ABSTRACT
Viruses masterfully regulate host gene expression during infection. Many do so, in part, by expressing non-coding RNAs. Recent work has shown that HSUR 2, a viral non-coding RNA expressed by the oncogenic Herpesvirus saimiri, regulates mRNA expression through a novel mechanism. HSUR 2 base pairs with both target mRNAs and host miRNAs in infected cells. This results in HSUR 2-dependent recruitment of host miRNAs and associated Ago proteins to target mRNAs, and the subsequent destabilization of target mRNAs. Using this mechanism, this virus regulates key cellular pathways during viral infection. Here I discuss the evolution of our thinking about HSUR function and explore the implications of recent findings in relation to the current views on the functions of interactions between miRNAs and other classes of non-coding RNAs, the potential advantages of this mechanism of regulation of gene expression, and the evolutionary origin of HSUR 2.
1. Introduction
Upon infection, viruses need to turn the cellular environment into one that allows for viral persistence and replication. This requires controlling and regulating a diverse array of cellular processes. Viruses achieve this in part by manipulating host gene expression. It is therefore not surprising that many viruses include non-coding RNAs (ncRNAs) in their molecular toolbox to help them exert control of host gene expression during infection. ncRNAs can offer viruses many advantages over proteins as regulators of gene expression. For example, ncRNAs can be quite small and take up a small portion of the viral genome. Since ncRNAs are not translated, they can act very quickly once transcribed. Also, ncRNAs do not trigger the adaptive immune response that is elicited by proteins. These characteristics make them particularly useful as components of regulatory pathways that operate over long periods of time, for example during viral latency.
Herpesviruses, a group of large DNA viruses that have the ability to establish life-long latent infections in their hosts, express the most diverse repertoire of viral ncRNAs[Citation1]. In most cases, the functions of ncRNAs expressed by herpesviruses are still unknown. Herpesvirus saimiri (HVS) is an oncogenic γ-herpesvirus that can cause T-cell lymphomas and leukemias in new world monkeys[Citation2]. HVS expresses seven uridine-rich ncRNAs called HSURs (Herpesvirus saimiri U-rich RNAs) in the latent phase of infection. HSURs were first identified in Northern Blot experiments aimed at detecting transcripts expressed from a region of HVS genome that is essential for virus-induced transformation [Citation3]. It was subsequently shown that HSUR genes contain consensus binding sites for Sm proteins and small nuclear RNA (snRNA) promoter and 3′ end processing sequences, and that HSURs can be co-immunoprecipitated with antibodies directed against either Sm proteins [Citation4] or the trimethylguanosine cap structure, thus classifying them as bona fide snRNAs of the Sm-class [Citation5]. Despite their structural similarities, HSURs show no significant sequence similarities with their cellular cousins that participate in splicing and histone pre-mRNA 3′ end processing [Citation6,Citation7]. Research efforts over the years have been mainly focused on HSURs 1 and 2. These two HSURs show the highest degree of conservation among different HVS subgroups and are the only ones present in the closely related Herpesvirus ateles [Citation8] (HVA), suggesting important roles in viral latency for these two ncRNAs. Here I discuss the history of these viral Sm-class RNAs and the implications for a newly discovered function for one of them as a posttranscriptional regulator of gene expression.
2. Some HSURs mimic 3′ UTRs
HSURs 1, 2, and 5 contain sequence elements that are normally found in the 3′ untranslated region (UTR) of messenger RNAs (mRNAs). These three viral ncRNAs display at least one conserved copy of the AUUUA sequence motif close to their 5′ end () that is perfectly conserved among the different strains of HVS. This sequence resembles AU-rich elements [Citation9–Citation11] (AREs) that are typically enriched in mRNAs that encode cytokines, growth factors, and oncogenes. The presence of AREs in the 3′UTR of mRNAs can induce their rapid decay and inhibition of their translation [Citation12]. It was originally hypothesized that HSURs 1, 2, and 5 could function to attenuate the rapid degradation of ARE-containing cellular mRNAs. This hypothesis was supported by the fact that the AREs present in these three HSURs can bind ARE-binding proteins [Citation9,Citation11] (ARE-BPs) and, in the case of HSUR 1, modulate its stability [Citation10]. In addition, this hypothesis was attractive because it also provided a mechanism by which HVS could promote the expression of cytokines and growth factors in the infected cell. However, the association of HSURs with ARE-BPs does not affect the half-life of ARE-containing mRNAs in latently infected cells [Citation9], strongly suggesting that HSURs 1 and 2 do not work as sinks for ARE-BPs.
Figure 1. Nucleotide sequences of HSURs 1, 2, and 5 and miR-142-3p, miR-16, and miR-27a. Binding sites for miR-142-3p (highlighted in blue), miR-16 (highlighted in green), and miR-27a (highlighted in purple) are shown. Seed regions of miRNAs are highlighted in yellow. AU-rich element (ARE)-like sequences are shown in red boxes, whereas the Sm-binding site is shown in a black box.
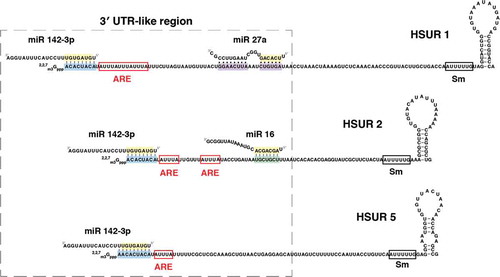
Interestingly, HSURs 1, 2, and 5 also have sequences that function as binding sites for host microRNAs (miRNAs) [Citation13] (). miRNAs constitute a class of small ncRNAs that usually bind to the 3′UTR of mRNAs and recruit Argonaute (Ago) proteins to post-transcriptionally downregulate gene expression. In mammalian cells, Ago proteins interact with the adaptor protein TNRC6. Recruitment of TNRC6 results in repression of mRNA translation and recruitment of deadenylase complexes including the PAN2-PAN3 complex and the CCR4-NOT complex [Citation14]. Shortening of the poly(A) tail leads to degradation of the mRNA via decapping and 5′-to-3′ digestion by XNR1, or 3′-to-5′ digestion by the exosome [Citation15]. mRNA destabilization through deadenylation accounts for most of the miRNA-mediated mRNA repression in post-embryonic mammalian cells [Citation16].
HSUR 1 binds host miR-142-3p and the miR-27 family of miRNAs, whereas HSUR 2 binds both miR-142-3p and the miR-16 family of miRNAs (). HSUR 5 is also predicted to interact with miR-142-3p (); however, this interaction has not been experimentally validated. Examples of interactions between miRNAs and other classes of ncRNAs, including pseudogenes, long non-coding (lnc) and circular (circ)RNAs, have also been documented [Citation17–Citation24]. The fact that ncRNAs could compete with mRNAs for the binding of miRNAs to decrease miRNA-mediated repression of protein-coding transcripts has led to the hypothesis of gene regulation by competing endogenous RNAs (ceRNAs) [Citation25]. Although the physiological relevance of this hypothesis is currently under debate [Citation26–Citation28], there are examples of lncRNAs and circRNAs that can effectively function as miRNA decoys or sponges [Citation18,Citation19,Citation22]. These examples contributed to the generally accepted notion that, when binding to miRNAs, ncRNAs could function as sponges. However, this is not the case for HSURs 1 and 2. The interaction between HSUR 1 and miR-27 involves complementarity with the seed region and also with the 3′ end of the miRNA. This type of complementarity results in the active degradation, rather than sequestration, of the miRNA [Citation13,Citation29,Citation30]. Indeed, several lines of evidence argue against a possible role of HSURs 1 and 2 as inhibitors of miR-142-3p and miR-16 function. The interactions between HSURs 1 and 2 and miR-142-3p and members of the miR-16 family involve only the seed regions of these miRNAs; thus, HSURs 1 and 2 do not affect the abundance of miR-142-3p and miR-16 in virally transformed cells[Citation13]. Immunoprecipitation experiments showed that only a small fraction of HSURs 1 and 2 interact with a small fraction of miR-16 and miR-142-3p[Citation13]. In addition, the fact that HSURs localize mostly to the nucleus whereas miRNAs are mostly cytoplasmic also argues against the hypothesis that HSURs 1 and 2 function as sponges for these two host miRNAs[Citation31]. Finally, HSURs 1 and 2 do not affect the levels of miR-16 and miR-142-3p targets in latently infected cells[Citation13,Citation32].
3. Change of paradigm: HSUR 2 functions as a miRNA adaptor
If HSURs 1 and 2 do not function as sponges for miR-142-3p and miR-16, and HSUR 2 is not polyadenylated and therefore cannot be destabilized by these two miRNAs, why do these two classes of ncRNAs bind each other? This conundrum has been partially solved with our recent work describing a function for HSUR 2 as a miRNA adaptor[Citation32] (). HSUR 2 not only base pairs with miR-142-3p and miR-16, but it can also base pair with mature mRNAs in latently infected cells. HSUR 2 can tether miR-142-3p and miR-16 to target mRNAs to promote their destabilization and lower the abundance of the proteins encoded by these mRNAs. Presumably, HSUR 2 could also affect the translation of target mRNAs; however, this hypothesis remains to be experimentally validated. By using HSUR 2 as a miRNA adaptor, HVS decreases the abundance of approximately 70 transcripts that encode proteins that are important for processes that viruses usually modulate during infection. Examples include mRNAs for genes involved in apoptosis (e.g., FAS), cell cycle progression (RB1), and immune response (JAK1, IFNG). Furthermore, HSUR 2 utilizes this mechanism of mRNA repression to modulate apoptosis [Citation32]. This finding assigns a novel role for an Sm-class RNA in the regulation of gene expression after pre-mRNA processing. In addition, it provides a mechanism by which this herpesvirus regulates host gene expression during viral latency and also an alternative interpretation for the potential functions of the many interactions described so far between miRNAs and other classes of ncRNAs [Citation33].
Figure 2. Model of HSUR 2 function. HSUR 2 base-pairs with a target mRNA and miRNAs miR-142-3p and miR-16 to recruit Ago proteins to the target mRNA, resulting in repression of the target mRNA. HSUR 2 could use sequences that do not involve the binding sites for miR-142-3p (blue box) and miR-16 (green box) to base-pair with the target mRNA, which would result in miR-142-3p- and miR-16-dependent mRNA repression. Alternatively, HSUR 2 could employ sequences that involve the miR-16 binding site to base-pair with the target mRNA, which would result in miR-142-3p- dependent, miR-16-independent, mRNA repression. Red box represents ARE-like sequence.
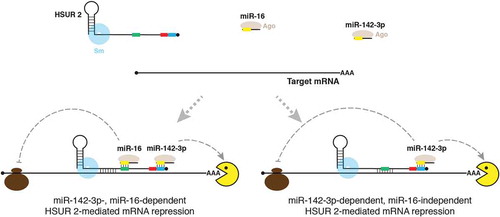
It is currently not clear how HSUR 2 specifically targets this diverse group of mRNAs. Psoralen crosslinking experiments showed that interactions between HSUR 2 and target mRNAs involve direct base-pairing, strongly suggesting that complementarity between sequences present in HSUR 2 and its target mRNAs may dictate target mRNA recognition and specificity. The specific sequences involved in base-pairing between HSUR 2 and target mRNAs remain to be defined. One possibility is that target mRNAs share a common sequence element that is targeted by HSUR 2, for example a binding site for a miRNA that is not expressed in latently infected T cells. In this scenario, HSUR 2 would have a ‘seed sequence’ to interrogate and specifically bind to its targets, a strategy used by several other RNA-based systems [Citation34]. A second, more intriguing possibility is that HSUR 2 utilizes different sequences to base-pair with different target mRNAs. In this scenario, HSUR 2 would function similar to spliceosomal Sm-class RNAs that utilize different sequences to interact with different RNA binding partners during splicing[Citation35]. This mode of binding could provide HSUR 2 with much greater flexibility in target recognition.
Two facts suggest that HSUR 2 base-pairs with sequences present in the 3′UTR of target mRNAs. First, just like miRNAs [Citation36,Citation37], HSUR 2 associates with actively translated mRNAs in polyribosomes, indicating that HSUR 2 remains bound to target mRNAs after the first round of translation. Second, regulatory elements that modulate mRNA stability, like miRNA binding sites and AREs, are usually enriched in mRNA 3′UTRs. Recently developed methods to map RNA-RNA interactions[Citation38–Citation41] might aid in the identification of sequences involved in base-pairing between HSUR 2 and target mRNAs.
Mutagenesis of the conserved AUUUA sequence located next to the miR-142-3p binding site in HSUR 2 () did not have a substantial effect on HSUR 2-mediated mRNA destabilization [Citation32]. This regulatory sequence element could potentially have a role in the regulation of the translation of target mRNAs. HSUR 2 associates with Ago proteins [Citation13], and expression of HSUR 2 results in increased association of HSUR 2 target mRNAs with Ago proteins and their subsequent destabilization [Citation32]. Presumably, HSUR 2-bound miR-142-3p and miR-16 recruit Ago proteins to HSUR 2 target mRNAs. Accordingly, HSUR 2-mediated mRNA destabilization requires binding of HSUR 2 to these two host miRNAs. However, HSUR 2 does not need to interact with both miRNAs to repress all target mRNAs [Citation32]. Binding of HSUR 2 to miR-142-3p only is sufficient for the destabilization of a subset of the target mRNAs tested. It is currently not clear why HSUR 2 requires miR-142-3p and miR-16 activity to effectively destabilize some targets while only requires miR-142-3p activity to repress others. Since most mRNAs are regulated by miRNAs [Citation42], it is a safe to assume that HSUR 2 target mRNAs may normally associate with Ago proteins through direct interactions with miRNAs. HSUR 2 may therefore need to recruit a different number of extra molecules of Ago to each target mRNA to tip the balance over toward destabilization. By base-pairing with a target mRNA, HSUR 2 provides the message with extra miRNA binding sites. For some targets, one extra molecule of Ago protein associated with their 3′ UTR through HSUR 2 might be sufficient to promote destabilization, whereas some HSUR 2 target mRNAs might require more molecules of Ago protein. HSUR 2 could, for example, provide only one extra binding site for miR-142-3p by using the same sequence that is used to bind to miR-16 to base-pair with only one site in the 3′ UTR of the target mRNA. In this scenario, HSUR 2 would not require miR-16 activity to destabilize that target mRNA (). Alternatively, HSUR 2 could bind to more than one site in the 3′ UTR of a target mRNA. HSUR 2 could, in some cases, base-pair with the target mRNA without compromising binding to miR-142-3p and miR-16 (e.g., through sequences located between the miR-16 and Sm protein binding sites, ) and effectively recruit both miRNAs to that site in the 3′ UTR of the target mRNA, while in some others it could base-pair with the target mRNA using the sequence involved in interactions with miR-16 as explained above, effectively tethering only miR-142-3p (). This mechanism provides HSUR 2 with extreme flexibility in the number of Ago protein molecules that it can recruit to target mRNAs to promote their destabilization. Future studies should reveal more mechanistic details about how HSUR 2 recognizes its targets. Furthermore, identification of binding sites in target mRNAs and sequences involved in base-pairing holds promise for understanding the different requirements for miR-142-3p and miR-16 activities in HSUR 2-mediated mRNA repression.
4. Why does HSUR 2 tether specifically miR-142-3p and miR-16 to target mRNAs?
It is unclear why HSUR 2 evolved to specifically bind miR-142-3p and miR-16. Experiments involving the use of mutant versions of HSUR 2 designed to bind mutant miR-142-3p and miR-16 with altered seed sequences showed that this viral Sm-class RNA can employ other miRNAs to repress target mRNAs[Citation32]. Nevertheless, the binding sites for these two host miRNAs are perfectly conserved in HSUR 2 in all the different subgroups of HVS and in the related Herpesvirus ateles[Citation13], strongly suggesting that the identity of the miRNAs employed in HSUR 2-mediated mRNA repression is important. Since HSUR 2 does not compromise the function of these two host miRNAs, one possibility is that they were chosen simply because their expression properties allow for HSUR 2-mediated mRNA repression to occur in the right place and at the right time.
miR-142-3p is abundantly expressed in hematopoietic tissues and is essential for normal hematopoietic lineage development and differentiation[Citation43–Citation45] but it is mostly absent in non-hematopoietic tissues[Citation46]. HVS establishes latency in predominantly CD8 + T-cells that express natural killer (NK) cell markers and show NK cell-like cytotoxicity[Citation47–Citation49], and in which this miRNA is abundantly expressed[Citation50]. Since miR-142-3p activity is required for repression of all HSUR 2 targets[Citation32], it is expected that HSUR 2 will not be able to modulate host gene expression in tissues where this miRNA is not expressed, curtailing the ability of HVS to successfully establish long-term latent infections in non-hematopoietic tissues. This possibility suggests that the choice of miR-142-3p might partially contribute to the tropism of HVS latent infections. Studies in animal models will be required to test this hypothesis.
The miR-16 family modulates cell cycle by targeting genes with important roles in the G1-S transition including those encoding cyclins D1, D2, D3, E1, and CDK6 [Citation51–Citation53]. Accordingly, overexpression of members of this family of miRNAs induce G1 arrest [Citation53]. In addition, one report showed that the abundance of members of the miR-16 family is dynamically regulated by the cell cycle through unknown mechanisms that involve transcriptional regulation and miRNA decay [Citation54]. Interestingly, this study showed that arrest in G0 dramatically increases the levels of this miRNA family. HSUR 2 requires miR-16 activity to repress only a subset of target mRNAs. Presumably, the abundance of miR-16 may determine the ability of HSUR 2 to efficiently repress these targets. By binding to miR-16, HSUR 2 may have acquired the ability to efficiently repress a subset of its targets in a cell cycle-dependent manner. HVS may use this mechanism to regulate the expression of key genes in specific situations, for example when the latently infected cell tries to arrest in G0. Further experimentation will be required to test this hypothesis.
5. Evolutionary origin of HSUR 2
Sm proteins were discovered almost 40 years ago [Citation55] as components of the small nuclear ribonucleoprotein (snRNP) complexes responsible for the excision of introns from pre-mRNAs. We now know that they are part of a family of proteins with members in all kingdoms of life that participate in numerous RNA processing and degradation pathways. In eukaryotes, these include rRNA and tRNA processing [Citation56–Citation58], histone mRNA 3′ processing [Citation59,Citation60], chromosome telomere replication [Citation61], and mRNA decapping and decay [Citation62] (). HSUR 2 provides the first example of an Sm-class RNA that promotes mRNA instability and, presumably, inhibition of translation to modulate protein expression in eukaryotic cells. However, this is not the first example of a small ncRNA that associates with Sm-like proteins to function in this manner. The host factor for bacteriophage Qβ RNA replication (Hfq) [Citation63] protein is the bacterial member of the Sm family of proteins. Similarly to eukaryotic Sm proteins, Hfq binds to U-rich sequences present in regulatory small RNAs (sRNAs) that also base-pair with target mRNAs to regulate their stability and translation [Citation64] (). Also, recent reports suggest that archaeal Sm-like proteins have a role in mRNA stability [Citation65,Citation66]
Figure 3. Functions of Sm and Sm-like proteins in the different life kingdoms. Herpesvirus saimiri’s HSUR 2 associates with Sm proteins in mammalian cells, but shows functional similarity to bacterial Hfq sRNAs.
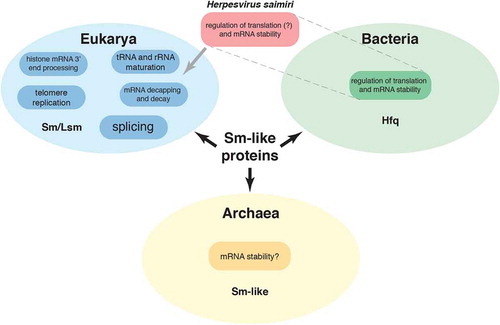
Viruses usually acquire host factors and modify them to exploit their normal cellular functions for their own benefit. HVS contains several open reading frames with striking homology to cellular genes that are thought to have been pirated from the host, including genes related to nucleotide metabolism, cell cycle control, apoptosis, and innate or adaptive immune response [Citation2]. It is therefore possible that HSURs were also acquired from the host by a common ancestor of HVS and HVA. One possibility is that this ancestor acquired genes for spliceosomal Sm-class RNAs from the host and repurposed them throughout evolution to function, similar to bacterial Hfq-associated sRNAs, in modulation of protein output from mRNAs. This hypothesis of convergent evolution with bacterial Hfq sRNAs implies that the initial acquisition of genes for spliceosomal Sm-class RNAs provided an advantage to the virus, but that this advantageous property was no longer required in subsequent evolutionary stages; it also implies that, in contrast to all other pirated host factors present in the HVS genome, these viral Sm-class RNAs would have a function that is completely different from those of their cellular counterparts. A second, more parsimonious hypothesis, is that a common ancestor of HVS and HVA acquired host genes for hitherto unknown Sm-class RNAs that also function by binding to mRNAs to regulate their expression. Consistent with this second hypothesis, low-throughput sequencing of cDNA libraries identified low-abundance Sm-class RNAs of unknown function in Hela cells [Citation67], indicating that original screens for Sm-class RNAs were not sensitive enough to exhaust the search for this class of ncRNAs. The application of high-throughput sequencing technology, which was developed several years after the search for Sm-class RNAs stalled, holds promise for identifying novel members of this class of ncRNAs in host cells that might function similarly to HSUR 2.
6. Perspectives
The ability of HSUR 2 to recruit miRNAs to target mRNAs to repress their expression challenges the notion that ncRNAs that bind miRNAs always function as sponges, and raises several questions. Two outstanding questions are how and where HSUR 2 finds its targets. miRNA-mediated mRNA silencing occurs in the cytoplasm[Citation68]. When new Sm-class RNAs are synthesized, they travel to the cytoplasm where they assemble with Sm proteins and acquire a trimethylguanosine cap structure, to return and concentrate in the nucleus for function[Citation69]. HSUR 2 could potentially identify and bind to target mRNAs during the cytoplasmic stage of its biogenesis; however, the fact that HSUR 2 is mostly nuclear[Citation70] favors a scenario in which it recognizes and binds its target mRNAs in the nucleus, and probably travels with them to the cytoplasm where it binds miRNAs to repress their expression. More research is required to detail this and other aspects of HSUR 2 function. Another fascinating question is whether HSUR 1 and HSUR 5 function similarly to HSUR 2 to bind target mRNAs and recruit miRNAs and ARE-BPs. This possibility would explain why these two viral ncRNAs contain binding sites for miR-142-3p, whose abundance and activity is not affected in latently infected cells. Another important question regards the conservation of this mechanism of gene regulation in other viruses and host cells. HSUR 1 binds and mediates the degradation of miR-27; this RNA-mediated degradation of host miRNAs is a strategy that is also used by other herpesviruses to downregulate the expression of host miRNAs[Citation71]. It would also be interesting to know if other viruses, or their hosts, also use RNA-mediated miRNA recruitment to target mRNAs as a post-transcriptional mechanism for regulation of gene expression. Further investigation is required to provide clear answers to these questions.
Acknowledgments
I would like to thank Dean Tantin and Carlos Gorbea for critical commentaries and helpful discussions.
Disclosure statement
No potential conflict of interest was reported by the author.
Additional information
Funding
References
- Tycowski KT, Guo YE, Lee N, et al. Viral noncoding RNAs: more surprises. Genes Dev. 2015 Mar 15;29(6):567–584. PubMed PMID: 25792595; PubMed Central PMCID: PMCPMC4378190.
- Ensser A, Fleckenstein B. T-cell transformation and oncogenesis by gamma2-herpesviruses. Adv Cancer Res. 2005;93: 91–128. PubMed PMID: 15797445; eng.
- Murthy S, Kamine J, Desrosiers RC. Viral-encoded small RNAs in herpes virus saimiri induced tumors. EMBO J. 1986 Jul;5(7):1625–1632. PubMed PMID: 2427336.
- Lerner EA, Lerner MR, Janeway CA Jr. et al. Monoclonal antibodies to nucleic acid-containing cellular constituents: probes for molecular biology and autoimmune disease. Proc Natl Acad Sci U S A. 1981 May;78(5):2737–2741. PubMed PMID: 6789322; eng.
- Lee SI, Murthy SC, Trimble JJ, et al. Four novel U RNAs are encoded by a herpesvirus. Cell. 1988 Aug 26;54(5):599–607. PubMed PMID: 2842058.
- Marzluff WF, Koreski KP. Birth and death of histone mRNAs. Trends Genet. 2017 Oct;33(10):745–759.. PubMed PMID: 28867047; PubMed Central PMCID: PMCPMC5645032.
- Matera AG, Terns RM, Terns MP. Non-coding RNAs: lessons from the small nuclear and small nucleolar RNAs. Nat Rev Mol Cell Biol. 2007 Mar;8(3):209–220. PubMed PMID: 17318225; eng.
- Albrecht JC. Primary structure of the Herpesvirus ateles genome. J Virol. 2000 Jan;74(2):1033–1037. PubMed PMID: 10623770.
- Cook HL, Mischo HE, Steitz JA. The Herpesvirus saimiri small nuclear RNAs recruit AU-rich element-binding proteins but do not alter host AU-rich element-containing mRNA levels in virally transformed T cells. Mol Cell Biol. 2004 May;24(10):4522–4533. PubMed PMID: 15121869.
- Fan XC, Myer VE, Steitz JA. AU-rich elements target small nuclear RNAs as well as mRNAs for rapid degradation. Genes Dev. 1997 Oct 1;11(19):2557–2568. PubMed PMID: 9334320.
- Myer VE, Lee SI, Steitz JA. Viral small nuclear ribonucleoproteins bind a protein implicated in messenger RNA destabilization. Proc Natl Acad Sci U S A. 1992 Feb 15;89(4):1296–1300. PubMed PMID: 1311093.
- Fukao A, Fujiwara T. The coupled and uncoupled mechanisms by which trans-acting factors regulate mRNA stability and translation. J Biochem. 2017 Apr 1;161(4):309–314. PubMed PMID: 28039391.
- Cazalla D, Yario T, Steitz JA. Down-regulation of a host microRNA by a Herpesvirus saimiri noncoding RNA. Science. 2010 Jun 18;328(5985):1563–1566. PubMed PMID: 20558719; PubMed Central PMCID: PMC3075239.
- Jonas S, Izaurralde E. Towards a molecular understanding of microRNA-mediated gene silencing. Nat Rev Genet. 2015 Jul;16(7):421–433. PubMed PMID: 26077373.
- Shirai YT, Suzuki T, Morita M, et al. Multifunctional roles of the mammalian CCR4-NOT complex in physiological phenomena. Front Genet. 2014;5:286. PubMed PMID: 25191340; PubMed Central PMCID: PMCPMC4139912.
- Bartel DP. Metazoan MicroRNAs. Cell. 2018 Mar 22;173(1):20–51. PubMed PMID: 29570994.
- Bian EB, Ma CC, He XJ, et al. Epigenetic modification of miR-141 regulates SKA2 by an endogenous ‘sponge’ HOTAIR in glioma. Oncotarget. 2016 May 24;7(21):30610–30625. PubMed PMID: 27121316; PubMed Central PMCID: PMCPMC5058705.
- Franco-Zorrilla JM, Valli A, Todesco M, et al. Target mimicry provides a new mechanism for regulation of microRNA activity. Nat Genet. 2007 Aug;39(8):1033–1037. PubMed PMID: 17643101.
- Hansen TB, Jensen TI, Clausen BH, et al. Natural RNA circles function as efficient microRNA sponges. Nature. 2013 Mar 21;495(7441):384–388. PubMed PMID: 23446346.
- Imig J, Brunschweiger A, Brummer A, et al. miR-CLIP capture of a miRNA targetome uncovers a lincRNA H19-miR-106a interaction. Nat Chem Biol. 2015 Feb;11(2):107–114. PubMed PMID: 25531890.
- Liu S, Song L, Zeng S, et al. MALAT1-miR-124-RBG2 axis is involved in growth and invasion of HR-HPV-positive cervical cancer cells. Tumour Biol. 2016 Jan;37(1):633–640. PubMed PMID: 26242259.
- Memczak S, Jens M, Elefsinioti A, et al. Circular RNAs are a large class of animal RNAs with regulatory potency. Nature. 2013 Mar 21;495(7441):333–338. PubMed PMID: 23446348.
- Poliseno L, Salmena L, Zhang J, et al. A coding-independent function of gene and pseudogene mRNAs regulates tumour biology. Nature. 2010 Jun 24;465(7301):1033–1038. PubMed PMID: 20577206; PubMed Central PMCID: PMCPMC3206313.
- Wang J, Liu X, Wu H, et al. CREB up-regulates long non-coding RNA, HULC expression through interaction with microRNA-372 in liver cancer. Nucleic Acids Res. 2010 Sep;38(16):5366–5383. PubMed PMID: 20423907; PubMed Central PMCID: PMCPMC2938198.
- Salmena L, Poliseno L, Tay Y, et al. A ceRNA hypothesis: the Rosetta Stone of a hidden RNA language? Cell. 2011 Aug 5;146(3):353–358. PubMed PMID: 21802130; PubMed Central PMCID: PMCPMC3235919.
- Bosson AD, Zamudio JR, Sharp PA. Endogenous miRNA and target concentrations determine susceptibility to potential ceRNA competition. Mol Cell. 2014 Nov 6;56(3):347–359. PubMed PMID: 25449132; PubMed Central PMCID: PMCPMC5048918.
- Denzler R, Agarwal V, Stefano J, et al. Assessing the ceRNA hypothesis with quantitative measurements of miRNA and target abundance. Mol Cell. 2014 Jun 5;54(5):766–776. PubMed PMID: 24793693; PubMed Central PMCID: PMCPMC4267251.
- Denzler R, McGeary SE, Title AC, et al. Impact of microRNA levels, target-site complementarity, and cooperativity on competing endogenous RNA-regulated gene expression. Mol Cell. 2016 Nov 3;64(3):565–579. PubMed PMID: 27871486; PubMed Central PMCID: PMCPMC5101187.
- Ameres SL, Horwich MD, Hung JH, et al. Target RNA-directed trimming and tailing of small silencing RNAs. Science. 2010 Jun 18; 328(5985):1534–1539. PubMed PMID: 20558712; eng.
- Pawlica P, Moss WN, Steitz JA. Host miRNA degradation by Herpesvirus saimiri small nuclear RNA requires an unstructured interacting region. RNA. 2016 Aug;22(8):1181–1189. PubMed PMID: 27335146; PubMed Central PMCID: PMCPMC4931111.
- Migault M, Donnou-Fournet E, Galibert MD, et al. Definition and identification of small RNA sponges: focus on miRNA sequestration. Methods. 2017 Mar 15;117:35–47. PubMed PMID: 27876678.
- Gorbea C, Mosbruger T, Cazalla D. A viral Sm-class RNA base-pairs with mRNAs and recruits microRNAs to inhibit apoptosis. Nature. 2017 Oct 12;550(7675):275–279. PubMed PMID: 28976967.
- Yamamura S, Imai-Sumida M, Tanaka Y, et al. Interaction and cross-talk between non-coding RNAs. Cell Mol Life Sci. 2018 Feb;75(3):467–484. PubMed PMID: 28840253; PubMed Central PMCID: PMCPMC5765200.
- Gorski SA, Vogel J, Doudna JA. RNA-based recognition and targeting: sowing the seeds of specificity. Nat Rev Mol Cell Biol. 2017 Apr;18(4):215–228.. PubMed PMID: 28196981.
- Wahl MC, Will CL, Luhrmann R. The spliceosome: design principles of a dynamic RNP machine. Cell. 2009 Feb 20;136(4):701–718. PubMed PMID: 19239890.
- Maroney PA, Yu Y, Fisher J, et al. Evidence that microRNAs are associated with translating messenger RNAs in human cells. Nat Struct Mol Biol. 2006 Dec;13(12):1102–1107. PubMed PMID: 17128271.
- Nottrott S, Simard MJ, Richter JD. Human let-7a miRNA blocks protein production on actively translating polyribosomes. Nat Struct Mol Biol. 2006 Dec;13(12):1108–1114. PubMed PMID: 17128272.
- Aw JG, Shen Y, Wilm A, et al. In vivo mapping of eukaryotic RNA interactomes reveals principles of higher-order organization and regulation. Mol Cell. 2016 May 19;62(4):603–617. PubMed PMID: 27184079.
- Lu Z, Zhang QC, Lee B, et al. RNA duplex map in living cells reveals higher-order transcriptome structure. Cell. 2016 May 19;165(5):1267–1279. PubMed PMID: 27180905; PubMed Central PMCID: PMCPMC5029792.
- Nguyen TC, Cao X, Yu P, et al. Mapping RNA-RNA interactome and RNA structure in vivo by MARIO. Nat Commun. 2016 Jun 24;7:12023. PubMed PMID: 27338251; PubMed Central PMCID: PMCPMC4931010.
- Sharma E, Sterne-Weiler T, O’Hanlon D, et al. Global mapping of human RNA-RNA interactions. Mol Cell. 2016 May 19;62(4):618–626. PubMed PMID: 27184080.
- Friedman RC, Farh KK, Burge CB, et al. Most mammalian mRNAs are conserved targets of microRNAs. Genome Res. 2009 Jan;19(1):92–105. PubMed PMID: 18955434; PubMed Central PMCID: PMCPMC2612969.
- Kramer NJ, Wang WL, Reyes EY, et al. Altered lymphopoiesis and immunodeficiency in miR-142 null mice. Blood. 2015 Jun 11;125(24):3720–3730. PubMed PMID: 25931583.
- Lu X, Li X, He Q, et al. miR-142-3p regulates the formation and differentiation of hematopoietic stem cells in vertebrates. Cell Res. 2013 Dec;23(12):1356–1368. PubMed PMID: 24165894; PubMed Central PMCID: PMCPMC3847575.
- Nimmo R, Ciau-Uitz A, Ruiz-Herguido C, et al. MiR-142-3p controls the specification of definitive hemangioblasts during ontogeny. Dev Cell. 2013 Aug 12;26(3):237–249. PubMed PMID: 23911199.
- Chen CZ, Li L, Lodish HF, et al. MicroRNAs modulate hematopoietic lineage differentiation. Science. 2004 Jan 2;303(5654):83–86. PubMed PMID: 14657504; eng.
- Johnson DR, Jondal M. Herpesvirus ateles and herpesvirus saimiri transform marmoset T cells into continuously proliferating cell lines that can mediate natural killer cell-like cytotoxicity. Proc Natl Acad Sci U S A. 1981 Oct;78(10):6391–6395. PubMed PMID: 6273869; PubMed Central PMCID: PMCPMC349045.
- Johnson DR, Jondal M. Herpesvirus-transformed cytotoxic T-cell lines. Nature. 1981 May 7;291(5810):81–83. PubMed PMID: 6262648.
- Kiyotaki M, Desrosiers RC, Letvin NL. Herpesvirus saimiri strain 11 immortalizes a restricted marmoset T8 lymphocyte subpopulation in vitro. J Exp Med. 1986 Sep 1;164(3):926–931. PubMed PMID: 3018122.
- Cazalla D, Xie M, Steitz JA. A primate herpesvirus uses the integrator complex to generate viral microRNAs. Molecular Cell. 2011 Sep 16;43(6):982–992. PubMed PMID: 21925386; PubMed Central PMCID: PMC3176678. eng.
- Bonci D, Coppola V, Musumeci M, et al. The miR-15a-miR-16-1 cluster controls prostate cancer by targeting multiple oncogenic activities. Nat Med. 2008 Nov;14(11):1271–1277. PubMed PMID: 18931683; eng.
- Cimmino A, Calin GA, Fabbri M, et al. miR-15 and miR-16 induce apoptosis by targeting BCL2. Proc Natl Acad Sci U S A. 2005 Sep 27;102(39):13944–13949. PubMed PMID: 16166262; eng.
- Liu Q, Fu H, Sun F, et al. miR-16 family induces cell cycle arrest by regulating multiple cell cycle genes. Nucleic Acids Res. 2008 Sep;36(16):5391–5404. PubMed PMID: 18701644; eng.
- Rissland OS, Hong SJ, Bartel DP. MicroRNA destabilization enables dynamic regulation of the miR-16 family in response to cell-cycle changes. Mol Cell. 2011 Sep 16;43(6):993–1004. PubMed PMID: 21925387; PubMed Central PMCID: PMCPMC3202612.
- Lerner MR, Steitz JA. Antibodies to small nuclear RNAs complexed with proteins are produced by patients with systemic lupus erythematosus. Proc Natl Acad Sci U S A. 1979 Nov;76(11):5495–5499. PubMed PMID: 316537; PubMed Central PMCID: PMCPMC411675.
- Kufel J, Allmang C, Petfalski E, et al. Lsm Proteins are required for normal processing and stability of ribosomal RNAs. J Biol Chem. 2003 Jan 24;278(4):2147–2156. PubMed PMID: 12438310.
- Kufel J, Allmang C, Verdone L, et al. Lsm proteins are required for normal processing of pre-tRNAs and their efficient association with La-homologous protein Lhp1p. Mol Cell Biol. 2002 Jul;22(14):5248–5256. PubMed PMID: 12077351; PubMed Central PMCID: PMCPMC139769.
- Tomasevic N, Peculis BA. Xenopus LSm proteins bind U8 snoRNA via an internal evolutionarily conserved octamer sequence. Mol Cell Biol. 2002 Jun;22(12):4101–4112. PubMed PMID: 12024024; PubMed Central PMCID: PMCPMC133881.
- Galli G, Hofstetter H, Stunnenberg HG, et al. Biochemical complementation with RNA in the Xenopus oocyte: a small RNA is required for the generation of 3ʹ histone mRNA termini. Cell. 1983 Oct;34(3):823–828. PubMed PMID: 6194891.
- Strub K, Galli G, Busslinger M, et al. The cDNA sequences of the sea urchin U7 small nuclear RNA suggest specific contacts between histone mRNA precursor and U7 RNA during RNA processing. EMBO J. 1984 Dec 1;3(12):2801–2807. PubMed PMID: 6084590; PubMed Central PMCID: PMCPMC557769.
- Seto AG, Zaug AJ, Sobel SG, et al. Saccharomyces cerevisiae telomerase is an Sm small nuclear ribonucleoprotein particle. Nature. 1999 Sep 9;401(6749):177–180. PubMed PMID: 10490028.
- Tharun S, He W, Mayes AE, et al. Yeast Sm-like proteins function in mRNA decapping and decay. Nature. 2000 Mar 30;404(6777):515–518. PubMed PMID: 10761922.
- Franze de Fernandez MT, Eoyang L, August JT. Factor fraction required for the synthesis of bacteriophage Qbeta-RNA. Nature. 1968 Aug 10;219(5154):588–590. PubMed PMID: 4874917.
- Vogel J, Luisi BF. Hfq and its constellation of RNA. Nat Rev Microbiol. 2011 Aug 15;9(8):578–589. PubMed PMID: 21760622; PubMed Central PMCID: PMCPMC4615618.
- Martens B, Hou L, Amman F, et al. The SmAP1/2 proteins of the crenarchaeon Sulfolobus solfataricus interact with the exosome and stimulate A-rich tailing of transcripts. Nucleic Acids Res. 2017 Jul 27;45(13):7938–7949. PubMed PMID: 28520934; PubMed Central PMCID: PMCPMC5570065.
- Martens B, Sharma K, Urlaub H, et al. The SmAP2 RNA binding motif in the 3ʹUTR affects mRNA stability in the crenarchaeum Sulfolobus solfataricus. Nucleic Acids Res. 2017 Sep 6;45(15):8957–8967. PubMed PMID: 28911098; PubMed Central PMCID: PMCPMC5587771.
- Yu YT, Tarn WY, Yario TA, et al. More Sm snRNAs from vertebrate cells. Exp Cell Res. 1996 Dec 15; 229(2):276–281. PubMed PMID: 8986610; eng.
- Leung AKL. The whereabouts of microRNA actions: cytoplasm and beyond. Trends Cell Biol. 2015 Oct;25(10):601–610. PubMed PMID: 26410406; PubMed Central PMCID: PMCPMC4610250.
- Patel SB, Bellini M. The assembly of a spliceosomal small nuclear ribonucleoprotein particle. Nucleic Acids Res. 2008 Nov;36(20):6482–6493. PubMed PMID: 18854356; PubMed Central PMCID: PMC2582628. eng.
- Chou CS, Geck P, Medveczky MM, et al. Induction of a herpesvirus saimiri small RNA AU binding factor (AUBF70) activity and lymphokine mRNAs by T cell mitogens. Arch Virol. 1995;140(3):415–435. PubMed PMID: 7733817.
- McCaskill J, Praihirunkit P, Sharp PM, et al. RNA-mediated degradation of microRNAs: A widespread viral strategy? RNA Biol. 2015;12(6):579–585. PubMed PMID: 25849078; PubMed Central PMCID: PMCPMC4615357.