ABSTRACT
Although it was long assumed that eukaryotic pre-mRNAs are almost always spliced to generate linear mRNAs, it is now clear that thousands of protein-coding genes can be non-canonically spliced using backsplicing to produce circular RNAs (circRNAs). Most mature circRNAs accumulate in the cytoplasm; however, little is known about how circRNAs are exported from the nucleus to the cytoplasm as they lack many of the common signals used for mRNA export. In this point-of-view article, we will discuss our recently identified circRNA nuclear export pathway and address important open questions in the field.
Biogenesis and functions of circRNAs
Although circular RNAs (circRNAs) have been identified decades ago [Citation1–Citation4], how they are produced and what cellular function(s) they may have remains largely unknown. circRNAs are generated from a number of protein-encoding genes when the pre-mRNA splicing machinery ‘backsplices’ to join a splice donor to an upstream splice acceptor. In many cases, the flanking introns contain complementary repeat sequences that bring the splice sites in close proximity and further facilitate circularization [Citation5–Citation8]. However, not all circRNAs contain flanking complementary sequences in their pre-mRNAs; thus, other mechanisms must be in place to produce circRNAs. Furthermore, several RNA binding proteins (e.g. FUS, ADAR, Quaking, MBL, etc.), known to impact splicing regulation or RNA editing, influence circRNAs production [Citation8–Citation11], some of which may act analogously as flanking complementary sequences. For example, the RNA binding protein Quaking (QKI) promotes RNA circularization by binding upstream and downstream of the circularized exons.
Most of circRNAs are expressed at lower levels compared to their parental linear mRNAs [Citation12,Citation13]; however, for some protein-coding genes, it is clear that the circRNA is the major RNA species produced [Citation7,Citation14]. For example, the laccase2 gene in Drosophila DL1 cells produces a circRNA ten times more abundant than the associated linear mRNA [Citation7].
While the function(s) of circRNAs remains largely unknown, some insight into how circRNAs can regulate gene expression has been reported. Two circRNAs (CDR1as/ciRS-7 and Sry) were found to act as microRNA sponges to sequester specific microRNAs, thereby modulating their target mRNAs translation in trans [Citation15,Citation16]. Two exon-intron circRNAs (circEIF3J and circPAIP2) regulate their parental mRNA transcription by interacting with U1 snRNP in the nucleus [Citation17]. Additionally, recent work has shown some circRNAs can be translated in a cap-independent manner [Citation18–Citation20]. What cellular functions these proteins have has not been thoroughly addressed, but future studies should provide critical insight if translation of circRNAs has any role in cellular fitness and homeostasis. Recent studies have now connected circRNAs to brain function, highlighting the potential importance of circRNAs in neurological disorders and other human pathology [Citation21]. Nevertheless, like many other non-coding RNAs (ncRNAs), circRNAs probably have various important roles in many biological events, but their functions are largely yet to be defined [Citation22].
Length-dependent nuclear export of circRNAs
Nuclear export of mature RNA transcripts is crucial for their proper functions. For example, tRNA and rRNA must be exported. Similarly, mRNAs must be exported to then be translated to produce the protein they encode. However, while these different classes of RNAs are all exported from the nucleus to the cytoplasm, they contain distinct ‘signals’ that are used to determine which export pathway can be used [Citation23,Citation24]. For example, the tRNA receptor exportin-t selectively exports tRNA by recognizing secondary and tertiary structural elements of tRNAs [Citation25]. The mRNA nuclear export pathway uses the transcription-export (TREX) complex to bind nascent transcripts via interactions with the processing and transcription factors during successive transcription steps. Typically, the two canonical receptors (Nxt1-Nxf1 heterodimer), recruited by the TREX component ALY as an adaptor, will bring nascent mRNA transcripts through nuclear pore to the cytoplasm [Citation26–Citation32]. Although some of circRNAs are retained in the nucleus (e.g. intronic circRNAs and exon-intron circRNAs) [Citation17,Citation33], most circRNAs accumulate in the cytoplasm [Citation12,Citation34] (). Little is known about how circRNAs are exported form the nucleus as they lack features utilized by RNA export pathways. However, our recent discovery has described a newly defined and evolutionarily conserved circRNA export pathway [Citation35] ().
Figure 1. Nuclear export of circRNAs utilizes two separate pathways based on length. Most circRNAs, generated from exon(s), are exported to the cytoplasm. The factors required for export is determined by the size of the circRNA. In Drosophila cells, only long circRNAs require DExH/D box RNA helicase Hel25E. In human cells, one of the two human homologs of Hel25E (UAP56) similarly regulates nuclear export of long circRNAs. In contrast, URH49, the other human homolog of Hel25E, is required for nuclear export of short circRNAs.
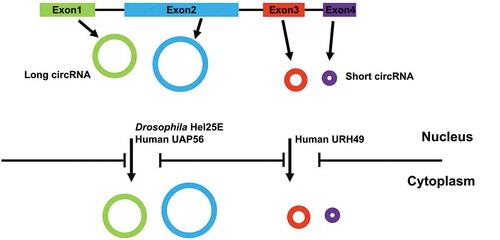
To identify factors involved in circRNA export, we used RNAi screening in Drosophila DL1 cells to knock down 26 potential proteins which have been associated with various RNA export pathways and determine the impact on circRNA cellular localization [Citation35]. We found that depletion of the Drosophila DExH/D box helicase Hel25E resulted in nuclear accumulation of an 1120nt endogenous circRNA (circdati), but not of a 490nt circRNA (circlaccase2) – both of which are typically predominately localized to the cytoplasm. Further investigation by examining 12 additional endogenous circRNAs of varying lengths showed that depletion of Hel25E led to nuclear accumulation of long (>800nt) but not short (<702nt) circRNAs. Likewise, nascent long circRNAs accumulated in the nucleus when the Hel25E was depleted from cells, suggesting that the circRNA nuclear accumulation was not a result of overall changes of circRNA levels, but in fact a defect in export. In addition, we transfected a series of circRNA-expression plasmids which can generate mature circRNAs of different lengths into Hel25E depleted cells. The plasmid-derived circRNA export defect upon Hel25E knockdown was consistent with previous observation. The length of the mature circRNA thus surprisingly dictates the export mechanism.
We further tested this length-dependent circRNA export pathway in human cells. To our surprise, we found that two human orthologues UAP56 and URH49 (which share 90% identity) of Drosophila Hel25E are responsible for separately exporting long and short circRNAs. UAP56/DDX39B is required for export of long circRNAs and URH49/DDX39A is required for short circRNAs. While it appears it may be more complex in human cells, length-dependent nuclear export of circRNA is evolutionarily conserved.
A four amino-acid sequence that regulates circRNA export length preference
While the human Hel25E orthologues UAP56 and URH49 share 90% sequence identity, it was not intuitive how they may regulate circRNA export by RNA length. Since the ATP binding and helicase activity domains are important for the enzymatic function of Hel25E [Citation36,Citation37], we set out to examine whether the ATP binding or helicase activity domains are required for nuclear export of circRNAs in a series of knockdown and rescue experiments. Surprisingly, we found that ATP binding and the helicase activity of Hel25E was largely dispensable for long circRNA nuclear export in fly cells. Given that the amino acid sequence might determine the circRNA length preference of Drosophila Hel25E and its human homologs in circRNA export, we thus reasoned that a motif of Drosophila Hel25E which is similar with human UAP56 but not URH49 might control the length preference. By generating chimeric proteins and rescue experiments, we identified a four amino-acid region, that is divergent between UAP56 and URH49, is necessary and sufficient to dictate their circular RNA length preferences [Citation35].
Together, our data provided evidence that the nuclear export of circRNAs is an evolutionarily conserved process that is regulated by Drosophila Hel25E and its human homologs in a length-dependent manner [Citation35,Citation38,Citation39] ().
Perspectives and moving forward
The length-based regulation of circRNA export is an attractive and exciting discovery that raises many important questions. For instance, (1) which factor(s) regulates short circRNA export in Drosophila cells? Different from human cells, Drosophila cells only contain one Hel25E homolog – which is only involved in long circRNA export. Considering that Hel25E, UAP56 and URH49 are RNA helicases, it would be interesting to test whether there are other equivalent RNA helicases or functionally redundant factors regulating short circRNA export in Drosophila cells. Furthermore, it is also possible that a similar mechanism involving these helicases may be used to measure the size of mRNAs. Additionally, (2) how do Hel25E homologs define long and short circRNAs? Although we provided evidence that supports that a small four amino-acid motif in Hel25E and its homologs directs their circRNA length preference, how this is defined at a molecular level is not known but is currently a main focus of my lab.
According to previous reports, UAP56 and URH49 can bind with different factors to preferentially form distinct mRNA export machineries [Citation40]. Specifically, URH49 forms a novel URH49–CIP29 complex, termed the AREX (alternative mRNA export) complex, whereas UAP56 interacts with THO complex to form TREX complex. In addition, they found that depletion of UAP56 or URH49 affects the cytoplasmic RNA levels of different subsets of genes at the genome-wide level [Citation40]. These observations raise a possibility that UAP56 and URH49 might measure the length of circRNAs (even linear RNAs) through their unique interactions. Also, some evidence suggests that the binding strength correlates with the length of RNA transcripts [Citation41]. It will be critical to determine if UAP56 and URH49 have any ‘tape measuring’ ability or if their direct affinity for RNA follows their length preference for circRNAs.
Interestingly, a length dependence has been showcased in linear mRNA export pathway [Citation42,Citation43]. The PHAX protein facilitates export of short linear transcripts (<200nt) via CRM1 receptor. Whereas, nascent transcripts longer than 200-300nt, are recruited to heterogeneous nuclear ribonucleoprotein C1/C2 (hnRNPC1/C2) heterotetramer in a cap-dependent manner and then committed to the canonical mRNA export pathway by hnRNPC1/C2. Taken together, all hints suggest that RNA length might play a key role in certain molecular processes more often than is currently appreciated. By further addressing how Hel25E homologs define circRNA length, as well as the length of other RNA substrates, we expect to define new examples and paradigms how transcript length may be an important feature in RNA biology.
Acknowledgments
The authors thank members of the Huang lab and the Wilusz lab (University of Pennsylvania) for discussions.
Disclosure statement
No potential conflict of interest was reported by the authors.
Additional information
Funding
References
- Hsu MT, Coca-Prados M. Electron microscopic evidence for the circular form of RNA in the cytoplasm of eukaryotic cells. Nature. 1979;280:339–340.
- Nigro JM, Cho KR, Fearon ER, et al. Scrambled exons. Cell. 1991;64:607–613.
- Capel B, Swain A, Nicolis S, et al. Circular transcripts of the testis-determining gene Sry in adult mouse testis. Cell. 1993;73:1019–1030.
- Cocquerelle C, Daubersies P, Majerus MA, et al. Splicing with inverted order of exons occurs proximal to large introns. Embo J. 1992;11:1095–1098.
- Liang D, Wilusz JE. Short intronic repeat sequences facilitate circular RNA production. Genes Dev. 2014;28:2233–2247.
- Zhang XO, Wang HB, Zhang Y, et al. Complementary sequence-mediated exon circularization. Cell. 2014;159:134–147.
- Kramer MC, Liang D, Tatomer DC, et al. Combinatorial control of Drosophila circular RNA expression by intronic repeats, hnRNPs, and SR proteins. Genes Dev. 2015;29:2168–2182.
- Ivanov A, Memczak S, Wyler E, et al. Analysis of intron sequences reveals hallmarks of circular RNA biogenesis in animals. Cell Rep. 2015;10:170–177.
- Errichelli L, Dini Modigliani S, Laneve P, et al. FUS affects circular RNA expression in murine embryonic stem cell-derived motor neurons. Nat Commun. 2017;8:14741.
- Conn SJ, Pillman KA, Toubia J, et al. The RNA binding protein quaking regulates formation of circRNAs. Cell. 2015;160:1125–1134.
- Ashwal-Fluss R, Meyer M, Pamudurti NR, et al. circRNA biogenesis competes with pre-mRNA splicing. Mol Cell. 2014;56:55–66.
- Salzman J, Gawad C, Wang PL, et al. Circular RNAs are the predominant transcript isoform from hundreds of human genes in diverse cell types. PLoS One. 2012;7:e30733.
- Guo JU, Agarwal V, Guo H, et al. Expanded identification and characterization of mammalian circular RNAs. Genome Biol. 2014;15:409.
- Liang D, Tatomer DC, Luo Z, et al. The output of protein-coding genes shifts to circular RNAs when the Pre-mRNA processing machinery is limiting. Mol Cell. 2017;68:940–54 e3.
- Memczak S, Jens M, Elefsinioti A, et al. Circular RNAs are a large class of animal RNAs with regulatory potency. Nature. 2013;495:333–338.
- Hansen TB, Jensen TI, Clausen BH, et al. Natural RNA circles function as efficient microRNA sponges. Nature. 2013;495:384–388.
- Li Z, Huang C, Bao C, et al. Exon-intron circular RNAs regulate transcription in the nucleus. Nat Struct Mol Biol. 2015;22:256–264.
- Pamudurti NR, Bartok O, Jens M, et al. Translation of CircRNAs. Mol Cell. 2017;66:9–21 e7.
- Yang Y, Fan X, Mao M, et al. Extensive translation of circular RNAs driven by N(6)-methyladenosine. Cell Res. 2017;27:626–641.
- Legnini I, Di Timoteo G, Rossi F, et al. Circ-ZNF609 is a circular RNA that can be translated and functions in myogenesis. Mol Cell. 2017;66:22–37 e9.
- Piwecka M, Glazar P, Hernandez-Miranda LR, et al. Loss of a mammalian circular RNA locus causes miRNA deregulation and affects brain function. Science. 2017;357:eaam8526.
- Huang C, Shan G. What happens at or after transcription: insights into circRNA biogenesis and function. Transcription. 2015;6:61–64.
- Williams T, Ngo LH, Wickramasinghe VO. Nuclear export of RNA: different sizes, shapes and functions. Semin Cell Dev Biol. 2018;75:70–77.
- Katahira J. Nuclear export of messenger RNA. Genes (Basel). 2015;6:163–184.
- Lipowsky G, Bischoff FR, Izaurralde E, et al. Coordination of tRNA nuclear export with processing of tRNA. Rna. 1999;5:539–549.
- Rehwinkel J, Herold A, Gari K, et al. Genome-wide analysis of mRNAs regulated by the THO complex in Drosophila melanogaster. Nat Struct Mol Biol. 2004;11:558–566.
- Masuda S, Das R, Cheng H, et al. Recruitment of the human TREX complex to mRNA during splicing. Genes Dev. 2005;19:1512–1517.
- Chi B, Wang Q, Wu G, et al. Aly and THO are required for assembly of the human TREX complex and association of TREX components with the spliced mRNA. Nucleic Acids Res. 2013;41:1294–1306.
- Cheng H, Dufu K, Lee CS, et al. Human mRNA export machinery recruited to the 5ʹ end of mRNA. Cell. 2006;127:1389–1400.
- Herold A, Klymenko T, Izaurralde E. NXF1/p15 heterodimers are essential for mRNA nuclear export in Drosophila. Rna. 2001;7:1768–1780.
- Herold A, Teixeira L, Izaurralde E. Genome-wide analysis of nuclear mRNA export pathways in Drosophila. Embo J. 2003;22:2472–2483.
- Abruzzi KC, Lacadie S, Rosbash M. Biochemical analysis of TREX complex recruitment to intronless and intron-containing yeast genes. Embo J. 2004;23:2620–2631.
- Zhang Y, Zhang XO, Chen T, et al. Circular intronic long noncoding RNAs. Mol Cell. 2013;51:792–806.
- Jeck WR, Sorrentino JA, Wang K, et al. Circular RNAs are abundant, conserved, and associated with ALU repeats. RNA. 2013;19:141–157.
- Huang C, Liang D, Tatomer DC, et al. A length-dependent evolutionarily conserved pathway controls nuclear export of circular RNAs. Genes Dev. 2018;32:639–644.
- Thakurta AG, Selvanathan SP, Patterson AD, et al. The nuclear export signal of splicing factor Uap56p interacts with nuclear pore-associated protein Rae1p for mRNA export in Schizosaccharomyces pombe. J Biol Chem. 2007;282:17507–17516.
- Kota KP, Wagner SR, Huerta E, et al. Binding of ATP to UAP56 is necessary for mRNA export. J Cell Sci. 2008;121:1526–1537.
- Wan Y, Hopper AK. Size matters: conserved proteins function in length-dependent nuclear export of circular RNAs. Genes Dev. 2018;32:600–601.
- Azmi AS. Nuclear export mechanisms of circular RNAs: size does matter. Noncoding RNA Invest. 2018;2:52.
- Yamazaki T, Fujiwara N, Yukinaga H, et al. The closely related RNA helicases, UAP56 and URH49, preferentially form distinct mRNA export machineries and coordinately regulate mitotic progression. Mol Biol Cell. 2010;21:2953–2965.
- Davidovich C, Wang X, Cifuentes-Rojas C, et al. Toward a consensus on the binding specificity and promiscuity of PRC2 for RNA. Mol Cell. 2015;57:552–558.
- Masuyama K, Taniguchi I, Kataoka N, et al. RNA length defines RNA export pathway. Genes Dev. 2004;18:2074–2085.
- McCloskey A, Taniguchi I, Shinmyozu K, et al. hnRNP C tetramer measures RNA length to classify RNA polymerase II transcripts for export. Science. 2012;335:1643–1646.