ABSTRACT
RNA interference (RNAi) utilizes a conserved cellular autoimmune defense mechanism involving the internalization of dsRNA into cells and the activation of a set of RNAi related genes. Using RNAi, complete sex reversal is achievable in males of the prawn Macrobrachium rosenbergii by knocking down the transcript level of an insulin-like androgenic gland hormone (Mr-IAG) through injections of dsRNA of the entire Mr-IAG ORF sequence (dsMr-IAG – 518bp). Interestingly, in-vivo knockdown success and dsMr-IAG lengths seemed to correlate, with long dsRNA being the most effective and short dsRNA fragments showing no effect. However, little is known about the RNAi machinery in M. rosenbergii. We discovered the Mr-Dicer and Mr-Argonaute gene families, associated with the major knockdown pathways, in our M. rosenbergii transcriptomic library. In response to dsMr-IAG administration, only post-transcriptional pathway-related gene transcript levels were upregulated. In addition, a passive dsRNA channel (a SID1 gene ortholog) that allows external dsRNA to enter cells was found. Its function was validated by observing Mr-SID1 specific upregulation dependent on dsRNA lengths, while attempted loss-of-function experiments were lethal. Our results, which suggest differential systemic responses to dsRNA lengths, provide evidence that the above RNAi-based manipulation occurs via the post-transcriptional pathway. The temporal nature of the latter pathway supports the safety of using such RNAi-based biotechnologies in aquaculture and environmental applications. Unlike reports of RNAi driven by the administration of small dsRNA fragments in-vitro, the case presented here demonstrates length dependency in-vivo, suggesting further complexity in the context of the entire organism.
Introduction
RNA interference (RNAi) has gained wide acceptance as an effective tool not only in molecular and gene function research, but also in a variety of technological applications. RNAi is based on an endogenous cellular defense mechanism against double-stranded RNA (dsRNA) viruses.Citation1 Potent and specific interference at the post-transcriptional-mRNA level by using synthetic dsRNA complementary to endogenous sequences was first observed in Caenorhabditis elegans.Citation2
Gene expression interference through dsRNA is achievable following in-vivo dsRNA administration through feeding, immersion and injection.Citation3,Citation4 Post administration, dsRNA is delivered into the cytoplasm from the extracellular matrix either by SID1, a specific dsRNA passive channel,Citation5-7 or through endocytosis.Citation8 In the cytosol, long dsRNA fragments are processed by Dicer2, a ribonuclease III-related enzyme, into 21–22-bp fragments widely known as siRNAs.Citation9 These siRNAs are incorporated with the Argonaute protein (Ago), the main component of the multiprotein RNA-induced silencing complex (RISC). In its unwound state, the antisense of the dsRNA facilitates specific identification of the mRNA targeted for endonucleolytic cleavage.Citation10
In general and similar to other non-coding RNAs (ncRNAs), dsRNA molecules are involved in many cellular pathways for regulating eukaryote gene expression during various life stages.Citation11 These ncRNAs take part in different cellular pathways and are all associated with Ago protein family members.Citation12 Interference with gene expression takes place not only through the post-transcriptional, siRNA pathway, it can also occur at the transcriptional level, via the miRNA pathway, in which small ncRNAs are processed into miRNA molecules.Citation13-15 Such miRNAs could regulate gene expression in the post-transcriptional pathway involving Dicer2 and Ago2. On the other hand, miRNA is also recognized by nuclear proteins, such as Dicer1, resulting in the generation of siRNAs that interact with Ago1, the main component of the RNA-induced initiation transcriptional silencing (RITS) complex. These proteins recruit nascent chromatin and chromatin modifying complexes resulting in chromatin remodeling and heterochromatic silencing.Citation16-20 Ago family proteins are also involved in ncRNAs associated with transposable element regulation pathways, in which Ago3, a protein that plays an important role in transcriptional regulation, participates.Citation21
Using the RNAi method, a biotechnological application was developed in the aquaculture field for the culture of preferred all-male monosex populations of the giant freshwater prawn, Macrobrachium rosenbergii.Citation22,Citation23 This species exhibits a dimorphic growth pattern, in which males grow faster and reach larger final sizes than females at harvest, rendering the culture of all-male populations economically beneficial.Citation24,Citation25
In crustaceans, sex differentiation is regulated by the androgenic gland, a male specific endocrine organ. The activity of the gland is mediated in decapod crustaceans through the insulin-like androgenic gland hormone (IAG).Citation26,Citation27 Administration of dsRNA complementary to the entire open reading frame (ORF) sequence of M. rosenbergii-IAG (Mr-IAG ORF – 518-bp) causes a dramatic knockdown that leads to an approximately 99% decrease in transcript level.Citation22,Citation28 At the phenotypic level, Mr-IAG knockdown causes the cessation of spermatogenesis and the inhibition of secondary masculine sex character development.Citation28 Furthermore, dsMr-IAG injection at a critical juvenile stage causes a full and functional sex reversal of genetic males (ZZ) into 'neo-females'. When crossed with regular males, such neo-females produce the preferred all-male progeny.Citation22
As in many other eukaryotic species, in several crustacean species the above-mentioned knockdown pathways are highly conserved and have important roles in gene transcriptional and post-transcriptional regulation, viral defense and the suppression of transposable elements.Citation29,Citation30 The current study examined the expression of the Dicer and Argonaute gene paralogs in M. rosenbergii following dsMr-IAG administration with the aim of elucidating possible Mr-IAG knockdown pathways, whether transcriptional, post-transcriptional or both.
Gene function studies and therapeutic manipulations that use dsRNA, which trigger RNAi pathways, typically exploit short dsRNA, like siRNA or shRNA.Citation4,Citation31,Citation32 These short dsRNA molecules, however, failed to cause Mr-IAG knockdown, in our preliminary work to the current study. Therefore, we sought to determine the minimum dsRNA length required to obtain Mr-IAG knockdown as effective as that achieved by the entire ORF sequence (518-bp). Furthermore, we also examined the possible cellular mechanism behind this manipulation and the changes that occurred in the expression levels of Mr-IAG and several RNAi associated genes, such as the SID1 and Dicer paralogs, in response to different dsMr-IAG lengths.
Results
Effect of dsRNA fragment lengths shorter than the full ORF on Mr-IAG knockdown efficiency
Attempts to knock down Mr-IAG expression levels using shorter dsMr-IAG fragments showed a clear correlation between dsRNA fragment length and Mr-IAG knockdown efficiency, i.e., the longer the dsRNA fragment, the greater the knockdown of Mr-IAG expression levels (). Of the four lengths tested, only the 250-bp dsRNA fragment caused a significant knockdown effect similar to the entire ORF (∼99% decrease compared with the negative control, F(2,15) = 18.871, P-value < 0.0001). The relative Mr-IAG expression levels while using the 250-bp fragments were significantly different from that of the negative control and strikingly similar to the effects of the entire ORF sequence (P-value < 0.001 and P-value = 0.93, respectively). A pair of dsRNA fragments of 100-bp (Serial no. 100_2 and 100_5, Fig. S1A) caused ∼75% decrease in expression, which was significantly different from expression levels in both the negative control group and the entire ORF group (P-value = 0.034 and P-value < 0.001, respectively). Attempts to improve the knockdown efficiency of the 100-bp fragments by covering the entire ORF sequence with a mixture of 100-bp fragments did not yield better results (See Fig. S2). A similar mixture of 75-bp dsRNA fragments caused a minor decrease in Mr-IAG transcript level, but statistically, that result was similar to those of both the negative control and the entire ORF injected groups (P-value = 0.37 and 0.178, respectively). Transcription level in the control (non-injected) group was significantly different from that of the entire ORF injected group (P-value = 0.012). All attempts to knockdown Mr-IAG expression using a mixture of 19-bp, short interference RNA (siRNA) did not cause down regulation compared with the negative control group (P-value = 1.0) and were significantly different from the results for the entire ORF (P-value < 0.01). In all the distinct length dependency experiments (mixture of 19-bp, 75-bp, 100-bp or 250-bp fragments), Mr-IAG expression levels in the negative control groups and in the entire ORF injected groups were significantly different.
Figure 1. dsRNA length knockdown dependency. (A) Effect of administered dsMr-IAG lengths (19-bp, 75-bp, 100-bp, and 250-bp) on Mr-IAG transcript levels in 4 distinct in-vivo experiments. Negative control – non-injected group, Positive control – entire ORF injected group (518-bp). (B) A general transcript reduction pattern following injection of dsMr-IAG at different lengths.
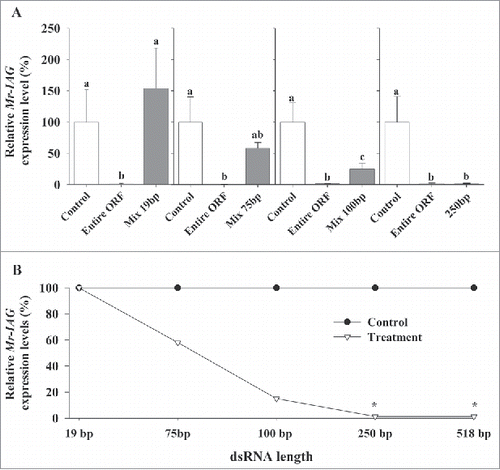
Presence and phylogeny of knockdown-pathway-related genes in M. rosenbergii
To understand the difference in M. rosenbergii in the activation of RNAi and its dependence on dsRNA length compared with its activation in other RNAi pathways, we examined the main RNAi associated genes, members of the Dicer and Argonaute families. Mining our M. rosenbergii transcriptomic library resulted in multiple sequence alignments with the Mr-Dicer family based on putative amino acid sequences showing cluster deviations for both Dicer1 and Dicer2 (). M. rosenbergii Dicer1 (Mr-Dicer1) showed homologies to Marsupenaeus japonicus Dicer1, Penaeus monodon Dicer1, Litopenaeus vannamei Dicer1 and Drosophila melanogaster Dicer1 (83%, 71%, 70% and 67%, respectively). Mr-Dicer2 showed homologies to Lv-Dicer2, Pm-Dicer2 and Dm-Dicer2 (51%, 51% and 27%, respectively). Homology of 32% was found between the Mr-Dicer paralogs.
Figure 2. Phylogenetic analysis knockdown pathway associated genes. Phylogenetic analysis based on multiple alignments using Mega 6.0 software. The neighbor-joining tree was constructed according to multiple alignments of the putative amino acid sequences: (A) Dicer constructed according to phylogenetic analysis of full amino acid sequences. (B) Argonaute phylogenetic analysis constructed according to multiple alignments of PIWI domain amino acid sequences. Tree scale indicates the evolutionary distance by tree branch lengths, which are proportional to sequence differences. Bootstrap values represented by numbers next to the branches. GenBank accession numbers are in Table S5.
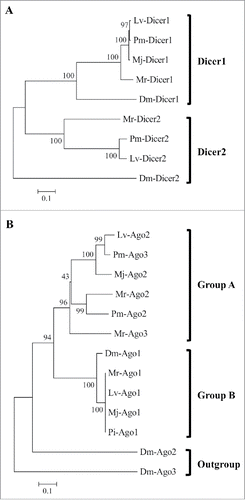
Phylogenetic analysis based on multiple amino acid sequence alignments of 3 Ago candidates demonstrated deviations into only two clusters: cluster A for Ago2 and Ago3, and cluster B for Ago1 (). Mr-Ago1 showed significant homology to Ago1 orthologs from other crustacean species such as Panulirus interruptus Ago1, Mj-Ago1 and Lv-Ago1 (99%, 98% and 98%, respectively) and to Dm-Ago1 (86% homology). The cases of Argonaute2 and Argonaute3 were difficult to differentiate by phylogenetic analysis. Homologies of 43% and 38% were noted between Mr-Argonaute paralogs of Mr-Ago1 to Mr-Ago2 and Mr-Ago3, respectively. A higher, homology (48%) was found between Mr-Ago2 and Mr-Ago3.
To elucidate which knockdown pathway was activated as a response to dsMr-IAG manipulation, dsMr-IAG was administered and RNAi associated gene induction (Dicer and Ago families) was tested. Mr-IAG expression levels were significantly lower, ∼99% of control (t(10) = 18.608, p-value < 0.0001), as a result of dsMr-IAG injection (). Comparing the expression levels of genes related to the RNAi mechanism between the group injected with dsMr-IAG to a non-injected group demonstrated that Mr-Dicer2 was significantly upregulated in the injected group (t(6) = −4.873, p-value < 0.01) () while Mr-Dicer1 expression levels were similar to those in the non-injected group (p-Value = 0.128). Similarly, in the Ago gene family, only one candidate, Mr-Ago2, was upregulated in response to dsMr-IAG injection (p-value < 0.001) (), while Mr-Ago1 and Mr-Ago3 expression levels were similar to those in the non-injected group (t-test, t(13) = −1.091, P-value = 0.295 and Mann-Whitney U-test, P-value = 0.779, respectively).
Figure 3. Expression levels of RNAi associated genes in response to entire ORF dsMr-IAG injection. (A) Mr-IAG expression level in dsMr-IAG injected group (entire ORF) vs. non-injected group (Control). (B) Expression levels of Mr-Dicer and Mr-Argonaute paralogs in the same experimental groups as in (A).
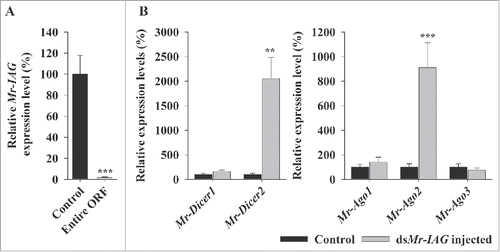
Mr-SID1, knockdown pathway and dsRNA length
Mr-SID1, which encodes for a passive channel with specificity to dsRNA molecules, was found by comparing the L. vannamei SID1 amino acid sequence to our M. rosenbergii transcriptomic library, resulting in a transcript with 52% homology. This transcript (Mr-SID1) was fully sequenced, and 11 transmembrane regions were predicted in its putative protein sequence by a bioinformatics hydrophobicity evaluation (Fig. S3A). Phylogenetic analysis demonstrated that SID1 is conserved between different arthropod species (Fig. S3B).
With the aim of confirming the function of Mr-SID1, its expression levels were measured in response to administration of dsRNA and DNA (both of GFP sequence) and PBS as a negative control (). Mr-SID1 was upregulated only in the presence of dsRNA (F(2,19) = 7.19, p-value = 0.004), showing the specificity of Mr-SID1 to dsRNA. Furthermore, loss of function of Mr-SID1 showed a lethal effect after two consecutive injections of dsMr-SID1 and dsGFP (). In all the control groups, including PBS, dsGFP and dual injections of two exogenous dsRNA (dsGFP + dsRB), high survival rates were demonstrated (100%, 100% and 87%, respectively). All the dsMr-SID1 and dsGFP injected individuals died during the 24 h following the second injection.
Figure 4. Mr-SID1 expression in response to dsRNA injection and its loss of function effects. (A) dsRNA specificity demonstrated by a comparison between Mr-SID1expression level in dsRNA-injected compared with dsDNA-injected groups (dsRNA and dsDNA contain the same GFP exogenous sequence) and negative control, PBS-injected group (n = 7 in each group). (B) Survival rates of juvenile prawns following dsMr-SID1 injection (with dsGFP). Controls included the PBS-injected group administered a double volume similar to the volume used in the dual injection of 2 dsRNAs, the dsGFP injected group, and the dsGFP and dsRB injected group.
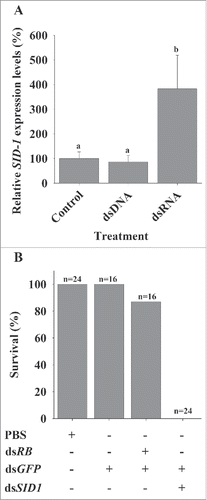
To conclude the study, expression levels of Mr-SID1 and the other components of the RNAi mechanism were tested in response to different fragment lengths of dsMr-IAG based on the same length dependency experiments shown in . The correlation clearly showed that dsMr-IAG fragment length was positively correlated with Mr-SID1 upregulation (). A significant induction of Mr-SID1 expression levels was measured by dsMr-IAG fragment lengths of 100-bp (P-value = 0.01) and 250-bp (P-value = 0.002), and this activity was similar to the upregulation of Mr-SID1 expression levels following injections of the entire ORF (P-value = 0.003 and 0.027, respectively). The administration of a mixture of 19-bp fragments did not cause Mr-SID1 induction, whose expression levels were similar to that of the negative control. The expression levels of Mr-SID1 following the injection of 75-bp fragments of dsMr-IAG were different from those of both the negative control and the entire ORF (P-value < 0.001, for both).
Figure 5. RNAi-associated gene expression levels following injection of Mr-IAG dsRNA of different lengths (19-bp, 75-bp, 100-bp, 250-bp, and entire ORF – 518-bp). Mr-SID (A), Mr-Dicer2 (B) and a reference gene, Mr-Dicer1, from the miRNA pathway (C). Expression levels were tested in the RNA extracted from the length dependency experiments ().
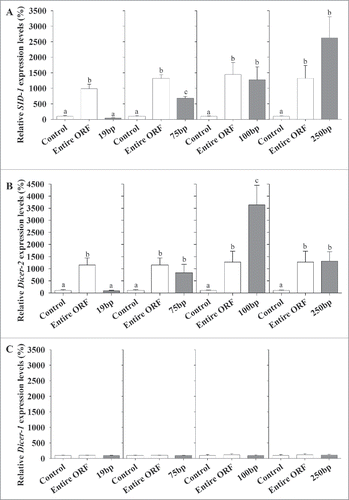
The same correlation shown above with respect to Mr-SID1 and different dsMr-IAG fragment lengths was also found for Mr-Dicer2 with the exception of the induction caused by 100-bp dsRNA fragments (). The expression levels of Mr-Dicer2 in response to 100-bp fragments of dsRNA were significantly higher than the entire ORF injected (P-value = 0.041), and those of both the 100-bp fragment and the entire ORF were significantly higher than that of the non-injected negative control group (P-value < 0.001, both). Administrations of 75-bp and 250-bp fragments of dsRNA elicited higher Mr-Dicer2 expression levels than those of the non-injected group (P-value = 0.005 and P-value < 0.001, respectively) and, in contrast to the results obtained for the 100-bp fragments, showed similar Mr-Dicer2 expression levels to that obtained in response to injection of the entire ORF (p-value = 0.7 and 0.98, respectively). In response to administration of the mixture of 19-bp fragments, the expression levels of Mr-Dicer2, similar to those of Mr-SID1, were not affected, and its expression levels were similar to those in the non-injected group (p-value = 0.99).
As a reference, we used the expression levels of Mr-Dicer1 from the miRNA pathway. This gene remained at the same low levels in all the treatments, thus demonstrating the absence of a correlation with dsMr-IAG fragment length ().
Discussion
The Mr-IAG knockdown pathway
Full and functional sex reversal of male crustaceans into females caused by the use of RNAi demonstrates the vast potential of RNAi-based biotechnologies in aquaculture and environmental applications.Citation3,Citation33,Citation34 The use of such a biotechnology was previously suggested to be safe based on the temporal nature of the intervention and its lack of any overtly apparent long-term consequences.Citation23,Citation35 These studies presented evidence based on social structure, growth performance and masculine reproductive characters at the anatomic and molecular levels. Moreover, our observations that the elapsed times until disappearance of the exogenous dsRNA from prawn tissues and the return of Mr-IAG transcript levels to normal during the post silencing period were comparable, suggesting that RNAi-based technology is temporal and indeed safe.Citation23,Citation35 The current study, which examined the mechanism of the knockdown pathway, provided additional support for the temporal nature of the RNAi-based production of all-male cultures and rejects the possible involvement of permanent or epigenetic interference processes on the genome associated with the transcriptional silencing pathway.
Three known RNAi pathways, i.e., micro RNAs (miRNA), short interfering RNA (siRNA) and PIWI interacting RNA (piRNA), are associated with Argonaute protein family members.Citation12 In the current study, the Dicer and Ago homologs were discovered and fully sequenced in M. rosenbergii. The clustering of each homolog to other members from related species further supports the correct identification of the distinct Mr-Dicer and Mr-Ago members allowing the examination of the response of each homolog transcript level to dsMr-IAG administration. Only transcripts of Mr-Dicer2 and Mr-Ago2, essential components for RNAi response and post-transcriptional silencing, were significantly increased after dsMr-IAG injection. Other transcripts in these families, such as Mr-Ago1 and Mr-Dicer1 associated with nuclear transcriptional silencingCitation16,Citation17 or Ago3 associated with piRNA,Citation21 were not affected by the introduction of dsMr-IAG. Since the Dicer2 and Ago2 proteins are known to be involved in the post-transcriptional pathway where they exert temporal cytoplasmic effects,Citation12,Citation36 the results of the present study support both the temporal nature of the effects of dsMr-IAG administration and the safety of its use in aquaculture and environmental applications.
Post-transcriptional knockdown pathway and dsRNA length
The current study also focused on the ability to knock down Mr-IAG using fragments with lengths shorter than the entire ORF. Gene knockdown in various organisms was demonstrated using short dsRNA fragments (siRNA) for loss of function procedures, mostly in-vitro.Citation37-39 Thus, we attempted to knock down Mr-IAG in-vivo using siRNA. These attempts failed, eliciting the need to examine the correlation between dsRNA length and knockdown success. A clear relationship between dsMr-IAG lengths and Mr-IAG transcript levels was found, in which the longer the administered dsMr-IAG fragment, the lower the Mr-IAG transcript levels. A similar tendency was demonstrated in D. melanogaster.Citation6 To gain insight into this phenomenon, different dsMr-IAG fragment lengths were evaluated for their effects on the expression levels of genes associated with the post-transcriptional knockdown pathway.
To investigate the mechanism of action, Mr-SID1, a passive transmembrane channel that specifically delivers dsRNA into somatic cells,Citation5,Citation7 was sequenced and aligned to orthologs from arthropod species. Mr-SID1 is the main gate for passive dsRNA uptake into the cell and indeed its transcript levels were only upregulated in the presence of dsRNA. The results are supported by those of Shih and Hunter,Citation7 who examined the differences in membrane conductance in SID1-expressing cells in the presence of dsRNA and DNA and showed that conductance changed only in the presence of dsRNA. Based on the amino acid homology of Mr-SID1 to other species with the same predicted 11 transmembrane regions and based on the results of the functional test of Mr-SID1, we suggest that Mr-SID1 is the dsRNA-gated channel in M. rosenbergii.
Yet SID1 is not the only way known for dsRNA delivery into the cytosol. Many metazoan cells can deliver an exogenous dsRNA via receptor mediated endocytosis, an uptake mechanism that seems to be evolutionarily conserved.Citation8 To determine whether Mr-SID1 is the main avenue for the delivery of dsRNA into M. rosenbergii cells, we attempted to knock down SID1, after which we planned to try SID1 knockdown in parallel with dsMr-IAG administration. The latter experiment, however, could not be performed since the effect of dsMr-SID1 administration was lethal unlike the cases of other documented RNAi-associated genes.Citation40,Citation41 It should be noted that dsMr-SID1 was designed based on a wide M. rosenbergii transcriptomic libraryCitation42 and found to be specific to the Mr-SID1 sequence, thereby minimizing the probability of off-target effects. However, due to the lack of a full genome sequence in our study organism, lethality caused by off-target effects could not be completely ruled out. In addition, while we also cannot rule out the possibility that dsRNA was internalized through endocytosis, we suggest that SID1 is a major component in dsRNA trafficking. This is supported by results from SID1 non expressing D. melanogaster S2 cells, in which dsRNA is endocytotically delivered,Citation8 while the expression of SID1 in those cells increases the efficiency of gene knockdown.Citation6 In the M. rosenbergii system, the presence and differential activity of Mr-SID1 seem to suggest that it plays a crucial role in regulation of systemic RNAi and the cell's defense mechanism against viruses.
The present study showed a positive correlation between injected dsMr-IAG fragment length and Mr-SID1 transcript induction level. In contrast to our findings, another study that examined C. elegans SID1 expression in D. melanogaster S2 cells did not find it to be selective to dsRNA length.Citation43 The authors of the latter article suggested that dsRNA length dependency probably does not occur through SID1.Citation43 It is thus suggested that the tendency of Mr-SID1 transcripts to respond to dsRNA lengths is regulated by factors downstream, and not upstream, of the dsRNA delivery, and that it is not self-regulated by the transmembrane channel itself. It is also suggested that dsRNA delivery depends on the dsRNA retention ability of the Dicer-RISC complex. Insofar as SID1 is a passive channel, dsRNA retention by Dicer-RISC is essential to allow the intra-cellular dsRNA concentration to decrease, resulting in more dsRNA molecules passing into the cell through SID1 according to intra- and extra-cellular dsRNA concentrations.Citation43
Mr-Dicer2 transcript levels also changed in response to dsMr-IAG length but without a clear, linear tendency as was found for Mr-SID1. In the case of Mr-Dicer2, the highest upregulation was obtained with dsRNA fragments of 100-bp compared with the other fragment sizes. Moreover, from among the fragment lengths tested, only the 19-bp fragments failed to cause any Mr-Dicer2 upregulation. These results seem to contradict observations of knockdown success, which was better for fragment lengths of 250-bp and 500-bp. It is suggested that Mr-Dicer2 is influenced by the ability of SID1 to deliver the dsRNA molecules and that the level of induction depends on the number of molecules. Among the solutions of the different fragments at the same dsRNA concentration (in this study 5 µg/g body weight), that with the 100-bp fragments contains many more molecules, and this could explain the finding that the highest upregulation obtained here was for Mr-Dicer2.
Concluding remarks
For the first time, the Dicer and Argonaute genes were fully sequenced in M. rosenbergii, which enabled us to elucidate the knockdown pathway of Mr-IAG, the target of the manipulation that causes the full and functional sex reversal of M. rosenbergii males into females. This study showed that the dsMr-IAG-based manipulation manifests through a temporal, post-transcriptional pathway, the well-known siRNA pathway. The temporal nature of the latter pathway further supports the safety of using such RNAi-based biotechnologies in aquaculture and environmental applications.
In this study, RNAi-based manipulation of the crustacean insulin-like androgenic hormone also demonstrated a clear dependency on dsRNA molecule length, such that dsMr-IAG fragment length was negatively correlated with Mr-IAG transcript level. Moreover, the induction of genes associated with the post-transcriptional pathway and SID1 was also differentially influenced according to dsMr-IAG fragment length. Unlike numerous clear cases of RNAi driven by the administration of small dsRNA fragments in-vitro, the case presented here demonstrates length dependency when the experiments are performed in-vivo. Our results thus support the need for further study of RNAi phenomena in the context of the entire organism.
Materials and methods
Animals
M. rosenbergii prawns of the BGU-line were maintained at Ben-Gurion University of the Negev (BGU) facilities as described by Shpak et al.Citation44 The all-male progenies originating from crosses between males and 'neo-females' were supplied by the Tiran Shipping group through its subcontractor hatchery, Colors Ltd., Hatzeva, Israel. This hatchery holds only genetic males form the BGU line, and genetic females have never been allowed at this farm.
All dsRNA length dependency experiments were performed using small males whose morphotype was determined according to Kuris et al.Citation45 It should be noted that small males are sexually active and have an active androgenic gland.Citation46
Mr-SID1 loss of function and specific induction of Mr-SID1 by dsRNA experiments were performed using post larvae all-male progeny from the all-male hatchery mentioned above.
Synthesis of double-stranded RNA
Eight dsRNA fragments of 19-bp and two fragments of 75-bp from the Mr-IAG mRNA sequence were synthesized and supplied by BioSpring, Frankfurt, Germany (Fig. S1A). Five dsRNA fragments of 100-bp covering approximately the entire Mr-IAG ORF and one fragment of 250-bp were synthesized in-vitro in our laboratory (Fig. S1A). A pGEM®-T Easy plasmid containing the Mr-IAG open reading frame sequence served as the template for dsMr-IAG synthesis. The template was amplified by PCR, primed by two gene-specific primers with a T7 promotor site at the 5' of one primer (T7P) (see primers and T7 promotor sequences for dsRNA synthesis in Table S1). Primer pairs were as follows: the sense strand was synthesized using primer T7P forward vs. reverse primer, while the antisense strand was synthesized by T7P reverse vs. forward primer. PCR amplicons were electrophoresed on a 1.3% agarose gel and visualized with ethidium bromide and UV light, excised from the gel, and purified with a Accuprep® PCR purification Kit (BIONEER Co., Daejeon, South Korea).
The TranscriptAid T7 High Yield Transcription Kit (Thermo Scientific, Lithuania) was used to generate single-stranded RNA according to the manufacturer's instructions. RNA molecules were purified by phenol-chloroform (1:1) and ammonium-acetate and precipitated with ethanol. Sense and antisense strands were hybridized by incubation at 70°C for 15 min, 65°C for 15 min and at RT for 30 min. dsRNA quality was assessed on an agarose gel and diluted to 5 µg/µl. dsRNA was kept at −80°C until used.
Mr-IAG knockdown attempts using fragments shorter than the entire ORF
Four distinct experiments were performed, one for each dsRNA length (19-bp, 75-bp, 100-bp, and 250-bp). Each experiment included a non-injected group as a negative control and an entire dsMr-IAG ORF injected group as a positive control. It is important to note that the determination of which animals would constitute the non-injected control groups was based on previous studies, which also used exogenous dsRNA or DDW injection as described in Lezer et al.Citation35 and in Sharabi et al.,Citation42 with similar results. Each experimental group included 6–8 small M. rosenbergii males (Mean body weight = 12.5 g). Single injections of the different dsMr-IAG lengths (5µg dsRNA/gr body weight) were performed once, and then 48 hours post-injection, prawns were anesthetized in ice cold water, dissected and total RNA was extracted from their androgenic glands using the EZ-RNA Total RNA Isolation Kit (Biological Industries, Beit Haemek, Israel) according to the manufacturer's instructions. cDNA was prepared in a reverse-transcriptase reaction containing 1 μg total RNA using a qScript cDNA synthesis kit (Quanta Biosciences, Gaithersburg, MD, USA) according to the manufacturer's instructions. Relative quantification of Mr-IAG expression levels was obtained as described in Ventura et al.Citation22 with the FastStart Universal Probe Master (Rox; Roche Diagnostics GmbH) and Universal ProbeLibrary Probe 144 (Roche). Mr-18S (GenBank accession no. GQ131934) was used as a normalizing gene and quantified using specific primers as described in Ventura et al.Citation22 with the above-mentioned mix and Universal ProbeLibrary Probe 152 (Roche). Reactions were performed with the ABI Prism 7300 Sequence Detection System (Applied Biosystems).
RNAi associated gene mining
RNAi associated-genes were mined from our composite M. rosenbergii transcriptomic library, which contains more than 290 million reads assembled into 108212 contigs, fully described by Ventura, et al.Citation47 and Sharabi, et al.Citation42 The mining was done based on orthologs from related crustacean species using a BLAST search to reveal Dicer and Ago homologs and SID1sequences. M. rosenbergii-Dicer1 (Mr-Dicer1) was compared with P. monodon Dicer1 (GenBank accession number ABR14013.1) and Mr-Dicer2 was compared with L. vannamei Dicer2 (GenBank accession number ACF96960.1). Mr-Argonaute1 was compared with M. japonicus Argonaute1 (GenBank accession number ADB44074.1). Mr-Argonaute2 was compared with M. japonicus Argonaute2 (GenBank accession number AB665954.1). Mr-Argonaute3 was compared with P. monodon Argonaute3 (GenBank accession number AGC95229.1). M. rosenbergii-SID1 was compared L. vannamei-SID1 (GenBank accession number HM234688.1).
Putative sequences obtained from the library were computationally translated using the ExPASy Proteomics Server (http://ca.expasy.org/tools/dna.html), and the longest open reading frame was selected. Transmembrane regions of Mr-SID1 were evaluated using the Simple Modular Architecture Research Tool (SMART) (http://smart.embl-heidelberg.de/).Citation48
In-vitro validation and sequencing
Total RNA was extracted from the hepatopancreas of dsMr-IAG-injected [5µg/µl] animals, while anesthetized in ice-cold water, 48 h post-injection. Total RNA was isolated and cDNA was prepared as described above.
Putative Mr-Dicers (Mr-Dicer1,2), Mr-Agos (Mr-Ago1,2,3) and Mr-SID1 sequences were in-vitro validated by PCR using gene specific primers (Table S2). The PCR products were cloned and sequenced as described by Ventura, et al.Citation28 Mr-Dicer2 and Mr-SID1 5' end were obtained by 5' rapid amplification of cDNA ends (RACE 5') performed with the Clontech SMART RACE kit (BD Biosciences, Palo Alto, CA) following the manufacturers protocol. PCR was performed using a gene-specific reverse primer (Table S3) and the Universal Primers Mix (UPM) provided in the kit. To confirm the obtained sequence, 5' RACE product was amplified by PCR using a reverse nested primer closer to the 5' end (Table S3) and a UPM nested primer, also provided in the kit. RACE 5' and nested 5' products were cloned and sequenced as described above. To ensure the quality of the sequence, each region of the gene was sequenced four times.
The 3' end of Mr-Ago3 was obtained by 3' rapid amplification of cDNA ends (RACE) as described above using a gene-specific forward primer (Table S3) and a UPM provided in the kit, and the PCR products were cloned and sequenced. To confirm the obtained sequence, the 3' RACE product was amplified by PCR using a forward nested primer closer to the 3' end (Table S3). This product was cloned and sequenced as described above. To ensure the quality of the sequence, each region of the gene was sequenced four times.
Phylogenetic analysis
Known sequences of Dicer and Ago from related Crustacean species and SID1 sequences from different species were obtained from the GenBank database. A phylogenetic neighbor-joining tree was constructed using Mega 6.0 software (Molecular Evolutionary Genetics Analysis, version 6.0) according to ClustalW multiple sequence alignments of the putative amino acid sequences of Dicer, Argonaute and SID1. Bootstrap values (%) of 1000 replicates were calculated for each node of the consensus tree attained.Citation49
Mr-IAG knockdown pathway determination
To elucidate the Mr-IAG knockdown pathway, 15 small M. rosenbergii males (mean body weight ∼15 gr) were divided into two groups, the non-injected group (n = 8) and the injected group (n = 7), whose members were injected with the entire dsMr-IAG ORF [5µg dsRNA/gr body weight] by a micro injector. Androgenic glands and hepatopancreases were dissected for total RNA extraction, 48 h post injection as described above. Total RNA was isolated and cDNA was prepared as described above. Mr-IAG expression levels were detected from total RNA extracted from androgenic glands to verify Mr-IAG knockdown success. Relative quantification of Mr-IAG expression levels was obtained as described above.
Relative quantification of Mr-Dicer (Mr-Dicer1,2) and Mr-Argonaute (Mr-Ago1,2,3) expression levels were tested using the total RNA isolated from the hepatopancreas, preliminarily showing high expression levels. Mr-Dicers and Mr-Argonautes expression levels were obtained using forward and reverse primers (Table S4) with FastStart Universal Probe Master (Rox; Roche Diagnostics GmbH) and Universal ProbeLibrary Probes (Roche) (Table S4). For all these relative quantifications, Mr-18S was also quantified by real-time RT PCR as a normalizing gene as described above.
Double-strand RNA and Mr-SID1 induction
To confirm the role of SID1 in M. rosenbergii, juvenile males (n = 22) (mean weight = 0.22 gr) were divided into three groups: phosphate-buffered saline (PBSX1) injected (n = 8), DNA-GFP injected (n = 7) and dsRNA-GFP injected (n = 7). dsRNA-GFP was synthesized as described above. DNA fragments that contained the same GFP sequence were amplified by PCR. PCR products were purified using NucleoSpin® Gel and PCR Clean-up (MACHEREY-NAGEL GmbH & Co., Düren, Germany). The procedure included two injections of 5µg per g body weight each every 24 h. Prawns were anesthetized in ice cold water and their hepatopancreases were dissected 24h post second injection. Total RNA was extracted and cDNA was prepared as described above. Relative quantification of Mr-SID1 expression levels was performed as described above (for primers and probe, see Table S4).
The effect of Mr-SID1 knockdown
With the aim of examining the crucial role of Mr-SID1 to gene knockdown in M. rosenbergii, the effect of Mr-SID1 knockdown was studied. Forty juvenile males (mean body weight = 0.67 gr) were divided to four groups: DDW injected, dsGFP injected, dual injection group of dsRB and dsGFP (two exogenous dsRNA) and dual injection group of dsGFP and dsMr-SID1 (the purpose of dsGFP was to induce Mr-SID1 expression level). The procedure included two injections of 10µg mixed dsRNA per g body weight with 24 h interval and survival rates were monitored. Synthesis of dsGFP was done as described above. dsRB, an exogenous gene, was synthesized as described by Lezer et al.Citation35 The synthesis of dsMr-SID1 was done as described above using specific primers (Table S1)
RNAi-associated genes expression levels as a response to different dsMr-IAG length
RNAi-associated gene expression levels (Mr-SID1, Mr-Dicer2 and Mr-Dicer1) were measured using the same RNA isolated from the prawns that was used in the Mr-IAG knockdown attempts, with the shorter dsMr-IAG fragments described above including control groups. Mr-SID1, Mr-Dicer2 and Mr-Dicer1 were quantified by real-time RT-PCR using the above described primers and Universal ProbeLibrary Probes ve.
Statistical analysis
Final real-time RT-PCR relative quantification values (RQ) were calculated using the formula 2−ddCt (built-in feature in the ABI Prism 7300 Sequence Detection System, Applied Biosystems) for analyzing qPCR results. The RQ values in the different experiments were analyzed by Statistica v12.0 software (StatSoft, Ltd., Tulsa, OK, USA). For all multiple comparisons, the tests were selected according to assumptions of homogeneity of the variances through Levene's test and normal distribution of the residuals as accepted. For all comparisons between two groups, the tests were selected according to the normal distribution of the samples and the homogeneity of the variances through a P-variance test.
The results of knockdown pathway elucidation experiments were analyzed using a t-test for independent variables for Mr-IAG, Mr-Dicer2 and Mr-Ago1 and the Mann-Whitney U-test for the results of Mr-Dicer1, Mr-Ago2 and Mr-Ago3. The results of evaluations of Mr-IAG response to shorter dsRNA fragment lengths were analyzed using One-way ANOVA after log(X) transformation and Post-hoc Tukey HSD for 100-bp and 250-bp experiments, One-Way ANOVA using welch correction and Tukey HSD for unequal samples for the 75-bp experiment, and Kruskal-Wallis was used for the 19-bp mixture experiment. The differences in Mr-SID1 expression levels were analyzed using One-way ANOVA after log(X+1) transformation and Post-hoc Tukey HSD. The correlation between dsRNA lengths and Mr-SID1 expression were analyzed using the Kruskal-Wallis test for the 19-bp, 100-bp and 250-bp experiments. One-Way ANOVA using welch correction and Tukey HSD for unequal samples were used to examine the correlation between 75-bp fragments and the Mr-SID1 transcript. The correlation between dsRNA length and Mr-Dicer2 were analyzed using One-way ANOVA after log(X+1) transformation and Post-hoc Tukey HSD.
Suppl_mat.zip
Download Zip (418.7 KB)Acknowledgments
This study was supported in part by the United States–Israel Binational Agricultural Research and Development Fund (BARD, Grant No. IS-4493–12), the Israel Science Foundation (ISF) within the ISF-UGC joint research program framework (grant No. 2728/16) and the Israel Science Foundation and the National Natural Science Foundation of China Grant 605/14. All-male progeny were supplied by Tiran Shipping, through their subcontractor Colors Ltd., Hatzeva, Israel.
References
- Saleh MC, Tassetto M, van Rij RP, Goic B, Gausson V, Berry B, Jacquier C, Antoniewski C, Andino R. Antiviral immunity in Drosophila requires systemic RNA interference spread. Nature. 2009;458:346–50. doi:10.1038/nature07712.
- Fire A, Xu S, Montgomery MK, Kostas SA, Driver SE, Mello CC. Potent and specific genetic interference by double-stranded RNA in Caenorhabditis elegans. Nature. 1998;391:806–11. doi:10.1038/35888.
- Sagi A, Manor R, Ventura T. Gene silencing in crustaceans: From basic research to biotechnologies. Genes. 2013;4:620–45. doi:10.3390/genes4040620.
- Czech MP, Aouadi M, Tesz GJ. RNAi-based therapeutic strategies for metabolic disease. Nat Rev Endocrinol. 2011;7:473–84. doi:10.1038/nrendo.2011.57.
- Winston WM, Molodowitch C, Hunter CP. Systemic RNAi in C-elegans requires the putative transmembrane protein SID-1. Science. 2002;295:2456–9. doi:10.1126/science.1068836.
- Feinberg EH, Hunter CP. Transport of dsRNA into cells by the transmembrane protein SID-1. Science. 2003;301:1545–7. doi:10.1126/science.1087117.
- Shih JD, Hunter CP. SID-1 is a dsRNA-selective dsRNA-gated channel. Rna-a Publication of the Rna Society. 2011;17:1057–65. doi:10.1261/rna.2596511.
- Saleh M-C, van Rij RP, Hekele A, Gillis A, Foley E, O'Farrell PH, Andino R. The endocytic pathway mediates cell entry of dsRNA to induce RNAi silencing. Nat Cell Biol. 2006;8:793–802. doi:10.1038/ncb1439.
- Elbashir SM, Lendeckel W, Tuschl T. RNA interference is mediated by 21-and 22-nucleotide RNAs. Gene Dev. 2001;15:188–200. doi:10.1101/gad.862301.
- Dykxhoorn DM, Novina CD, Sharp PA. Killing the messenger: Short RNAs that silence gene expression. Nat Rev Mol Cell Biol. 2003;4:457–67. doi:10.1038/nrm1129.
- Carthew RW, Sontheimer EJ. Origins and Mechanisms of miRNAs and siRNAs. Cell. 2009;136:642–55. doi:10.1016/j.cell.2009.01.035.
- Ender C, Meister G. Argonaute proteins at a glance. J Cell Sci. 2010;123:1819–23. doi:10.1242/jcs.055210.
- Lee Y, Ahn C, Han JJ, Choi H, Kim J, Yim J, Lee J, Provost P, Rådmark O, Kim S, et al. The nuclear RNase III Drosha initiates microRNA processing. Nature. 2003;425:415–9. doi:10.1038/nature01957.
- Kadener S, Rodriguez J, Abruzzi KC, Khodor YL, Sugino K, Marr MT, Nelson S, Rosbash M. Genome-wide identification of targets of the drosha-pasha/DGCR8 complex. Rna-a Publication of the Rna Society. 2009;15:537–45. doi:10.1261/rna.1319309.
- Kim VN, Han J, Siomi MC. Biogenesis of small RNAs in animals. Nat Rev Mol Cell Biol. 2009;10:126–39. doi:10.1038/nrm2632.
- Okamura K, Ishizuka A, Siomi H, Siomi MC. Distinct roles for Argonaute proteins in small RNA-directed RNA cleavage pathways. Gene Dev. 2004;18:1655–6. doi:10.1101/gad.1210204.
- Janowski BA, Huffman KE, Schwartz JC, Ram R, Nordsell R, Shames DS, Minna JD, Corey DR. Involvement of AGO1 and AGO2 in mammalian transcriptional silencing. Nat Struct Mol Biol. 2006;13:787–92. doi:10.1038/nsmb1140.
- Noma K, Sugiyama T, Cam H, Verdel A, Zofall M, Jia ST, Moazed D, Grewal SI. RITS acts in cis to promote RNA interference-mediated transcriptional and post-transcriptional silencing. Nat Genet. 2004;36:1174–80. doi:10.1038/ng1452.
- Moazed D. Small RNAs in transcriptional gene silencing and genome defence. Nature. 2009;457:413–20. doi:10.1038/nature07756.
- Verdel A, Jia ST, Gerber S, Sugiyama T, Gygi S, Grewal SIS, Martienssen RA, Yanagida M. RNAi-mediated targeting of heterochromatin by the RITS complex. Science. 2004;303:672–6. doi:10.1126/science.1093686.
- Thomson T, Lin HF. The biogenesis and function of PIWI proteins and piRNAs: progress and prospect. Annu Rev Cell Dev Biol. 2009;25:355–76. doi:10.1146/annurev.cellbio.24.110707.175327.
- Ventura T, Manor R, Aflalo ED, Weil S, Rosen O, Sagi A. Timing sexual differentiation: Full functional sex reversal achieved through silencing of a single insulin-like gene in the prawn, Macrobrachium rosenbergii. Biol Reprod. 2012;86:90. doi:10.1095/biolreprod.111.097261.
- Shpak N, Manor R, Aflalo ED, Sagi A. Three generations of cultured prawn without W chromosome. Aquaculture. 2017;467:41–8. doi:10.1016/j.aquaculture.2016.06.008
- Aflalo E, Hoang T, Nguyen V, Lam Q, Nguyen D, Trinh Q, Raviv S, Sagi A. A novel two-step procedure for mass production of all-male populations of the giant freshwater prawn Macrobrachium rosenbergii. Aquaculture. 2006;256:468–78. doi:10.1016/j.aquaculture.2006.01.035
- Nair CM, Salin KR, Raju MS, Sebastian M. Economic analysis of monosex culture of giant freshwater prawn (Macrobrachium rosenbergii De Man): A case study. Aquac Res. 2006;37:949–54. doi:10.1111/j.1365-2109.2006.01521.x.
- Manor R, Weil S, Oren S, Glazer L, Aflalo ED, Ventura T, Chalifa-Caspi V, Lapidot M, Sagi A. Insulin and gender: An insulin-like gene expressed exclusively in the androgenic gland of the male crayfish. Gen Comp Endocrinol. 2007;150:326–36. doi:10.1016/j.ygcen.2006.09.006.
- Ventura T, Rosen O, Sagi A. From the discovery of the crustacean androgenic gland to the insulin-like hormone in six decades. Gen Comp Endocrinol. 2011;173:381–8. doi:10.1016/j.ygcen.2011.05.018.
- Ventura T, Manor R, Aflalo ED, Weil S, Raviv S, Glazer L, Sagi A. Temporal silencing of an androgenic gland-specific insulin-like gene affecting phenotypical gender differences and spermatogenesis. Endocrinology. 2009;150:1278–86. doi:10.1210/en.2008-0906.
- Labreuche Y, Warr GW. Insights into the antiviral functions of the RNAi machinery in penaeid shrimp. Fish Shellfish Immunol. 2013;34:1002–10. doi:10.1016/j.fsi.2012.06.008.
- Dowling D, Pauli T, Donath A, Meusemann K, Podsiadlowski L, Petersen M, Peters RS, Mayer C, Liu S, Zhou X, et al. Phylogenetic origin and diversification of RNAi pathway genes in insects. Genome Biology and Evolution. 2017;8(12):3784–3793. doi:10.1093/gbe/evw281..
- Rao DD, Vorhies JS, Senzer N, Nemunaitis J. siRNA vs. shRNA: Similarities and differences. Adv Drug Delivery Rev. 2009;61:746–59. doi:10.1016/j.addr.2009.04.004.
- van Rij RP, Andino R. The silent treatment: RNAi as a defense against virus infection in mammals. Trends Biotechnol. 2006;24:186–93. doi:10.1016/j.tibtech.2006.02.006.
- Aflalo ED, Dandu RVSN, Verghese JT, Rao N, Samraj TYC, Ovadia O, Sagi A. Neo-females production and all-male progeny of a cross between two Indian strains of prawn (Macrobrachium rosenbergii): Population structure and growth performance under different harvest strategies. Aquaculture 2014;428:7–15. doi:10.1016/j.aquaculture.2014.02.021
- Alkalay AS, Rosen O, Sokolow SH, Faye YPW, Faye DS, Aflalo ED, Jouanard N, Zilberg D, Huttinger E, Sagi A. The prawn Macrobrachium vollenhovenii in the senegal river basin: Towards sustainable restocking of all-male populations for biological control of schistosomiasis. PLoS Negl Trop Dis. 2014;8:e3060. doi:10.1371/journal.pntd.0003060.
- Lezer Y, Aflalo ED, Manor R, Sharabi O, Abilevich LK, Sagi A. On the safety of RNAi usage in aquaculture: The case of all-male prawn stocks generated through manipulation of the insulin-like androgenic gland hormone. Aquaculture. 2015;435:157–66. doi:10.1016/j.aquaculture.2014.09.040
- Meister G, Tuschl T. Mechanisms of gene silencing by double-stranded RNA. Nature. 2004;431:343–9. doi:10.1038/nature02873.
- Myers JW, Ferrell JE. Silencing gene expression with Dicer-generated siRNA pools. RNA Silencing: Methods and Protocols. 2005:93–196. doi:10.1385/1-59259-935-4:093.
- Xie JF, Lu L, Deng M, Weng SP, Zhu JY, Wu Y, Gan L, Chan SM, He J. Inhibition of reporter gene and Iridovirus-tiger frog virus in fish cell by RNA interference. Virology. 2005;338:43–52. doi:10.1016/j.virol.2005.04.040.
- Dang LT, Kondo H, Hirono I, Aoki T. Inhibition of red seabream iridovirus (RSIV) replication by small interfering RNA (siRNA) in a cell culture system. Antivir Res. 2008;77:142–9. doi:10.1016/j.antiviral.2007.10.007.
- Dechklar M, Udomkit A, Panyim S. Characterization of Argonaute cDNA from Penaeus monodon and implication of its role in RNA interference. Biochem Biophys Res Commun. 2008;367:768–74. doi:10.1016/j.bbrc.2008.01.031.
- Wang S, Chen AJ, Shi LJ, Zhao XF, Wang JX. TRBP and eIF6 homologue in Marsupenaeus japonicus play crucial roles in antiviral response. PLoS ONE. 2012;7:e30057. doi:10.1371/journal.pone.0030057.
- Sharabi O, Manor R, Weil S, Aflalo ED, Lezer Y, Levy T, Aizen J, Ventura T, Mather PB, Khalaila I, et al. Identification and characterization of an insulin-like receptor involved in crustacean reproduction. Endocrinology. 2016;157:928–41. doi:10.1210/en.2015-1391.
- Shih JD, Fitzgerald MC, Sutherlin M, Hunter CP. The SID-1 double-stranded RNA transporter is not selective for dsRNA length. RNA. 2009;15:384–90. doi:10.1261/rna.1286409.
- Shpak N, Manor R, Aflalo ED, Sagi A. Three generations of cultured prawn without W chromosome. Aquaculture. 2017;467:41–48. doi:10.1016/j.aquaculture.2016.06.008.
- Kuris AM, Raanan Z, Sagi A, Cohen D. Morphotypic differentiation of male malaysian giant prawns, Macrobrachium rosenbergii. J Crustac Biol. 1987;7:219–37. doi:10.2307/1548603
- Ventura T, Manor R, Aflalo ED, Weil S, Khalaila I, Rosen O, Sagi A. Expression of an androgenic gland-specific insulin-like peptide during the course of prawn sexual and morphotypic differentiation. ISRN endocrinology. 2011;2011:476283. doi:10.5402/2011/476283.
- Ventura T, Manor R, Aflalo ED, Chalifa-Caspi V, Weil S, Sharabi O, Sagi A. Post-embryonic transcriptomes of the prawn Macrobrachium rosenbergii: Multigenic succession through metamorphosis. PLoS ONE. 2013;8:e55322. doi:10.1371/journal.pone.0055322.
- Schultz J, Milpetz F, Bork P, Ponting CP. SMART, a simple modular architecture research tool: Identification of signaling domains. Proc Natl Acad Sci U S A. 1998;95:5857–64. doi:10.1073/pnas.95.11.5857.
- Tamura K, Stecher G, Peterson D, Filipski A, Kumar S. MEGA6: Molecular evolutionary genetics analysis version 6.0. Mol Biol Evol. 2013;30:2725–9. doi:10.1093/molbev/mst197.