ABSTRACT
The unusually high number of toxin-antitoxin (TA) systems in Mycobacterium tuberculosis, the etiological agent of tuberculosis, is thought to contribute to the unique ability of this pathogen to evade killing by the immune system and persist as a latent infection. One TA family, designated mazEF (for the MazE antitoxin and MazF toxin), comprises 10 of the >80 TA systems in the M. tuberculosis genome. Here we discuss the significance of our recent Nucleic Acids Res. paper that reports a surprising enzymatic activity for the MazF-mt9 toxin—sequence- and structure-specific cleavage of tRNA to generate tRNA halves—that underlies the growth-regulating properties of this toxin. This activity is distinct from all characterized MazF family members in M. tuberculosis and other bacteria; instead it is strikingly similar to that documented for members of another toxin family, VapC, despite the absence of sequence or structural similarity.
Abbreviations
TA | = | toxin-antitoxin |
MazF toxins and latent tuberculosis infection
The M. tuberculosis genome harbors an unusual abundance of TA systems (>80) relative to other free-living bacteria.Citation1 Therefore, TA systems have been implicated in M. tuberculosis stress survival and/or the switch to a non-replicating persistent state characteristic of latent tuberculosis infection. Among the >80 M. tuberculosis TA systems, the vapBC and mazEF families (assigned by amino acid sequence similarity) are the largest, with 50 and 10 members, respectively.Citation2 By comparison, most free-living bacteria have just one mazEF and when a vapBC module is present, there is only one.Citation1
M. tuberculosis has the highest number of mazEF TA systems of any pathogen. summarizes the general features of mazEF TA systems and illustrates the multiple mechanisms enlisted by MazF toxins to modulate bacterial cell growth. In addition to this mechanistic view, the association between M. tuberculosis MazF toxin activity and virulence, stress survival and nonreplicating persistence is based on a collection of published work. M. tuberculosis MazF toxin transcripts or protein levels are influenced by stresses associated with infection: nutrient starvation,Citation3-6 hypoxia,Citation6-8 macrophage infectionCitation7,9-11 or antibiotic treatment.Citation6,12-14 MazF toxin expression increases the number of persistersCitation15 and collection, simultaneous deletion of 3 mazF genes reduces persisters.Citation6 Finally, a triple mazF deletion hinders mycobacterial growth and lessens the pathology in the lungs and liver in the guinea pig model, suggesting that some MazF toxins may act cooperatively as virulence factors.Citation6
Figure 1. MazF toxins can specifically target mRNA, rRNA, or tRNA. All mazEF operons encode the intracellular toxin MazF downstream of the gene for the antitoxin MazE. In the absence of stress, the MazE antitoxin neutralizes the toxicity of MazF by forming a stable complex with the toxin. The MazEF complex and to a lesser extent, the MazE dimer, autoregulate the operon. Thus, the amount of free, active MazF toxin is dynamic and dependent on the concentration of the unstable MazE antitoxin that is susceptible to the action of cellular proteases. Many MazF family members appear to regulate growth by cleaving single-stranded mRNA at unique and specific 3-, 5- or 7-base sequences. However, E. coli MazF also cleaves 16S rRNA in the 30S ribosomal subunit. M. tuberculosis toxins MazF-mt6 and MazF-mt3 also cleave 23S rRNA in the 50S subunit or both 16S and 23S rRNA, respectively. By contrast, M. tuberculosis MazF-mt9 cleaves tRNA.
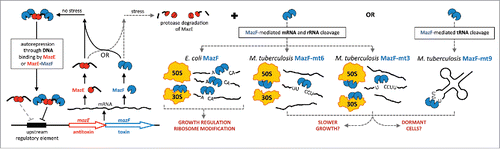
An evolving view of the mode of action of MazF toxins
All MazF toxins are unified by their hallmark single-strand, sequence-specific endoribonuclease activity. For example, all characterized MazF orthologs from bacteria and archaea arrest growth by cleaving single-stranded RNA at specific 3-, 5-, or 7-base recognition sequences.Citation16-22 Our studies and others to date portend that each of the 10 M. tuberculosis MazF toxins are likely to recognize distinct RNA sequences, some of different lengths.Citation22-26 While initially thought to exclusively target mRNAs—and thus surgically alter the transcriptome as a means to a desired end—we and others have demonstrated that MazF toxins can also target 23S and 16S rRNA to modify or inactivate the ribosome.Citation24,25,27 The focus of this Point of View article is on the unexpected function of one MazF toxin in M. tuberculosis, MazF-mt9, which regulates cell growth through very selective, precise cleavage of tRNA.
tRNA as a new MazF target
The breadth of RNA targets for MazF toxins further widened when we recently discovered that one of the M. tuberculosis MazF toxins, MazF-mt9, was a tRNase that specifically targeted cleavage of tRNALys.Citation23 From the start, we found that this toxin behaves very differently. We initially tried to identify the cleavage consensus sequence of MazF-mt9 using conventional approaches, i.e. in vivo and/or in vitro primer extension experiments after expression of, or incubation with, the toxin. We only identified a UU↓U sequence (where ↓ represents the location of RNA cleavage) in common in the 11 RNAs tested. However, only 2% of the UUU sequences in these 11 RNAs were cleaved. In contrast, other MazF toxins typically cleave at all consensus sequences, as we demonstrated for MazF-mt6.Citation24 These results indicated that while that presence of a UUU sequence is necessary, it was not sufficient for MazF-mt9 cleavage.
Then the question remains: what is the context required for MazF-mt9 cleavage at selected UUUs? This was answered only after exploiting a powerful modified RNA-seq technology.Citation24 In contrast to conventional RNA-seq routinely used to survey the entire transcriptome, our RNA-seq method enabled differential detection of subpopulations of RNA depending on the chemical moiety present at the 5′ end of the transcripts (i.e., 5′-OH for MazF family members). These RNA-seq experiments revealed that the proper context for the UU↓U cleavage site deduced from primer extension analysis was within a tRNA, more specifically tRNALys (), representing the first example of a MazF toxin that targets tRNA.
Figure 2. MazF-mt9 cleaves tRNALys in its anticodon stem loop. The site of cleavage is within the red UUU anticodon (5′UU↓U3′, yellow arrow). The sequence shown is identical between E. coli and Mtb. In the full length tRNA image above the boxed sequence, the D-arm is highlighted orange, the anticodon arm in light green, the anticodon in dark green, the T-arm in blue. Numbering in the anticodon indicates mature tRNA nucleotide position.
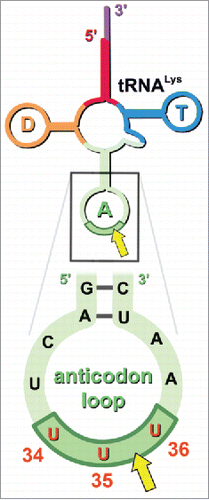
This finding contrasts with the literature that relates to MazF cleavage specificity, representing >45 publications that reach the conclusion that MazF toxins only cleave mRNA, not tRNA. In fact, due to the preponderance of double-stranded regions and conserved tertiary fold, it was assumed that tRNA is specifically immune from MazF cleavage. A model invoking mRNA as the exclusive target for MazF family members was so well accepted in the field that all MazF toxins had been almost universally referred to as “mRNA interferases.” Given that there are now examples of MazF toxins with specificity for tRNA, 23S rRNA, and 16S rRNA in addition to mRNA, use of the term mRNA interferase for MazF family members in general is obsolete as it does not accurately reflect the true spectrum of MazF targets.
Context matters
MazF-mt9 requires a precise structural context for target recognition and cleavage.Citation23 The determinants for toxin cleavage were analyzed by mutagenesis of the anticodon stem loop of tRNALys to either 1) alter the cleavage consensus sequence, 2) abolish the stem or 3) reconstitute the stem using a different complementary sequence ().
Figure 3. MazF-mt9 cleavage of tRNA is sequence- and structure-dependent. A yellow arrow indicates that cleavage occurs at the position shown. Wild type sequence at the anticodon, red; mutated sequences, blue. Mutants with a red X over the structure were not cleaved by MazF-mt9. Full-length tRNA colored as in . Numbering in the anticodon indicates mature tRNA nucleotide position.
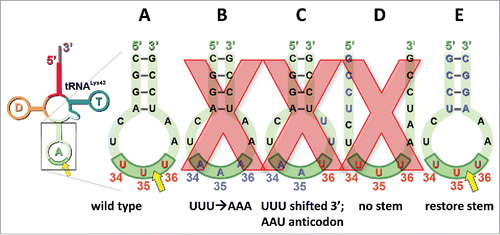
An intact UU↓U consensus is essential for MazF-mt9 cleavage of tRNALys (). No cleavage occurs when the UUU is mutated to AAA (). In addition, the UUU recognition sequence must also be in the proper context spatially and structurally. First, the UUU must be in the anticodon position of an intact anticodon stem loop. No cleavage occurs when the UUU is moved further up the anticodon loop (i.e. 3′), also resulting in a mutated anticodon (UUU to AAU, ). Therefore the UUU must be in the anticodon position within the anticodon loop, and in concordance with all other MazF toxins, the actual site of cleavage must be single-stranded. Second, the UUU must be in the anticodon position of the loop and adjacent to an intact stem. Disruption of stem formation prevents toxin cleavage (). However, restoration of the stem using a different, but complementary sequence restored MazF-mt9 cleavage (). No other MazF toxin is known to exhibit properties that are consistent with structure-dependent cleavage.
This MazF acts like a VapC
Although MazF-mt9 is the first example of a MazF toxin that targets tRNA, it is not the first toxin known to target tRNA for cleavage at the anticodon stem loop. The Gerdes laboratoryCitation28,29 and our laboratoryCitation30 have previously shown that some VapC toxins a have similar specificity. Yet VapC toxins have an entirely different amino acid sequence with no similarity to MazF toxins, use a completely different mechanism for catalysis that involves a PIN domain comprising 4 highly conserved acidic residues coordinated with a metal ion, and the structures of VapC toxins do not share any obvious features with the structures of MazF.Citation31-38 This conundrum will only be solved once a high resolution crystal structure of MazF-mt9 bound its tRNA substrate is obtained and compared to other MazF toxins as well as tRNA-cleaving VapC toxins.
A cautionary tale: One is not always like the others
Once again, as we had earlier demonstrated for MazF-mt3 and MazF-mt6, we have debunked the notion that the function of one MazF toxin mirrors that of the family as a whole. MazF-mt9 not only targets a different class of RNA distinct from other MazFs (), its mode of recognition is also different. No other MazF toxin is known to select its RNA target based on both sequence and structure. Each RNA species – tRNA, mRNA, and rRNA – folds in a unique way and interacts with specific enzymes, structural proteins, or cofactors to bestow a distinct function in protein synthesis and possibly other cellular processes. As a result, cleavage of one or more of these subtypes of RNA by a particular MazF toxin should yield distinct outcomes. Since many of the 10 MazF toxins in M. tuberculosis have yet to be characterized in detail, perhaps even more surprises lie ahead.
Table 1. Compilation of MazF RNA cleavage recognition sequences and targets.
Disclosure of potential conflicts of interest
No potential conflicts of interest were disclosed.
Funding
This work was supported in part by National Institutes of Health (NIH) grants R21AI072399, R56AI119055 to Woychik, NA and Husson, RN as well as New Jersey Health Foundation Signature Initiatives Research Grant #PC38-15 and a Busch Biomedical Research Grant to Woychik, NA; Schifano, JM was supported in part by NIH training grant T32AI007403, Virus-Host Interactions in Eukaryotic Cells to awarded to Brewer, G.
References
- Pandey DP, Gerdes K. Toxin-antitoxin loci are highly abundant in free-living but lost from host-associated prokaryotes. Nucleic Acids Res 2005; 33:966-76; PMID:15718296; http://dx.doi.org/10.1093/nar/gki201
- Sala A, Bordes P, Genevaux P. Multiple toxin-antitoxin systems in Mycobacterium tuberculosis. Toxins 2014; 6:1002-20; PMID:24662523; http://dx.doi.org/10.3390/toxins6031002
- Albrethsen J, Agner J, Piersma SR, Hojrup P, Pham TV, Weldingh K, Jimenez CR, Andersen P, Rosenkrands I. Proteomic profiling of Mycobacterium tuberculosis identifies nutrient-starvation-responsive toxin-antitoxin systems. Mol Cell Proteomics 2013; 12:1180-91; PMID:23345537; http://dx.doi.org/10.1074/mcp.M112.018846
- Betts JC, Lukey PT, Robb LC, McAdam RA, Duncan K. Evaluation of a nutrient starvation model of Mycobacterium tuberculosis persistence by gene and protein expression profiling. Mol Microbiol 2002; 43:717-31; PMID:11929527; http://dx.doi.org/10.1046/j.1365-2958.2002.02779.x
- Cortes T, Schubert OT, Rose G, Arnvig KB, Comas I, Aebersold R, Young DB. Genome-wide mapping of transcriptional start sites defines an extensive leaderless transcriptome in Mycobacterium tuberculosis. Cell Rep 2013; 5:1121-31; PMID:24268774; http://dx.doi.org/10.1016/j.celrep.2013.10.031
- Tiwari P, Arora G, Singh M, Kidwai S, Narayan OP, Singh R. MazF ribonucleases promote Mycobacterium tuberculosis drug tolerance and virulence in guinea pigs. Nat Commun 2015; 6:6059; PMID:25608501; http://dx.doi.org/10.1038/ncomms7059
- Ramage HR, Connolly LE, Cox JS. Comprehensive functional analysis of Mycobacterium tuberculosis toxin-antitoxin systems: implications for pathogenesis, stress responses, and evolution. PLoS genetics 2009; 5:e1000767; PMID:20011113; http://dx.doi.org/10.1371/journal.pgen.1000767
- Rustad TR, Harrell MI, Liao R, Sherman DR. The enduring hypoxic response of Mycobacterium tuberculosis. PloS one 2008; 3:e1502; PMID:18231589; http://dx.doi.org/10.1371/journal.pone.0001502
- Cappelli G, Volpe E, Grassi M, Liseo B, Colizzi V, Mariani F. Profiling of Mycobacterium tuberculosis gene expression during human macrophage infection: upregulation of the alternative sigma factor G, a group of transcriptional regulators, and proteins with unknown function. Res Microbiol 2006; 157:445-55; PMID:16483748; http://dx.doi.org/10.1016/j.resmic.2005.10.007
- Fontan P, Aris V, Ghanny S, Soteropoulos P, Smith I. Global transcriptional profile of Mycobacterium tuberculosis during THP-1 human macrophage infection. Infect Immun 2008; 76:717-25; PMID:18070897; http://dx.doi.org/10.1128/IAI.00974-07
- Korch SB, Contreras H, Clark-Curtiss JE. Three Mycobacterium tuberculosis Rel toxin-antitoxin modules inhibit mycobacterial growth and are expressed in infected human macrophages. J Bacteriol 2009; 191:1618-30; PMID:19114484; http://dx.doi.org/10.1128/JB.01318-08
- Denkin S, Byrne S, Jie C, Zhang Y. Gene expression profiling analysis of Mycobacterium tuberculosis genes in response to salicylate. Arch Microbiol 2005; 184:152-7; PMID:16175359; http://dx.doi.org/10.1007/s00203-005-0037-9
- Provvedi R, Boldrin F, Falciani F, Palu G, Manganelli R. Global transcriptional response to vancomycin in Mycobacterium tuberculosis. Microbiology 2009; 155:1093-102; PMID:19332811; http://dx.doi.org/10.1099/mic.0.024802-0
- Singh R, Barry CE, 3rd, Boshoff HI. The three RelE homologs of Mycobacterium tuberculosis have individual, drug-specific effects on bacterial antibiotic tolerance. J Bacteriol 2010; 192:1279-91; PMID:20061486; http://dx.doi.org/10.1128/JB.01285-09
- Han JS, Lee JJ, Anandan T, Zeng M, Sripathi S, Jahng WJ, Lee SH, Suh JW, Kang CM. Characterization of a chromosomal toxin-antitoxin, Rv1102c-Rv1103c system in Mycobacterium tuberculosis. Biochem Biophys Res Commun 2010; 400:293-8; PMID:20705052; http://dx.doi.org/10.1016/j.bbrc.2010.08.023
- Rothenbacher FP, Suzuki M, Hurley JM, Montville TJ, Kirn TJ, Ouyang M, Woychik NA. Clostridium difficile MazF Toxin Exhibits Selective, Not Global, mRNA Cleavage. J Bacteriol 2012; 194:3464-74; PMID:22544268; http://dx.doi.org/10.1128/JB.00217-12
- Yamaguchi Y, Nariya H, Park JH, Inouye M. Inhibition of specific gene expressions by protein-mediated mRNA interference. Nat Commun 2012; 3:607; PMID:22215082; http://dx.doi.org/10.1038/ncomms1621
- Zhang J, Zhang Y, Zhu L, Suzuki M, Inouye M. Interference of mRNA function by sequence-specific endoribonuclease PemK. J Biol Chem 2004; 279:20678-84; PMID:15024022; http://dx.doi.org/10.1074/jbc.M314284200
- Zhang Y, Zhang J, Hara H, Kato I, Inouye M. Insights into the mRNA cleavage mechanism by MazF, an mRNA interferase. J Biol Chem 2005; 280:3143-50; PMID:15537630; http://dx.doi.org/10.1074/jbc.M411811200
- Zhang Y, Zhang J, Hoeflich KP, Ikura M, Qing G, Inouye M. MazF cleaves cellular mRNAs specifically at ACA to block protein synthesis in Escherichia coli. Mol Cell 2003; 12:913-23; PMID:14580342; http://dx.doi.org/10.1016/S1097-2765(03)00402-7
- Zhu L, Inoue K, Yoshizumi S, Kobayashi H, Zhang Y, Ouyang M, Kato F, Sugai M, Inouye M. Staphylococcus aureus MazF specifically cleaves a pentad sequence, UACAU, which is unusually abundant in the mRNA for pathogenic adhesive factor SraP. J Bacteriol 2009; 191:3248-55; PMID:19251861; http://dx.doi.org/10.1128/JB.01815-08
- Zhu L, Phadtare S, Nariya H, Ouyang M, Husson RN, Inouye M. The mRNA interferases, MazF-mt3 and MazF-mt7 from Mycobacterium tuberculosis target unique pentad sequences in single-stranded RNA. Mol Microbiol 2008; 69:559-69; PMID:18485066; http://dx.doi.org/10.1111/j.1365-2958.2008.06284.x
- Schifano JM, Cruz JW, Vvedenskaya IO, Edifor R, Ouyang M, Husson RN, Nickels BE, Woychik NA. tRNA is a new target for cleavage by a MazF toxin. Nucleic Acids Res 2016; 44:1256-70; PMID:26740583; http://dx.doi.org/10.1093/nar/gkv1370
- Schifano JM, Edifor R, Sharp JD, Ouyang M, Konkimalla A, Husson RN, Woychik NA. Mycobacterial toxin MazF-mt6 inhibits translation through cleavage of 23S rRNA at the ribosomal A site. Proc Natl Acad Sci U S A 2013; 110:8501-6; PMID:23650345; http://dx.doi.org/10.1073/pnas.1222031110
- Schifano JM, Vvedenskaya IO, Knoblauch JG, Ouyang M, Nickels BE, Woychik NA. An RNA-seq method for defining endoribonuclease cleavage specificity identifies dual rRNA substrates for toxin MazF-mt3. Nat Commun 2014; 5:3538; PMID:24709835; http://dx.doi.org/10.1038/ncomms4538
- Zhu L, Zhang Y, Teh JS, Zhang J, Connell N, Rubin H, Inouye M. Characterization of mRNA interferases from Mycobacterium tuberculosis. J Biol Chem 2006; 281:18638-43; PMID:16611633; http://dx.doi.org/10.1074/jbc.M512693200
- Vesper O, Amitai S, Belitsky M, Byrgazov K, Kaberdina AC, Engelberg-Kulka H, Moll I. Selective translation of leaderless mRNAs by specialized ribosomes generated by MazF in Escherichia coli. Cell 2011; 147:147-57; PMID:21944167; http://dx.doi.org/10.1016/j.cell.2011.07.047
- Winther K, Tree JJ, Tollervey D, Gerdes K. VapCs of Mycobacterium tuberculosis cleave RNAs essential for translation. Nucleic Acids Res 2016.
- Winther KS, Gerdes K. Enteric virulence associated protein VapC inhibits translation by cleavage of initiator tRNA. Proc Natl Acad Sci U S A 2011; 108:7403-7; PMID:21502523; http://dx.doi.org/10.1073/pnas.1019587108
- Cruz JW, Sharp JD, Hoffer ED, Maehigashi T, Vvedenskaya IO, Konkimalla A, Husson RN, Nickels BE, Dunham CM, Woychik NA. Growth-regulating Mycobacterium tuberculosis VapC-mt4 toxin is an isoacceptor-specific tRNase. Nat Commun 2015; 6:7480; PMID:26158745; http://dx.doi.org/10.1038/ncomms8480
- Arcus VL, McKenzie JL, Robson J, Cook GM. The PIN-domain ribonucleases and the prokaryotic VapBC toxin-antitoxin array. Protein Eng Des Sel 2011; 24:33-40; PMID:21036780; http://dx.doi.org/10.1093/protein/gzq081
- Dienemann C, Boggild A, Winther KS, Gerdes K, Brodersen DE. Crystal structure of the VapBC toxin-antitoxin complex from Shigella flexneri reveals a hetero-octameric DNA-binding assembly. Journal of molecular biology 2011; 414:713-22; PMID:22037005; http://dx.doi.org/10.1016/j.jmb.2011.10.024
- Kamada K, Hanaoka F, Burley SK. Crystal structure of the MazE/MazF complex: molecular bases of antidote-toxin recognition. Mol Cell 2003; 11:875-84; PMID:12718874; http://dx.doi.org/10.1016/S1097-2765(03)00097-2
- Mattison K, Wilbur JS, So M, Brennan RG. Structure of FitAB from Neisseria gonorrhoeae bound to DNA reveals a tetramer of toxin-antitoxin heterodimers containing pin domains and ribbon-helix-helix motifs. J Biol Chem 2006; 281:37942-51; PMID:16982615; http://dx.doi.org/10.1074/jbc.M605198200
- Miallau L, Faller M, Chiang J, Arbing M, Guo F, Cascio D, Eisenberg D. Structure and proposed activity of a member of the VapBC family of toxin-antitoxin systems. VapBC-5 from Mycobacterium tuberculosis. J Biol Chem 2009; 284:276-83; PMID:18952600; http://dx.doi.org/10.1074/jbc.M805061200
- Min AB, Miallau L, Sawaya MR, Habel J, Cascio D, Eisenberg D. The crystal structure of the Rv0301-Rv0300 VapBC-3 toxin-antitoxin complex from M. tuberculosis reveals a Mg(2)(+) ion in the active site and a putative RNA-binding site. Protein Sci 2012; 21:1754-67; PMID:23011806; http://dx.doi.org/10.1002/pro.2161
- Simanshu DK, Yamaguchi Y, Park JH, Inouye M, Patel DJ. Structural basis of mRNA recognition and cleavage by toxin MazF and its regulation by antitoxin MazE in Bacillus subtilis. Mol Cell 2013; 52:447-58; PMID:24120662; http://dx.doi.org/10.1016/j.molcel.2013.09.006
- Zorzini V, Mernik A, Lah J, Sterckx YG, De Jonge N, Garcia-Pino A, De Greve H, Versées W, Loris R. Substrate Recognition and Activity Regulation of the Escherichia coli mRNA Endonuclease MazF. J Biol Chem 2016; 291:10950-60; PMID:27026704; http://dx.doi.org/10.1074/jbc.M116.715912
- Miyamoto T, Kato Y, Sekiguchi Y, Tsuneda S, Noda N. Characterization of MazF-Mediated Sequence-Specific RNA Cleavage in Pseudomonas putida Using Massive Parallel Sequencing. PloS one 2016; 11:e0149494; PMID:26885644; http://dx.doi.org/10.1371/journal.pone.0149494
- Munoz-Gomez AJ, Santos-Sierra S, Berzal-Herranz A, Lemonnier M, Diaz-Orejas R. Insights into the specificity of RNA cleavage by the Escherichia coli MazF toxin. FEBS Lett 2004; 567:316-20; PMID:15178344; http://dx.doi.org/10.1016/j.febslet.2004.05.005
- Park JH, Yamaguchi Y, Inouye M. Bacillus subtilis MazF-bs (EndoA) is a UACAU-specific mRNA interferase. FEBS letters 2011; 585:2526-32; PMID:21763692; http://dx.doi.org/10.1016/j.febslet.2011.07.008
- Pellegrini O, Mathy N, Gogos A, Shapiro L, Condon C. The Bacillus subtilis ydcDE operon encodes an endoribonuclease of the MazF/PemK family and its inhibitor. Mol Microbiol 2005; 56:1139-48; PMID:15882409; http://dx.doi.org/10.1111/j.1365-2958.2005.04606.x
- Fu Z, Donegan NP, Memmi G, Cheung AL. Characterization of MazFSa, an endoribonuclease from Staphylococcus aureus. J Bacteriol 2007; 189:8871-9; PMID:17933891; http://dx.doi.org/10.1128/JB.01272-07
- Schuster CF, Park JH, Prax M, Herbig A, Nieselt K, Rosenstein R, Inouye M, Bertram R. Characterization of a mazEF toxin-antitoxin homologue from Staphylococcus equorum. J Bacteriol 2013; 195:115-25; PMID:23104807; http://dx.doi.org/10.1128/JB.00400-12
- Miyamoto T, Yokota A, Tsuneda S, Noda N. AAU-Specific RNA Cleavage Mediated by MazF Toxin Endoribonuclease Conserved in Nitrosomonas europaea. Toxins 2016; 8; PMID:27271670; http://dx.doi.org/10.3390/toxins8060174