ABSTRACT
Circular RNAs (circRNAs) are a novel class of non-coding RNA characterized by a covalently closed-loop structure generated through a special type of alternative splicing termed backsplicing. CircRNAs are emerging as a heterogeneous class of molecules involved in modulating gene expression by regulation of transcription, protein and miRNA functions. CircRNA expression is cell type and tissue specific and can be largely independent of the expression level of the linear host gene, indicating that regulation of expression might be an important aspect with regard to control of circRNA function. In this review, a brief introduction to the characteristics that define a circRNA will be given followed by a discussion of putative biogenesis pathways and modulators of circRNA expression as well as of the stage at which circRNA formation takes place. A brief summary of circRNA functions will also be provided and lastly, an outlook with a focus on unanswered questions regarding circRNA biology will be included.
Alternative splicing is a pivotal process regulated by many factors that gives rise to functional diversity in the transcriptome. As products of a novel type of alternative splicing, circular RNAs (circRNAs) are a recently discovered class of molecules with functional relevance for regulation of gene expression. CircRNAs differ from other types of RNA by their structure: the 3′ and 5′ ends of circRNAs are covalently joined in a covalently closed loop structure leaving no free ends.Citation1-3 Both the function and biogenesis of circRNAs as a class is currently relatively uncharacterized and their potential biologic importance has been the issue of debate.Citation4,5 However, circRNAs are widely expressedCitation2,6 and examples of circRNAs capable of modulating steps in the gene expression pathway are accumulating,.Citation1,7-9 This indicates that circRNAs, at large, may play a role in this complex regulatory network and underpins the importance of gaining knowledge about this new type of molecule. Furthermore, as many circRNAs are conserved and dynamically expressed across cell types and differentiation stages in a manner that may diverge from and even oppose the expression of the linear host gene,Citation10,11 this indicates that circRNA biogenesis is a regulated process and that control of circRNA expression could be one means of controlling circRNA function. Hence, an understanding not only of how these circRNAs function, but also how they are generated, are important areas of research.
Characteristics of circular RNAs
circRNAs are a novel type of RNA by virtue of their unique structure resulting from a 3′to 5′ end-joining event (backsplicing). circRNAs most often originate from exons close to the 5′ end of a protein coding geneCitation2 and may consist of only a single or multiple exons.Citation4 Furthermore, multiple circRNAs can be produced from a single gene (alternative circularization),Citation12,13 and alternative splicing events (alternative 5′ and 3′ splice sites, exon skipping and intron retention) including alternative splicing events that alter the backsplice junction have been reported.Citation14 Although the majority of circRNAs consist of exons from protein coding genes, circRNAs can also arise from introns, intergenic regions, UTRs, ncRNA loci and from locations antisense to known transcripts.Citation1 Furthermore, a recent analysis using a new tool for circRNA detection, CIRIexplorer2, has uncovered novel less conserved exons that are almost exclusively present in circRNAs and not in linear RNAs derived from the same gene.Citation14 Whether these novel exons are only seen in circRNAs because their incorporation in a linear transcript induces degradation of linear transcripts through a pathway that circRNAs are resistant to, or whether the novel exons found may be included specifically in circRNAs due to selective splicing,Citation14 remains to be clarified.
Once produced, circRNAs may reside either in the nucleus, as observed for multiexon-circles with retained introns,Citation9 or they may be exported to the cytoplasm like the majority of circRNAs.Citation15 However, regardless of localization, the unique structure of circRNAs ensures another key feature associated with these molecules namely a very high stability,Citation13,16 presumably due to protection from exonucleolytic decay.Citation4
Prevalence and abundance of circular RNAs
Expression of circRNAs has been widely detected across every domain of life and in every one of the diverse array of cell types and organisms from humans to Drosophila and Archaea analyzed to date.Citation1,2,6,10,11,13,17-22 Analyses of transcriptome sequencing data sets with pipelines developed to look specifically for backsplicing events, revealed that circRNAs are frequently but not pervasively produced. Generally, 5.8% to 23% of the human genes that are actively transcribed give rise to circRNAsCitation13,21,23,24 in a way that is dynamically regulated between tissues and cell typesCitation11,14 as well as throughout differentiation.Citation10,21,24,25 For the most part, expression changes in circRNAs correlate with the linear transcripts derived from the same gene, but many genes exhibit differentially regulated circRNAs and linear RNA variants.Citation8,10,11 This widespread and regulated expression of circRNAs is consistent with these circRNAs potentially being functional and not just rare non-functional products of aberrant splicing as previously assumed.Citation5,26-31 In terms of expression level, circRNAs are generally low-abundant species but can be more abundant than the linear counterpart produced from the same gene.Citation13,21 For instance in the porcine cortex at embryonic day 23, 1012 circular RNAs can be detected and 45 of these are higher expressed than the linear transcript.Citation21 As the expression level at steady-state correlates with the rate of synthesis,Citation20 this difference in abundance appears mainly to be caused by a difference in the rate of biogenesis rather than a difference in decay rate.
Conservation of circular RNA producing loci
On a global scale circRNAs display an appreciable degree of conservation between closely related species such as human and mouseCitation4,10,25 with a subset of circRNAs conserved in the evolutionary distant Drosophila.Citation10 Nonetheless, despite the observed level of conservation being greater than expected if circRNA production were random,Citation2,11,13 only 5–30% of human circRNAs are completely conserved in mouse with regard to splice site use.Citation1,4,13 Hence, although there is a substantial level of conservation, species-specific circRNAs also exist.
Circular RNA biogenesis
As previously mentioned, circRNAs are distinct from other RNA species in that the otherwise free 3′ and 5′ ends are covalently linked.Citation1 The site at which the circRNA ends are joined is flanked by canonical splice signals,Citation1 indicating that the spliceosome is involved in production of circular RNA species in addition to its well-known role in constitutive and alternative linear RNA splicing.Citation32-34 However, in opposition to linear splicing where a downstream 3′ splice site is joined to an upstream 5′ splice site (), circRNA generation requires that an upstream 3′ splice site be joined to a downstream 5′ splice site (backsplicing ( and )).
Figure 1. Putative pathways for circRNA biogenesis. A constitutive linear RNA splicing pattern is shown for comparison purposes (A). CircRNA formation may proceed through a direct backsplicing pathway in which circularization can be driven by intron pairing (B, left upper panel) or RNA-binding protein pairing (B, right upper panel) that brings the appropriate splice signals within proximity of each other. A linear RNA containing the skipped exons may be formed as shown in the gray box or may be degraded depending on the kinetics of splicing vs. debranching and exonuclease mediated degradation. Alternatively, circRNAs may be produced through a lariat driven pathway (C) where exon skipping removes the exons to be backspliced from the primary transcript and promotes circularization because the splice signals of the circRNA exon to be are juxtaposed in the lariat structure. The circRNA may be formed concurrently with the linear RNA as shown in the gray box or may not be formed depending on the kinetics of intra-lariat splicing vs. debranching and exonuclease mediated degradation. In all instances, the intronic lariat products are likely rapidly degraded (gray, hatched boxes).
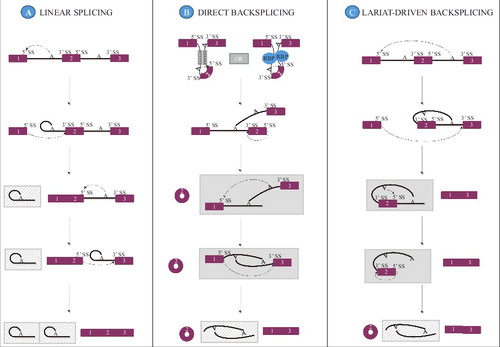
Evidence for the involvement of the spliceosome in circular RNA biogenesis
The requirement of the spliceosome in circRNA formation has been investigated by mutation of canonical splice signals in the form of both the 3′ and 5′ splice sites joined upon circularization, as well as putative branch points (BPs) and the polypyrimidine tract (PPT). Mutation of either the 3′ or the 5′ splice site flanking the exon to be circularized often lead to utilization of new cryptic splice sites,Citation16 but was in some cases shown to decrease circRNA expression.Citation35,36 However, although cryptic splice sites might support circRNA formation upon removal of stronger splice sites, intronic cryptic 5′ splice sites located upstream of the 5′ splice site used in backsplicing may also inhibit the circRNA production, presumably through competition for recognition.Citation35 BP optimization did not increase circRNA production, and only very extensive inactivation of potential BP adenosine candidates disturbed the circRNA splicing pattern.Citation16 In contrast, weakening the PPT caused a marked decrease in the circRNA level.Citation16 Furthermore, by blocking spliceosome assembly it was revealed that synthesis of the examined circRNAs diminished after spliceosome inhibition.Citation16 Hence, production of circRNAs seems to involve the spliceosome and may be viewed as a novel type of alternative splicing.Citation37 Nonetheless, if and how the spliceosome or any of the many splicing factors that direct spliceosome assembly discriminate between linear splicing and backsplicing is still vastly unknown. Nonetheless, research into putative circRNA biogenesis mechanisms has uncovered a plethora of elements capable of modulating circRNA formation. Although the mechanism in which these elements influence circRNA biogenesis is not fully characterized, 3 not mutually exclusive pathways for how circRNA production is facilitated ( and ),Citation38 have been proposed based on a positive effect of (1) flanking inverted repeats (2) RBP binding and (3) exon skipping on circRNA expression and will be reviewed in detail below. As the mechanistic aspects of circRNA induction performed by flanking inverted repeats and RBP binding are very similar, these are here grouped as one under the direct backsplicing category ().
Inverted repeats can promote circRNA expression
The presence of inverted repeats situated on either side of the exon(s) subject to backsplicing and base-pairing between these is believed to promote circularization rather than linear splicing due to the induced proximity between the splice signals involved in backsplicing ().Citation12,13,18,35,39 The potential prevalence of this mechanism is high as the presence of inverted repeats in the flanking introns appear to be a prevalent feature of circRNAs expressed in brain and conserved across human, pig and mouse.Citation10,21 Furthermore, enrichment of complementary sequence motifs in the flanking introns is one of the recurring features associated with circRNAs in metazoans.Citation12,13,18 Analysis of the types of intronic motifs in metazoans that can influence circularization has revealed that both non-repetitiveCitation12,18 and repetitive elementsCitation12,18,35 may act to stimulate circularization. Specifically in humans, the repetitive Alu elements could be a major contributor to circRNA biogenesis as it is significantly enriched in introns flanking exons undergoing circularization.Citation13 Furthermore, in an analysis of all repeats flanking circRNA-generating exons, 88% of the identified complementary regions overlapped Alu elements.Citation18 The length required for a complementary inverted repeat to induce backsplicing seems to be context dependent and range from more than 15 kb endogenouslyCitation26,39 to a minimal requirement of ∼30–40 nucleotides in mini-gene setups.Citation35 The extent to which circRNA formation is induced by these inverted repeats appears to depend on both the stability of the intramolecular interaction between the inverted repeats and the sequence composition of the complementary regions.Citation35 Furthermore, competitive base-pairing between different pairs of complementary regions may influence backsplicing efficiency, as evidenced by the finding that expression of a circRNA was reduced when both inter-intronic and intra-intronic pairing was allowed compared with a situation in which only inter-intronic base-pairing could take place ().Citation12 In addition to the length and number of repeat regions, it could be imagined that the distance from the inverted element to the circularized exon might also influence circRNA production efficiency but this has yet to be determined.
Figure 2. Modulators of circRNA biogenesis. (A) Competitive base-pairing between multiple pairs of inverted repeats can modulate circRNA formation as only base-pairing between IRs across an exon (inter-intronic pairing, up) promotes circRNA formation whereas intra-intronic base-pairing does not (down). The 2 mutually exclusive base-pairing patterns are indicated with arrows in the middle panel. (B) Additionally, when dependent on intron-pairing, the efficiency with which circRNAs are formed may be modulated by the activity of the RNA editing enzyme ADAR 1 that acts as an inhibitor of circRNA formation by mediating the conversion of adenosines (A) to inosines (I) thereby destabilizing the base-pairing that promotes backsplicing. (C) Splicing factors, both tissue specific and general, can affect circRNA expression and may either inhibit or promote the expression of a circRNA. (D) CircRNA formation is affected by the rate of transcription, with a high transcriptional elongation rate being favorable for circRNA expression.
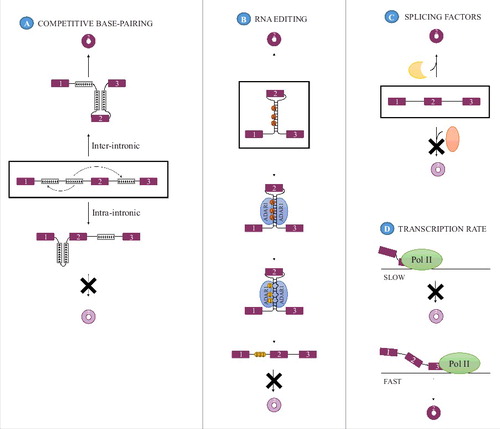
Experimental support for the intron pairing model has mainly been derived from analysis of circRNA formation from mini-gene constructs that only encompass the exon(s) to be circularized and portions of the flanking regions,Citation12,35,36,40 as human genes are generally too large to be ectopically expressed.Citation37 However, the finding that the endogenous GCN1L1Citation12 and HIPK3 locusCitation22 produce circRNA from an exon surrounded by complementary regions and that disruption of presumed inter-intronic base-pairing using CRISPR/Cas9 technology at these loci selectively abolished circRNA production,Citation20,22 confirmed that intron pairing-driven circularization may indeed prevail at endogenous loci.
RNA editing can impair intron-pairing dependent circRNA expression
Although intron pairing is an appealing biogenesis model, pairing between complementary repeats was until recently not experimentally verified and does not by itself explain the observed tissue or developmental stage specific expression of circRNAsCitation1,10,11 because it is an intrinsic structural element that is present regardless of the cell type analyzed. However, evidence that intron pairing does take place and that its formation can be regulated was derived from the discovery that the RNA editing enzyme adenosine deaminase acting on RNA-1 (ADAR1) that binds to double stranded RNA regions and converts adenosines to inosinesCitation41,42 works as an antagonist of circRNA production.Citation10,18 This was evident as depletion of ADAR1 in 3 different human and mouse cell lines lead to an increase in the production of a subset of circRNAs without a similar expression change in the accompanying linear variant,Citation10,18 and consistent with the observation that decreased RNA editing in Drosophila lead to increased circRNA steady-state levels. Moreover, editing of the flanking introns correlated negatively with the steady-state level of one examined circRNA during neuronal development.Citation10 and introns flanking circRNA-producing exons displayed increased A-to-I editing relative to control exons, specifically in the regions 200–600 nucleotides away from the splice sites in both humans and C.elegans, providing evidence for base-pairing between these regions in vivo.Citation18 Based on these data, the current hypothesis is that nuclear editing performed by ADAR1 before backsplicing may destabilize pairing between complementary motifs present in the introns flanking the exons that can undergo circularization, thereby selectively preventing the biogenesis of a subset of circRNAs depending on such base-pairing for production (). However, impaired circRNA biogenesis could be caused by indirect effects of ADAR1.Citation10,18
RNA binding proteins can promote circRNA formation
Although mechanistically similar to backsplicing driven by cis-acting intron-pairing (), backsplicing can also be promoted by trans-acting factors in the form of RNA binding proteins (RBPs). Upon binding of such RBPs to introns flanking the exon(s) to be circularized, the intervening RNA stretch may be looped out, out upon dimerization of the RBPs, thereby inducing proximity between the splice signals involved in backsplicing ().Citation7,24 Hence, in opposition to ADAR1 discussed above that antagonizes circRNA formation, the RBPs discussed in this section act to facilitate circularization. The experimental evidence of this backsplicing mechanism stems from the investigation of 2 tissue specific splicing factors: Muscleblind (MBL) and Quaking (QKI).
Drosophila MBL was shown to enhance formation of a circRNA from its own gene, circMbl, as well as 2 other circRNAs in a manner dependent on the presence of binding sites for MBL in the introns flanking the circularized exon(s). Given that MBL is able to dimerize, and that no backsplicing was evident unless MBL binding sites were present in both flanking introns, MBL pairing across the introns was proposed as the mechanism of circularization.Citation7 The other tissue specific splicing factor known to be involved in circRNA biogenesis, QKI, was shown to act as an enhancer of circRNA production during epithelial-to-mesenchymal transition (EMT) in immortalized human mammary epithelial cells (HMEC). Consequently, QKI knockdown (KD) reduced/abolished upregulation of 1/3 of the 300 most abundant circRNAs during this transition. The stimulation of circRNA production was isoform specific, correlated with QKI level, and was only observed when QKI binding sites were present in both the upstream and the downstream intron. Similar to MBL, QKI acts as a dimer, leading to the same type of hypothesis with regard to circularization mechanism as for MBL.Citation24 However, direct evidence that these tissue-specific splicing factors pair across the intron has not been presented. Hence, it is possible that although the action of the splicing factor is necessary, it is not sufficient to facilitate splice site proximity.
Regulation of circular RNA expression by general splicing factors
In addition to the RBPs that promote or antagonize circRNA formation and for which a suggested mode of action is in place, other trans-acting factors have also been implicated in circRNA biogenesis, such as various SR proteins, hnRNPs and the tissue-specific splicing factor RBM20.Citation40 These may act either to promote or to prevent circRNA formation (),Citation36,40,43 but their mechanism of action and whether they are connected to a specific mode of biogenesis has not yet been determined, although it has been suggested that RBM20 facilitates circRNA formation through a lariat-driven mechanismCitation43 Nevertheless, it is evident that different subsets of splicing factors regulate expression of distinct circRNAs,Citation40,43 in a fashion similar to that of conventional alternative splicing. Moreover, simultaneous depletion of 2 splicing factors had an additive effect on circularization in most but not all combinations tested, indicative of non-redundant roles for each of these splicing factors.Citation40 However, it was not examined whether the splicing factors acted directly on the circRNA precursor or whether the effect was indirect. In another study, engineered insertion of exonic cis-elements known to attract 4 specific splicing factors into a mini-gene vector and co-expression with each splicing factor was used to show that circRNA production from a mini-gene was altered when the splicing factor was combined with the cognate cis-element.Citation36 However, the pattern of regulation was generally complex with sequence independent effects on circRNA but not linear RNA production. Furthermore, the splicing factors had distinct and even opposing function on circRNA production compared with linear RNA generation.Citation36 Hence, regulation of circRNA expression seems to be governed by combinatorial recognition of a unique set of binding motifs in and around the exons giving rise to circRNA in a complex manner.Citation36,40
Circular RNA biogenesis can be driven by exon skipping
A third potential pathway for circRNA generation is initiated by an exon skipping event that generates an exon-containing lariat (). Due to the structural limitations imposed by a lariat shape, the splice signals necessary for backsplicing are then brought into closer proximity of each other, potentially facilitating backsplicing mediated by the spliceosome.Citation13 However, although there are exceptions, lariats are generally not stable but degraded rapidly through the action of debranching enzymes and exonucleases once spliceosomal components are released from the lariat intron.Citation44 Hence, in order for circRNAs to be generated through this pathway, backsplicing has to precede debranching, as linearization of the lariat structure would abrogate the lariat-induced proximity between the splice signals necessary for backsplicing. The mechanism(s) in which intra-lariat backsplicing could be promoted or preferentially selected over debranching is undetermined at the moment. However, a 7 nt GU-rich element close to the 5′ splice site along with a 11 nt C-rich motif in the vicinity of the intronic branch point have been shown to be important for avoiding debranchingCitation45 and could be one way to stall this process long enough for backsplicing to take place.
With regard to the prevalence of this mechanism, sporadic association of exon skipping with circRNA generation has been observed in endogenous loci,Citation30,31,46,47 but only few global studies on the link between circRNA producing exons and exon skipping have been conducted.Citation13,23 Kelly et al. used exon skipping events as reference and found that a corresponding circRNA containing the skipped exon(s) could be identified for most single and multi-exon skipping events. Conversely, Jeck and colleagues used detected circRNAs as a reference to search for linear transcripts in which the exons giving rise to a circRNA was absent and found that this was the case for 45% of all circRNAs analyzed,Citation13 indicating that although a subset of circRNAs may be connected to exon skipping, this may not apply to all circRNAs. However, given that correlation does not necessarily confer causality, the observed production of circRNAs and skipped exons could be uncoupled events. Furthermore, a concern with these types of analyses is that the validity of the conclusions depends on the assumption that the linear skipped transcript should be stable enough to be detected. However, as approximately 1/3 of all alternative splicing events give rise to an RNA variant with a premature termination codon (PTC) that may be substrate for nonsense mediated decay (NMD),Citation48 these linear skipped transcripts might be rapidly degraded. Additionally, a linear skipped product could conceivably be generated through all 3 biogenesis pathways,Citation5 ( and ). Therefore, the finding of a circRNA with a corresponding linear skipped product is not necessarily indicative of a lariat-driven circularization event but could also be because of a direct backsplicing event. Nonetheless, in Schizosaccharomyces pombe, mutation of the 5′ splice site in the intron upstream of the circRNA exon or the BP in the intron downstream of the circRNA exon in the debranching mutant strain both prevented formation of the exon-containing lariat and the circRNA. As these sites are only involved in backsplicing if it proceeds through the exon-skipping pathway but not if it proceeds through the intron pairing or the RBP pairing pathway,Citation37 this indicates that exon skipping may contribute to circRNA formation through a lariat-driven pathway at least in some organisms. In fact, distinct species may have adopted different primary biogenesis pathways to generate circRNAsCitation37 as illustrated by the observation that intron pairing-driven backsplicing, although seemingly prevalent in metazoans, may not be a mechanism of choice in non-metazoan eukaryotes as repeat sequences are often relatively rare in these species but circRNAs are still widely expressed.Citation6,37
Comparison of the pathways that promote circRNA expression
A common feature of all the circRNA-promoting pathways suggested to date is that the splice sites that are backspliced should be brought into proximity of each other. However, the models differ as to the way in which this proximity is induced and at what stage backsplicing takes place. The intron pairing-driven and RBP pairing-driven circRNA biogenesis differ only in what means are used to achieve the appropriate splice site proximity (direct base pairing between complementary motifs versus interaction between the splicing factors bound to separate motifs) (). With regard to the stage, direct backsplicing events can precede or be concurrent with the linear splicing event joining the flanking exons. However, it cannot be excluded that RBP or intron pairing might also promote backsplicing after a linear exon skipping alternative splicing event has taken place. The lariat-driven circularization differs from the other 2 models in that no specific sequence elements are necessary to induce the restricted circRNA promoting structure apart from the ones needed to prompt exon skipping. Furthermore, a prior splicing event is essential for backsplicing to take place in the lariat-driven circularization model. As both RNA secondary structure and RBP-mediated intron looping are known to affect the rate of exon skipping,Citation49-52 these elements might be involved in promoting circRNA biogenesis through a lariat driven pathway in addition to promoting circRNA biogenesis by stimulating direct backsplicing, highlighting the interconnectedness of the proposed circRNA biogenesis models. At least when analyzing mini-gene constructs both inverted repeats and splicing factors may all regulate backsplicing efficiency.Citation40 Hence, the reviewed biogenesis models may be very simplified versions of the complex regulatory system that governs circRNA expression.
Even though the biogenesis models differ with regard to the exact nature and sequence of splicing events, all 3 pathways are, at least theoretically, capable of producing a circRNA as well as a linear RNA in which the circularized exons are absent, thereby concomitantly generating a double-lariat structure that is presumably degraded ( and ). However, unless the 2 distinct splicing reactions necessary to produce both a linear and a circRNA takes place concurrently, exon-containing splicing intermediates with branch point junctions that are not immediately resolved in a subsequent splicing step are generated in all circRNA biogenesis pathways ( and ). This is in contrast to conventional linear splicing where all such exon-containing splicing intermediates with branch point junctions are resolved in the subsequent splicing step (). Hence, the intermediates generated during backsplicing might be more susceptible to degradation than those generated during linear splicing as the window of opportunity for release of spliceosomal factors from the BP junction, recognition of these BPs by the debranching enzyme and recruitment of nucleases is increased compared with linear splicing. If this degradation of intermediates does take place, it could prevent the concurrent generation of both a linear and a circRNA from the same primary transcript. Since the sequence of events differ between the biogenesis pathways, the nature of the reaction intermediate that is most sensitive to degradation during the backsplicing process also differs. Hence, in the lariat driven backsplicing model, the circRNA lariat intermediate () is the one disposed to debranching and nucleolytic degradation, whereas it is the linear RNA intermediate () in the direct backsplicing mechanism that is susceptible to debranching and nucleolytic degradation. Hence, lariat-driven circRNA formation requires a positive correlation between circRNA and linear RNA formation (unless the linear RNA is an NMD substrate), whereas circRNA formation through direct backsplicing can be more loosely coupled to linear RNA production.
Circular RNA biogenesis is regulated by the rate of transcription
Besides cis- and trans-acting factors, the transcriptional elongation rate has also been shown to have an impact on circRNA expression. Studies on circRNA formation using 4sU labeling to identify newly synthesized RNA established that circular RNA producing genes are generally longer and are transcribed at a faster rate (2.9 kb/min) than non-circRNA producing genes (2.29 kb/min).Citation20 Additionally, use of either a standard or one of 2 mutant versions of RNA polymerase II (slow or fast) established that backsplicing efficiency correlated positively with elongation rate ().Citation20 This observation complies with the results of the same type of analysis conducted in Drosophila using a mutated RNA polymerase II with a slow elongation rate showing that slowing down transcription decreased circRNA levels.Citation7 Based on this, one could argue that circRNA generation is in competition with formation of canonical linear transcripts and that the rate of transcription may impact which type of event is favored.Citation7 Interestingly, that fast polymerase II elongation correlates with circRNA biogenesis could comply with all 3 biogenesis models. Firstly, fast transcription enhances the probability of inter-intronic base pairing,Citation20,53 consistent with pairing across introns due to the presence of inverted repeats. Secondly, RBP binding motifs present in the downstream intron would also be revealed faster, supporting that RBP-pairing might also occur more frequently with fast transcription. Lastly, transcriptional elongation rate has also been known to impact the rate of exon skipping, with slow transcription shown both to be able to inhibitCitation54,55 and promoteCitation55,56 skipping depending on the context and specific splice signals and regulatory motifs present.
Co- or post-transcriptional generation of circular RNAs?
Although it has been determined that circRNA biogenesis can be modulated by transcriptional elongation rate, whether backsplicing predominantly is a co- or post-transcriptional event has not yet been finally determined. Using mini-genes, it has been demonstrated that features within the flanking introns may determine whether backsplicing takes place co- or post-transcriptionally.Citation40 Hence, although functional 3′ end processing was required for circularization when only very short inverted repeats (∼40 nt) were present, indicative of post-transcriptional processing,Citation35 extension of these repeats allowed processing of the circRNA to take place independently of 3′ end processing,Citation40 pointing to a co-transcriptional backsplicing mechanism.
Transcriptome-wide analyses of nascent RNA have also been used to distinguish between co- and post-transcriptional processing. As circRNAs could be detected in samples of nascent RNA isolated from Drosophila fly heads, this was taken as evidence in favor of co-transcriptional processing.Citation7 However, the chromatin fraction analyzed by Ashwal-Fluss et al. also includes transcripts with mature polyadenylated 3′ ends and may therefore not exclusively consist of nascent RNA.Citation53,57 A separate analysis of nascent RNA labeled with 4-thiouridine (4sU) in mammalian PA1 cells supported that in vivo the majority of circRNAs are processed post-transcriptionally. This was inferred as only a very small number of circRNAs were detectable after short 4sU labeling periods (10–15 minutes), suggestive of co-transcriptional processing. In contrast an increased number of circRNAs was detected when labeling extended beyond the point where all circRNA producing genes had been transcribed to their termini, which was taken as an indicator of post-transcriptional processing.Citation20 However, on a cautionary note, as the cells in this experiment were treated with 5,6-dichloro-1-β-d-ribofuranosylbenzimidazole (DRB) for 3 hours to arrest RNA polymerase II elongation,Citation20 transcription and processing events, including circRNA biogenesis might be biased by this disruption of the cellular balance.
Functions of circular RNAs
Whereas clues with regard to the biogenesis of circRNA are emerging, functional classification of circRNAs is still at a very early stage. However, some circRNAs have been demonstrated to interact with the miRNA-Argonaute 2 (Ago2) complexCitation1,8,22 and seem to inhibit miRNA function by redirecting the miRNAs for which the circRNA possesses binding sites away from other targets (sponging) (). Since miRNAs through sequence mediated recognition of targets are important regulators of gene expression by suppressing translation and enhancing exonucleolytic decay of mRNAsCitation58, circRNA-mediated regulation may affect the expression of miRNA targets. As circRNAs themselves are inherently resistant to miRNA mediated exonucleolytic decay given that they possess no free ends, they are believed to be very efficient miRNA inhibitors capable of sequestering the miRNAs.Citation1,4,8 Although at least 2 circRNAs, ciRS-7Citation1,8 and Sry circRNA,Citation8 possess an abundant number of miRNA target sites for one or more specific miRNAs, this principle does not appear to be common to many circRNAs.Citation4,5 Whether the circRNAs with a smaller number of miRNA binding sites per miRNA function as miRNA regulators awaits disclosure. However, at least one very abundant circRNA, circHIPK3, seems to function as a regulator of miR-124 even though it only possesses 2 binding sites for this miRNA,Citation22 indicating that circRNAs may act as miRNA regulators despite a limited number of miRNA binding sites. Furthermore, circRNAs might target multiple miRNAs involved in the same process and thereby potentially circumvent the need for many binding sites for a single miRNA, as illustrated by circHIPK3 that possesses 18 miRNA binding sites for 9 miRNAs, all known to suppress growth.Citation22
Figure 3. Functions of circular RNAs. In the cytoplasm, circRNAs can interact with miRNA-Ago2 complexes to inhibit miRNA action on linear targets (A). Additionally, circRNAs can interact with RNA-binding proteins (RBPs) as can linear RNAs and may either facilitate interaction between RBPs to inactivate/activate them (B) or sequester these to prevent them from functioning (C). In the nucleus, (D) circRNAs with retained introns can promote transcription of their parental gene by interacting with RNA polymerase II (Pol II) and U1 snRNP at the promoter of the gene. (E) During splicing, the elements facilitating backsplicing and linear splicing compete with each other. As a result of this competition either a linear RNA or an alternatively spliced linear RNA and a circRNA is generated. Hence, the backsplicing pattern may alter the expression of the linear gene product.
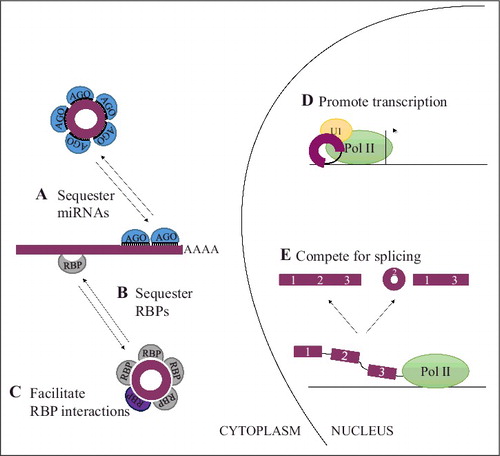
Additionally, circRNAs have also been shown to interact with RNA-binding proteinsCitation7,59-61 and might function as a scaffold to facilitate protein interactions, regulate protein function or to sequester the bound protein depending on the specific circRNA-protein combination (). Furthermore, through interactions with U1 small nuclear RNA (snRNA), RNA polymerase II and the promoter of their parental gene, 2 circRNAs with retained introns have been shown to accumulate in the nucleus and promote transcription of their parental gene ().Citation9 However, no analysis of whether the observed increased transcription was translated into increased protein expression from these genes was performed.
Aside from regulating their parental gene, one circRNA, ciRS-7, appears to take part in a feedback loop that involves the protein coding CDR1 transcript that is derived from a locus located antisense to ciRS-7.Citation3 However, it is questionable whether this mechanism is relevant endogenously as ciRS-7 seems to be the most abundant species produced from this locus with only limited evidence for CDR1 expression.Citation3 In fact functions previously ascribed to CDR1 may be enclosed in ciRS-7.Citation62
In addition to functions carried out after the circRNA has been generated, the production of the circRNA may in itself function to regulate linear RNA expression from the same gene ().Citation5,7,15 This is because the majority of circRNAs are derived from exonic regions within protein coding genes and because the exon(s) incorporated into the circRNA would not be a part of any potential linear skipped transcript derived from the same primary transcript as the circRNA. Hence, particularly for the subset of circRNAs that contain the translational start codon,Citation5,7 it is easy to imagine how circRNA production may directly affect protein expression from the parental gene, as this would remove the translational start codon from the corresponding linear transcript. Nonetheless, removal of other exons from the linear transcript during alternative splicing leading to circRNA formation may give rise to transcript variants that could be recognized by RNA surveillance apparatuses and degradedCitation63 or give rise to proteins with an altered function.
The exact relationship between circRNAs and the linear counterparts at large are mostly uncharacterizedCitation64 and would be an interesting avenue for future research. However, circRNA synthesis is generally less efficient than linear RNA synthesisCitation20 and it is believed that the majority of circRNAs mainly accumulate to detectable levels due to their extraordinary stability.Citation13,20 This implies that the linear RNA splicing patterns most often outcompete backsplicing. Hence, based on the current data most circRNAs do not appear to impact linear RNA expression through competitive splicing. Nonetheless, circRNAs contain a unique potential for regulation of linear gene expression both through production of the circRNA and through functions performed after the circRNA has been generated, as has been proposed for circMbl in Drosophila.Citation7
In addition to these validated functions, several other putative modes of action have been suggested for circRNAs, including transport vehicles and long-term sensors.Citation5,10,15,24,65 One of the most intriguing possibilities concerns the subset of circRNAs that possess the translational start codon and suggests that they might function as templates for protein synthesis. However, although insertion of internal ribosomal entry sites (IRES) elementsCitation24,36,66 or a prokaryotic ribosome binding siteCitation67 can lead to translatable circRNAs, no evidence of endogenous circRNA translation has been uncovered to date.Citation4,5,13,60,68
Unexplored areas of circular RNA biology: Nuclear export and degradation
Whereas the biogenesis of circRNAs is subject to investigation and clues are emerging as to the function of circRNAs, no headway has been made as to how circRNAs are exported from the nucleus to the cytoplasm where the majority of circRNAs reside. As the location of the circRNA determines which functions can be exerted and what cellular milieu the circRNA is exposed to, it will be important to determine the export mechanism. Potential mechanisms for export include nuclear escape during mitosis or regulated export, for instance through deposition of an exon-junction complex during splicing, which may stimulate export.Citation5,15 Moreover, whether the mechanism that keeps the found intron-containing circRNAs (EIcircRNAs) localized to the nucleus is linked to intron retention, as observed for mRNAs,Citation69,70 could be examined. Interestingly, some circRNAs exhibit developmentally regulated nucleo-cytoplasmic localization suggesting that an active nuclear export mechanism for circRNAs exist.Citation21 Additionally, the exact degradation route for circRNAs remain largely uncharacterized but seeing as circRNAs appear to accumulate to detectable levels despite a low synthesis rateCitation20 this implies that degradation is very inefficient. However, endonucleolytic Ago2-miRNA-mediated cleavage appears to be one approachCitation3,8 to initiate degradation. Nonetheless, the prevalence of Ago2-miRNA mediated endonucleolytic cleavage of circRNAs in general has not yet been reported. The possibility of other endonucleasesCitation71 such as the Dis3/Dis3L exosome proteins involved in mRNA turnoverCitation72,73 being important for circRNA degradation is also currently undisclosed.
Concluding remarks
Although the research conducted to date does provide insights into circRNA biology, many questions regarding circRNAs have yet to be answered. Therefore, continued research into the biogenesis of circRNAs to uncover the rules that govern circRNA generation is necessary. It will also be of importance to determine the mechanisms used to ensure the degradation of circRNAs to gain complete insights into the metabolism of circRNAs. Furthermore, additional research into the functions of circRNAs is essential for the field as well as a clarification of the nuclear export mechanism for circRNAs. Moreover, given the proposed model of circRNAs acting as transport vehiclesCitation62,65 a determination of the general movements of circRNAs within the cells and a determination of potential transport cargo could be exciting new avenues of research. To facilitate this research, new tools to study circRNAs would be advantageous. To date, a large number of the studies conducted take advantage of a mini-gene construct containing only the exon to be circularized and parts of the flanking introns to express a circRNA of interest,7,8,12,35,37 as human genes are generally too large to be ectopically expressed.Citation37 However, although often used as a very convenient tool that allows overexpression of circRNAs and easy manipulation of the sequences incorporated into the vector, facilitating studies of circRNA function and biogenesis respectively, technical limitations are a concern with this approach. For instance, additional linear and circRNA products are often produced in addition to the expected one.Citation35,37,40 These products are problematic both because it means that biogenesis of the wanted circRNA in the mini-gene setup might not mimic the endogenous system and because studies of circRNA function may be precluded by functions exerted by these aberrant productsCitation74 To expand the utility of these vectors, mini-gene design should be improved to eliminate these and although vectors for circRNA expression that increase the ratio of circRNA-to-unwanted-product have been generated,Citation40 none that only give rise to circRNA have been published. Hence, to further research into circRNAs, mini-gene construct design should be improved and/or alternative methods to produce circRNAs in vitroCitation75 could be used to facilitate circRNA overexpression and functional studies. Additionally, the application of CRISPR/Cas9 to manipulate genomic regions, which allows circRNA biogenesis to be studied at their endogenous lociCitation20,22 instead of using mini-gene constructs, has been initiated. This approach permits a more reliable analysis of factors influencing circRNA biogenesis as the complex interplay of transcription and processing in the natural environment is taken into account and is likely to enhance insights into the biogenesis of circRNAs.
Disclosure of potential conflicts of interest
No potential conflicts of interest were disclosed.
Funding
This work was supported by The Danish Council for Independent Research (DFF) and the Novo Nordisk Foundation (NNF16OC0019874 to TBH).
References
- Memczak S, Jens M, Elefsinioti A, Torti F, Krueger J, Rybak A, Maier L, Mackowiak SD, Gregersen LH, Munschauer M, et al. Circular RNAs are a large class of animal RNAs with regulatory potency. Nature 2013; 495:333-8; PMID:23446348; https://doi.org/10.1038/nature11928
- Salzman J, Gawad C, Wang PL, Lacayo N, Brown PO. Circular RNAs are the predominant transcript isoform from hundreds of human genes in diverse cell types. PLoS One 2012; 7:e30733; PMID:22319583; https://doi.org/10.1371/journal.pone.0030733
- Hansen TB, Wiklund ED, Bramsen JB, Villadsen SB, Statham AL, Clark SJ, Kjems J. miRNA-dependent gene silencing involving Ago2-mediated cleavage of a circular antisense RNA. EMBO J 2011; 30:4414-22; PMID:21964070; https://doi.org/10.1038/emboj.2011.359
- Guo JU, Agarwal V, Guo H, Bartel DP. Expanded identification and characterization of mammalian circular RNAs. Genome Biol 2014; 15:409; PMID:25070500; https://doi.org/10.1186/s13059-014-0409-z
- Jeck WR, Sharpless NE. Detecting and characterizing circular RNAs. Nat Biotechnol 2014; 32:453-61; PMID:24811520; https://doi.org/10.1038/nbt.2890
- Wang PL, Bao Y, Yee MC, Barrett SP, Hogan GJ, Olsen MN, Dinneny JR, Brown PO, Salzman J. Circular RNA is expressed across the eukaryotic tree of life. PLoS One 2014; 9:e90859; PMID:24609083; https://doi.org/10.1371/journal.pone.0090859
- Ashwal-Fluss R, Meyer M, Pamudurti NR, Ivanov A, Bartok O, Hanan M, Evantal N, Memczak S, Rajewsky N, Kadener S. circRNA Biogenesis Competes with Pre-mRNA Splicing. Mol Cell 2014; 56:55-66; PMID:25242144; https://doi.org/10.1016/j.molcel.2014.08.019
- Hansen TB, Jensen TI, Clausen BH, Bramsen JB, Finsen B, Damgaard CK, Kjems J. Natural RNA circles function as efficient microRNA sponges. Nature 2013; 495:384-8; PMID:23446346; https://doi.org/10.1038/nature11993
- Li Z, Huang C, Bao C, Chen L, Lin M, Wang X, Zhong G, Yu B, Hu W, Dai L, et al. Exon-intron circular RNAs regulate transcription in the nucleus. Nat Struct Mol Biol 2015; 22:256-64; PMID:25664725; https://doi.org/10.1038/nsmb.2959
- Rybak-Wolf A, Stottmeister C, Glažar P, Jens M, Pino N, Giusti S, Hanan M, Behm M, Bartok O, Ashwal-Fluss R, et al. Circular RNAs in the Mammalian Brain Are Highly Abundant, Conserved, and Dynamically Expressed. Mol Cell 2015; 58:870-85; PMID:25921068; https://doi.org/10.1016/j.molcel.2015.03.027
- Salzman J, Chen RE, Olsen MN, Wang PL, Brown PO. Cell-type specific features of circular RNA expression. PLoS Genet 2013; 9:e1003777; PMID:24039610; https://doi.org/10.1371/journal.pgen.1003777
- Zhang XO, Wang HB, Zhang Y, Lu X, Chen LL, Yang L. Complementary sequence-mediated exon circularization. Cell 2014; 159:134-47; PMID:25242744; https://doi.org/10.1016/j.cell.2014.09.001
- Jeck WR, Sorrentino JA, Wang K, Slevin MK, Burd CE, Liu J, Marzluff WF, Sharpless NE. Circular RNAs are abundant, conserved, and associated with ALU repeats. RNA 2013; 19:141-57; PMID:23249747; https://doi.org/10.1261/rna.035667.112
- Zhang XO, Dong R, Zhang Y, Zhang JL, Luo Z, Zhang J, Chen LL, Yang L. Diverse alternative back-splicing and alternative splicing landscape of circular RNAs. Genome Res 2016; 26:1277-87; PMID:27365365; https://doi.org/10.1101/gr.202895.115
- Lasda E, Parker R. Circular RNAs: diversity of form and function. RNA 2014; 20:1829-42; PMID:25404635; https://doi.org/10.1261/rna.047126.114
- Starke S, Jost I, Rossbach O, Schneider T, Schreiner S, Hung LH, Bindereif A. Exon circularization requires canonical splice signals. Cell Rep 2015; 10:103-11; PMID:25543144; https://doi.org/10.1016/j.celrep.2014.12.002
- Danan M, Schwartz S, Edelheit S, Sorek R. Transcriptome-wide discovery of circular RNAs in Archaea. Nucleic Acids Res 2012; 40:3131-42; PMID:22140119; https://doi.org/10.1093/nar/gkr1009
- Ivanov A, Memczak S, Wyler E, Torti F, Porath HT, Orejuela MR, Piechotta M, Levanon EY, Landthaler M, Dieterich C, et al. Analysis of intron sequences reveals hallmarks of circular RNA biogenesis in animals. Cell Rep 2015; 10:170-7; PMID:25558066; https://doi.org/10.1016/j.celrep.2014.12.019
- Westholm JO, Miura P, Olson S, Shenker S, Joseph B, Sanfilippo P, Celniker SE, Graveley BR, Lai EC. Genome-wide analysis of drosophila circular RNAs reveals their structural and sequence properties and age-dependent neural accumulation. Cell Rep 2014; 9:1966-80; PMID:25544350; https://doi.org/10.1016/j.celrep.2014.10.062
- Zhang Y, Xue W, Li X, Zhang J, Chen S, Zhang JL, Yang L, Chen LL. The Biogenesis of Nascent Circular RNAs. Cell Rep 2016; 15:611-24; PMID:27068474; https://doi.org/10.1016/j.celrep.2016.03.058
- Venø MT, Hansen TB, Venø ST, Clausen BH, Grebing M, Finsen B, Holm IE, Kjems J. Spatio-temporal regulation of circular RNA expression during porcine embryonic brain development. Genome Biol 2015; 16:245; PMID:26541409; https://doi.org/10.1186/s13059-015-0801-3
- Zheng Q, Bao C, Guo W, Li S, Chen J, Chen B, Luo Y, Lyu D, Li Y, Shi G, et al. Circular RNA profiling reveals an abundant circHIPK3 that regulates cell growth by sponging multiple miRNAs. Nat Commun 2016; 7:11215; PMID:27050392; https://doi.org/10.1038/ncomms11215
- Kelly S, Greenman C, Cook PR, Papantonis A. Exon Skipping Is Correlated with Exon Circularization. J Mol Biol 2015; 427(15):2414-7; PMID:25728652; https://doi.org/10.1016/j.jmb.2015.02.018
- Conn SJ, Pillman KA, Toubia J, Conn VM, Salmanidis M, Phillips CA, Roslan S, Schreiber AW, Gregory PA, Goodall GJ. The RNA Binding Protein Quaking Regulates Formation of circRNAs. Cell 2015; 160:1125-34; PMID:25768908; https://doi.org/10.1016/j.cell.2015.02.014
- Dang Y, Yan L, Hu B, Fan X, Ren Y, Li R, Lian Y, Yan J, Li Q, Zhang Y, et al. Tracing the expression of circular RNAs in human pre-implantation embryos. Genome Biol 2016; 17:130; PMID:27315811; https://doi.org/10.1186/s13059-016-0991-3
- Capel B, Swain A, Nicolis S, Hacker A, Walter M, Koopman P, Goodfellow P, Lovell-Badge R. Circular transcripts of the testis-determining gene Sry in adult mouse testis. Cell 1993; 73:1019-30; PMID:7684656; https://doi.org/10.1016/0092-8674(93)90279-Y
- Cocquerelle C, Mascrez B, Hétuin D, Bailleul B. Mis-splicing yields circular RNA molecules. FASEB J 1993; 7:155-60; PMID:7678559
- Nigro JM, Cho KR, Fearon ER, Kern SE, Ruppert JM, Oliner JD, Kinzler KW, Vogelstein B. Scrambled exons. Cell 1991; 64:607-13; PMID:1991322; https://doi.org/10.1016/0092-8674(91)90244-S
- Pasman Z, Been MD, Garcia-Blanco MA. Exon circularization in mammalian nuclear extracts. RNA 1996; 2:603-10; PMID:8718689
- Surono A, Takeshima Y, Wibawa T, Ikezawa M, Nonaka I, Matsuo M. Circular dystrophin RNAs consisting of exons that were skipped by alternative splicing. Hum Mol Genet 1999; 8:493-500; PMID:9949208; https://doi.org/10.1093/hmg/8.3.493
- Zaphiropoulos PG. Circular RNAs from transcripts of the rat cytochrome P450 2C24 gene: correlation with exon skipping. Proc Natl Acad Sci U S A 1996; 93:6536-41; PMID:8692851; https://doi.org/10.1073/pnas.93.13.6536
- Lee Y, Rio DC. Mechanisms and Regulation of Alternative Pre-mRNA Splicing. Annu Rev Biochem 2015; 84:291-323; PMID:25784052; https://doi.org/10.1146/annurev-biochem-060614-034316
- Will CL, Lührmann R. Spliceosome structure and function. Cold Spring Harb Perspect Biol 2011; 3:a003707; PMID:21441581; https://doi.org/10.1101/cshperspect.a003707
- Matera AG, Wang Z. A day in the life of the spliceosome. Nat Rev Mol Cell Biol 2014; 15:108-21; PMID:24452469; https://doi.org/10.1038/nrm3742
- Liang D, Wilusz JE. Short intronic repeat sequences facilitate circular RNA production. Genes Dev 2014; 28(20):2233-47; PMID:25281217; https://doi.org/10.1101/gad.251926.114
- Wang Y, Wang Z. Efficient backsplicing produces translatable circular mRNAs. RNA 2015; 21:172-9; PMID:25449546; https://doi.org/10.1261/rna.048272.114
- Barrett SP, Wang PL, Salzman J. Circular RNA biogenesis can proceed through an exon-containing lariat precursor. Elife 2015; 4:e07540; PMID:26057830; https://doi.org/10.7554/eLife.07540
- Ebbesen KK, Kjems J, Hansen TB. Circular RNAs: Identification, biogenesis and function. Biochim Biophys Acta 2016; 1859:163-8; PMID:26171810; https://doi.org/10.1016/j.bbagrm.2015.07.007
- Dubin RA, Kazmi MA, Ostrer H. Inverted repeats are necessary for circularization of the mouse testis Sry transcript. Gene 1995; 167:245-8; PMID:8566785; https://doi.org/10.1016/0378-1119(95)00639-7
- Kramer MC, Liang D, Tatomer DC, Gold B, March ZM, Cherry S, Wilusz JE. Combinatorial control of Drosophila circular RNA expression by intronic repeats, hnRNPs, and SR proteins. Genes Dev 2015; 29:2168-82; PMID:26450910; https://doi.org/10.1101/gad.270421.115
- Nishikura K. Functions and regulation of RNA editing by ADAR deaminases. Annu Rev Biochem 2010; 79:321-49; PMID:20192758; https://doi.org/10.1146/annurev-biochem-060208-105251
- Savva YA, Rieder LE, Reenan RA. The ADAR protein family. Genome Biol 2012; 13:252; PMID:23273215; https://doi.org/10.1186/gb-2012-13-12-252
- Khan MA, Reckman YJ, Aufiero S, van den Hoogenhof MM, van der Made I, Beqqali A, Koolbergen DR, Rasmussen TB, Van Der Velden J, Creemers EE, et al. RBM20 Regulates Circular RNA Production From the Titin Gene. Circ Res 2016; 119(9):996-1003; PMID:27531932; https://doi.org/10.1161/CIRCRESAHA.116.309568
- Hesselberth JR. Lives that introns lead after splicing. Wiley Interdiscip Rev RNA 2013; 4:677-91; PMID:23881603; https://doi.org/10.1002/wrna.1187
- Zhang Y, Zhang XO, Chen T, Xiang JF, Yin QF, Xing YH, Zhu S, Yang L, Chen LL. Circular intronic long noncoding RNAs. Mol Cell 2013; 51:792-806; PMID:24035497; https://doi.org/10.1016/j.molcel.2013.08.017
- Zaphiropoulos PG. Exon skipping and circular RNA formation in transcripts of the human cytochrome P-450 2C18 gene in epidermis and of the rat androgen binding protein gene in testis. Mol Cell Biol 1997; 17:2985-93; PMID:9154796; https://doi.org/10.1128/MCB.17.6.2985
- Burd CE, Jeck WR, Liu Y, Sanoff HK, Wang Z, Sharpless NE. Expression of linear and novel circular forms of an INK4/ARF-associated non-coding RNA correlates with atherosclerosis risk. PLoS Genet 2010; 6:e1001233; PMID:21151960; https://doi.org/10.1371/journal.pgen.1001233
- McGlincy NJ, Smith CW. Alternative splicing resulting in nonsense-mediated mRNA decay: what is the meaning of nonsense? Trends Biochem Sci 2008; 33:385-93; PMID:18621535; https://doi.org/10.1016/j.tibs.2008.06.001
- Han SP, Tang YH, Smith R. Functional diversity of the hnRNPs: past, present and perspectives. Biochem J 2010; 430:379-92; PMID:20795951; https://doi.org/10.1042/BJ20100396
- Chabot B, Blanchette M, Lapierre I, La Branche H. An intron element modulating 5′ splice site selection in the hnRNP A1 pre-mRNA interacts with hnRNP A1. Mol Cell Biol 1997; 17:1776-86; PMID:9121425; https://doi.org/10.1128/MCB.17.4.1776
- Martinez-Contreras R, Fisette JF, Nasim FU, Madden R, Cordeau M, Chabot B. Intronic binding sites for hnRNP A/B and hnRNP F/H proteins stimulate pre-mRNA splicing. PLoS Biol 2006; 4:e21; PMID:16396608; https://doi.org/10.1371/journal.pbio.0040021
- Miriami E, Margalit H, Sperling R. Conserved sequence elements associated with exon skipping. Nucleic Acids Res 2003; 31:1974-83; PMID:12655015; https://doi.org/10.1093/nar/gkg279
- Bentley DL. Coupling mRNA processing with transcription in time and space. Nat Rev Genet 2014; 15:163-75; PMID:24514444; https://doi.org/10.1038/nrg3662
- Dujardin G, Lafaille C, de la Mata M, Marasco LE, Muñoz MJ, Le Jossic-Corcos C, Corcos L, Kornblihtt AR. How slow RNA polymerase II elongation favors alternative exon skipping. Mol Cell 2014; 54:683-90; PMID:24793692; https://doi.org/10.1016/j.molcel.2014.03.044
- Ip JY, Schmidt D, Pan Q, Ramani AK, Fraser AG, Odom DT, Blencowe BJ. Global impact of RNA polymerase II elongation inhibition on alternative splicing regulation. Genome Res 2011; 21:390-401; PMID:21163941; https://doi.org/10.1101/gr.111070.110
- de la Mata M, Alonso CR, Kadener S, Fededa JP, Blaustein M, Pelisch F, Cramer P, Bentley D, Kornblihtt AR. A slow RNA polymerase II affects alternative splicing in vivo. Mol Cell 2003; 12:525-32; PMID:14536091; https://doi.org/10.1016/j.molcel.2003.08.001
- Chen LL. The biogenesis and emerging roles of circular RNAs. Nat Rev Mol Cell Biol 2016; 17:205-11; PMID:26908011; https://doi.org/10.1038/nrm.2015.32
- Pasquinelli AE. MicroRNAs and their targets: recognition, regulation and an emerging reciprocal relationship. Nat Rev Genet 2012; 13:271-82; PMID:22411466; https://doi.org/10.1038/nrg3162
- Du WW, Yang W, Liu E, Yang Z, Dhaliwal P, Yang BB. Foxo3 circular RNA retards cell cycle progression via forming ternary complexes with p21 and CDK2. Nucleic Acids Res 2016; 44:2846-58; PMID:26861625; https://doi.org/10.1093/nar/gkw027
- Schneider T, Hung LH, Schreiner S, Starke S, Eckhof H, Rossbach O, Reich S, Medenbach J, Bindereif A. CircRNA-protein complexes: IMP3 protein component defines subfamily of circRNPs. Sci Rep 2016; 6:31313; PMID:27510448; https://doi.org/10.1038/srep31313
- Holdt LM, Stahringer A, Sass K, Pichler G, Kulak NA, Wilfert W, Kohlmaier A, Herbst A, Northoff BH, Nicolaou A, et al. Circular non-coding RNA ANRIL modulates ribosomal RNA maturation and atherosclerosis in humans. Nat Commun 2016; 7:12429; PMID:27539542; https://doi.org/10.1038/ncomms12429
- Hansen TB, Kjems J, Damgaard CK. Circular RNA and miR-7 in cancer. Cancer Res 2013; 73:5609-12; PMID:24014594; https://doi.org/10.1158/0008-5472.CAN-13-1568
- Lykke-Andersen J, Bennett EJ. Protecting the proteome: Eukaryotic cotranslational quality control pathways. J Cell Biol 2014; 204:467-76; PMID:24535822; https://doi.org/10.1083/jcb.201311103
- Chen I, Chen CY, Chuang TJ. Biogenesis, identification, and function of exonic circular RNAs. Wiley Interdiscip Rev RNA 2015; 6:563-79; PMID:26230526; https://doi.org/10.1002/wrna.1294
- Hentze MW, Preiss T. Circular RNAs: splicing's enigma variations. EMBO J 2013; 32:923-5; PMID:23463100; https://doi.org/10.1038/emboj.2013.53
- Chen CY, Sarnow P. Initiation of protein synthesis by the eukaryotic translational apparatus on circular RNAs. Science 1995; 268:415-7; PMID:7536344; https://doi.org/10.1126/science.7536344
- Perriman R, Ares M. Circular mRNA can direct translation of extremely long repeating-sequence proteins in vivo. RNA 1998; 4:1047-54; PMID:9740124; https://doi.org/10.1017/S135583829898061X
- You X, Vlatkovic I, Babic A, Will T, Epstein I, Tushev G, Akbalik G, Wang M, Glock C, Quedenau C, et al. Neural circular RNAs are derived from synaptic genes and regulated by development and plasticity. Nat Neurosci 2015; 18(4):603-10; PMID:25714049; https://doi.org/10.1038/nn.3975
- Xu Q, Walker D, Bernardo A, Brodbeck J, Balestra ME, Huang Y. Intron-3 retention/splicing controls neuronal expression of apolipoprotein E in the CNS. J Neurosci 2008; 28:1452-9; PMID:18256266; https://doi.org/10.1523/JNEUROSCI.3253-07.2008
- Yap K, Lim ZQ, Khandelia P, Friedman B, Makeyev EV. Coordinated regulation of neuronal mRNA steady-state levels through developmentally controlled intron retention. Genes Dev 2012; 26:1209-23; PMID:22661231; https://doi.org/10.1101/gad.188037.112
- Wilusz JE. Circular RNAs: Unexpected outputs of many protein-coding genes. RNA Biol 2016; PMID:27571848; https://doi.org/10.1080/15476286.2016.1227905
- Lebreton A, Tomecki R, Dziembowski A, Séraphin B. Endonucleolytic RNA cleavage by a eukaryotic exosome. Nature 2008; 456:993-6; PMID:19060886; https://doi.org/10.1038/nature07480
- Tomecki R, Dziembowski A. Novel endoribonucleases as central players in various pathways of eukaryotic RNA metabolism. RNA 2010; 16:1692-724; PMID:20675404; https://doi.org/10.1261/rna.2237610
- Barrett SP, Salzman J. Circular RNAs: analysis, expression and potential functions. Development 2016; 143:1838-47; PMID:27246710; https://doi.org/10.1242/dev.128074
- Petkovic S, Müller S. RNA circularization strategies in vivo and in vitro. Nucleic Acids Res 2015; 43:2454-65; PMID:25662225; https://doi.org/10.1093/nar/gkv045