ABSTRACT
Cap-dependent translation initiation begins by assembly of a pre-initiation ribosomal complex that scans the 5ʹ Untranslated Region in order to localise the start codon. During this process, RNA secondary structures are melted by RNA helicases. Guenther et al reported that the yeast helicase Ded1, an orthologue of the mammalian DDX3 helicase, is responsible for this activity. When Ded1 is non-functional, RNA structures in the 5ʹUTR promote translation initiation on Alternative Translation Initiation Sites (ATIS) lead to uORF translation and consequently down-regulation of the main ORF. This mechanism is driven by the sole presence of RNA secondary structures located downstream of ATIS. Translation initiation mediated by RNA structures is found in other messenger RNAs. We propose to name this novel mechanism STructure-Assisted-RNA-Translation or START.
GRAPHICAL ABSTRACT
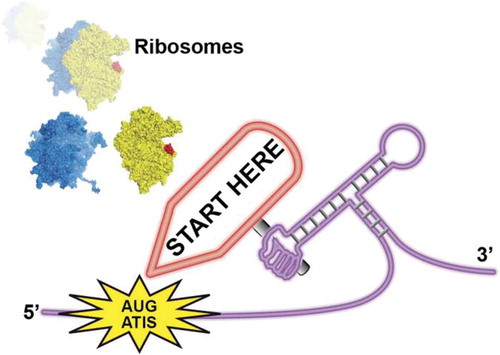
RNAs are versatile molecules that are highly flexible and can therefore adopt many distinct secondary and tertiary structures. In messenger RNAs, structural elements are found in various positions although they are predominantly localised in UTRs. In the 5ʹ UTR they play a critical role in the regulation of translation initiation [Citation1]. Translation of upstream Open Reading Frames (uORF) that starts by AUG or AUG-like codons have a great impact on the translation regulation of downstream coding sequences. Cis-acting such as RNA structural elements and trans-acting factors such as RNA helicases modulate the translation of uORF. Indeed they are several RNA helicases that unwind these RNA elements during the scanning process by the translation initiation 43S particle [Citation2,Citation3]. Among these, the yeast RNA helicase Ded1, an orthologue of the mammalian DDX3, is an essential DEAD-box RNA helicase. During translation initiation, Ded1 plays a critical role for unwinding structural elements in the 5ʹ UTR that might hamper the scanning process [Citation4]. Guenther et al recently reported that Ded1 action in the 5ʹ UTR during translation initiation is directly linked to the translation of uORF [Citation5]. The recognition of Alternative Translation Initiation Site (ATIS) by the ribosome is guided by an RNA structural element that is localised ~ 16–19 nucleotides downstream from ATIS (). The presence of this RNA structure slows down the scanning process and thereby promotes translation initiation on the ATIS. Ded1 inhibits the translation of uORF by unwinding these key structures in the 5ʹUTR. When the downstream structure is unwound, the scanning complex ignores the ATIS in a leaky-scanning mechanism. On the contrary, when Ded1 is inactivated, the presence of RNA structural elements stalls the scanning complex on the ATIS and therefore promotes translation initiation on this codon. In other words, the RNA structure guides the use of ATIS in order to promote translation of uORF. Therefore, Ded1 is required for the regulation of uORF translation.
Figure 1. Examples of mRNAs that are translated by the START mechanism. In yeast, Ded1 sensitive mRNAs contain uORF that begin with an Alternative Initiation Site (ATIS). Secondary RNA structures downstream of ATIS stall the scanning complex and trigger translation initiation on ATIS [Citation5]. In metazoan histone H4 mRNA, a highly stable Three Way Junction (TWJ) positions the AUG start codon in the P site of the ribosome [Citation6,Citation10]. In ALS/FTD patients, C9ORF72 transcripts contained G4C2 repeats that fold into highly stable G-quartet structures. The G-quadruplexes stall the scanning complex and forces translation initiation on the near-cognate CUG codon [Citation7]. In some prokaryotic mRNAs, the ribosome is positioned on the AUG start codon by an upstream interaction between the 16S rRNA with the Shine-Dalgarno (SD) sequence and by a stable hairpin downstream of the AUG. Small regulatory RNAs regulate the folding of the downstream hairpins [Citation8]. During viral translation of EMCV genome, an RNA-protein complex formed by the viral protease 2A bound to a stem-loop structure guides the ribosome for −1 programmed frameshifting [Citation9].
![Figure 1. Examples of mRNAs that are translated by the START mechanism. In yeast, Ded1 sensitive mRNAs contain uORF that begin with an Alternative Initiation Site (ATIS). Secondary RNA structures downstream of ATIS stall the scanning complex and trigger translation initiation on ATIS [Citation5]. In metazoan histone H4 mRNA, a highly stable Three Way Junction (TWJ) positions the AUG start codon in the P site of the ribosome [Citation6,Citation10]. In ALS/FTD patients, C9ORF72 transcripts contained G4C2 repeats that fold into highly stable G-quartet structures. The G-quadruplexes stall the scanning complex and forces translation initiation on the near-cognate CUG codon [Citation7]. In some prokaryotic mRNAs, the ribosome is positioned on the AUG start codon by an upstream interaction between the 16S rRNA with the Shine-Dalgarno (SD) sequence and by a stable hairpin downstream of the AUG. Small regulatory RNAs regulate the folding of the downstream hairpins [Citation8]. During viral translation of EMCV genome, an RNA-protein complex formed by the viral protease 2A bound to a stem-loop structure guides the ribosome for −1 programmed frameshifting [Citation9].](/cms/asset/3f18cc09-1de2-4224-8ff4-b62bd8ad6735/krnb_a_1518855_f0001_oc.jpg)
We propose to name this novel mechanism STructure Assisted RNA Translation or START. The START mechanism is reminiscent of other examples of translation initiation that is guided by RNA structural elements located downstream of the start codon. In the case of Histone H4 translation, the ribosome localises the AUG start with the help of a highly stable Three-Way Junction (TWJ) in order to promote highly efficient translation initiation during the S-phase of the cell cycle [Citation6]. The TWJ is located 19 nucleotides downstream of the AUG codon. In the neurodegenerative diseases Amytrophic Lateral Sclerosis and Fronto Temporal Dementia (ALS/FTD), the hexamer G4C2 is repeated from 30 to several thousands times in C9ORF72 transcripts. The accumulation of these transcripts in the cytoplasm of ALS/FTD patient motor neurons leads to the production of toxic dipeptide repeats (DPR) proteins. DPR are produced by ribosomes that translate these repeated sequences by a so-called Repeated Associated Non-AUG (RAN) mechanism. The initiation starts on a CUG codon located upstream of the G4C2 repeated sequences that folds into a highly stable G-quartet structure. The sole presence of this highly stable structure stalls the scanning complex on the AUG-like codon and promotes aberrant translation initiation [Citation7]. Therefore, this pathological case of RAN translation can be considered as another example of START mechanism. Likewise, translation initiation by START is also found in prokaryotes [Citation8]. The ribosome is positioned on the start codon by the interaction with the Shine Dalgarno (SD) sequence upstream of the AUG and by the presence of stable stem-loops further downstream. Interestingly, the folding of the downstream stem loop is regulated by small RNAs that control the presence or the absence of this stem-loop and therefore regulates the translation in a switch-on/switch-off mechanism. The ribosome can also be controlled during elongation by a downstream structure formed by an RNA-protein complex. This is the case in programmed −1 frameshifting during viral infection by Encephalomyocarditis Virus (EMCV). The viral protease 2A that is produced in the early stage of infection interacts specifically with a stem loop structure. This RNA-protein complex stalls the elongating ribosome and triggers −1 frameshifting [Citation9]. The protein produced after frameshifting is essential for viral propagation.
In order to assess the functional importance of RNA helicase Ded1 in the translation landscape of the cell, Guenther et al. performed ribosome profiling on a mutant Ded-1 thermosensitive yeast strain. Ded-1 inactivation affected translation initiation of most of the cellular mRNAs, however, translation of subsets of mRNAs such as mRNAs coding for proteins involved in gluconeogenesis and histones mRNAs remained unchanged, indicating that these mRNA subsets do not require Ded1 for their translation [Citation5]. By performing iCLIP experiments, Guenther et al showed that Ded1p interacts with the 18S rRNA at three positions: helix h36, Expansion Segment 6 (ES6) and helix h16 (). Interestingly, translation initiation of histone H4 mRNA does not proceed through a scanning mechanism [Citation10]. Instead, the start codon is positioned in the P-site of the ribosome by a direct interaction between an AGG triplet located 17 to 19 nucleotides downstream the AUG and helix h16 of the 18S rRNA [Citation6]. Therefore, helix h16 is not accessible for Ded1 interaction at the mRNA entry channel of the ribosome possibly explaining the lack of scanning during histone H4 translation initiation. It is tempting to speculate that other subclasses of mRNA are blocking the ribosomal helix h16 to prevent Ded1 interaction in order to prevent any RNA structural elements required for translation initiation from unwinding. Consequently, these mRNA subsets are potentially also translated by a START mechanism. Recent technological developments such as Ribosome Profiling [Citation11], Translation Complex Profile sequencing (TCP-seq) [Citation12], Genome-wide probing (DMS-seq) [Citation13], in vivo click selective 2ʹ-hydroxyl acylation and profiling experiment (icSHAPE-seq) [Citation14], Transcripts Isoform in Polysomes sequencing (TrIP-seq) [Citation15] should lead to the discovery of more mRNA families that are translated by a START mechanism.
Figure 2. Ribosomal helix h16 from 18S rRNA interacts with Ded1 helicase. Ded1 is located in the scanning complex by interacting with helix h16. Ded1 unwinds secondary structures in the 5ʹ UTR during 5ʹ-3ʹ scanning until the AUG start codon is found. When Ded1 is non-functional, secondary structures in the 5ʹUTR promote translation initiation on ATIS and lead to uORF translation and down-regulation of the main ORF. During histone H4 mRNA translation, an AGG triplet upstream of the Three-Way Junction (TWJ) interacts specifically with the loop of helix h16 and thereby prevents Ded1 interaction . The ribosome is positioned directly on the AUG start codon with the help of TWJ and without scanning.

Acknowledgments
We are grateful to Prof. Eric Westhof for critical reading of the manuscript and constant support.
Disclosure statement
No potential conflict of interest was reported by the authors.
Additional information
Funding
References
- Leppek K, Das R, Barna M. Functional 5′ UTR mRNA structures in eukaryotic translation regulation and how to find them. Nat Rev Mol Cell Biol. 2018;19:158–174.
- Parsyan A, Svitkin Y, Shahbazian D, et al. mRNA helicases: the tacticians of translational control. Nat Rev Mol Cell Biol. 2011;12:235–245.
- Marintchev A. Roles of helicases in translation initiation: A mechanistic view. Biochim Biophys Acta Gene Regul Mech. 2013;1829:799–809.
- Sharma D, Jankowsky E. The Ded1/DDX3 subfamily of DEAD-box RNA helicases. Crit Rev Biochem Mol Biol. 2014;49:343–360.
- Guenther U-P, Weinberg DE, Zubradt MM, et al. The helicase Ded1p controls use of near-cognate translation initiation codons in 5′ UTRs. Nature. 2018;1:130–134.
- Martin F, Ménétret JF, Simonetti A, et al. Ribosomal 18S rRNA base pairs with mRNA during eukaryotic translation initiation. Nat Commun. 2016;7:12622.
- Tabet R, Schaeffer L, Freyermuth F, et al. CUG initiation and frameshifting enable production of dipeptide repeat proteins from ALS/FTD C9ORF72 transcripts. Nat Commun. 2018;9:152.
- Jagodnik J, Chiaruttini C, Guillier M. Stem-loop structures within mRNA coding sequences activate translation initiation and mediate control by small regulatory RNAs. Mol Cell. 2017;68:158–170.e3.
- Napthine S, Ling R, Finch LK, et al. Protein-directed ribosomal frameshifting temporally regulates gene expression. Nat Commun. 2017;8:15582.
- Martin F, Barends S, Jaeger S, et al. Cap-assisted internal initiation of translation of histone H4. Mol Cell. 2011;41:197–209.
- Ingolia NT, Ghaemmaghami S, Newman JRS, et al. Genome-wide analysis in vivo of translation with nucleotide resolution using ribosome profiling. Science. 2009;324:218–223.
- Archer SK, Shirokikh NE, Beilharz TH, et al. Dynamics of ribosome scanning and recycling revealed by translation complex profiling. Nature. 2016;535:570–574.
- Rouskin S, Zubradt M, Washietl S, et al. Genome-wide probing of RNA structure reveals active unfolding of mRNA structures in vivo. Nature. 2014;505:701–705.
- Spitale RC, Flynn RA, Zhang QC, et al. Structural imprints in vivo decode RNA regulatory mechanisms. Nature. 2015;519:486–490.
- Floor SN, Doudna JA. Tunable protein synthesis by transcript isoforms in human cells. Elife. 2016;5:e10921.