and humans,Citation18 the template consists of 1½ copies of the complement of a single repeat of telomeric DNA. Base pairing between the very end of the single-stranded telomeric DNA and the TER template positions the telomerase RNP at the end of the telomere. This interaction is facilitated by TER-TERT interactions, which expose the TER template.Citation19 However, telomerase substrate affinity is not necessarily dependent on the extent of telomeric DNA:TER RNA base pairing.Citation20 Typically, the last third of the template binds to telomeric DNA, although the Saccharomyces cerevisiae TER utilizes multiple telomere-template alignments.Citation21
The number of base pairs between telomeric DNA and the TER template is constant during elongation in S. cerevisiae.Citation22 As new nucleotides are added to the chromosome end, base pairs at the beginning of the template:substrate hybrid are broken. After the full template is copied, the template translocates within the RNP to start another cycle of telomere extension. Recent biochemical and FRET experiments show that during this translocation, gross rearrangements occur within the RNP during repositioning of the RNA template/telomere DNA hybrid for the next round of telomere addition. These rearrangements, which include extrusion of the DNA substrate from the RNP active site, are the rate limiting step in the telomerase reaction.Citation23,24
In fission yeast, the RNA template and telomeric DNA substrate interact by a single base pair that generates a conformation called the “stuttering alignment” (, left; reviewed in Citation25). This register promotes the addition of a variable number of guanines before the core telomeric repeat sequence (5′-GGTTACA-3′) is added to generate a 5′-(G)1–6GGTTACA-3′ repeat. However, if a cytosine is added to the end of the repeat, it generates the rare variant repeat 5′-(G)1–6GGTTACAC-3′ (rare cytosine is underlined). Stuttering (i.e., addition of a variable length guanine tract) does not occur when a rare variant repeat is lengthened in the next elongation cycle because the rare cytosine forces the telomeric DNA:RNA template into the “non-stuttering alignment” Citation17,25,26 (, right), which reduces guanine tract incorporation. Thus, the “non-stuttering alignment” produces telomeric DNA containing more rare cytosines and fewer guanine tracts.
Figure 2. Mechanism for incorporation of sequence heterogeneity into fission yeast telomeres. (Left) TER interactions with its substrate is mediated by 3 bp formed between the telomere end and the TER1 template leaving the rest of the template available for nucleotide addition. This alignment leaves the terminal adenine unpaired and contributes to the stuttering reaction that produces variable guanine tracts in fission yeast. (Right) If reverse transcription proceeds into the 5′ TBE, then a cytosine is added to the end of the core 5′-GGTTACA-3′ repeat to generate 5′-GGTTACAC-3′, which causes a non-stuttering register after translocation (underlined C is the rare cytosine incorporated by TBE read-through). (Reproduced from Citation1).
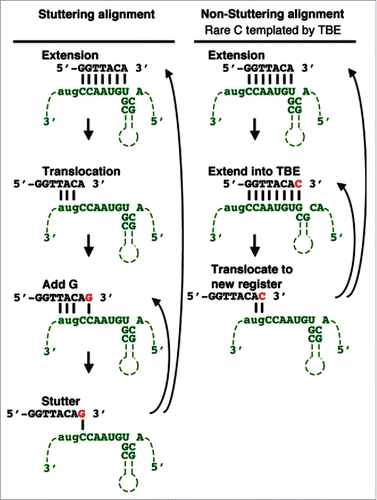
TER TBE
How is the rare cytosine incorporated into fission yeast telomeric DNA when the last template residue is not a guanine? The rare cytosine is added when elongation continues into the second basepair of the TBE helix. The TBE helix forms 0–2 nucleotides from the template, as in fission and budding yeasts and filamentous fungi,Citation27 or a short distance from the 5′ end of the TER template, as in some vertebrates and ciliates (; reviewed in Citation10). Therefore, by steric interference or by sequestration, the TBE prevents run-on reverse transcription past the end of the TER template. However, in wild type fission yeast, the first guanine in the TBE helix is occasionally accessible to template the rare cytosine that generates 5′-GGTTACAC-3′ repeats (, right). TBE function is compromised by basepair mutations that prevent the base of the helix from forming. These mutations increase access to the guanine that templates the rare cytosine, which increases rare cytosine incorporation and decreases guanine tracts by forcing the “non-stuttering alignment.”Citation17,26
TER pseudoknot
The pseudoknot is a triple helix within the core of the telomerase RNP that is formed by long distance base pairing between a hairpin and single stranded RNA (). The template, pseudoknot and TBE are enclosed by a helix at the TER 5′ end to form a larger combined core domain.Citation28 In some vertebrates TERs, including humans, the core enclosing helix also functions as the TBE.Citation4 Pseudoknots contribute to telomerase activity in S. cerevisiase and humans and may function by positioning the telomere and template within the RNP active site.Citation29 Mutations in the Kluyveromyces lactis pseudoknot produce altered telomeric repeats in vivo independently of the telomerase activity defects assessed in vitro.Citation30 The critical role of the human pseudoknot is demonstrated by a 2 nt pseudoknot mutation that reduces its folding 400 fold in vitro Citation31 and is linked to the telomeropathy dyskeratosis congenita.Citation32
TER STE
The STE was discovered in mammals where it was shown to be essential for telomerase activity in vitro and in vivo.Citation33 The human STE includes a 3-way junction (TWJ) and the P6.1 hairpin, whose sequence is highly conserved between mice and humans (). Phylogenetic comparisons of predicted TER secondary structures in yeast and vertebrates identified TWJs in every RNA analyzed.Citation34,35 Although the TWJ is widespread, the P6.1 hairpin that is adjacent to the TWJ in human TER is not present in some yeasts, including S. cerevisiae.Citation27 Moreover, the S. cerevisiae STE is not essential for telomerase activity,Citation36 which is consistent with this structural difference, although other domains may perform functions similar to those of the P6.1 helix.Citation37,38 Phylogenetic covariation analysis demonstrates that filamentous fungal TERs contain a TWJ and P6.1 hairpin.Citation27 Ciliate TERs do not have TWJs, but the ciliate TER stem loop IV has functional and structural similarities with the vertebrate P6.1 helix.Citation39,40
Fission yeast TER STE
The fission yeast STE consists of a TWJ and a P6.1-like helix that are well conserved with their human counterparts (). Based on the sequence and structural conservation between the human and fission yeast STEs,Citation1 we reasoned that analysis of STE function in fission yeast might shed light on its role in human telomerase. The STE had previously only been assessed for in vitro activity in a rabbit reticulocyte lysate with truncated TER fragments.Citation12 To this end, we generated a panel of STE mutants that allowed us to use genetic methods to examine STE function in vivo. Complete ablation of the fission yeast STE causes rapid telomere loss. However, a less severe partial loss-of-function substitution mutation that changes the 4-uridine tetraloop in the STE to 4 adenines (called ter1-STEloop) maintains short but stable telomeres by a telomerase-dependent mechanism. ter1-STEloop cells can maintain telomeres for at least a hundred generations in cells lacking the ALT recombination pathway that acts in telomerase deficient cells. Because the ter1-STEloop allele provides telomere function but has a telomere length defect, it was ideal for analyzing STE function(s) in vivo.Citation1
Figure 3. Schematic comparing fission yeast and human STE sequence and structure. STE nucleotides conserved between fission yeast and humans are depicted in orange. A gray box denotes the human P6.1 helix (Modified from Citation1).
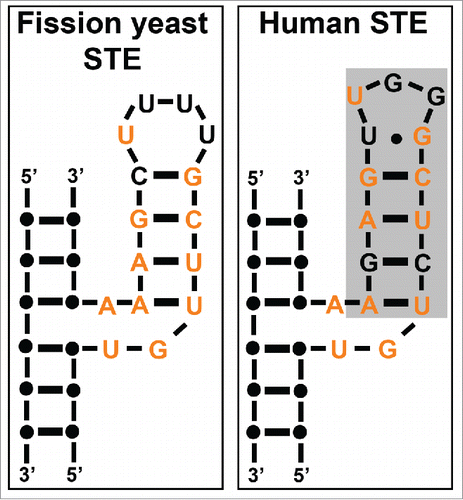
We tested ter1-STEloop cells for defects in telomerase RNA biogenesis and function.Citation1 Despite its short telomeres, TER1-STEloop RNA interacts normally with TERT, localizes to the nucleus, and the telomerase RNP interacts normally with telomeres. However, an in vitro telomerase assay demonstrates that TER1-STEloop has a catalytic defect that reduces nucleotide incorporation by >90%, which is similar to the in vitro defects of mouse Citation41 and human Citation42 STE loop mutants.
The combination of very low telomerase activity in vitro and short telomeres in vivo, suggested that an aspect of the telomere repeat addition cycle is defective in the ter1-STEloop mutant. To address this possibility, we determined if the sequence of ter1-STEloop telomeres is normal. Newly added telomeric DNA was obtained by shifting cells to 37°C, which causes a ∼50% reduction in telomere length.Citation1 Once the cells are returned to 30°C, new sequence is incorporated, and telomeres return to wild-type length. Analysis of the new sequence templated in vivo by TER1-STEloop shows that the number of rare cytosines (5′-GGTTACAC-3′) is increased significantly while the number and length of guanine tracts is reduced significantly.
Because the sequence of ter1-STEloop telomeric DNA is so different from the wild-type sequence, we considered that it might not support the proper assembly of the 6-member telomere capping complex called shelterin (reviewed in Citation43). Two of the 6 shelterin proteins are sequence specific DNA binding proteins, Taz1 (Telomere–associated in Schizosaccharomyces pombe 1), which binds double-stranded telomeric DNA, and Pot1 (Protection of telomeres 1), which binds the single-stranded terminal G-tail. Taz1 is an ortholog of 2 human telomere binding proteins, human TRF1/2 (TTAGGG repeat factor 1/2) and fission yeast Pot1 is similar to human POT1. The fission yeast shelterin complex is functionally and structurally similar to human shelterin. In both organisms, the non-DNA binding shelterin components associate with telomeric DNA via protein-protein interactions that form a bridge connecting single-stranded and double-stranded telomeric DNA.
We used chromatin immuno-precipitation to determine if telomeric chromatin formation is impaired in ter1-STEloop cells and found that both Taz1- and Pot1-telomere interactions are significantly reduced in ter1-STEloop cells.Citation1 Furthermore, one-hybrid experiments carried out in a bacterial system showed that Taz1 binding is compromised by a reduction in the density of guanine tracts. Together these data argue that, as in vertebrates, the fission yeast STE loop affects the catalysis of nucleotide addition,Citation41 and the fidelity of telomere repeat incorporation. When STE function is reduced, both telomeric sequence and assembly of telomere chromatin are abnormal ().
Figure 4. The fission yeast STE enforces telomere sequence identity and telomere chromatin organization. (Upper) In wild type fission yeast cells the TER1 (green) STE promotes TBE function, which maintains the helix that sequesters the rare cytosine templating guanine. Reduced rare cytosine content increases guanine tracts incorporation (blue) by the “stuttering” reaction and promotes telomere binding of the shelterin components Taz1 and Pot1. (Lower) The TER1-STEloop RNP is unable to maintain TBE function, which allows run-on reverse transcription to use the TBE sequestered guanine to incorporate rare cytosines into ter1-STEloop telomeres. 5′-GGTTACAC-3′ repeats force the “non-stuttering” extension reaction that prevents guanine tract incorporation. The rare cytosines and reduced guanine tracts in ter1-STEloop telomeres are bound less well by Taz1 and Pot1 (Modified from Citation1).
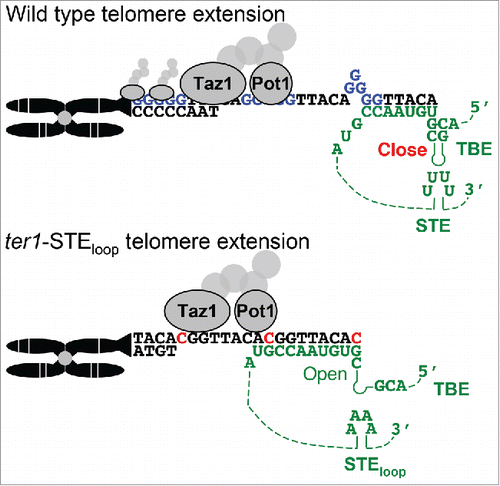
How does the STE loop affect telomere sequence?
How the fission yeast STE enforces telomere sequence fidelity is an open question. The simplest model is that the STEloop loss-of-function allele causes higher incorporation of the rare cytosine by increasing the frequency of read-through into the TBE. However, rare cytosines could be templated by either the guanine before or the guanine after the core templating sequence (sequence of fission yeast TER template is in bold: 3′-GCCAAUGUG-5′; ). Each of these candidate templating guanines is sequestered in a helix and is not readily available as a templating residue. The guanine at the 3′ end of the template bonds with a cytosine in the base of helix downstream of the template while the guanine at the 5′ end of the template is within the TBE (, top left). In vitro cross-linking experiments with fragments of human TER support the idea that the STE loop could affect usage of either guanine, as the human STE loop makes contact with the 3′ end of the template and makes multiple contacts with the template 5′ boundary.Citation44
Figure 5. Access to the guanine sequestered in the helix at the 3′ end of the template does not change rare cytosine or guanine tract incorporation into fission yeast telomeric DNA. (Upper left) RNA secondary structure model depicting the fission yeast TER template, downstream helix, TBE and surrounding RNA.Citation17 Arrows indicate core “Template” sequence (5′-UGUAACC-3′; bold); “TBE” (Template boundary element); “tac/ATG” (location of ter1-tac/ATG substitution mutant). The mutated nucleotides in ter1-tac/ATG cells that open the base of the helix adjacent to the template 3′ end by preventing basepairing are marked by a red oval. The guanine in the TBE that templates the rare cytosine is depicted in bold green and the putative templating guanine in the helix downstream of the template is in bold orange. (Upper Right) Telomere blot. Genomic DNA was extracted from ter1-tac/ATG cells that were streaked 8 times and hybridized with a telomere probe Citation17 to compare to the lengths of wild type telomeres. A bracket denotes telomere signal. (Lower left) Analysis of rare cytosine content in ∼50 ter1-tac/ATG and wild type telomeres as in.Citation1 (Lower right) Analysis of guanine tract content in ∼50 ter1-tac/ATG and wild type telomeres.
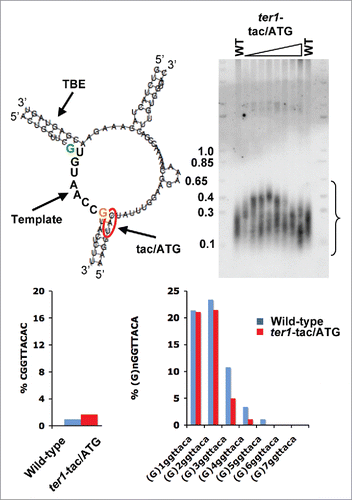
To determine which guanine templates the rare cytosine, we generated the ter1-tac/ATG allele (, top left). In this mutant, the guanine residue at the 3′ end of the template is no longer part of the helix as the cytosine to which it is normally base-paired is changed to guanine. Although the mutation forces open the base of the helix downstream of the normal template, it does not increase rare cytosine incorporation into telomeres (, bottom left) nor does it affect the occurrence of G-tracts (, bottom right). Moreover, unlike the short telomere phenotype of ter1-STEloop cells, telomeres in ter1-tac/ATG cells are on average longer than in wild type cells (, top right). Thus we conclude that greater access to the guanine at the 3′ end of the TER template does not account for the telomere phenotypes of ter1-STEloop cells.
In contrast to the ter1-tac/ATG allele, TER1 mutations that prevent the base of the TBE helix from forming produce the same phenotype as the ter1-STEloop mutation.Citation1 In both TBE helix formation mutants and ter1-STEloop telomeres 5′-GGTTACAC-3′ repeats with the rare cytosine are increased with a concomitant decrease in the variable length guanine tracts that precede the core repeat.Citation1,17,26 Furthermore, in vitro telomerase activity is dramatically reduced in ter1-STEloop Citation1 and mutants that prevent formation of the TBE.Citation26 Given that the telomere phenotypes of ter1-STEloop cells are similar to those of cells with TBE mutations, we surmise that TER1-STEloop RNA affects telomere sequence by allowing reverse transcription to proceed into the TBE. Therefore, the increased cytosines and reduced guanines are most likely the result of the guanine in the TBE being used to template the rare cytosine that causes the “non-stuttering” telomere addition register (described above; ).
Four uridine tetraloops, such as the fission yeast STE tetraloop, have exceptional flexibility Citation45 that increases their ability to sample different structural conformations.Citation46 The 4 uridine tetraloop in the fission yeast STE could affect TBE structure directly by aiding helix annealing or by stabilizing the formed helix. Two fission yeast TBE helix structures are predicted by Mfold.Citation26 In one, the TBE structure is a long helix with a bulged uridine in which the guanine that templates the rare cytosine is sequestered by basepairing. The second predicted TBE structure is a shorter but continuous helix that does not sequester the guanine in a base pair.Citation26 Stabilization of the first TBE structure by the STE predicts incorporation of fewer rare cytosines, as seen in the sequence of wild type telomeric DNA. In support of the idea that the STE could stabilize the longer bulged uridine TBE helix, some hairpin tetraloops bind helices with bulged nucleotides.Citation47 However, to our knowledge, there are no examples of a 4-uridine tetraloop making such a contact. Alternatively, it is possible that the STE loop affects processivity and reduces run-on reverse transcription into the TBE by inhibition of nucleotide addition at the 5′ end of the template or by destabilizing template:substrate base pairing during elongation. If the TER1-STEloop mutant RNA is compromised in either of these processes, it could reduce the TBE's ability to sequester the guanine that templates the rare cytosine.
Does the STE function during telomere length homeostasis?
Regardless of how the STE promotes nucleotide addition and prevents access to the TBE sequestered guanine, its action could be constitutive or regulated. If the STE only acts in certain situations, its selectivity could control telomere length at a step after telomerase recruitment to telomeres by affecting nucleotide addition catalysis and/or by affecting the organization of telomeric chromatin. Pseudouridylation and allostery are 2 non-exclusive mechanisms that could regulate STE loop activity, which might in turn control STE function.
There are at least 18 pseudouridylated sites in human TER Citation19,48 of which 2 are in the STE loop.Citation48 Pseudouridylation of the human STE loop increases hairpin stability, changes its conformation and decreases flexibility. In vitro, the pseudouridylated human TER STE loop decreases telomerase activity, but increases its repeat addition processivity. Because pseudouridylation affects telomerase activity in vitro, it is possible that selective pseudouridylation in vivo could regulate telomerase on the telomere by changing catalytic activity. As TERT binds the STE P6.1 hairpin,Citation33 whose stability is affected by pseuoduridylation,Citation48 selective pseudouridylation could affect telomerase holoenzyme formation off of the telomere and thereby affect telomerase activity by lowering the cellular concentration of the telomerase holoenzyme.
TERT binds 2 TER regions, the templating/pseudoknot region at the TER core and the STE (reviewed in Citation10). TERT interacts with the STE through TRBD (Telomerase RNA binding domain) contacts, although the specific binding residues are not conserved through evolution.Citation49 Ciliate and fish/insect composite structural models predict that the catalytic active site of TERT is not closely STE-associated.Citation50,51,52 However, because the STE affects catalysis in ciliates, vertebrates, fission yeast and K. lactis,Citation34 STE interaction with the TRBD may affect the conformation of the STE loop in a manner that regulates the TERT CTE domain (C-terminal extension). As the CTE affects template binding and telomerase nucleotide addition processivity,Citation53 a conformational change in the STE loop due to TRBD binding might cause a rearrangement in the CTE that, in turn, alters telomerase activity.Citation52 The putative allosteric effect that the TRBD:STE interaction has on CTE function is a possible point of regulation of telomere-bound telomerase activity.
Targeting the STE loop as an anti-cancer therapy
The reactivation of telomerase allows cancer cells to escape the Hayflick limit on cell division and contributes to their immortalization. The development of small molecules that interfere with the telomerase RNP is an attractive strategy because telomerase is not expressed in most somatic cells, but is active in most cancer cells.Citation3 A drawback to most anti-telomerase RNP strategies is that the anti-proliferative effect is dependent on telomeres shortening to a critically short length, which typically takes many generations to achieve, too many to be effective as an anti-tumor strategy.Citation3 In contrast, therapies directed against shelterin act much more quickly by disrupting capping, which leads to telomere dysfunction and apoptosis in a relatively short period of time. For unknown reasons, the anti-proliferative effects arising from shelterin disruption appear to be restricted to cancer cells, which may be due to aberrant shelterin arrangements in cancer cells (Reviewed in Citation3).
Because the terminal region of human telomeres consist of precise telomeric repeats, a change in telomere sequence induced by a small molecule Citation54 or by a mutation in the TER template Citation55 causes telomere de-protection, activation of the DNA damage response and end-to-end fusions, which cause apoptotic cell death. It is possible that the human STE functions like its fission yeast counterpart. The human STE may help to ensure telomeric sequence by preventing run-on reverse transcription by promoting TBE formation Citation56 or by another mechanism that affects processivity. In this scenario, therapeutics directed against the human STE loop could change telomerase activity, disrupt the sequence of telomeric DNA, alter the composition of shelterin and trigger apoptosis. In support of this strategy, the human TER STE loop is accessible to chemical probes in vivo when complexed with TERT.Citation19 Furthermore, RNA hairpin loops have already been targeted by small molecule selection strategies Citation57 for the treatment of disease.Citation58
Conclusion
The STE is a highly conserved region of telomerase RNAs that is distant from the telomerase core, but affects the catalysis of nucleotide addition and, as shown recently,Citation1 the fidelity of the telomere sequence and hence shelterin composition. The STE's physical separation from the core suggests an opportunity for regulating telomerase activity by mechanisms that bring the STE into a position where it can affect catalytic events. Furthermore, the STE's exposure in vivo Citation19 indicates that it is likely accessible to therapeutic agents.
Disclosure of potential conflicts of interest
No potential conflicts of interest were disclosed.
References
- Webb CJ, Zakian VA. Telomerase RNA stem terminus element affects template boundary element function, telomere sequence, and shelterin binding. Proc Natl Acad Sci U S A 2015; 112:11312-7; PMID:26305931; http://dx.doi.org/10.1073/pnas.1503157112
- Olovnikov AM. A theory of marginotomy. The incomplete copying of template margin in enzymic synthesis of polynucleotides and biological significance of the phenomenon. J Theor Biol 1973; 41:181-90; PMID:4754905
- Rousseau P, Autexier C. Telomere biology: Rationale for diagnostics and therapeutics in cancer. RNA Biol 2015; 12:1078-82; PMID:26291128; http:/dx.doi.org/10.1080/15476286.2015.1081329
- Chen JL, Blasco MA, Greider CW. Secondary structure of vertebrate telomerase RNA. Cell 2000; 100:503-14; PMID:10721988
- Mitchell JR, Wood E, Collins K. A telomerase component is defective in the human disease dyskeratosis congenita. Nature 1999; 402:551-5; PMID:10591218; http://dx.doi.org/10.1038/990141
- Zhou J, Hidaka K, Futcher B. The Est1 subunit of yeast telomerase binds the Tlc1 telomerase RNA. Mol Cell Biol 2000; 20:1947-55; PMID:10688642
- Webb CJ, Zakian VA. Schizosaccharomyces pombe Ccq1 and TER1 bind the 14-3-3-like domain of Est1, which promotes and stabilizes telomerase-telomere association. Gen Dev 2012; 26:82-91; http://dx.doi.org/10.1101/gad.181826.111
- Witkin KL, Collins K. Holoenzyme proteins required for the physiological assembly and activity of telomerase. Gen Dev 2004; 18:1107-18; http://dx.doi.org/10.1101/gad.1201704
- Zappulla DC, Cech TR. RNA as a flexible scaffold for proteins: yeast telomerase and beyond. Cold Spring Harb Symp Quant Biol 2006; 71:217-24; PMID:17381300
- Blackburn EH, Collins K. Telomerase: an RNP enzyme synthesizes DNA. Cold Spring Harb Perspect Biol 2011; 3:1-9; PMID:20660025; http://dx.doi.org/10.1101/cshperspect.a003558
- Greider CW, Blackburn EH. A telomeric sequence in the RNA of Tetrahymena telomerase required for telomere repeat synthesis. Nature 1989; 337:331-7; PMID:2463488
- Qi X, Li Y, Honda S, Hoffmann S, Marz M, Mosig A, Podlevsky JD, Stadler PF, Selker EU, Chen JJ. The common ancestral core of vertebrate and fungal telomerase RNAs. Nucleic acids research 2013; 41:450-62; PMID:23093598; http://dx.doi.org/10.1093/nar/gks980
- Chakrabarti K, Pearson M, Grate L, Sterne-Weiler T, Deans J, Donohue JP, Ares M Jr. Structural RNAs of known and unknown function identified in malaria parasites by comparative genomics and RNA analysis. RNA (New York, NY) 2007; 13:1923-39; PMID:17901154; http://dx.doi.org/10.1261/rna.751807
- Zhang Q, Kim NK, Feigon J. Architecture of human telomerase RNA. Proc Natl Acad Sci U S A 2011; 108:20325-32; PMID:21844345; http://dx.doi.org/10.1073/pnas.1100279108
- Egan ED, Collins K. Biogenesis of telomerase ribonucleoproteins. RNA (New York, NY) 2012; 18:1747-59; PMID:22875809; http://dx.doi.org/10.1261/rna.034629.112
- Leonardi J, Box JA, Bunch JT, Baumann P. TER1, the RNA subunit of fission yeast telomerase. Nat Struct Mol Biol 2008; 15:26-33; PMID:18157152; http://dx.doi.org/10.1038/nsmb1343
- Webb CJ, Zakian VA. Identification and characterization of the Schizosaccharomyces pombe TER1 telomerase RNA. Nat Struct Mol Biol 2008; 15:34-42; PMID:18157149; http://dx.doi.org/10.1038/nsmb1354
- Feng J, Funk WD, Wang SS, Weinrich SL, Avilion AA, Chiu CP, Adams RR, Chang E, Allsopp RC, Yu J, et al. The RNA component of human telomerase. Science (New York, NY) 1995; 269:1236-41; PMID:7544491; http://dx.doi.org/10.1126/science.7544491
- Zemora G, Handl S, Waldsich C. Human telomerase reverse transcriptase binds to a pre-organized hTR in vivo exposing its template. Nucl Acid Res 2015; 44(1):413-25; http://dx.doi.org/10.1093/nar/gkv1065
- Hammond PW, Cech TR. Euplotes telomerase: evidence for limited base-pairing during primer elongation and dGTP as an effector of translocation. Biochemistry 1998; 37:5162-72; PMID:9548747; http://dx.doi.org/10.1021/bi972988o
- Singer MS, Gottschling DE. TLC1: template RNA component of Saccharomyces cerevisiae telomerase. Science (New York, NY) 1994; 266:404-9; PMID:7545955; http://dx.doi.org/10.1126/science.7545955
- Forstemann K, Lingner J. Telomerase limits the extent of base pairing between template RNA and telomeric DNA. EMBO Rep 2005; 6:361-6; PMID:15776019; http://dx.doi.org/10.1038/sj.embor.7400374
- Parks JW, Stone MD. Coordinated DNA dynamics during the human telomerase catalytic cycle. Nat Commun 2014; 5:4146; PMID:24923681; http://dx.doi.org/10.1038/ncomms5146
- Qi X, Xie M, Brown AF, Bley CJ, Podlevsky JD, Chen JJ. RNA/DNA hybrid binding affinity determines telomerase template-translocation efficiency. EMBO J 2012; 31:150-61; PMID:21989387; http://dx.doi.org/10.1038/emboj.2011.363
- Ares M, Jr., Chakrabarti K. Stuttering against marginotomy. Nat Struct Mol Biol 2008; 15:18-9; PMID:18176550; http://dx.doi.org/10.1038/nsmb0108-18
- Box JA, Bunch JT, Zappulla DC, Glynn EF, Baumann P. A flexible template boundary element in the RNA subunit of fission yeast telomerase. J Biol Chem 2008; 283:24224-33; PMID:18574244; http://dx.doi.org/10.1074/jbc.M802043200
- Kuprys PV, Davis SM, Hauer TM, Meltser M, Tzfati Y, Kirk KE. Identification of telomerase RNAs from filamentous fungi reveals conservation with vertebrates and yeasts. PLoS One 2013; 8:e58661; PMID:23555591; http://dx.doi.org/10.1371/journal.pone.0058661
- Lin J, Ly H, Hussain A, Abraham M, Pearl S, Tzfati Y, Parslow TG, Blackburn EH. A universal telomerase RNA core structure includes structured motifs required for binding the telomerase reverse transcriptase protein. Proc Natl Acad Sci U S A 2004; 101:14713-8; PMID:15371596; http://dx.doi.org/10.1073/pnas.0405879101
- Qiao F, Cech TR. Triple-helix structure in telomerase RNA contributes to catalysis. Nat Struct Mol Biol 2008; 15:634-40; PMID:18500353; http://dx.doi.org/10.1038/nsmb.1420
- Tzfati Y, Knight Z, Roy J, Blackburn EH. A novel pseudoknot element is essential for the action of a yeast telomerase. Gen Dev 2003; 17:1779-88; PMID:12832393; http://dx.doi.org/10.1101/gad.1099403
- Holmstrom ED, Nesbitt DJ. Single-molecule fluorescence resonance energy transfer studies of the human telomerase RNA pseudoknot: temperature-/urea-dependent folding kinetics and thermodynamics. J Phys Chem B 2014; 118:3853-63; PMID:24617561; http://dx.doi.org/10.1021/jp501893c
- Vulliamy T, Marrone A, Goldman F, Dearlove A, Bessler M, Mason PJ, Dokal I. The RNA component of telomerase is mutated in autosomal dominant dyskeratosis congenita. Nature 2001; 413:432-5; PMID:11574891; http://dx.doi.org/10.1038/35096585
- Mitchell JR, Collins K. Human telomerase activation requires two independent interactions between telomerase RNA and telomerase reverse transcriptase. Molecular cell 2000; 6:361-71; PMID:10983983; http://dx.doi.org/10.1016/S1097-2765(00)00036-8
- Brown Y, Abraham M, Pearl S, Kabaha MM, Elboher E, Tzfati Y. A critical three-way junction is conserved in budding yeast and vertebrate telomerase RNAs. Nucl Acid Res 2007; 35:6280-9; PMID:17855392; http://dx.doi.org/10.1093/nar/gkm713
- Gunisova S, Elboher E, Nosek J, Gorkovoy V, Brown Y, Lucier JF, Laterreur N, Wellinger RJ, Tzfati Y, Tomaska L. Identification and comparative analysis of telomerase RNAs from Candida species reveal conservation of functional elements. RNA (New York, NY) 2009; 15:546-59; PMID:19223441; http://dx.doi.org/10.1261/rna.1194009
- Zappulla DC, Goodrich K, Cech TR. A miniature yeast telomerase RNA functions in vivo and reconstitutes activity in vitro. Nat Struct Mol Biol 2005; 12:1072-7; PMID:16299517; http://dx.doi.org/10.1038/nsmb1019
- Laterreur N, Eschbach SH, Lafontaine DA, Wellinger RJ. A new telomerase RNA element that is critical for telomere elongation. Nucl Acid Res 2013; 41(16):7713-24; PMID:23783570; http://dx.doi.org/10.1093/nar/gkt514
- Lebo KJ, Niederer RO, Zappulla DC. A second essential function of the Est1-binding arm of yeast telomerase RNA. RNA (New York, NY) 2015; 21:862-76; PMID:25737580; http://dx.doi.org/10.1261/rna.049379.114
- Mason DX, Goneska E, Greider CW. Stem-loop IV of tetrahymena telomerase RNA stimulates processivity in trans. Mol Cell Biol 2003; 23:5606-13; PMID:12897134; http://dx.doi.org/10.1128/MCB.23.16.5606-5613.2003
- Chen Y, Fender J, Legassie JD, Jarstfer MB, Bryan TM, Varani G. Structure of stem-loop IV of Tetrahymena telomerase RNA. EMBO J 2006; 25:3156-66; PMID:16778765; http://dx.doi.org/10.1038/sj.emboj.7601195
- Chen JL, Opperman KK, Greider CW. A critical stem-loop structure in the CR4-CR5 domain of mammalian telomerase RNA. Nucl Acid Res 2002; 30:592-7; PMID:11788723; http://dx.doi.org/10.1093/nar/30.2.592
- Robart AR, Collins K. Investigation of human telomerase holoenzyme assembly, activity, and processivity using disease-linked subunit variants. J Biol Chem 2010; 285:4375-86; PMID:20022961; http://dx.doi.org/10.1074/jbc.M109.088575
- Webb CJ, Wu Y, Zakian VA. DNA repair at telomeres: keeping the ends intact. Cold Spring Harb Perspect Biol 2013; 51-27; PMID:23732473
- Ueda CT, Roberts RW. Analysis of a long-range interaction between conserved domains of human telomerase RNA. RNA (New York, NY) 2004; 10:139-47; PMID:14681592; http://dx.doi.org/10.1261/rna.5118104
- Koplin J, Mu Y, Richter C, Schwalbe H, Stock G. Structure and dynamics of an RNA tetraloop: a joint molecular dynamics and NMR study. Structure 2005; 13:1255-67; PMID:16154083; http://dx.doi.org/10.1016/j.str.2005.05.015
- Persson T, Hartmann RK, Eckstein F. Selection of hammerhead ribozyme variants with low Mg2+ requirement: importance of stem-loop II. Chembiochem 2002; 3:1066-71; PMID:12404631; http://dx.doi.org/10.1002/1439-7633(20021104)3:11%3c1066::AID-CBIC1066%3e3.0.CO;2-G
- Butcher SE, Pyle AM. The molecular interactions that stabilize RNA tertiary structure: RNA motifs, patterns, and networks. Acc Chem Res 2011; 44:1302-11; PMID:21899297; http://dx.doi.org/10.1021/ar200098t
- Kim NK, Theimer CA, Mitchell JR, Collins K, Feigon J. Effect of pseudouridylation on the structure and activity of the catalytically essential P6.1 hairpin in human telomerase RNA. Nucl Acid Res 2010; 38:6746-56; PMID:20554853; http://dx.doi.org/10.1093/nar/gkq525
- Bley CJ, Qi X, Rand DP, Borges CR, Nelson RW, Chen JJ. RNA-protein binding interface in the telomerase ribonucleoprotein. Proc Natl Acad Sci U S A 2011; 108:20333-8; PMID:22123986; http://dx.doi.org/10.1073/pnas.1100270108
- Jiang J, Chan H, Cash DD, Miracco EJ, Ogorzalek Loo RR, Upton HE, Cascio D, O'Brien Johnson R, Collins K, Loo JA, et al. Structure of Tetrahymena telomerase reveals previously unknown subunits, functions, and interactions. Science (New York, NY) 2015; 350:aab4070; PMID:26472759; http://dx.doi.org/10.1126/science.aab4070
- Jiang J, Miracco EJ, Hong K, Eckert B, Chan H, Cash DD, Min B, Zhou ZH, Collins K, Feigon J. The architecture of Tetrahymena telomerase holoenzyme. Nature 2013; 496:187-92; PMID:23552895; http://dx.doi.org/10.1038/nature12062
- Huang J, Brown AF, Wu J, Xue J, Bley CJ, Rand DP, Wu L, Zhang R, Chen JJ, Lei M. Structural basis for protein-RNA recognition in telomerase. Nat Struct Mol Biol 2014; 21:507-12; PMID:24793650; http://dx.doi.org/10.1038/nsmb.2819
- Hossain S, Singh S, Lue NF. Functional analysis of the C-terminal extension of telomerase reverse transcriptase. A putative “thumb” domain. J Biol Chem 2002; 277:36174-80; PMID:12151386; http://dx.doi.org/10.1074/jbc.M201976200
- Mender I, Gryaznov S, Dikmen ZG, Wright WE, Shay JW. Induction of telomere dysfunction mediated by the telomerase substrate precursor 6-thio-2′-deoxyguanosine. Cancer Discov 2015; 5:82-95; PMID:25516420; http://dx.doi.org/10.1158/2159-8290.CD-14-0609
- Goldkorn A, Blackburn EH. Assembly of mutant-template telomerase RNA into catalytically active telomerase ribonucleoprotein that can act on telomeres is required for apoptosis and cell cycle arrest in human cancer cells. Cancer Res 2006; 66:5763-71; PMID:16740715; http://dx.doi.org/10.1158/0008-5472.CAN-05-3782
- Chen JL, Greider CW. Template boundary definition in mammalian telomerase. Gen Dev 2003; 17:2747-52; PMID:14630939; http://dx.doi.org/10.1101/gad.1140303
- Thomas JR, Hergenrother PJ. Targeting RNA with small molecules. Chem Rev 2008; 108:1171-224; PMID:18361529; http://dx.doi.org/10.1021/cr0681546
- Warui DM, Baranger AM. Identification of small molecule inhibitors of the HIV-1 nucleocapsid-stem-loop 3 RNA complex. J Med Chem 2012; 55:4132-41; PMID:22480197; http://dx.doi.org/10.1021/jm2007694
- Podlevsky JD, Li Y, Chen JJ. Structure and function of echinoderm telomerase RNA. RNA (New York, NY) 2016; 22:204-15; PMID:26598712; http://dx.doi.org/10.1261/rna.053280.115