ABSTRACT
Viruses are powerful tools to uncover cellular processes. Through viral studies we have recently identified a novel translational control mechanism that involves the DEAD-box helicase Dhh1/DDX6 and RNA folding within coding sequences (CDSs). All Dhh1-dependent mRNAs, viral and cellular ones, (i) contain long and highly structured CDSs, (ii) are directly bound by Dhh1 with a specific pattern, (iii) are activated at the translation initiation step and (iv) express proteins associated with the endoplasmic reticulum. The obtained results uncover a novel layer of translation regulation associated with translation at the endoplasmic reticulum conserved from yeast to humans and hijacked by viruses.
Abbreviations
BMV | = | Brome mosaic virus |
CDS | = | coding sequence |
CRAC | = | crosslinking and cDNA analysis |
ER | = | endoplasmic reticulum |
(+)RNA virus | = | positive-strand RNA virus |
SRP | = | signal recognition particle |
UTR | = | untranslated regions |
Because of their intimate interaction with the cellular machinery and abundance, viruses are powerful tools to uncover cellular processes. Indeed, fundamental post-transcriptional processes such as splicing, capping and polyadenylation were all discovered through viral research.Citation1 Of particular interest to study translational regulation mechanisms are the group of positive-strand RNA [(+)RNA] viruses. Upon entering the cell, their genomes act as mRNAs to translate the viral proteins required for their replication, which for multiple (+)RNA viruses occurs associated to virus-induced invaginations of the endoplasmic reticulum (ER). Thus, (+)RNA viruses need to tightly and efficiently control translation of the viral RNA genomes to ensure enough expression while avoiding viral RNA degradation. An intense focus of study in the translational control field is how host mRNAs are targeted and translated at the ER. Through viral studies we have recently uncovered a novel translational control mechanism at the ER that relies on the presence of long and structured ORFs in both (+)RNA genomes and host mRNAs, and the DEAD-box helicase Dhh1.Citation2
Pioneered experimental in vitro genome-wide measurements of RNA structures in yeast unexpectedly found that the coding sequences (CDSs) of Saccharomyces cerevisiae mRNAs present a higher degree of secondary structure than untranslated regions (UTRs).Citation3 Subsequent studies demonstrated that, in vivo, the CDSs are less structured and that this remodeling is ATP-dependent.Citation4 Structured CDSs are not a unique feature of cellular mRNAs. They are also found in viruses like the Hepatitis C RNA genome, a (+)RNA virus that contains conserved and functionally diverse structural RNA motifs within the CDS.Citation5 In spite of the now widely recognized presence of RNA structures within CDSs, their functional implications and remodeling in both host and viral mRNAs are poorly understood. ATP-dependent helicases are strong candidates to mediate such remodeling.
The highly conserved DEAD-box helicase Dhh1 (named DDX6 in humans) is a well-described translation repressor and decapping activator in the major deadenylation-dependent 5´-3´decay pathway.Citation6 Additionally, in humans DDX6 functions in the miRNA-mediated silencing pathway.Citation7-9 In contrast to this repressing function, we and others demonstrated that Dhh1/DDX6 activates translation of (+)RNA genomes.Citation10-12 How does Dhh1/DDX6 exert these 2 apparently opposing functions? To approach this puzzling issue we used a well-established model system to study the (+)RNA viral life cycle, the replication of the (+)RNA Brome mosaic virus (BMV) in yeast.Citation13 With this viral system we have learnt now that Dhh1 promotes the translation initiation step of BMV RNA2. Moreover, it does so using the same features, including the ATPase activity, that are required for its cellular function. In agreement with a role in translation initiation, Dhh1 interacts with translation initiation factors.Citation2 Unexpectedly, Dhh1-dependence of BMV RNA2 translation is linked not only to the UTRs but also to the CDS. Full-dependence is only achieved when both UTRs and the CDS are present, suggesting that Dhh1 remodels tertiary contacts involving the 3 regions. Consistently, Dhh1 directly binds to sequences in the 3’UTR and the CDS, as shown by crosslinking and cDNA analysis (CRAC) analyses.Citation2 The involvement of the CDS was unexpected because CDSs have been rarely associated with control of translation initiation. Successive deletion analyses of the BMV RNA2 identified a stem-loop located 42 nucleotides after the start codon that strongly represses translation and mediates the dependence on Dhh1 for translational stimulation. Importantly, the Dhh1-dependence for translation is not restricted to BMV RNA2 translation but also to other viral RNAs such as BMV RNA3 (unpublished results) and hepatitis C genomes.Citation12
Given the pioneered role of virus research in uncovering cellular post-transcriptional processes, we questioned whether Dhh1 also activates translation of cellular mRNAs. By combining in yeast RNAseq, ribosome profiling and CRAC, we identified a set of around 245 mRNAs whose translation depends on Dhh1 and are directly bound by Dhh1.Citation2 Interestingly, these mRNAs share common features with the viral BMV RNA genome. First, they present highly structured 5´ UTRs and CDSs, including a region between 40 and 70 nucleotides after the start codon. Second, polysome and ribosome profiling analyses are consistent with a role of Dhh1 in translation initiation. Third, Dhh1 does not affect the steady-state of the viral or cellular mRNAs. Fourth, they are directly bound by Dhh1 with a specific binding distribution different from that to translationally repressed mRNAs, suggesting a link between binding and mode of action. Last, the human homologs of the yeast mRNAs translationally activated by Dhh1 contain long and structured regions in the CDSs, including the one located close after the start codon.Citation2 This suggested that DDX6 functions as a translation activator in humans as well. Indeed, as a proof of principle, we show that the DDX6 activates translation of the PTCH1 mRNA, the human counterpart of the yeast NCR1, which is translationally activated by Dhh1.Citation2 We focus on PTCH1 because its deregulation is a hallmark of pancreatic cancer. Given that DDX6 is overexpressed in several cancers,Citation14-19 it would be plausible that translation regulation by DDX6 promotes malignant transformation. Altogether, these features point out to an additional layer of translational control involving a DEAD-box helicase and RNA folding within CDS that is conserved from yeast to humans and hijacked by viruses.
Interestingly, both the viral and the cellular mRNAs translationally activated by Dhh1 express proteins associated with the ER. This was of great interest to us since ER proteins are preferentially translated at the ER by mechanisms that are under intense study. A well-studied cotranslational pathway recruits nascent-chain ribosome mRNA complexes to the ER via the signal recognition particle (SRP). Recent exciting work uncovered that the SRP is specifically pre-recruited to transcripts that will express proteins with functional ER-targeting signals.Citation20 Non-coding mRNA elements are proposed to mediate this SRP recruitment. Other ER targeting mechanisms include the less understood co-translational SRP-independent routes and pathways based on trans-acting factors and cis-acting elements that function independent of translation.Citation21 Importantly, the cellular mRNAs translationally activated by Dhh1 include cellular mRNAs targeted by SRP-dependent and SRP-independent mechanisms indicating that Dhh1 functions across pathways. In this context, Dhh1 might assist guiding the mRNAs to the ER by binding and remodeling structural cis-acting signals in CDSs. This might be also the case for viral RNA genomes. The ER plays an essential role in the life cycle of (+)RNA viruses. Viral replication occurs in so-called viral replication factories, viral-induced invaginations in the ER membrane. As many viral proteins function close to or at the ER, it makes sense that many (+)RNA genomes translate as well at the ER.Citation22 How the viral RNA genome would be guided to the ER for expression of the viral proteins remains to be fully understood. Interestingly, deletions in the N-terminal region of the BMV RNA2 CDS that include the stem-loop structure conferring Dhh1 dependence for translation, decreases RNA2 localization to the ER.Citation23 This suggests a link between structural cis-acting signals in the BMV RNA2 CDS, Dhh1 and BMV RNA2 localization at the ER.
Based on the above observations we propose the following working model (). mRNAs encoding membrane and secreted proteins are not efficiently translated in the cytoplasm. Dhh1 would bind and remodel a subset of these mRNAs with long and highly structured CDSs, thereby mediating their targeting to the ER by still unknown mechanisms. The decapping activators Pat1 and Lsm1–7, a heptameric ring, would be also involved in such targeting as they interact with Dhh1 and are required for efficient translation of BMV RNA2.Citation24-26 Once at the ER, translation would be favored conceivably by a higher concentration of specific factors, such as initiation factors or chaperones (reviewed inCitation27) or by the release of Dhh1 and the other decapping activators. This mechanism would ensure that mRNAs encoding membrane proteins are only efficiently translated at the ER, their final destination.
Figure 1. Working model of Dhh1 targeting long highly-structured mRNAs to the ER for efficient translation. Long, highly structured mRNAs are not efficiently translated in the cytoplasm. Dhh1 recognizes these mRNAs and binds them in a specific pattern. Subsequently, Dhh1 recruits these mRNAs to the ER where Dhh1 is released and efficient translation occurs.
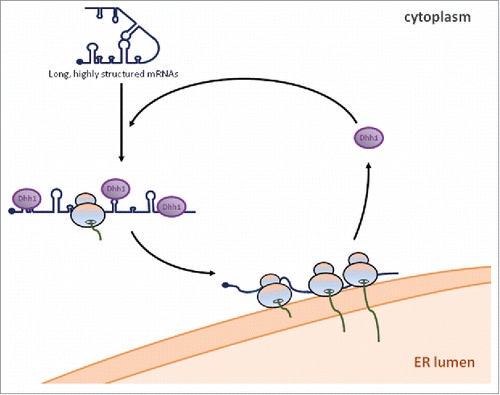
Conclusions and future work
Once again, viruses taught us about a highly conserved cellular process. The simple initial question of how the translational repressor Dhh1 can promote viral BMV RNA translation led us to identify a novel and highly conserved host translation regulation mechanism that involves mRNA secondary structures within CDSs, the DEAD-box helicase Dhh1 and ER translation of membrane proteins This mechanism opens the possibility of co-regulating different subsets of mRNAs encoding proteins with similar function in response to environmental changes. Changes in Dhh1 modifications or location would allow fine-tuning expression of multiple mRNAs. Is this mechanism extended to other helicases? As Ded1, another DEAD-box helicase, is also involved in favoring BMV RNA2 translation we expect that not only Dhh1 but also other DEAD-box helicases control translation by similar mechanisms. This novel layer of translational control might require a complex cooperation between distinct DEAD-box helicases, providing novel opportunities for regulation of functionally related mRNAs. The detailed mechanism of this regulation still remains to be uncovered. Maybe viruses will help us again.
Disclosure of potential conflicts of interest
No potential conflicts of interest were disclosed.
Funding
This work was supported by the Spanish Ministry of Economy and Competitiveness through grant BFU2016–80039-R (AEI/MINEICO/FEDER, UE) and the “Maria de Maeztu” Program for Units of Excellence in R&D (MDM-2014–0370).
References
- Cullen BR. Viral RNAs: lessons from the enemy. Cell 2009; 136:592-7; PMID:19239880; https://doi.org/10.1016/j.cell.2009.01.048
- Jungfleisch J, Nedialkova DD, Dotu I, Sloan KE, Martinez-Bosch N, Bruning L, Raineri E, Navarro P, Bohnsack MT, Leidel SA, et al. A novel translational control mechanism involving RNA structures within coding sequences. Genome Res 2017; 27:95-106; PMID:5204348; https://doi.org/10.1101/gr.209015.116
- Kertesz M, Wan Y, Mazor E, Rinn JL, Nutter RC, Chang HY, Segal E. Genome-wide measurement of RNA secondary structure in yeast. Nature 2010; 467:103-7; PMID:20811459; https://doi.org/10.1038/nature09322
- Rouskin S, Zubradt M, Washietl S, Kellis M, Weissman JS. Genome-wide probing of RNA structure reveals active unfolding of mRNA structures in vivo. Nature 2014; 505:701-5; PMID:24336214; https://doi.org/10.1038/nature12894
- Pirakitikulr N, Kohlway A, Lindenbach BD, Pyle AM. The Coding Region of the HCV Genome contains a network of regulatory RNA structures. Mol Cell 2016; 62:111-20; PMID:26924328; https://doi.org/10.1016/j.molcel.2016.01.024
- Presnyak V, Coller J. The DHH1/RCKp54 family of helicases: an ancient family of proteins that promote translational silencing. Biochim Biophys Acta 2013; 1829:817-23; PMID:23528737; https://doi.org/10.1016/j.bbagrm.2013.03.006
- Chen Y, Boland A, Kuzuoglu-Ozturk D, Bawankar P, Loh B, Chang CT, Weichenrieder O, Izaurralde E. A DDX6-CNOT1 complex and W-binding pockets in CNOT9 reveal direct links between miRNA target recognition and silencing. Mol Cell 2014; 54:737-50; PMID:24768540; https://doi.org/10.1016/j.molcel.2014.03.034
- Chu CY, Rana TM. Translation repression in human cells by microRNA-induced gene silencing requires RCK/p54. PLoS Biol 2006; 4:e210; PMID:16756390; https://doi.org/10.1371/journal.pbio.0040210
- Mathys H, Basquin J, Ozgur S, Czarnocki-Cieciura M, Bonneau F, Aartse A, Dziembowski A, Nowotny M, Conti E, Filipowicz W. Structural and biochemical insights to the role of the CCR4-NOT complex and DDX6 ATPase in microRNA repression. Mol Cell 2014; 54:751-65; PMID:24768538; https://doi.org/10.1016/j.molcel.2014.03.036
- Alves-Rodrigues I, Mas A, Diez J. Xenopus Xp54 and human RCK/p54 helicases functionally replace yeast Dhh1p in brome mosaic virus RNA replication. J Virol 2007; 81:4378-80; PMID:17301158; https://doi.org/10.1128/JVI.02246-06
- Huys A, Thibault PA, Wilson JA. Modulation of hepatitis C virus RNA accumulation and translation by DDX6 and miR-122 are mediated by separate mechanisms. PLoS One 2013; 8:e67437; PMID:23826300; https://doi.org/10.1371/journal.pone.0067437
- Scheller N, Mina LB, Galao RP, Chari A, Gimenez-Barcons M, Noueiry A, Fischer U, Meyerhans A, Díez J. Translation and replication of hepatitis C virus genomic RNA depends on ancient cellular proteins that control mRNA fates. Proc Natl Acad Sci U S A 2009; 106:13517-22; PMID:19628699; https://doi.org/10.1073/pnas.0906413106
- Alves-Rodrigues I, Galao RP, Meyerhans A, Diez J. Saccharomyces cerevisiae: a useful model host to study fundamental biology of viral replication. Virus Res 2006; 120:49-56; PMID:16698107; https://doi.org/10.1016/j.virusres.2005.11.018
- Hashimoto K, Nakagawa Y, Morikawa H, Niki M, Egashira Y, Hirata I, Katsu K, Akao Y. Co-overexpression of DEAD box protein rck/p54 and c-myc protein in human colorectal adenomas and the relevance of their expression in cultured cell lines. Carcinogenesis 2001; 22:1965-70; PMID:11751426; https://doi.org/10.1093/carcin/22.12.1965
- Lin F, Wang R, Shen JJ, Wang X, Gao P, Dong K, Zhang HZ. Knockdown of RCK/p54 expression by RNAi inhibits proliferation of human colorectal cancer cells in vitro and in vivo. Cancer Biol Ther 2008; 7:1669-76; PMID:18769115; https://doi.org/10.4161/cbt.7.10.6660
- Miyaji K, Nakagawa Y, Matsumoto K, Yoshida H, Morikawa H, Hongou Y, Arisaka Y, Kojima H, Inoue T, Hirata I, et al. Overexpression of a DEAD box/RNA helicase protein, rck/p54, in human hepatocytes from patients with hepatitis C virus-related chronic hepatitis and its implication in hepatocellular carcinogenesis. J Viral Hepat 2003; 10:241-8; PMID:12823589; https://doi.org/10.1046/j.1365-2893.2003.00447.x
- Nakagawa Y, Morikawa H, Hirata I, Shiozaki M, Matsumoto A, Maemura K, Nishikawa T, Niki M, Tanigawa N, Ikegami M, et al. Overexpression of rck/p54, a DEAD box protein, in human colorectal tumours. Br J Cancer 1999; 80:914-7; PMID:10360675; https://doi.org/10.1038/sj.bjc.6690441
- Sen ND, Zhou F, Ingolia NT, Hinnebusch AG. Genome-wide analysis of translational efficiency reveals distinct but overlapping functions of yeast DEAD-box RNA helicases Ded1 and eIF4A. Genome Res 2015; 25:1196-205; PMID:26122911; https://doi.org/10.1101/gr.191601.115
- Taniguchi K, Sugito N, Kumazaki M, Shinohara H, Yamada N, Matsuhashi N, Futamura M, Ito Y, Otsuki Y, Yoshida K, et al. Positive feedback of DDX6/c-Myc/PTB1 regulated by miR-124 contributes to maintenance of the Warburg effect in colon cancer cells. Biochim Biophys Acta 2015; 1852:1971-80; PMID:26144048; https://doi.org/10.1016/j.bbadis.2015.06.022
- Chartron JW, Hunt KC, Frydman J. Cotranslational signal-independent SRP preloading during membrane targeting. Nature 2016; 536:224-8; PMID:27487213; https://doi.org/10.1038/nature19309
- Cui XA, Palazzo AF. Localization of mRNAs to the endoplasmic reticulum. Wiley Interdiscip Rev RNA 2014; 5:481-92; PMID:24644132; https://doi.org/10.1002/wrna.1225
- Ravindran MS, Bagchi P, Cunningham CN, Tsai B. Opportunistic intruders: how viruses orchestrate ER functions to infect cells. Nat Rev Microbiol 2016; 14:407-20; PMID:27265768; https://doi.org/10.1038/nrmicro.2016.60
- Chen J, Noueiry A, Ahlquist P. Brome mosaic virus Protein 1a recruits viral RNA2 to RNA replication through a 5' proximal RNA2 signal. J Virol 2001; 75:3207-19; PMID:11238847; https://doi.org/10.1128/JVI.75.7.3207-3219.2001
- Diez J, Ishikawa M, Kaido M, Ahlquist P. Identification and characterization of a host protein required for efficient template selection in viral RNA replication. Proc Natl Acad Sci U S A 2000; 97:3913-8; PMID:10759565; https://doi.org/10.1073/pnas.080072997
- Jungfleisch J, Chowdhury A, Alves-Rodrigues I, Tharun S, Diez J. The Lsm1-7-Pat1 complex promotes viral RNA translation and replication by differential mechanisms. RNA 2015; 21:1469-79; PMID:26092942; https://doi.org/10.1261/rna.052209.115
- Noueiry AO, Diez J, Falk SP, Chen J, Ahlquist P. Yeast Lsm1p-7p/Pat1p deadenylation-dependent mRNA-decapping factors are required for brome mosaic virus genomic RNA translation. Mol Cell Biol 2003; 23:4094-106; PMID:12773554; https://doi.org/10.1128/MCB.23.12.4094-4106.2003
- Reid DW, Nicchitta CV. Diversity and selectivity in mRNA translation on the endoplasmic reticulum. Nat Rev Mol Cell Biol 2015; 16:221-31; PMID:25735911; https://doi.org/10.1038/nrm3958