ABSTRACT
Enhancers are cis- regulatory genetic elements crucial for controlling temporal and cell-type specific patterns of gene expression. Active enhancers generate bi-directional non-coding RNA transcripts called enhancer RNAs (eRNAs). eRNAs are important for stimulating gene expression, but precise mechanisms for this ability remain unclear. Here we highlight recent findings that demonstrate a direct interaction between RNAs and the transcriptional co-activator Creb-binding protein (CBP). Notably, RNA binding could stimulate the core histone acetyltransferase activity of the enzyme, observable in cells as a link between eRNA production, CBP-dependent histone acetylation and expression of genes regulated by specific enhancers. Although RNA binding was independent of RNA sequence, specificity arises in a locus-specific manner at transcribed sites where CBP was bound to chromatin. The results suggest a functional role for eRNAs as regulatory molecules that are able to stimulate the activity of a key epigenetic regulatory enzyme, thereby promoting gene expression. Furthermore, they suggest an intriguing role for eRNAs: by modulating the activity of chromatin modifying enzymes, they could directly impact transcription by altering the chromatin environment.
Introduction
The transcription of bi-directional, non-coding RNAs known as enhancer RNAs (eRNAs) is a key signature of active enhancer regions.Citation1 It is now widely recognized that eRNA transcripts themselves play direct roles in promoting enhancer dependent gene expression; depletion of eRNAs can therefore affect transcription from enhancer-associated genes.Citation2-7 eRNAs have been implicated in controlling looping between enhancers and promoters.Citation5,Citation8 In human breast cancer cells, eRNAs stabilize interactions between estrogen-receptor activated enhancers and promoters by interacting directly with cohesin.Citation5 eRNAs also facilitate recruitment of Mediator to promotersCitation4 and promote the release of paused RNA Polymerase II (Pol II) from the promoter into the gene body at poised genes.Citation9 However, until recently eRNAs were not thought to play a direct role in directing the post-translational modification of histones to modify the chromatin environment. Indeed, enhancer specific histone methylation changes that signpost enhancer activity, such as di-methylation of histone H3 on lysine 4 (H3K4me2), arise due to transcription of the active enhancer by RNA polymerase II; these modifications are independent of eRNA transcripts.Citation10 As H3K4me2 occurs at enhancers without the need for eRNAs, this raises the question of whether eRNAs are capable of sculpting the chromatin milieu.
The transcriptional co-activator Creb binding protein (CBP) contains a core enzymatic histone acetyltransferase (HAT) activity within a central catalytic domain.Citation11,Citation12 CBP acts as a transcriptional ‘network-hub’, interacting with hundreds of transcription factor (TF) binding partners.Citation13 Enhancers are typically formed of clusters of TF binding sites; consequently, CBP is recruited to almost all enhancers through its interactions with TFs, and CBP occupancy is considered a defining characteristic of enhancer elements.Citation14,Citation15 Localizing CBP HAT activity to enhancers using de-activated Cas9 nuclease (dCas9) is sufficient to promote transcription of enhancer-associated genes.Citation16 Moreover, CBP-dependent histone acetylation, specifically acetylation of histone H3 on lysine 27 (H3K27ac), is a characteristic epigenetic signature of enhancers across the genome.Citation14,Citation17
A number of recent studies have revealed that chromatin associated proteins interact with RNA in cells at high frequency.Citation18-20 In many cases, these interactions do not involve canonical or previously annotated RNA binding regions (RBRs) within the interacting proteins.Citation18,Citation19 Notably, RNA binding through non-canonical regions appears to be especially common for chromatin-modifying epigenetic enzymes.Citation18 Chromatin-associated enzymes or enzyme complexes that bind to RNA, such as PRC2 and DNMT1, demonstrate a slight preference for structured RNA elements, but binding is often considered to be promiscuous as they have similar affinities for more than one RNA sequence.Citation21-25 Moreover, several examples have now been described where RNA binding can directly modulate the catalytic activity of these enzymes.Citation21,Citation22,Citation25-28
We recently demonstrated an interaction between CBP and RNA,Citation29 where specificity for RNA binding arises in a locus-specific manner, dictated by locations of CBP recruitment to chromatin. Although independent of RNA sequence, these interactions were sufficient to stimulate the catalytic HAT activity of the CBP enzyme, increasing CBP-dependent H3K27ac and thus promoting transcription.Citation29 Given the high frequency of interactions between RNAs and chromatin associated proteins, this raises an intriguing question: Does RNA binding convey a localized activity profile to chromatin bound epigenetic enzymes, and does this enable eRNAs to modify the local chromatin environment at a particular locus? Here, we utilize the example of CBP to explore the potential role of eRNAs as regulators of chromatin-modifying enzyme activity, and explore the implications of such a mechanism for enhancer function.
Locus specificity for RNA binding
Our data revealed that CBP could interact with RNA in cells under native conditions, and importantly that CBP binds directly to RNA in photoactivatable ribonucleoside-enhanced crosslinking and immunoprecipitation (PAR-CLIP) experiments.Citation29-31 Profiling of CBP-bound RNAs across the genome using high-throughput sequencing of PAR-CLIP libraries, revealed that bound RNAs arise from sites where CBP is recruited to chromatin. As CBP is strongly bound to enhancer regions and promoters, we observed a strong bias toward RNAs transcribed from these regions. These transcripts were especially enriched given their relatively low abundance and high turnover compared with coding transcripts from exonic regions. CBP therefore preferentially binds to RNAs transcribed from sites where it is recruited to chromatin.Citation29 Thus, although binding does not rely on a particular RNA sequence, RNA binding to CBP displays locus-specificity – transcripts are preferentially bound according to where CBP is bound to chromatin (). We note that, in common with other interactions between chromatin modifiers and RNA, a preference for specific RNA structures within eRNAs remains a possibility and requires further investigation for CBP.
Figure 1. RNA binding can generate localized CBP activity profiles and tailored patterns of histone acetylation. (A) Locus specificity for RNA binding to CBP. CBP bound RNAs (Orange) correspond to sites of CBP recruitment to chromatin (Blue) and nascent RNA transcription (Gray) at enhancers and promoters; (B) RNA binding to the activation loop of CBP stimulates catalytic HAT activity by displacing the loop from the active site, and thereby promotes CBP dependent histone acetylation (H3K27ac); (C) eRNA binding could generate enhancer specific patterns of CBP activity by differential regulation of CBP activity. At more active enhancers, CBP activity is stimulated more strongly by eRNA binding (green arrows) than at enhancers with weak stimulation of CBP activity (red cross); (D) Differences in the ability of sense and antisense eRNA transcripts to stimulate CBP activity. The eRNA strand that binds and stimulates CBP strongly (Green arrows) could promote associated gene expression more strongly than weaker binding eRNA strands (Red cross).
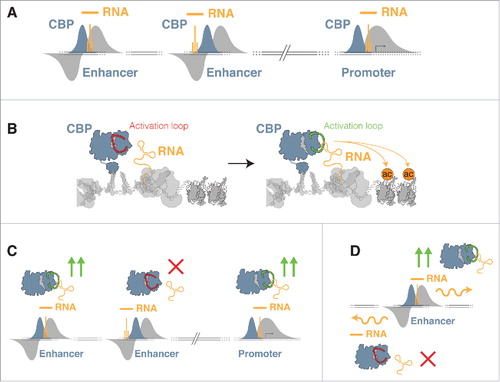
One important consequence of locus-specific binding, was that RNAs interacting with CBP in vivo included a large number of eRNAs due to the high frequency of CBP recruitment at enhancers. A locus-specific binding model is logical in the context of the biologic function of CBP: CBP is almost universally recruited to enhancer elements across the genome, regions that differ in their underlying DNA sequence. eRNAs arising from these enhancers will therefore also display different sequences and structural features. By retaining the ability to interact with RNAs independent of sequence, the activity of CBP could thus be modulated by RNAs wherever it binds to chromatin, i.e., by eRNAs transcribed from any enhancer element where CBP is bound. Locus-specificity therefore opens the possibility that RNA binding to CBP, and potentially other epigenetic enzymes, could confer highly localized effects on specific CBP populations across the genome. Thus, RNAs, and eRNAs in particular could function as local regulators of CBP activity.
Direct binding to eRNAs stimulates CBP HAT activity
To investigate this potential regulatory function of eRNAs in more detail, we reconstituted binding to CBP in vitro.Citation29 RNA pull downs and EMSAs revealed that eRNAs bind to the large, full-length CBP protein, but also to the much smaller catalytic HAT domain of CBP. We mapped RNA binding within the HAT domain to a contiguous region of 49 basic and disordered amino acids.Citation11,Citation12,Citation29 Importantly, this RBR had been previously identified as an important regulatory motif for the HAT activity of CBP.Citation32 This region, known as the activation loop, binds within the active site of CBP and blocks substrate binding until it is displaced by auto-acetylation.Citation32 Consistent with the importance of the activation loop for regulating catalytic activity, we found that RNA binding to the RBR within the HAT domain caused a pronounced stimulation of the HAT activity of CBP (). This was evident both in radioactive filter binding HAT assays, and by western blot for specific CBP-dependent histone modifications, H3K27ac and H4K5ac, on reconstituted nucleosomes in vitro. HAT assays under steady-state conditions revealed that stimulation results from displacement of the activation loop from the active site upon RNA bindingCitation29 (). Our results also revealed that eRNAs possess variable abilities to bind to CBP, and to stimulate its HAT activity. For example, eRNAs that failed to bind strongly to CBP in pull-down experiments, also failed to strongly upregulate its HAT activity, and different eRNA sequences produced different patterns of stimulation.Citation29 These findings support a model that various sequences, and potentially structures of eRNAs, may have differential binding to CBP and generate alternative patterns of HAT stimulation.
Therefore, one intriguing possibility is that the activity of CBP can be modulated by eRNAs at different enhancers to generate a tailored activity profile at that enhancer (). Such a model could help to explain the observation that some eRNAs display strong strand-specificity in their ability to stimulate gene expression: if one strand is able to bind strongly to CBP and stimulate activity, but the other is unable to bind, then a difference in the ability of each eRNA strand to direct transcription would be observed at that enhancer (). We also note that while we observe a bias toward eRNA binding to CBP due to the prevalence of enhancer-bound CBP,Citation33 promoter-derived transcripts could also potentially bind to and stimulate CBP activity (). The observation that eRNAs can control the activity of CBP in an enhancer specific manner, can also help explain the surprising observation that recruitment of CBP to enhancers and promoters is a relatively poor predictor of downstream gene activation.Citation33 For example, eRNAs that strongly stimulate the activity of CBP could contribute to a stronger enhancer activity profile. However, more work is required to fully characterize the relationship between transcribed eRNAs, stimulation of CBP activity and enhancer strength.
Could eRNA have a general role as a regulatory molecule?
The regulatory activation loop within the HAT domain of CBP, which contains the RBR, binds in the active site of the enzyme and blocks substrate binding. The ability to bind within the CBP active site is derived from the positive charge of the RBR within the activation loop; this positive charge also enables the loop to bind to RNAs. Other histone acetyltransferases, such as the MYST family member Males Absent on the First (hMOF) are not regulated by an activation loop in the manner of CBP, thus their HAT activity remains unaffected by the presence of RNA.Citation29
Nevertheless, it is clear that the activity of other epigenetic-enzyme complexes, such as PRC2 and DNMT1, are sensitive to RNA binding: Binding of RNA to PRC2 decreases both its methyltransferase activity and recruitment to promoter regions,Citation22,Citation25,Citation26 and ncRNAs transcribed proximal to the C/EBPA locus interact with DNMT1 to block DNA methylation at the promoter.Citation21 In both instances, RNA binding was driven more by RNA secondary structure than sequence, with more structured RNAs binding more strongly, and different RNA sequences producing different effects on enzyme activity.Citation21-23 In cells, the ability of ncRNA to inhibit the methyltransferase activity of PRC2 is thought to arise from competition between RNA and nucleosomes for binding to PRC2.Citation25 For DNMT1, RNA binding was mapped to the catalytic domain of the enzyme.Citation21 This suggests that in order for a chromatin-modifying enzyme to be susceptible to direct regulation by RNA binding, there must be a link between potential RBRs within the protein and the catalytic domain. Interestingly, many epigenetic complexes - such as PRC2 - contain multiple subunits and components, often with individual abilities to bind to RNA. It remains to be determined what the combined effect of RNA binding to individual components of these complexes plays to regulate overall complex activity. Full elucidation of the mechanisms of RNA-dependent stimulation will require further biochemical and structural studies of the interactions between the enzyme and bound RNA species.
Concluding remarks
Recent studies have highlighted the high frequency of binding between chromatin-associated proteins or enzyme complexes and RNA in cells, and the importance of non-canonical RBRs for these interactions.Citation18,Citation19 The observation that locus-specific RNA binding to CBP can directly stimulate its catalytic HAT activity presents an interesting role for these interactions: RNA binding events could enable local ‘tailoring’ of enzymatic-activity profiles.
In the case of CBP, this tailoring is driven by the binding of RNAs transcribed proximal to sites where CBP is recruited to chromatin. RNAs bind to CBP through a non-canonical, positively charged RBR within the catalytic HAT domain. Because this region is crucial for the regulation of CBP activity, RNA binding can stimulate the catalytic activity of the enzyme and promote gene expression. The structure of CBP, and its method of regulation by the activation loop, renders it particularly susceptible to regulation by RNA binding to this region. While it is increasingly clear that RNA binding to other chromatin-modifying enzymes is widespread, the extent to which this binding can modulate their enzymatic activity is less well understood. A number of examples suggest that RNA does play such a regulatory role,Citation21,Citation22,Citation25-28 however further biochemical work is required to fully determine the mechanisms that RNAs use to modulate the activity of these complexes.
For CBP, locus-specific RNA binding leads to preferential binding of CBP to eRNAs, as CBP is enriched at enhancers. Our results suggest a functional role for eRNAs as regulatory molecules that are able to stimulate the activity of a key epigenetic regulatory enzymes, thereby promoting gene expression. Furthermore, they suggest an intriguing, RNA sequence-independent (but potentially structure-dependent) role for eRNAs in modulating the activity of chromatin modifying enzymes, that could directly impact transcription by altering the chromatin environment. In contrast, we note that enhancer-specific methylation changes appear to be reliant on transcription at enhancers, rather than eRNA transcripts themselves.Citation10 A key outstanding question is whether eRNA transcripts can play a role in modifying the activity of epigenetic complexes when enhancers loop to contact promoter regions.
Chromatin-modifying enzymes and enzyme complexes are crucial for directing gene expression profiles across the genome. In particular, eRNAs are attractive candidates as regulatory molecules due to their highly localized expression profiles, rapid turnover and low abundance.Citation2,Citation3,Citation5 The prevalence of RNA binding to chromatin-modifying enzymes, and the potential for modulation of their catalytic activity, suggests that RNAs could modulate their activity in a similar manner to CBP to generate tailored patterns of histone modifications and gene expression profiles.
Disclosure of potential conflicts of interest
No potential conflict of interest were disclosed.
References
- Kim TK, Shiekhattar R. Architectural and functional commonalities between enhancers and promoters. Cell. 2015;162:948–59. doi:10.1016/j.cell.2015.08.008.
- Lam MTY, Cho H, Lesch HP, Gosselin D, Heinz S, Tanaka-Oishi Y, Benner C, Kaikkonen MU, Kim AS, Kosaka M, et al. Rev-Erbs repress macrophage gene expression by inhibiting enhancer-directed transcription. Nature. 2013;498:511–5. doi:10.1038/nature12209.
- Kim TK, Hemberg M, Gray JM, Costa AM, Bear DM, Wu J, Harmin DA, Laptewicz M, Barbara-Haley K, Kuersten S, et al. Widespread transcription at neuronal activity-regulated enhancers. Nature. 2010;465:182–7. doi:10.1038/nature 09033.
- Lai F, Orom UA, Cesaroni M, Beringer M, Taatjes DJ, Blobel GA, Shiekhattar R. Activating RNAs associate with Mediator to enhance chromatin architecture and transcription. Nature. 2013;494:497–501. doi:10.1038/nature11884.
- Li W, Notani D, Ma Q, Tanasa B, Nunez E, Chen AY, Merkurjev D, Zhang J, Ohgi K, Song X, et al. Functional roles of enhancer RNAs for oestrogen-dependent transcriptional activation. Nature 2013;498:516–20. doi:10.1038/nature12210.
- Melo CA, Drost J, Wijchers PJ, van de Werken H, de Wit E, Oude Vrielink JAF, Elkon R, Melo SA, Léveillé N, Kalluri R, et al. eRNAs are required for p53-dependent enhancer activity and gene transcription. Mol Cell. 2013;49:524–35. doi:10.1016/j.molcel.2012.11.021.
- Mousavi K, Zare H, Dell'Orso S, Grontved L, Gutierrez-Cruz G, Derfoul A, Hager GL, Sartorelli V. eRNAs promote transcription by establishing chromatin accessibility at defined genomic loci. Mol Cell. 2013;51:606–17. doi:10.1016/j.molcel.2013.07.022.
- Hsieh CL, Fei T, Chen Y, Li T, Gao Y, Wang X, Sun T, Sweeney CJ, Lee GSM, Chen S, et al. Enhancer RNAs participate in androgen receptor-driven looping that selectively enhances gene activation. Proc Natl Acad Sci. 2014;111:7319–24. doi:10.1073/pnas.1324151111.
- Schaukowitch K, Joo JY, Liu X, Watts JK, Martinez C, Kim T-K. Enhancer RNA facilitates NELF release from immediate early genes. Mol Cell. 2014;56:29–42. doi:10.1016/j.molcel.2014.08.023.
- Kaikkonen MU, Spann NJ, Heinz S, Romanoski CE, Allison KA, Stender JD, Chun HB, Tough DF, Prinjha RK, Benner C, et al. Remodeling of the enhancer landscape during macrophage activation is coupled to enhancer transcription. Mol Cell. 2013;51:310–25. doi:10.1016/j.molcel.2013.07.010.
- Wang L, Tang Y, Cole PA, Marmorstein R. Structure and chemistry of the p300/CBP and Rtt109 histone acetyltransferases: Implications for histone acetyltransferase evolution and function. Curr Opin Struct Biol. 2008;18:741–7. doi:10.1016/j.sbi.2008.09.004.
- Delvecchio M, Gaucher J, Aguilar-Gurrieri C, Ortega E, Panne D. Structure of the p300 catalytic core and implications for chromatin targeting and HAT regulation. Nat Struct Biol. 2013;20:1040–6. doi:10.1038/nsmb.2642.
- Bedford DC, Kasper LH, Fukuyama T, Brindle PK. Target gene context influences the transcriptional requirement for the KAT3 family of CBP and p300 histone acetyltransferases. Epigenetics. 2010;5:9–15. doi:10.4161/epi.5.1.10449.
- Creyghton MP, Cheng AW, Welstead GG, Kooistra T, Carey BW, Steine EJ, Hanna J, Lodato MA, Frampton GM, Sharp PA, et al. Histone H3K27ac separates active from poised enhancers and predicts developmental state. PNAS. 2010;107:21931–6. doi:10.1073/pnas.1016071107.
- May D, Blow MJ, Kaplan T, McCulley DJ, Jensen BC, Akiyama JA, Holt A, Plajzer-Frick I, Shoukry M, Wright C, et al. Large-scale discovery of enhancers from human heart tissue. Nat Genet. 2012;44:89–93. doi:10.1038/ng.1006
- Hilton IB, D'Ippolito AM, Vockley CM, Thakore PI, Crawford GE, Reddy TE, Gersbach CA. Epigenome editing by a CRISPR-Cas9-based acetyltransferase activates genes from promoters and enhancers. Nat Biotechnol. 2015;33:510–7. doi:10.1038/nbt.3199.
- Jin Q, Yu LR, Wang L, Zhang Z, Kasper LH, Lee J-E, Wang C, Brindle PK, Dent SYR, Ge K. Distinct roles of GCN5/PCAF-mediated H3K9ac and CBP/p300-mediated H3K18/27ac in nuclear receptor transactivation. EMBO J. 2011;30:249–62. doi:10.1038/emboj.2010.318.
- He C, Sidoli S, Warneford-Thomson R, Tatomer DC, Wilusz JE, Garcia BA, Bonasio R. High-resolution mapping of RNA-binding regions in the nuclear proteome of embryonic stem cells. Mol Cell. 2016;64:416–30. doi:10.1016/j.molcel.2016.09.034.
- Castello A, Fischer B, Frese CK, Horos R, Alleaume A-M, Foehr S, Curk T, Krijgsveld J, Hentze MW. Comprehensive identification of RNA-binding domains in human cells. Mol Cell. 2016;63:696–710. doi:10.1016/j.molcel.2016.06.029.
- G Hendrickson D, Kelley DR, Tenen D, Bernstein B, Rinn JL. Widespread RNA binding by chromatin-associated proteins. Genome Biol. 2016;17:28. doi:10.1186/s13059-016-0878-3.
- Di Ruscio A, Ebralidze AK, Benoukraf T, Amabile G, Goff LA, Terragni J, Figueroa ME, De Figueiredo Pontes LL, Alberich-Jorda M, Zhang P, et al. DNMT1-interacting RNAs block gene-specific DNA methylation. Nature. 2013;503:371–6. doi:10.1038/nature12598.
- Cifuentes-Rojas C, Hernandez AJ, Sarma K, Lee JT. Regulatory interactions between RNA and polycomb repressive complex 2. Mol Cell. 2014;55:171–85. doi:10.1016/j.molcel.2014.05.009.
- Davidovich C, Zheng L, Goodrich KJ, Cech TR. Promiscuous RNA binding by Polycomb repressive complex 2. Nat Struct Biol. 2013;20:1250–7. doi:10.1038/nsmb.2679.
- Kaneko S, Bonasio R, Saldaña-Meyer R, Yoshida T, Son J, Nishino K, Umezawa A, Reinberg D. Interactions between JARID2 and noncoding RNAs regulate PRC2 recruitment to chromatin. Mol Cell. 2014;53:290–300. doi:10.1016/j.molcel.2013.11.012.
- Beltran M, Yates CM, Skalska L, Dawson M, Reis FP, Viiri K, Fisher CL, Sibley CR, Foster BM, Bartke T, et al. The interaction of PRC2 with RNA or chromatin is mutually antagonistic. Genome Res. 2016;26:896–907. doi:10.1101/gr.197632.115.
- Kaneko S, Son J, Bonasio R, Shen SS, Reinberg D. Nascent RNA interaction keeps PRC2 activity poised and in check. Genes Dev. 2014;28:1983–8. doi:10.1101/gad.247940.114.
- Wongtrakoongate P, Riddick G, Fucharoen S, Felsenfeld G. Association of the long non-coding RNA Steroid Receptor RNA Activator (SRA) with TrxG and PRC2 complexes. PLoS Genet. 2015;11:e1005615. doi:10.1371/journal.pgen.1005615.
- Yang YW, Flynn RA, Chen Y, Qu K, Wan B, Wang KC, Lei M, Chang HY. Essential role of lncRNA binding for WDR5 maintenance of active chromatin and embryonic stem cell pluripotency. Elife. 2014;3:e02046. doi:10.7554/eLife.02046.
- Bose DA, Donahue G, Reinberg D, Shiekhattar R, Bonasio R, Berger SL. RNA binding to CBP stimulates histone acetylation and transcription. Cell. 2017;168:135–149.e22. doi:10.1016/j.cell.2016.12.020.
- Hafner M, Landthaler M, Burger L, Khorshid M, Hausser J, Berninger P, Rothballer A, Ascano M, Jungkamp A-C, Munschauer M, et al. Transcriptome-wide identification of RNA-binding protein and microRNA target sites by PAR-CLIP. Cell. 2010;141:129–41. doi:10.1016/j.cell.2010.03.009.
- Huppertz I, Attig J, D'Ambrogio A, Easton LE, Sibley CR, Sugimoto Y, Tajnik M, König J, Ule J. iCLIP: Protein-RNA interactions at nucleotide resolution. Methods. 2014;65:274–87. doi:10.1016/j.ymeth.2013.10.011.
- Thompson PR, Wang D, Wang L, Fulco M, Pediconi N, Zhang D, An W, Ge Q, Roeder RG, Wong J, et al. Regulation of the p300 HAT domain via a novel activation loop. Nat Struct Biol. 2004;11:308–15. doi:10.1038/nsmb740.
- Kasper LH, Qu C, Obenauer JC, McGoldrick DJ, Brindle PK. Genome-wide and single-cell analyses reveal a context dependent relationship between CBP recruitment and gene expression. NAR. 2014;42:11363–82. doi:10.1093/nar/gku827.