ABSTRACT
It is obvious that the majority of cellular transcripts are long noncoding RNAs (lncRNAs). Although studies suggested that lncRNAs participate in many biological processes through diverse mechanisms, however, little is known about their effects on epidermal mechanoreceptors. Here, we identified one novel Drosophila lncRNA, Scutellar Macrochaetes Regulatory Gene (SMRG), which regulates scutellar macrochaetes that act as mechanoreceptors by antagonizing the proneural gene scute (sc), through the repressor Enhancer-of-split mβ (E(spl)mβ). SMRG deficiency induced supernumerary scutellar macrochaetes and simultaneously a high sc RNA level in the adult thorax. Genetically, sc overexpression enhanced this supernumerary phenotype, while heterozygous sc mutant rescued this phenotype, both of which were mediated by E(spl)mβ. At the molecular level, SMRG recruited E(spl)mβ to the sc promoter region, which in turn suppressed sc expression. Our work presents a novel function of lncRNA and offers insights into the molecular mechanism underlying mechanoreceptor development.
Introduction
The efforts to uncover the complexity of the mammalian transcriptome have revealed a large number of lncRNAs [Citation1,Citation2], which are defined as noncoding RNAs longer than 200 nucleotides, without protein-coding ability; most of them are transcribed by RNA polymeraseII, carrying a 5′-7-methylguanosine cap and a 3′-poly(A) tail [Citation3]. The discovery of extensive transcription of lncRNAs provides an important new perspective on the centrality of RNA in gene regulation [Citation4]. Studies have increasingly indicated that lncRNAs regulate gene expression through diverse mechanisms. They exert their effects by the following trans-regulatory activity. (i) lncRNAs interact with chromatin-modifying complexes to promote either the suppression or the activation of genes [Citation5,Citation6]. (ii) lncRNAs also act as modulators of proteins or enzyme cofactors by interfering in the interaction between proteins and protein-modifying enzymes to affect post-translational modifications and subsequent deregulation of signaling pathways [Citation7] or by modulating enzymatic activity [Citation8]. (iii) lncRNAs interact with DNA/RNA-binding proteins, such as transcription factors, to positively or negatively regulate the expression of their target genes [Citation9,Citation10]. In addition to these actions, lncRNAs also have the following cis regulatory roles. (i) lncRNA-mediated silencing or activation of the adjacent coding gene was shown to be caused by transcriptional interference, where the transcriptional overlap of lncRNA decreased the occupancy of RNAPII on the promoter of the coding gene or through positive regulation by a cis element within the promoter of lncRNA, not by the lncRNA product itself [Citation11]. (ii) lncRNA can also regulate gene expression by forming R-loops or RNA–DNA triplexes [Citation12,Citation13]. Additionally, lncRNA acts as sponge of microRNAs to control the expression of the target genes of microRNAs indirectly [Citation14]. However, despite these findings, the biological significance of most lncRNAs remains to be determined.
Macrochaetes (large bristles) are sensory organs of the Drosophila peripheral nervous system, acting as external mechanoreceptors [Citation15]. The number and location of macrochaetes are strictly constant, and the pattern they form is species-specific in Drosophila [Citation16,Citation17]. Especially, there are 11 pairs of macrochaetes located on the adult notum, among which, two pairs on the scutellum [Citation18,Citation19]. Each macrochaete is named based on its stereotypical position [Citation18]. As the primary external mechanoreceptors, these macrochaetes can sense transient mechanical deflections, such as those produced by contact with foreign objects, so as to alert the fly to the arrival of items such as dirt or a parasite on its body surface [Citation20]. Stimulation of only one or two macrochaetes is sufficient to trigger complex grooming behavior, in which the fly attempts to get rid of such items [Citation21].
Each mechanoreceptor contains four specialized cells: trichogen (shaft), tormogen (socket), neuron, and thecogen (glial cell). All of these cells are produced by a single cell, the so-called sensor organ precursor (SOP) cell [Citation22].The main feature of the SOP cell compared with the encompassing cells lies in the maximal amount of AS-C proteins, produced by the achaete–scute gene complex (AS-C) [Citation15]. AS-C is composed of four proneural genes, achaete (ac), scute (sc), lethal of scute (l’sc), and asense (ase), encoding transcription factors of the Class II bHLH family [Citation23]. These genes play important roles in the commitment of epidermal cells toward a neural fate, promoting the formation of sensory organs in the peripheral nervous system at the adult stage, such as macrochaetes [Citation23]. Among these four proneural genes, sc is the major one positively regulating the development of macrochaetes [Citation24,Citation25].
We show here that one novel lncRNA, SMRG, regulates the scutellar macrochaetes in Drosophila. Moreover, we demonstrate that SMRG exerts its effects by antagonizing the proneural gene sc, which is mediated by a repressor, E(spl)mβ. Furthermore, we reveal that SMRG can promote the recruitment of the repressor E(spl)mβ to the sc promoter region to negatively regulate its expression, which in turn suppresses the appearance of scutellar macrochaetes. Our study offers another functional evidence of lncRNA, namely, its involvement in the regulation of macrochaetes mediated by a specific signaling pathway, and further enriches our understanding of the biological significance of lncRNAs.
Results
SMRG is one novel long noncoding RNA in Drosophila
Initially, using a bioinformatic approach, SMRG was predicted to be one of neural lncRNA candidates in Drosophila (), as described in previous publications [Citation26,Citation27]. Consistent with this, its exact presence in adult fly head was confirmed experimentally by RT-PCR ().The predicted expressed sequence tag (EST) of SMRG is 609 nt in length (). To investigate its possible function, the full sequence of SMRG needed to be determined, for which 5′ and 3′ RACE were performed. Both the 5′ and the 3′ ends of the EST representing SMRG were extended in different lengths: 978 nt at the 5′ end and 292 nt at the 3′ end (). Thus, SMRG transcription produced one 1879-nt, nonspliced, and polyadenylated lncRNA (Supplementary Fig. S1). The independent SMRG transcript was also confirmed by northern blotting, with the size of SMRG being in accordance with the result of 5′ and 3′ RACE (). Open reading frame (ORF) analysis of the SMRG full sequence showed no ORFs longer than 200nt. For all ORFs longer than 50nt in the SMRG sequence, there was no match to any protein sequence in the Swiss-Prot database, so we assumed that SMRG is a ncRNA. To confirm this, a computational method [Citation28] was then used to predict the protein-coding potential of SMRG. The indicative support vector machine (SVM) score of SMRG is −1.30477, classifying it as a noncoding transcript. An in vitro translation assay also proved experimentally that SMRG expression does not produce any protein products, which further confirmed the lack of protein-coding ability of SMRG (). Thus, we demonstrated that SMRG is indeed a lncRNA.
Figure 1. Identification of SMRG as a lncRNA.
(a)Schematic diagram of the SMRG locus and strategy for the generation of SMRG and DTIS11 mutants. The primers used to identify SMRG by RT-PCR and check the identity of the SMRGM mutant by PCR and qPCR, the predicted EST region, the probe used for northern blotting, and the deleted DNA region in SMRGM are shown. The protospacer adjacent motif (PAM) sequences are marked in red. The gRNA binding sequences are underlined. Scissors indicate where the Cas9 cleaves at the DTIS11 and SMRG loci. Red dots represent the 2-bp deletion in the DTIS11 genomic sequence. (b) Exact expression of SMRG in WT CS, flies. M, DNA marker. P, positive control, amplified from genomic DNA. N, negative control, amplified without cDNA template. (c) 5′ and 3′ RACE of SMRG in WT CS fly. (d) Northern blotting of SMRG in WT CS and SMRGM flies. A ~ 1.9-kb SMRG transcript (arrow) was observed in the adult whole fly of the WT CS, but not in the whole fly of the SMRGM flies. M, RNA marker. (e) In vitro translation assay for SMRG. M1, low-range protein ladder. M2, high-range protein ladder. N, negative control, containing no DNA template to show the background. P, positive control, expressing biotinylated luciferase. SMRG, SMRG cloned downstream of the T7 promoter expressed in the in vitro translation system.
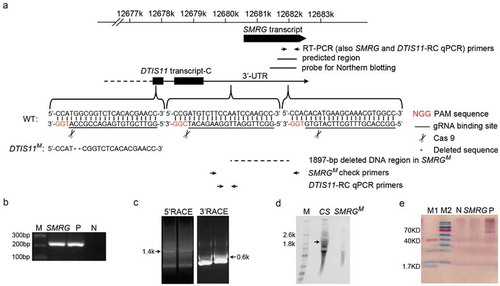
SMRG shows selective distribution in adult fly
Next, the expression level of SMRG in three body parts, head, thorax, and abdomen, of adult wild-type (WT) Canton-S (CS) flies was evaluated by quantitative RT-PCR (qPCR). In the Drosophila genome, the whole SMRG transcript was located in the 3′ UTR (3′ untranslated region) of the protein-coding gene DTIS11 transcript-C (DTIS11-RC), in the same transcription orientation (). To specifically quantify the distribution of SMRG throughout the body, two pairs of primers were utilized. One pair was used to quantify the total expression levels of SMRG and DTIS11-RC. The other pair was used to quantify the expression level of DTIS11-RC alone. Therefore, the specific expression level of SMRG in each body part was calculated by subtracting the expression level of DTIS11-RC from the total expression level including both SMRG and DTIS11-RC. The results indicated that SMRG was distributed mainly in adult head and thorax, and rarely in abdomen ().
Figure 2. Distribution of SMRG in three body parts of WT adult fly.
SMRG showed the highest expression level in adult head and thorax, and very low expression in the abdomen. For DTIS11-RC+SMRG transcript, comparison of head versus thorax, t(20.95) = 1.89, p = 0.0727, For DTIS11-RC transcript, comparison of head versus thorax, t(18.24) = 3.94, p = 0.0009, For SMRG transcript, comparison of head versus thorax, t(30) = −1.205, p = 0.2376, *** p < 0.001; n.s., not significant. Error bars indicate the SEM (Independent-Samples T test).
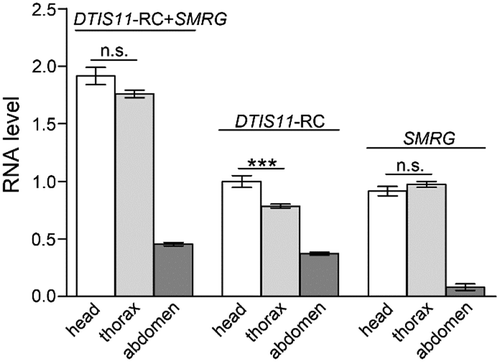
SMRG regulates scutellar macrochaetes in Drosophila
To investigate the function of SMRG, its null mutant was generated by CRISPR/Cas9-mediated gene targeting. First, the conservation of the full SMRG sequence was analyzed across 23 Drosophila species and housefly, honeybee, mosquito, and beetle. The results showed that highly conserved regions were spread across nearly the whole of the SMRG sequence (Supplementary Fig. S2). To construct an SMRG-deficiency line, two guide RNAs (gRNAs) were selected and applied. One gRNA binding site was located 546-bp upstream of the SMRG transcription start site, while the other was located 1351-bp downstream of it. The 1897-bp fragment between these two gRNA binding sites was deleted to generate the SMRG null mutant line SMRGM ().
The identity of SMRGM was first confirmed by PCR using primers flanking the deleted region and sequencing (). Regarding the RNA level of SMRG, as described above, its specific expression level was quantified by using the expression level of DTIS11-RC subtracted from the total expression level including both SMRG and DTIS11-RC; using this approach, no SMRG expression was detected in the whole body of SMRGM by qPCR (). Moreover, no SMRG band was observed in the whole body of SMRGM by northern blotting (). These results indicated that SMRGM is an SMRG null mutant line.
Figure 3. SMRG is required for regulating scutellar macrochaetes.
(a) Identification of SMRGM. PCR was performed using genomic DNA from CS and SMRGM flies with primers flanking the deficiency region in SMRGM. The fragment amplified from SMRGM was 532-bp in length, 1897-bp shorter than the ~2.4-kb product from the WT control. (b) The levels of expression of both SMRG and DTIS11-RC were measured by qPCR, both of which were abolished in SMRGM, p≪0.001 (Mann-Whitney U test). (c) The expression level of DTIS11-RC was measured by qPCR, which was abolished in SMRGM, p≪0.001 (Mann-Whitney U test). (d) Extra scutellar macrochaetes were observed in SMRGM (white arrows). (e) Quantification of the number of scutellar macrochaetes in SMRGM, DTIS11M, and CS flies. Comparison of CS versus SMRGM, t(153.63) = −12.255, p≪0.001, Comparison of CS versus DTIS11M, t(131.50) = −1.934, p = 0.0553. (Independent-Samples T test). (f) The supernumerary scutellar macrochaetes of SMRGM were rescued by the overexpression of SMRG driven by two proneural Gal4s, Comparison of group1 versus 2, t(86.591) = −3.557, p = 0.0006, Comparison of group1 versus 3, t(260.428) = −6.043, p≪0.001, Comparison of group 3 versus 5, t(214) = 6.755, p≪0.001, Comparison of group 4 versus 5, t(196) = 6.325, p≪0.001 (Independent-Samples T test). ***p < 0.001; n.s., not significant. Error bars indicate the SEM. The number of adult flies with each genotype is shown in parentheses.
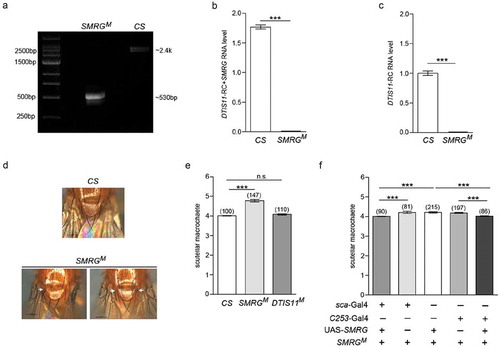
Adult SMRGM flies are viable and fertile. However, the number of scutellar macrochaetes, which function as mechanoreceptors, of these mutant flies was found to be more than that of WT CS flies (). Because DTIS11-RC was also absent in SMRGM (), to exclude the effects of deficiency of DTIS11-RC on the phenotype of supernumerary scutellar macrochaetes, a DTIS11 null mutant line (DTIS11M) was also constructed by CRISPR/Cas9-mediated gene targeting (). This DTIS11M null mutant contained a 2-bp deletion that induced a frameshift in DTIS11 (); however, it showed no difference in the number of scutellar macrochaetes compared to WT CS flies (). To further confirm that the supernumerary scutellar macrochaetes was indeed induced by SMRG deficiency, rescue experiments were carried out by overexpressing SMRG in the SMRGM genetic background. The results indicated that the phenotype of supernumerary scutellar macrochaetes in SMRGM could be rescued completely by the limited expression of SMRG driven by one of two proneural cluster Gal4s, sca-Gal4 and C253-Gal4 (). Taking these findings together, SMRG may function in the proneural cluster and be required for the regulation of scutellar macrochaetes.
SMRG antagonizes the proneural gene sc to regulate scutellar macrochaetes
Since supernumerary scutellar macrochaetes was shown in SMRGM, we investigated whether the expression level of the major proneural gene sc [Citation24,Citation25] was influenced in this mutant line. For this purpose, we measured the RNA level of sc in the thorax of adult SMRGM flies by qPCR; the results indicated that sc RNA was upregulated compared with that in WT CS flies (), which indicated that SMRG may act as a regulator of sc expression.
Figure 4. SMRG regulates scutellar macrochaetes by antagonizing sc.
(a) The sc RNA level in the thorax was higher in SMRGM than in CS flies, t(21.77) = −6.268, p≪0.001 (Independent-Samples T test). (b) The supernumerary scutellar macrochaetes in heterozygous SMRGM were rescued in the double-heterozygous for SMRGM and sc mutants, comparison of group 3 versus 4, t(161.6) = 7.242, p≪0.001, comparison of group 3 versus 5, t(184.53) = 6.502, p≪0.001 (Independent-Samples T test). (c) The phenotype of supernumerary scutellar macrochaetes in homozygous SMRGM was intensified by sc overexpression driven by sca-Gal4, comparison of group 1 versus 3, t(205.42) = −8.603, p≪0.001, comparison of group 2 versus 3, t(183.348) = −16.422, p≪0.001 (Independent-Samples T test). ***p < 0.001. Error bars indicate the SEM. The number of adult flies with each genotype is shown in parentheses.
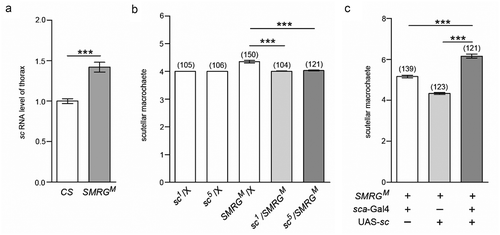
To evaluate whether sc upregulation caused by SMRG deficiency was responsible for the supernumerary scutellar macrochaetes in SMRGM, the genetic interaction between sc and SMRG was examined. Compared with WT CS flies, heterozygous SMRGM mutants were haplo-insufficient, exhibiting supernumerary scutellar macrochaetes, while heterozygous sc mutants, sc1 [Citation29,Citation30] and sc5 [Citation30,Citation31], showed no such phenotype. Importantly, when heterozygous SMRGM was combined with heterozygous sc1 or sc5, the supernumerary scutellar macrochaetes were suppressed (). In addition, sc overexpression in the proneural cluster driven by sca-Gal4 enhanced the supernumerary phenotype of scutellar macrochaetes caused by homozygous SMRGM (). These genetic interaction results suggested that SMRG participates in regulating the scutellar macrochaetes by antagonizing the proneural gene sc.
The antagonistic effect of SMRG on sc is mediated by E(spl)mβ
Among the seven E(spl) genes, E(spl)mβ is expressed more broadly in the notum region of the imaginal disc [Citation32], where the notal macrochaetes arise from the proneural cluster. These prefigure the sites of each of the future macrochaetes, including the scutellar ones [Citation33,Citation34]. Moreover, E(spl)mβ has been reported to act as a repressor, interacting and inhibiting the activity of the transcriptional activation domain (TAD) of the proneural gene sc [Citation35]. We thus examined whether E(spl)mβ is involved in the antagonistic effect of SMRG on sc, which presented with a phenotype of supernumerary scutellar macrochaetes caused by homozygous SMRGM. To investigate this, E(spl)mβ null mutant was generated using the CRISPR/Cas9 system. One gRNA-binding site was chosen within the 5′ half of the coding region of E(spl)mβ (). The E(spl)mβ12−11 null mutant contained a 7-bp deletion that induced a frameshift in E(spl)mβ (). Notably, adult homozygous E(spl)mβ12−11 mutant flies also showed supernumerary scutellar macrochaetes compared with WTCS flies (). Next, we determined whether the expression level of the proneural gene, sc, was also influenced in the mutant line. The sc RNA level in the thorax of adult homozygous E(spl)mβ12−11 flies was proved to be upregulated compared with that of WTCS flies (). To further confirm that the sc upregulation caused by E(spl)mβ deficiency was involved in the supernumerary phenotype in E(spl)mβ12−11, genetic interaction between E(spl)mβ and sc was examined. Compared with WTCS flies, heterozygous E(spl)mβ12−11 mutants were also haplo-insufficient, displaying extra scutellar macrochaetes. However, when heterozygous E(spl)mβ12−11 was combined with heterozygous sc1 or sc5, the extra scutellar macrochaetes phenotype was suppressed (). Furthermore, the supernumerary scutellar macrochaetes caused by homozygous E(spl)mβ12−11 were exacerbated by sc overexpression in the proneural cluster driven by sca-Gal4 ().
Figure 5. E(spl)mβ also regulates scutellar macrochaetes by antagonizing sc.
(a) Schematic diagram of the E(spl)mβ locus and strategy of the generation of its mutant. The PAM sequence is marked in red. The gRNA binding sequence is underlined. Scissors indicate where Cas9 cleaves at the E(spl)mβ locus. Red dots represent the 7-bp deletion in the E(spl)mβ genomic sequence. (b) Quantification of the number of scutellar macrochaetes in E(spl)mβ12−11 and CS flies, t(172.112) = −9.972, p≪0.001 (Independent-Samples T test). (c) The sc RNA level in the thorax of E(spl)mβ12−11 was higher than in CS flies, t(19.622) = −12.748, p≪0.001 (Independent-Samples T test). (d) The supernumerary scutellar macrochaetes in heterozygous E(spl)mβ12−11 were restored in the double-heterozygous for E(spl)mβ12−11 and sc mutants, comparison of group 3 versus 4, p≪0.001 (Mann-Whitney U test), comparison of group 3 versus 5, t(151.374) = 7.176, p≪0.001 (Independent-Samples T test). (e) The phenotype of supernumerary scutellar macrochaetes in homozygous E(spl)mβ12−11 was intensified by sc overexpression driven by sca-Gal4, comparison of group 1 versus 3, t(228.729) = −15.332, p≪0.001, comparison of group 2 versus 3, t(213.259) = −16.626, p≪0.001, (Independent-Samples T test). ***p < 0.001. Error bars indicate the SEM. The number of adult flies with each genotype is shown in parentheses.
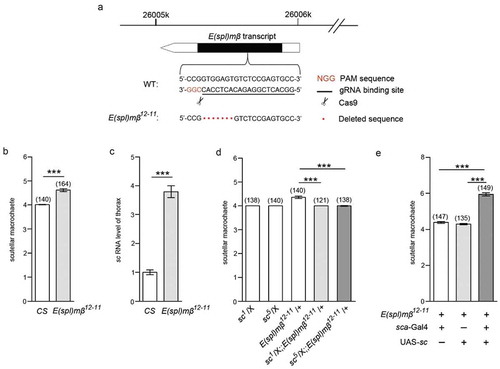
Because the genetic interaction between E(spl)mβ and sc was similar to that between SMRG and sc, we supposed that SMRG exerted its effect on the scutellar macrochaetes through E(spl)mβ-sc signaling.
SMRG recruits e(spl)mβ to the sc promoter to negatively regulate sc expression
Since sc RNA level was upregulated in the thorax of the SMRG deficiency line, luciferase assays were performed to determine whether SMRG transcripts affected the sc promoter region to regulate sc transcription. A segment corresponding to about 1.7 kb upstream (1.7 kb-up) of the sc transcription start site was chosen and confirmed to include the sc promoter in the luciferase reporter system ().
Figure 6. SMRG negatively regulates sc promoter by promoting the occupancy of E(spl)mβ repressor.
(a) Schematic diagram of the sc gene showing the transcription start site (arrow). The detected occupancy sites CHIP1-7 within the potential sc promoter region are shown. (b, c) Introduction of the sc 1.7kb-up fragment into the pGL3-Basic luciferase reporter plasmid induced luciferase expression, suggesting that it contains the sc promoter region. Additional expression of the SMRG, comparison of group 1 versus 2, t(2.16) = −63.542, p = 0.000142, comparison of group 2 versus 3, t(4) = 34.395, p≪0.001 (b) or E(spl)mβ, comparison of group 1 versus 2, t(4) = −13.418, p = 0.000178, comparison of group 2 versus 3, t(2.01) = 11.492, p = 0.00735 (c) transcript in pcDNA3.1 inhibited the sc 1.7 kb-up-induced luciferase expression (Independent-Samples T test). (D) In vivo occupancy of E(spl)mβ on the sc promoter (CHIP7) was decreased in SMRGM flies, indicating that such occupancy is positively regulated by the SMRG transcript, t(21.389) = 15.844, p≪0.001 (Independent-Samples T test). **p < 0.01,***p < 0.001. Error bars indicate the SEM.
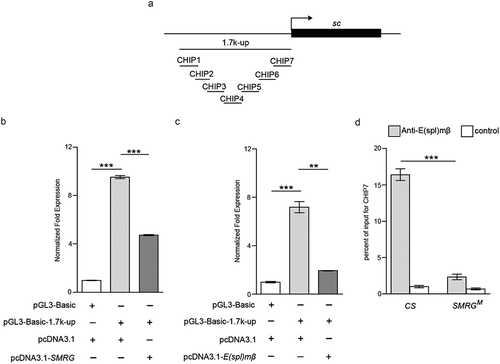
Then, our results showed that the SMRG transcript clearly inhibited the sc 1.7 kb-up promoter-induced luciferase expression (). We also determined the effect of E(spl)mβ on the sc promoter. Consistent with that of SMRG, E(spl)mβ also had an inhibitory effect on the luciferase expression induced by the sc 1.7 kb-up promoter ().
These results indicated that the sc 1.7 kb-up promoter region was the common targeted segment of SMRG and E(spl)mβ. Moreover, both SMRG and E(spl)mβ had inhibitory effects on the sc 1.7 kb-up promoter, leading to negative regulation of sc expression.
To discern how SMRG inhibits the sc promoter, and because E(spl)mβ is a repressive transcription factor [Citation35], we investigated whether SMRG influenced the association between E(spl)mβ and the sc promoter. Seven truncated segments (CHIP1 to CHIP7) within the sc 1.7 kb-up promoter were selected to examine the interaction affected by SMRG (). We observed that the occupancy of E(spl)mβ on the sc promoter in CHIP7 was decreased in the SMRG null mutant, as determined by CHIP assays (). Hence, the SMRG transcript negatively regulates sc expression by promoting the association between E(spl)mβ and the sc promoter region.
Next, we investigated whether the SMRG transcript exerts its effects by interacting with E(spl)mβ. To clarify this, we carried out RIP assays to identify the candidate SMRG fragments that interacted with E(spl)mβ. Three consecutive truncated SMRG fragments (RIP4 to RIP6) jointly spanning 778nt within SMRG were amplified from the immunoprecipitate of E(spl)mβ extracted from WTCS tissues (.
Figure 7. SMRG regions associated with E(spl)mβ were identified.
(a) Schematic representation of the SMRG gene showing the transcription start site (arrow). All of the detected truncated coprecipitated SMRG fragments are shown. (b-d), In vivo identification of the SMRG transcript fragments (RIP4-RIP6) associated with E(spl)mβ. M, DNA marker. +RT, reverse transcription. -RT, control for DNA contamination. (e-g), Quantification of the association of RIP4-RIP6 with E(spl)mβ, t(24.602) = −7.714, p≪0.001 (e), t(26) = −7.078, p≪0.001 (f), t(21.067) = −8.391, p≪0.001(G) (Independent- Samples T test). ***p < 0.001. Error bars indicate the SEM.
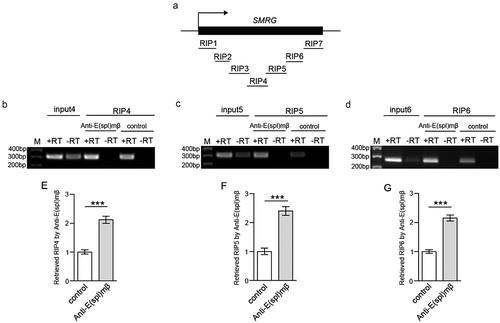
Taken together, these results indicated that the SMRG transcript negatively regulates sc expression by interacting with the repressive transcription factor E(spl)mβ, and recruiting it to the sc promoter region.
Discussion
In this study, we identified one novel lncRNA, SMRG, in Drosophila. SMRG has particular biological significance in that it modulates scutellar macrochaetes, which is attributed to its antagonistic effect on the proneural gene sc. This antagonism is achieved by recruiting the repressor E(spl)mβ to the promoter region of sc.
The finding that conserved regions were spread across almost the whole of the SMRG sequence strongly suggests that it has a biological function. Our understanding of a gene’s function can be boosted by observing the phenotype induced by its absence [Citation36]. Thus, SMRG null mutant (SMRGM) was established using the CRISPR/Cas9 system, which exhibited supernumerary scutellar macrochaetes. Because SMRG completely localizes within the 3′UTR of the adjacent coding gene DTIS11-RC, with the same transcriptional orientation, the deleted DNA fragment in SMRGM also causes a deficiency of DTIS11-RC. Based on the results of the SMRG rescue experiment driven by two proneural cluster Gal4s, combined with the result of a lack of supernumerary scutellar macrochaetes in the DTIS11 null mutant, we conclude that the phenotype of supernumerary scutellar macrochaetes is specifically caused by SMRG deficiency.
Among the four proneural genes, sc plays the most important role in the formation of macrochaetes in the peripheral nervous system at the adult stage [Citation24,Citation25]. We consider that sc may be the target gene of SMRG. In this study, we found that the RNA level of sc was upregulated and proved that this was responsible for the phenotype of supernumerary scutellar macrochaetes in the SMRG mutant.
Seven enhancer-of-split (E(spl)) genes, namely, mδ, mγ, mβ, m3, m5, m7, and m8, in Drosophila encode Class VI bHLH transcription factors [Citation37,Citation38]. Among them, E(spl)mβ is expressed more broadly in the notum region of the imaginal disc [Citation32], where the notal macrochaetes arise from the proneural cluster [Citation33,Citation34]. Our study demonstrates that E(spl)mβ deficiency could indeed result in the phenotype of supernumerary scutellar macrochaetes; moreover, our findings showed that the RNA level of sc is also upregulated and contributes to the supernumerary phenotype in the E(spl)mβ mutant.
Since there are similarities in the supernumerary phenotype and sc upregulation between SMRG and E(spl)mβ null mutant, we attempted to discern the relationship between them and the underlying molecular mechanism. Owing to the upregulation of the sc RNA level being induced by SMRG or E(spl)mβ deficiency, we determined whether SMRG or E(spl)mβ has a certain effect on sc’s promoter region. Our results showed that both of them negatively regulated sc promoter-induced luciferase expression, which is consistent with the changes of sc RNA.
The previous study demonstrated that lncRNA regulates the recruitment of proteins to the promoter region of target gene [Citation26]. E(spl)mβ has an inhibitory effect on the sc’s promoter, combined with the upregulation of sc RNA induced by SMRG deficit, so we determined whether the occupancy of E(spl)mβ on the sc’s promoter region is influenced by SMRG deficiency. As expected, the recruitment of E(spl)mβ onto the sc’s promoter region decreased in the absence of SMRG. Based on this, we further confirmed the existence of interplay between SMRG and E(spl)mβ.
In conclusion, our study indicates that the lncRNA SMRG recruits E(spl)mβ repressor to the proneural gene sc’s promoter region, which in turn suppresses sc expression and thereby inhibits the development of Drosophila mechanoreceptors, scutellar macrochaetes (). In contrast, SMRG deficiency decreases the occupancy of E(spl)mβ repressor on the sc’s promoter region, which in turn derepresses sc expression and promotes the development of scutellar macrochaetes (). This better understanding of the mechanisms underlying the functions of lncRNA extends our awareness of their biological significance and should also benefit fundamental research on lncRNAs.
Figure 8. Model for SMRG function in scutellar macrochaetes.
(a) SMRG recruits the repressor of E(spl)mβ to the sc promoter region, thereby negatively regulating sc expression and subsequent emergence of scutellar macrochaetes. (b) While SMRG deficiency causes low occupancy of E(spl)mβ on the sc promoter region, which in turn derepresses sc expression and promotes responsive supernumerary scutellar macrochaetes.
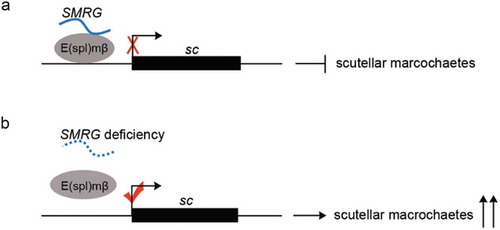
Materials and methods
Fly stocks and genetics
All flies were kept at 25℃ on standard corn meal/molasses medium in a 12 h light/12 h dark cycle at 60% humidity [Citation39]. Wild-type (WT) Canton-S (CS) was used as a control strain. sc-Gal4 (6479), C253-Gal4 (6980), UAS-sc (26,687), sc1 (176), sc5 (178) were obtained from the Bloomington Stock Center (Indiana University, Bloomington, Indiana). {nos-Cas9}attP2 and y [Citation1] sc [Citation1] v [Citation1] P{y[+t7.7] = nos-phiC31\int.NLS}X; P{y[+t7.7] = CaryP}attP2 were obtained from Gene Regulatory Laboratory (School of Medicine, Tsinghua University, Beijing). SMRG null mutant (SMRGM), DTIS11 mutant (DTIS11M), E(spl)mβ mutant (E(spl)mβ12−11) were generated by CRISPR/Cas9-mediated gene targeting. gRNA design conformed with the target sequence principle:5ʹ-GG-N17-19-NGG-3ʹ, in which NGG represents the protospacer adjacent motif (PAM) [Citation40]. The binding sequences of gRNAs used in this study were as follows:
gRNAs-SMRGM: 5ʹ-GGCTTGGATTGGAAGACATCGG-3ʹ and 5ʹ-GGCCACGTTTGCTTCATGTGTGG-3ʹ, gRNA-DTIS11M:5ʹ-GGTTCGTGTGAGACCGCCATGG-3ʹ, gRNA-E(spl)mβ12−11:5ʹ-GGCACTCGGAGACACTCCACCGG-3ʹ. Cas9 mRNAs and gRNAs were transcribed in vitro. The prepared gRNAs or Cas9 mRNAs and gRNAs mixtures were injected into {nos-Cas9}attP2 or CS embryos according to a previously described protocol [Citation41,Citation42]. The PCR primers used for the confirmation of mutant lines were: SMRGM-forward:5ʹ-GCTAGCATGAATGCTATAGCT-3ʹ, SMRGM-reverse:5ʹ-GATCGTTCAAGAGTACCAACC-3ʹ, DTIS11M-forward:5ʹ-GCATTCGCAGATGAACGATCAC-3ʹ, DTIS11M-reverse:5ʹ-CGTTCTTGTGAAGGGTTTAGGC-3ʹ, E(spl)mβ12−11-forward:5ʹ-GTTGCGTGCACACAGAGTCT-3ʹ, E(spl)mβ12−11-reverse:5ʹ- AGCTTG AAGAGGCACTCCAAT-3ʹ.
The USA-SMRG transgenic line was constructed with a full-length cDNA of the SMRG transcript sequence according to the protocol described previously [Citation43]. The full-length sequence of SMRG were amplified from WT cDNA using the follow primers: SMRG-forward: 5ʹ-GAGAAGAATTC CATGGGACACACTCAGACAC-3ʹ, SMRG-reverse:5ʹ-GGCGGTCTAGA TTTGTTTTTATTTTTCATTT-3ʹ. The construct was verified by sequencing.
5ʹ and 3ʹ RACE
5ʹ and 3ʹ RACE were performed with SMARTerTM RACE cDNA amplification kit (Clontech) according to the manufacturer’s instructions.
The primers used in 5ʹ RACE for SMRG were:
5ʹRACE outer primer: 5ʹ-AGTAGGGTTTCATTCCGATAAGGCCACG −3ʹ,
5ʹRACE inner primer: 5ʹ-CCACGTTTGCTTCATGTGTGGGGCACGG −3ʹ.
The primers used in 3ʹ RACE for SMRG were:
3ʹRACE outer primer: 5ʹ- TGTTTTCGTACTCCAATACG −3ʹ,
3ʹRACE inner primer: 5ʹ- CCTAACTTAGACCTGAGCTT −3ʹ.
The PCR products were purified and cloned into a pGEM-T vector (Promega) for sequencing.
Northern blotting
The specific fragment used for probe synthesis was amplified by RT-PCR from WTCS fly cDNA. The primers used in the reaction were:
probe-forward: 5ʹ- ATTACGACCAACAGCAGC −3ʹ,
probe-reverse: 5ʹ-CGATCATACGATTTAGGTGACACTATAGAAATATTATGGTATATGG −3ʹ.
After sequencing, the specific SMRG RNA probe was then synthesized and labeled by in vitro transcription using the fragment carrying SP6 promoter sequence as template with SP6 RNA polymerase (Roche) and DIG RNA Labeling Kit (Roche). The length of probe is 846 bases. Northern blotting was performed using polyA-RNA isolated from total RNA of the adult CS flies with Poly(A) Purist-MAG Magnetic mRNA Purification Kit (Ambion), and the blots were hybridized in DIG Easy Hyb (Roche) at 57°C overnight, followed by washing and incubation with DIG Wash and Block Buffer Set kit (Roche) and CDP-Star kit (Roche) respectively according to the manufacturer’s instructions. Chemiluminescent signals were visualized using X-ray film.
In vitro translation
The coding potential of SMRG was also examined experimentally. SMRG-cloned downstream of T7 promoter was expressed in the in vitro translation TNT® Coupled Reticulocyte Lysate Systems (Promega). Meanwhile, the construction expressing biotinylated luciferase was used as positive control; the reaction lacking DNA template as negative control. The three samples corresponding to SMRG, negative and positive control were separated by sodium dodecyl sulfate polyacrylamide gel electrophoresis (SDS-PAGE; 8% polyacrylamide) and electrophoretically transferred to polyvinylidene difluoride membranes. Membranes were blocked in phosphate-buffered saline containing 5% nonfat milk powder for 1 h at room temperature, and then incubated with the Streptavidin-Alkaline Phosphatase Conjugate (Promega) (1:1600) overnight at 4°C. Membranes were subjected to three PBST washes (10 min each time) and finally visualized by Western Blue® Stabilized Substrate for Alkaline Phosphatase (Promega).
RT-PCR and quantitative RT-PCR
RT-PCR was carried out to confirm that SMRG was transcribed exactly in the head of CS flies. The primers used in the reaction were:
SMRG RT-PCR-forward:5ʹ-CGCGTTCTTCCCTTTGTACAA-3ʹ,
SMRG RT-PCR-reverse:5ʹ-CCTCTGTTTATCGATCGTTC-3ʹ.
Quantitative RT-PCR (qPCR) was carried out to quantify SMRG and DTIS11transcript-C (DTIS11-RC) RNA levels in head, thorax and abdomen parts of adult CS flies. Besides, qPCR was also performed to quantify the RNA levels of SMRG and DTIS11-RC in the whole body of adult CS and SMRGM flies and also used to quantify the sc RNA level in the throax part of adult CS, SMRGM and E(spl)mβ12−11 flies following a protocol described previously [Citation26,Citation27].
The primers used for qPCR were:
SMRG and DTIS11-RC qPCR-forward:5ʹ-CGCGTTCTTCCCTTTGTACAA-3ʹ.
SMRG and DTIS11-RC qPCR-reverse:5ʹ-CCTCTGTTTATCGATCGTTC-3ʹ.
DTIS11-RC qPCR-forward:5ʹ-CACACTTTGGATAGGTCTCA −3ʹ.
DTIS11-RC qPCR-reverse:5ʹ-CTGTCCGTCTATCGTTCTCA −3ʹ.
sc qPCR-forward: 5ʹ- AACTTGGTGGGCACATCCGTAC −3ʹ.
sc qPCR-reverse: 5ʹ- TAATTCGTTGTCCAGATGATCC −3ʹ.
actin qPCR-forward: 5ʹ- CAGGCGGTGCTTTCTCTCTA −3ʹ.
actin qPCR-reverse: 5ʹ- AGCTGTAACCGCGCTCAGTA −3ʹ.
Luciferase assay
Luciferase assay was performed following the protocol published before [Citation26]. The potential sc promoter region from the sc transcription start site, 1.7 kb-up (−1673/-1), was amplified from CS genomic DNA and sub-cloned into empty pGL3-Basic luciferase reporter plasmid to generate recombinant reporter plasmid. The primers used to amplify the fragment were:
1.7 kb-up-forward: 5ʹ-ACGCGTCCGGAATTGGACCTATGA −3ʹ, 1.7 kb-up-reverse:5ʹ- AGATCTCGTCCTAAACCCTCGCGTTAT −3ʹ. The full SMRG or E(spl)mβ cDNA sequence was amplified from adult WT flies by RT-PCR and sub-cloned into the empty expression plasmid pcDNA3.1 to generate the expression plasmid for SMRG or E(spl)mβ respectively.
The primers used to amplify the two cDNA fragments were:
SMRG-forward: 5ʹ-GAGAAGAATTC CATGGGACACACTCAGACAC-3ʹ, SMRG-reverse:5ʹ-GGCGGTCTAGA TTTGTTTTTATTTTTCATTT-3ʹ.
E(spl)mβ-forward:5ʹ-GAATTCATGGTTCTGGAAATGGAGAT-3ʹ, E(spl)mβ-reverse:5ʹ-CTCGAGTCACCAGGGACGCCACAT −3ʹ.
Generation of anti-E(spl)mβ antibody
Polyclonal anti-E(spl)mβ antibody was generated using two synthetic coupled peptides, CLQAPVEDQAMVTPPPSE and CPAPSEASSTSGPMWRPW, as antigen. The peptides were used to immunize a rabbit for anti-E(spl)mβ serum production. The preimmune serum was used as control (B&M Biotech).
Chromatin immunoprecipitation
The chromatin immunoprecipitation (CHIP) assay was performed using EZ Chip Kit (Millipore). 24 adult flies from CS and SMRGM line were treated respectively with formaldehyde to crosslink the proteins to the genomic DNA. The tissue was then homogenized in lysis buffer to obtain a cell lysate that was subsequently sonicated to shear the genomic DNA into lengths of between 200 and 1000 bp. The sheared supernatant was immunoprecipitated using anti-E(spl)mβ polyclonal antibody; The preimmune serum was used as control. The specific protein-DNA crosslinks were reversed at 65℃, and the DNA was purified to remove chromatin proteins and quantified by qPCR. All experiments were performed in quadruplicate.
The primers used for qPCR were:
CHIP1
5ʹ- GGTGACCGGAATTGGACCTATGAT −3ʹ
5ʹ-GAGGGATCGGTAGTTGGTTTCCAT −3ʹ
CHIP2
5ʹ-TCACCGGCATTTCTCCCAACCATT −3ʹ
5ʹ-AAACGGCGCTTCTCTGTGTTGTTC-3ʹ
CHIP3
5ʹ-ATGGAAACCAACTACCGATCCCTC −3ʹ
5ʹ-ATTGTCCTCAACTCGTTACCCTCG −3ʹ
CHIP4
5ʹ-GAACAACACAGAGAAGCGCCGTTT −3ʹ
5ʹ-TAACTTGCCATTGGCCACTGTGTC −3ʹ
CHIP5
5ʹ- TTTACAGTCGCCGTTTAGCACGAC −3ʹ
5ʹ- GGATGTGACACAAGTTCTAGAAGG −3ʹ
CHIP6
5ʹ- CCGCAGTTTTCACTGCAAACTTCC −3ʹ
5ʹ-ATCAAGGTATCTGAGTGAGGGCTC −3ʹ
CHIP7
5ʹ-AGAAATCAAATTGCTGGCGTGCCG −3ʹ
5ʹ- CGTCCTAAACCCTCGCGTTATGTA −3ʹ
RNA immunoprecipitation
The RNA immunoprecipitation (RIP) assay was carried out using EZ-Magna kit (Millipore). 100 adult CS flies were collected and washed three times with ice-cold PBS, then homogenized in ice-cold PBS until a single-cell suspension was obtained. The cells were collected by centrifugation and resuspended in RIP lysis buffer, incubated on ice for 5 min and stored at −80℃ overnight. Magnetic beads were preincubated with 8µg anti-E(spl)mβ polyclonal antibody or the preimmune serum; the latter was used as a negative control. The frozen RIP lysate was thawed and centrifuged. The supernatant was incubated with the magnetic beads-antibody complex at 4℃ overnight. The immunoprecipitates were digested with protease K to remove the proteins and release the RNAs. The RNAs were then purified and reverse transcribed into cDNA using random hexamers. Using these cDNA as template, qPCR was performed with the following primers and all experiments were performed in quadruplicate.
RIP1
5ʹ- CATGGGACACACTCAGACAC −3ʹ
5ʹ-TCATTATCATCGACGTAAGC −3ʹ
RIP2
5ʹ- GCTTACGTCGATGATAATGA −3ʹ
5ʹ-CTAACACTGTACTCAGATTG −3ʹ
RIP3
5ʹ- CAATCTGAGTACAGTGTTAG −3ʹ
5ʹ-GCTGCTGTTGGTCGTAATGT −3ʹ
RIP4
5ʹ- CACTAGGCGTATTTAAGTTAC −3ʹ
5ʹ- TATGCCTTTTGCACTCCGTC −3ʹ
RIP5
5ʹ- ATGGAAAAGGAGACGCAGAG −3ʹ
5ʹ-AGCTCAGGTCTAAGTTAGGC −3ʹ
RIP6
5ʹ- GCCTAACTTAGACCTGAGCT −3ʹ
5ʹ-CCTCTGTTTATCGATCGTTC −3ʹ
RIP7
5ʹ- GAACGATCGATAAACAGAGG −3ʹ
5ʹ-GTAACGCTTGTTGTTTTAAAGC −3ʹ
Statistical analysis
Data analyses were performed with SPSS (Version 17.0, SPSS Inc.). A p value < 0.05 was regarded as statistically significant, and specific statistical tests are reported within each figure legend. Data in all other formats are presented as mean ± SEM, and significance is represented with asterisks (*) within the figures. The sample size used for scutellar macrochaetes analysis or molecular biological experiment (Luciferase assay, CHIP assay, RIP assay) was chosen according to the previous publications [Citation26,Citation44]. The Kolmogorov-Smirnov test was used for normality test. For the data conforming to the normal distribution, experiments with two groups were analyzed using Independent-Samples T test. For the data not conforming to the normal distribution, experiments were analyzed using Mann-Whitney U test.
Author contributions
Mengbo Xu, Yuanhang Xiang and Xiaojun Liu performed molecular biological experiments and analyzed data. Baoyan Bai and Runsheng Chen performed bioinformatic analysis. Meixia Li designed experiments, performed developmental tests and molecular biological experiments and analyzed data. Li Liu and Meixia Li supervised the project and wrote the paper.
Availability of data and materials
All data generated or analyzed during this study are included in the article and its supplementary materials.
Supplemental Material
Download MS Word (381.9 KB)Acknowledgments
We thank Bloomington Drosophila Stock Center (Indiana University, Bloomington, Indiana) and Gene Regulatory Laboratory (School of Medicine, Tsinghua University, Beijing).
Disclosure statement
No potential conflict of interest was reported by the authors.
Supplementary Material
Supplemental data for this article can be accessed here.
Additional information
Funding
References
- Hon CC, Ramilowski JA, Harshbarger J, et al. An atlas of human long non-coding RNAs with accurate 5ʹ ends. Nature. 2017;543:199–204.
- Guttman M, Amit I, Garber M, et al. Chromatin signature reveals over a thousand highly conserved large non-coding RNAs in mammals. Nature. 2009;458:223–227.
- Marchese FP, Raimondi I, Huarte M. The multidimensional mechanisms of long noncoding RNA function. Genome Biol. 2017;18:206.
- Rinn JL, Chang HY. Genome regulation by long noncoding RNAs. Annu Rev Biochem. 2012;81:145–166.
- Gendrel AV, Heard E. Noncoding RNAs and epigenetic mechanisms during X-chromosome inactivation. Annu Rev Cell Dev Biol. 2014;30:561–580.
- Wang KC, Yang YW, Liu B, et al. A long noncoding RNA maintains active chromatin to coordinate homeotic gene expression. Nature. 2011;472:120–124.
- Liu B, Sun L, Liu Q, et al. A cytoplasmic NF-kappaB interacting long noncoding RNA blocks IkappaB phosphorylation and suppresses breast cancer metastasis. Cancer Cell. 2015;27:370–381.
- Marchese FP, Grossi E, Marin-Bejar O, et al. A long noncoding RNA regulates sister chromatid cohesion. Mol Cell. 2016;63:397–407.
- Schmitt AM, Garcia JT, Hung T, et al. An inducible long noncoding RNA amplifies DNA damage signaling. Nat Genet. 2016;48:1370–1376.
- Kino T, Hurt DE, Ichijo T, et al. Noncoding RNA gas5 is a growth arrest- and starvation-associated repressor of the glucocorticoid receptor. Sci Signal. 2010;3:ra8.
- Latos PA, Pauler FM, Koerner MV, et al. Airn transcriptional overlap, but not its lncRNA products, induces imprinted Igf2r silencing. Science. 2012;338:1469–1472.
- Boque-Sastre R, Soler M, Oliveira-Mateos C, et al. Head-to-head antisense transcription and R-loop formation promotes transcriptional activation. Proc Natl Acad Sci USA. 2015;112:5785–5790.
- Postepska-Igielska A, Giwojna A, Gasri-Plotnitsky L, et al. LncRNA Khps1 regulates expression of the protooncogene SPHK1 via triplex-mediated changes in chromatin structure. Mol Cell. 2015;60:626–636.
- Laneve P, Po A, Favia A, et al. The long noncoding RNA linc-NeD125 controls the expression of medulloblastoma driver genes by microRNA sponge activity. Oncotarget. 2017;8:31003–31015.
- Golubyatnikov VP, Bukharina TA, Furman DP. A model study of the morphogenesis of D. melanogaster mechanoreceptors: the central regulatory circuit. J Bioinform Comput Biol. 2015;13:1540006.
- Plunkett CR. The interaction of genetic and environmental factors in development. J Exp Zool. 1926;46:181–244.
- Sturtevant AH. Studies on the bristle pattern of Drosophila. Dev Biol. 1970;21:48–61.
- Furman DP, Bukharina TA. Morphogenesis of Drosophila melanogaster macrochaetes: cell fate determination for bristle organ. J Stem Cells. 2012;7:19–41.
- Calleja M, Renaud O, Usui K, et al. How to pattern an epithelium: lessons from achaete-scute regulation on the notum of Drosophila. Gene. 2002;292:1–12.
- Tuthill JC, Wilson RI. Mechanosensation and adaptive motor control in insects. Curr Biol. 2016;26:R1022–R1038.
- Seeds AM, Ravbar P, Chung P, et al. A suppression hierarchy among competing motor programs drives sequential grooming in Drosophila. eLife. 2014;3:e02951.
- Lai EC, Orgogozo V. A hidden program in Drosophila peripheral neurogenesis revealed: fundamental principles underlying sensory organ diversity. Dev Biol. 2004;269:1–17.
- García-Bellido A, de Celis JF. The complex tale of the achaete-scute complex: a paradigmatic case in the analysis of gene organization and function during development. Genetics. 2009;182:631–639.
- Gómez-Skarmeta JL, Rodríguez I, Martínez C, et al. Cis-regulation of achaete and scute: sharedenhancer-like elements drive their coexpression in proneural clusters of the imaginal discs. Genes Dev. 1995;9:1869–1882.
- Marcellini S, Gibert JM, Simpson P. achaete, but not scute, is dispensable for the peripheral nervous system of Drosophila. Dev Biol. 2005;285:545–553.
- Li M, Wen S, Guo X, et al. The novel long non-coding RNA CRG regulates Drosophila locomotor behavior. Nucleic Acids Res. 2012;40:11714–11727.
- Li M, Xu M, Wen S, et al. One novel long noncoding RNA lnc10 in Drosophila. J Genet Genomics. 2014;41:79–82.
- Kong L, Zhang Y, Ye ZQ, et al. CPC: assess the protein-coding potential of transcripts using sequence features and support vector machine. Nucleic Acids Res. 2007;35:W345–W349.
- Cassidy JJ, Jha AR, Posadas DM, et al. miR-9a minimizes the phenotypic impact of genomic diversity by buffering a transcription factor. Cell. 2013;155:1556–15567.
- Campuzano S, Carramolino L, Cabrera CV, et al. Molecular genetics of the achaete-scute gene complex of D. melanogaster. Cell. 1985;40:327–338.
- Golovnin A, Biryukova I, Romanova O, et al. An endogenous Su(Hw) insulator separates the yellow gene from the Achaete-scute gene complex in Drosophila. Development. 2003;130:3249–3258.
- de Celis JF, de Celis J, Ligoxygakis P, et al. Functional relationships between Notch, Su(H) and the bHLH genes of the E(spl) complex: the E(spl) genes mediate only a subset of Notch activities during imaginal development. Development. 1996;122:2719–2728.
- Romani S, Campuzano S, Macagno ER, et al. Expression of achaete and scute genes in Drosophila imaginal discs and their function in sensory organ development. Genes Dev. 1989;3:997–1007.
- Cubas P, de Celis JF, Campuzano S, et al. Proneural clusters of achaete-scute expression and the generation of sensory organs in the Drosophila imaginal wing disc. Genes Dev. 1991;5:996–1008.
- Giagtzoglou N, Koumbanakis KA, Fullard J, et al. Role of the Sc C terminus in transcriptional activation and E(spl) repressor recruitment. J Biol Chem. 2005;280:1299–1305.
- García-Bellido A, Santamaria P. Developmental analysis of the achaete-scute system of DROSOPHILA MELANOGASTER. Genetics. 1978;88:469–486.
- Fisher A, Caudy M. The function of hairy-related bHLH repressor proteins in cell fate decisions. Bioessays. 1998;20:298–306.
- Delidakis C, Monastirioti M, Magadi SS. E(spl): genetic, developmental, and evolutionary aspects of a group of invertebrate Hes proteins with close ties to Notch signaling. Curr Top Dev Biol. 2014;110:217–262.
- Guo A, Li L, Xia SZ, et al. Conditioned visual flight orientation in Drosophila: dependence on age, practice, and diet. Learn Mem. 1996;3:49–59.
- Essletzbichler P, Konopka T, Santoro F, et al. Megabase-scale deletion using CRISPR/Cas9 to generate a fully haploid human cell line. Genome Res. 2014;24:2059–2065.
- Ren X, Sun J, Housden BE, et al. Optimized gene editing technology for Drosophila melanogaster using germ line-specific Cas9. Proc Natl Acad Sci USA. 2013;110:19012–19017.
- Yu Z, Chen H, Liu J, et al. Various applications of TALEN- and CRISPR/Cas9-mediated homologous ecombination to modify the Drosophila genome. Biol Open. 2014;3:271–280.
- Wang Z, Pan Y, Li W, et al. Visual pattern memory requires foraging function in the central complex of Drosophila. Learn Mem. 2008;15:133–142.
- Hainaut M, Sagnier T, Berenger H, et al. The MYST-containing protein Chameau is required for proper sensory organ specification during Drosophila thorax morphogenesis. PLoS One. 2012;7:e32882.