ABSTRACT
The σ subunit of bacterial RNA polymerase is required for promoter recognition during transcription initiation but may also regulate transcription elongation. The principal σ70 subunit of Escherichia coli was shown to travel with RNA polymerase and induce transcriptional pausing at promoter-like motifs, with potential regulatory output. We recently demonstrated that an alternative σ38 subunit can also induce RNA polymerase pausing. Here, we outline proposed regulatory roles of σ-dependent pausing in bacteria and discuss possible interplay between alternative σ variants and regulatory factors during transcription elongation.
The problem of transcription initiation
Recognition of promoter sequences by RNA polymerase (RNAP) is a prerequisite for gene-specific transcription initiation but it also imposes an inevitable problem for RNA synthesis since persisting RNAP-promoter contacts would prevent RNA elongation, as well as subsequent rounds of transcription initiation. The stronger contacts with the promoter are, the harder it becomes to break them during transition to productive elongation.Citation1,2 This problem is solved in part by using dedicated promoter-specificity factors, which only transiently associate with RNAP for transcription initiation and dissociate during promoter escape. (The use of specialized factors is not required for transcription initiation by single-subunit phage RNAPs but they face much less need for transcription regulation.) In bacterial RNAP, the main function in promoter recognition is played by the σ subunit, and multiple transcription activators can also contribute to RNAP recruitment at regulated promoters. Modular recognition of promoters through specific contacts with several DNA elements recognized by the σ subunit, RNAP core enzyme, and transcription activators may facilitate stepwise breakage of these contacts during transcription initiation. Promoter escape is also facilitated by direct competition between the nascent RNA and the σ subunit. Conserved region 3.2 of the σ subunit (σ finger), which approaches the RNAP active site, imposes a block for RNA extension beyond 6 nucleotides,Citation3-5 and its extrusion from the RNA exit channel by the growing RNA induces σ dissociation and promoter complex disassembly.Citation6-8
σ70-dependent pausing
The principal σ70 subunit of E. coli was recognized as a bona fide transcription elongation factor two decades ago, when it was shown to induce promoter-proximal pausing at lambdoid phage genes.Citation9 Later in vitro studies unambiguously established that σ70 can remain stably bound to the transcription elongation complex (TEC) after transcription initiation from various tested promoters, through contacts of its regions 1.2 through 2.4 with the β′ clamp-helixes of core RNAP.Citation10-14 Further in vivo analysis revealed significant association of σ70 with transcribed genomic regions, suggesting a stochastic model of σ dissociation during initial transcription,Citation15-17 with many opportunities for σ to induce pausing. Indeed, analysis of σ-dependent pauses at selected promoter-proximal regions suggested that it is a ubiquitous phenomenon,Citation18 with up to 20% of all cellular promoters containing potential pause-inducing signals.Citation19 The phenomenon of σ-dependent pausing is unlikely to be limited to E. coli but may also exist in other bacteria. We demonstrated that the principal σA subunits from Thermus aquaticus or Deinococcus radiodurans can indeed induce pausing by their cognate RNAPs at consensus pause sites in vitro.Citation20,21
The molecular mechanism of σ70-dependent pausing has been studied in much detail ().Citation22 During pausing, σ70 first recognizes a promoter-like -10 element (TATAAT consensus) in the nontemplate DNA strand, which can be complemented by upstream TGCitation23 and -35 motifs.Citation24,25 Further RNA extension by several nucleotides is accompanied by formation of stressed TECs with scrunched DNA, due to anchoring of RNAP at the -10-like element by σ. The half-life of such complexes can be increased by an elemental pause that occurs during RNA extension, followed by either pause escape or RNAP backtracking.Citation26 Backtracked complexes are then reactivated through RNA cleavage in the RNAP active site (stimulated by Gre proteins) and can enter the next pausing cycle.Citation27 Accordingly, σ-dependent pausing can be modulated by any factors that affect backtracking.Citation23 In particular, we recently showed that Gre factor homologue (Gfh) proteins from the radioresistant bacterium Deinococcus radiodurans, which bind within the secondary channel of RNAP, stimulate RNAP backtracking and increase σA-dependent pausing ((20) and our unpublished observations).
Figure 1. Proposed scenarios for σ-dependent regulation of transcription elongation. (A) σ can remain bound to the TEC and induce pausing, dissociate and rebind RNAP during RNA elongation. (B) σ retention in the TEC may prevent binding of competing transcription factors, such as RfaH and NusG, which regulate transcriptional pausing and termination. (C) σ-dependent pausing serves to recruit transcription factors, such as λQ, to the TEC. (D) Promoter-proximal pausing and TEC arrest may inhibit the next round of transcription initiation and suppress recognition of cryptic promoters. (E) Promoter-proximal pausing may prevent binding of transcription repressors and facilitate continuous transcription initiation.
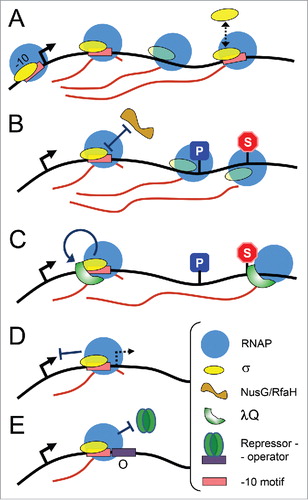
In principle, σ could access the TEC either in cis, without dissociating during transcription initiation,Citation9,28 or in trans, by binding to σ-free TECs.Citation14,23,29-31 In support of the in cis action, mutations in σ region 3.2 increase σ70-dependent promoter-proximal pausing in vitro, likely by impairing σ dissociation during promoter escape.Citation8 Since other factors, such as NusG or RfaH, likely compete with σ for TEC binding (see below), the in cis scenario of σ action is also more probable for the in vivo system. At the same time, overexpression of σ70 allows it to recognize pause-inducing motifs in trans, even at genes controlled by alternative σ subunits,Citation30 thus extending its potential regulatory network.
σ38-dependent pausing
Five out of six alternative σ subunits of E. coli RNAP belong to the σ70 family, but their possible involvement in σ-dependent pausing was never studied in detail. Only σ38 was tested, and it did not induce pausing at a lambdoid phage promoter-proximal regionCitation28 or at a promoter-distal consensus pause signalCitation31 on σ70-dependent templates. It was therefore proposed that σ38 may not be able to interact with the TEC due to substitutions in its core-interacting region 2.Citation31
In contrast, we have revealed efficient σ38-dependent pausing on both synthetic and natural σ38 promoter templates and demonstrated that σ38 binds the TEC with almost the same affinity as σ70.Citation32 The parameters of σ38-dependent pausing are similar to σ70-dependent pausing in terms of DNA recognition, paused complex structure and its sensitivity to transcription factors. σ70 and σ38 recognize similar pause-inducing motifs, in parallel with their similar promoter specificities. The σ38-pause formation is highly sensitive to changes in both the pause motif and the σ-core interface, is accompanied by RNAP backtracking, and is suppressed by Gre factors.Citation32
A prominent fraction (up to 30%) of σ38 promoters contains potential pause-inducing signals, and we indeed revealed efficient σ38-dependent pausing in promoter-proximal regions of two out of four tested templates, adhE and ecnB (). The pause observed on the adhE template is exceptionally long-lived (t1/2>>10 min), so that the complexes become essentially inactivated in the absence of Gre factors.Citation32 Pausing is insensitive to changes in σ38 concentration, while deletion of σ38 region 3.2 increases its efficiency, suggesting that σ38 likely acts in cis, by remaining bound to RNAP after transcription initiation. Such mechanism may prevent binding of competing transcription factors (including σ70) to the TEC during RNA elongation.
Figure 2. Possible effects of σ38-dependent pausing on repressor binding. (A) Sequences of the initially transcribed regions of adhE and ecnB promoters. The transcription start points are blue; the -10-like pause-inducing motifs are pink; the pause sites are light green; the Cra and OmpR binding sites are indicated. (B) In vivo analysis of the adhE P1 activity. E. coli cells were transformed with plasmids containing either wild-type or +11G adhE P1 variants (positions from -164 to +56 relative to the start of transcription), cloned upstream of the luxCDABE operon from Photorhabdus luminescens (shown on the top). The cultures were grown in LB medium containing 0.1 M MOPS, pH 7.4, 0.2% glucose and kanamycin in anaerobic conditions for 3, 3.5, 4 and 7 hours prior to measurements of OD600 and luminescence values. For each time interval, the luminescence was normalized to the OD values; the experiments were independently repeated three times and the OD and normalized luminescence values were averaged.
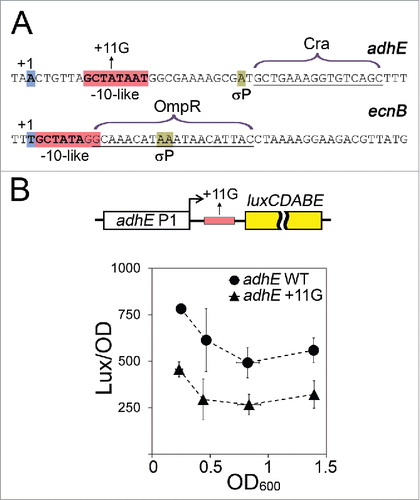
Proposed regulatory roles of σ-dependent pausing
Despite the many years of research, very limited information is available on possible functions of σ-dependent pausing in bacterial cells. Below, we outline already proven as well as speculative mechanisms of transcription regulation that involve σ-dependent pausing.
(1) | The presence of pause-inducing signals during initial transcription was shown to stabilize σ binding to the TEC and increase the probability of σ staying associated with the TEC in downstream operon regions ().Citation12,19 Under certain conditions, σ may also re-associate with the TEC to recognize pause-inducing signals.Citation30 Increased σ occupancy, in its turn, may modulate the properties of transcribing RNAP and influence recognition of pausing and termination signals (in particular, increase σ-dependent pausing in promoter-distal operon parts).Citation12,19 σ-associated TECs were also shown to have higher rates of multiple-round transcription,Citation11,33 suggesting that σ-TEC interactions may increase RNAP turnover, either by facilitating dissociation of termination complexes or decreasing the time needed for holoenzyme formation. Yet, any effect of σ association on gene expression in vivo remains to be demonstrated, since previous studies revealed no changes in the expression of a reporter gene in response to substitutions in the pause-inducing signal.Citation19 | ||||
(2) | In vivo, σ binding to the TEC can be suppressed by competing transcription elongation factors, including universal factor NusG and its gene-specific paralogue RfaH, both of which interact with the same site of core RNAP as σ (β′ clamp helixes). Both factors have no effect on transcription initiation but can effectively prevent σ re-association with the TEC during transcription elongation.Citation15,31 On the opposite, increased σ retention at genes containing pause-inducing signals may prevent binding of NusG or RfaH to the TEC (). Since NusG plays general roles in coordination of transcription with translation, by directly interacting with the ribosome, and in ρ-dependent termination, by directly interacting with the ρ factor, its exclusion from the TEC by σ might have profound (but as yet undiscovered) effects on gene expression. RfaH is recruited to the TEC through specific recognition of ops sites and is required for operon-specific suppression of transcriptional pausing and termination, and stimulation of translation.Citation34 Only one of RfaH-responsive promoters that contain ops-sites in E. coliCitation35 also contains a probable σ-pause signal in its initially transcribed region. This may help to decrease σ retention at such genes, which would otherwise prevent RfaH binding. Interestingly, B. subtilis NusG can by itself recognize specific motifs in the nontemplate DNA strand and increase pausing.Citation36,37 Thus, recognition of nontemplate DNA by transcribing RNAP and associated factors is a common theme in bacterial transcription. | ||||
(3) | The σ subunit may help to recruit other transcription factors to the TECs (), the only regulatory mechanism that was firmly established to date. In the case of lambdoid phage promoters, the σ70-dependent pause brings in antitermination protein Q that associates with transcribing RNAP and suppresses transcriptional pausing and termination, thus allowing transcription of downstream operon genes.Citation9,22 Although highly elegant, this system remains the only example of σ-dependent factor recruitment to the TEC. Interestingly, RNAP stalled at a pause site was recently shown to attract the transcription-repair coupling factor Mfd, thus facilitating repair of downstream DNA lesions.Citation38 σ-dependent pausing might similarly induce Mfd binding, while preventing RNAP interactions with another nucleotide excision factor, UvrD. It remains to be established whether σ-TEC association could modulate the nucleotide excision repair processes in vivo. | ||||
(4) | Strong σ-dependent pauses might suppress uncontrolled transcription initiation from the same promoter or inhibit activity of a cryptic promoter coinciding with the pause-inducing signal (). Stable promoter-proximal paused complexes would allow rapid transcription activation under stress conditions (e.g., through activation by Gre factors), similarly to certain eukaryotic promoter-proximal pauses (such as heat-shock promoter in Drosophila melanogaster).Citation39 Promoter-proximal pausing may also decrease stochastic transcription noise and lead to uniform transcription initiation, by introducing an additional rate-limiting step during initial transcription (as proposed in Ref.Citation3) Indeed, it was found that promoter-proximal Pol II pausing in D. melanogaster cells inhibits transcription initiation, which may in turn reduce noise between transcription bursts.Citation40 | ||||
(5) | Finally, we propose that σ-dependent promoter-proximal pauses may modulate transcription regulation by repressor proteins (). This hypothesis is discussed in more detail in the following section. |
Possible function of σ-dependent pausing in antirepression
In both genes for which we observed σ38-dependent pausing, the pause sites overlap with the binding sites of transcription repressors, Cra for adhE (alcohol dehydrogenase) and OmpR for ecnB (entericidin B) ().Citation41,42 To reveal possible effect of the pause-inducing signal on the function of the adhE promoter in vivo, we compared activities of two promoter variants, the wild-type promoter and its derivative with the +11G substitution in the pause-inducing sequence. We analyzed the activity of luciferase operon placed downstream of the adhE promoter or its mutant variant under anaerobic conditions (since adhE is induced during fermentative growth on sugars) ().Citation42,43 The adhE gene was shown previously to be under the control of both σ70 and σ38 subunits.Citation43 Accordingly, we did not reveal dramatic growth-dependent changes in the adhE P1 activity in the luciferase assay (). In contrast to what could be expected from RNAP arrest at the pause site, mutation in the pause-inducing sequence in adhE did not increase but rather decreased promoter activity (∼2-fold at all culture ODs) suggesting that the pause-inducing signal somehow activates transcription. We therefore speculate that temporary RNAP stalling at the pause sites during ongoing transcription may prevent the binding of transcription repressors to promoter-proximal regions, thus allowing continuous transcription initiation.
The overlap of the pause and operator sites is not unique for the adhE and ecnB genes. Previously, the σ70-dependent pause at the lac promoter was hypothesized to prevent lac repressor binding,Citation29 and several σ38-dependent promoters also contain repressor binding sites overlapping with the potential σ-pause signals (galEp1, csgDp, hyaAp, frdAp, puuAp, puuDp, as revealed in the EcoCyc and Regulon databases).
Analogies from other transcription systems
Our unpublished observations suggest that other alternative σ factors in addition to σ38 may also interact with the TEC and induce pausing. Thus, to reveal the prevalence of σ-dependent pausing in vivo, future studies should include genome-wide analyses of pausing under wide spectrum of conditions, corresponding to activation of alternative σ regulons, as well as analysis of mutant strains with reduced σ-dependent pausing. While it still remains to be established whether such pausing has any regulatory functions, the σ-dependent ‘pauseome’ (a network of σ-dependent pauses and regulatory factors) may significantly complicate the transcription paysage in bacteria.
The general transcription initiation factor TFIIB in eukaryotic Pol II (TFB in archaeal RNAP; Rrn7 in Pol I; Brf1 in Pol III) plays a similar role to σ factors in transcription initiation, by recognizing two promoter elements (upstream and downstream TFIIB recognition elements, BRE), facilitating RNA priming and then promoting ITC disassembly by competing with the nascent RNA in the RNA exit channel.Citation44,45 Promoter-proximal pausing is ubiquitous in eukaryotes, where it plays essential functions in transcription regulation, RNA processing, and transcript quality control.Citation39 It would therefore be interesting to establish whether TFIIB and its paralogs could play a role in promoter-proximal pausing in eukaryotic cells, probably by recognizing BRE-like motifs in the transcribed DNA.
Acknowledgements
This work was supported by the Russian Science Foundation [grant number 16-14-10377].
References
- Ellinger T, Behnke D, Bujard, H, Gralla JD. Stalling of Escherichia coli RNA polymerase in the +6 to +12 region in vivo is associated with tight binding to consensus promoter elements. J Mol Biol. 1994;239:455–465. doi:10.1006/jmbi.1994.1388.
- Vo NV, Hsu LM, Kane CM, Chamberlin MJ. In vitro studies of transcript initiation by Escherichia coli RNA polymerase. 3. Influences of individual DNA elements within the promoter recognition region on abortive initiation and promoter escape. Biochemistry. 2003;42:3798–3811. doi:10.1021/bi026962v.
- Duchi D, Bauer DL, Fernandez L, Evans G, Robb N, Hwang LC, Gryte K, Tomescu A, Zawadzki P, Morichaud Z, et al. RNA polymerase pausing during initial transcription. Mol Cell. 2016;63:939–950. doi:10.1016/j.molcel.2016.08.011.
- Basu RS, Warner BA, Molodtsov V, Pupov D, Esyunina D, Fernandez-Tornero C, Kulbachinskiy A, Murakami KS. Structural basis of transcription initiation by bacterial RNA polymerase holoenzyme. J Biol Chem. 2014;289:24549–559. doi:10.1074/jbc.M114.584037.
- Zhang Y, Feng Y, Chatterjee S, Tuske S, Ho MX, Arnold E, Ebright RH. Structural basis of transcription initiation. Science. 2012;338:1076–80. doi:10.1126/science.1227786.
- Murakami KS, Masuda S, Darst SA. Structural basis of transcription initiation: RNA polymerase holoenzyme at 4 A resolution. Science. 2002;296:1280–84. doi:10.1126/science.1069594.
- Kulbachinskiy A, Mustaev A. Region 3.2 of the sigma subunit contributes to the binding of the 3'-initiating nucleotide in the RNA polymerase active center and facilitates promoter clearance during initiation. J. Biol. Chem. 2006;281:18273–276. doi:10.1074/jbc.C600060200.
- Pupov D, Kuzin I, Bass I, Kulbachinskiy A. Distinct functions of the RNA polymerase sigma subunit region 3.2 in RNA priming and promoter escape. Nucleic Acids Res. 2014;42:4494–4504. doi:10.1093/nar/gkt1384.
- Ring BZ, Yarnell WS, Roberts JW. Function of E. coli RNA polymerase sigma factor sigma 70 in promoter-proximal pausing. Cell. 1996;86:485–493. doi:10.1016/S0092-8674(00)80121-X.
- Mukhopadhyay J, Kapanidis AN, Mekler V, Kortkhonjia E, Ebright YW, Ebright RH. Translocation of sigma(70) with RNA polymerase during transcription: Fluorescence resonance energy transfer assay for movement relative to DNA. Cell. 2001;106:453–463. doi:10.1016/S0092-8674(01)00464-0.
- Bar-Nahum G, Nudler E. Isolation and characterization of sigma(70)-retaining transcription elongation complexes from Escherichia coli. Cell. 2001;106:443–451. doi:10.1016/S0092-8674(01)00461-5.
- Harden TT, Wells CD, Friedman LJ, Landick R, Hochschild A, Kondev J, Gelles J. Bacterial RNA polymerase can retain sigma70 throughout transcription. Proc Natl Acad Sci U S A. 2016;113:602–607. doi:10.1073/pnas.1513899113.
- Kapanidis AN, Margeat E, Laurence TA, Doose S, Ho SO, Mukhopadhyay J, Kortkhonjia E, Mekler V, Ebright RH, Weiss S. Retention of transcription initiation factor sigma70 in transcription elongation: Single-molecule analysis. Mol Cell. 2005;20:347–356. doi:10.1016/j.molcel.2005.10.012.
- Zenkin N, Kulbachinskiy A, Yuzenkova Y, Mustaev A, Bass, I, Severinov K, Brodolin K. Region 1.2 of the RNA polymerase sigma subunit controls recognition of the -10 promoter element. EMBO J. 2007;26:955–964. doi:10.1038/sj.emboj.7601555.
- Mooney RA, Davis SE, Peters JM, Rowland JL, Ansari AZ, Landick R. Regulator trafficking on bacterial transcription units in vivo. Mol Cell. 2009;33:97–108. doi:10.1016/j.molcel.2008.12.021.
- Raffaelle M, Kanin EI, Vogt J, Burgess RR, Ansari AZ. Holoenzyme switching and stochastic release of sigma factors from RNA polymerase in vivo. Mol Cell. 2005;20:357–366. doi:10.1016/j.molcel.2005.10.011.
- Reppas NB, Wade JT, Church GM, Struhl K. The transition between transcriptional initiation and elongation in E. coli is highly variable and often rate limiting. Mol Cell. 2006;24:747–757. doi:10.1016/j.molcel.2006.10.030.
- Hatoum A, Roberts J. Prevalence of RNA polymerase stalling at Escherichia coli promoters after open complex formation. Mol Microbiol. 2008;68:17–28. doi:10.1111/j.1365-2958.2008.06138.x.
- Deighan P, Pukhrambam C, Nickels BE, Hochschild A. Initial transcribed region sequences influence the composition and functional properties of the bacterial elongation complex. Genes Dev. 2011;25:77–88. doi:10.1101/gad.1991811.
- Agapov A, Olina A, Esyunina D, Kulbachinskiy A. Gfh factors and NusA cooperate to stimulate transcriptional pausing and termination. FEBS Lett. 2017;591:946–953. doi:10.1002/1873-3468.12609.
- Zhilina E, Miropolskaya N, Bass I, Brodolin K, Kulbachinskiy A. Characteristics of sigma-dependent pausing in RNA polymerases from E. coli and T. aquaticus. Biochemistry (Mosc). 2011;76:1348–58. doi:10.1134/S0006297911100038.
- Perdue SA, Roberts JW. sigma(70)-dependent transcription pausing in escherichia coli. J Mol Biol. 2011;412:782–792. doi:10.1016/j.jmb.2011.02.011.
- Zhilina E, Esyunina D, Brodolin K, Kulbachinskiy A. Structural transitions in the transcription elongation complexes of bacterial RNA polymerase during sigma-dependent pausing. Nucleic Acids Res. 2012;40:3078–91. doi:10.1093/nar/gkr1158.
- Nickels BE, Roberts CW, Sun H, Roberts JW, Hochschild A. The sigma(70) subunit of RNA polymerase is contacted by the (lambda)Q antiterminator during early elongation. Mol Cell. 2002;10:611–622. doi:10.1016/S1097-2765(02)00648-2.
- Devi PG, Campbell EA, Darst SA, Nickels BE. Utilization of variably spaced promoter-like elements by the bacterial RNA polymerase holoenzyme during early elongation. Mol Microbiol. 2010;75, 607–622. doi:10.1111/j.1365-2958.2009.07021.x.
- Strobel EJ, Roberts JW. Two transcription pause elements underlie a sigma70-dependent pause cycle. Proc Natl Acad Sci U S A. 2015;112:E4374–80. doi:10.1073/pnas.1512986112.
- Marr MT, Roberts JW. Function of transcription cleavage factors GreA and GreB at a regulatory pause site. Mol Cell. 2000;6:1275–85. doi:10.1016/S1097-2765(00)00126-X.
- Marr MT, Datwyler SA, Meares CF, Roberts JW. Restructuring of an RNA polymerase holoenzyme elongation complex by lambdoid phage Q proteins. Proc Natl Acad Sci U S A. 2001;98:8972–78. doi:10.1073/pnas.161253298.
- Brodolin K, Zenkin N, Mustaev A, Mamaeva D, Heumann H. The sigma 70 subunit of RNA polymerase induces lacUV5 promoter-proximal pausing of transcription. Nat Struct Mol Biol. 2004;11:551–557. doi:10.1038/nsmb768.
- Goldman SR, Nair NU, Wells CD, Nickels BE, Hochschild A. The primary sigma factor in Escherichia coli can access the transcription elongation complex from solution in vivo. eLife. 2015;4. doi:10.7554/eLife.10514.
- Sevostyanova A, Svetlov V, Vassylyev DG, Artsimovitch I. The elongation factor RfaH and the initiation factor sigma bind to the same site on the transcription elongation complex. Proc Natl Acad Sci U S A. 2008;105:865–870. doi:10.1073/pnas.0708432105.
- Petushkov I, Esyunina D, Kulbachinskiy A. sigma38-dependent promoter-proximal pausing by bacterial RNA polymerase. Nucleic Acids Res. 2017;45:3006–16. doi:10.1093/nar/gkw1213.
- Arndt KM, Chamberlin MJ. Transcription termination in Escherichia coli. Measurement of the rate of enzyme release from Rho-independent terminators. J Mol Biol. 1988;202:271–285. doi:10.1016/0022-2836(88)90457-3.
- Burmann BM, Knauer SH, Sevostyanova A, Schweimer K, Mooney RA, Landick R, Artsimovitch I, Rosch P. An alpha helix to beta barrel domain switch transforms the transcription factor RfaH into a translation factor. Cell. 2012;150:291–303. doi:10.1016/j.cell.2012.05.042.
- Artsimovitch I, Landick R. Pausing by bacterial RNA polymerase is mediated by mechanistically distinct classes of signals. Proc Natl Acad Sci U S A. 2000;97:7090–95. doi:10.1073/pnas.97.13.7090.
- Mondal S, Yakhnin AV, Babitzke P. Modular organization of the NusA- and NusG-stimulated RNA polymerase pause signal that participates in the Bacillus subtilis trp operon attenuation mechanism. J Bacteriol. 2017. doi:10.1128/JB.00223-17.
- Yakhnin AV, Murakami KS, Babitzke P. NusG is a sequence-specific RNA polymerase pause factor that binds to the non-template DNA within the paused transcription bubble. J Biol Chem. 2016;291:5299–5308. doi:10.1074/jbc.M115.704189.
- Haines NM, Kim YI, Smith AJ, Savery NJ. Stalled transcription complexes promote DNA repair at a distance. Proc Natl Acad Sci U S A. 2014;111:4037–42. doi:10.1073/pnas.1322350111.
- Jonkers I, Lis JT. Getting up to speed with transcription elongation by RNA polymerase II. Nat Rev Mol Cell Bio. 2015;16:167–177. doi:10.1038/nrm3953.
- Shao W, Zeitlinger J. Paused RNA polymerase II inhibits new transcriptional initiation. Nat Genet. 2017;49:1045–1051.
- Bishop RE, Leskiw BK, Hodges RS, Kay CM, Weiner JH. The entericidin locus of Escherichia coli and its implications for programmed bacterial cell death. J Mol Biol. 1998;280:583–596. doi:10.1006/jmbi.1998.1894.
- Mikulskis A, Aristarkhov A, Lin EC. Regulation of expression of the ethanol dehydrogenase gene (adhE) in Escherichia coli by catabolite repressor activator protein Cra. J Bacteriol. 1997;179:7129–34. doi:10.1128/jb.179.22.7129-7134.1997.
- Membrillo-Hernandez J, Lin EC. Regulation of expression of the adhE gene, encoding ethanol oxidoreductase in Escherichia coli: Transcription from a downstream promoter and regulation by fnr and RpoS. J Bacteriol. 1999;181:7571–79
- Pal M, Ponticelli AS, Luse DS. The role of the transcription bubble and TFIIB in promoter clearance by RNA polymerase II. Mol Cell. 2005;19:101–110. doi:10.1016/j.molcel.2005.05.024.
- Sainsbury S, Niesser J, Cramer P. Structure and function of the initially transcribing RNA polymerase II-TFIIB complex. Nature. 2013;493:437–440. doi:10.1038/nature11715.