ABSTRACT
As one type of the most common endogenous short noncoding RNAs (ncRNAs), microRNAs (miRNAs) act as posttranscriptional regulators of gene expression and have great potential biological functions in the physiological and pathological processes of various diseases. The role of miRNAs in renal fibrosis has also attracted great attention in the previous 20 years, and new therapeutic strategies targeting miRNAs appear to be promising. Some researchers have previously reviewed the roles of miRNA in renal fibrosis disease, but numerous studies have emerged over the recent 5 years. It is necessary to update and summarize research progress in miRNAs in renal fibrosis. Thus, in this review, we summarize progress in miRNA-mediated renal fibrosis over the last 5 years and evaluate the biological functions of some miRNAs in different stages of renal fibrosis. Furthermore, we also expound the recent clinical applications of these miRNAs to provide new insights into the treatment of renal fibrosis disease.
KEYWORDS:
Introduction
Renal fibrosis is the final common outcome and fundamental pathological process of chronic kidney disease (CKD), which represents an important public health problem and results in increased morbidity and mortality. The mechanism of renal fibrosis is not fully understood to date. microRNAs (miRNAs) are small noncoding RNAs (ncRNAs) of ~22 nt in length with great potential biological functions and participate in various kinds of physiological and pathological processes [Citation1–Citation4]. A large amount of evidence suggests that miRNAs contributes to fibrosis in various organs [Citation3,Citation5], and the roles of miRNAs in renal fibrosis have also received great attraction [Citation6,Citation7]. Some reviews have summarized the roles of miRNAs in renal fibrosis [Citation8,Citation9]. However, a large number of new studies in this field have emerged over the last few years [Citation10–Citation12]. From 2013 to 2016, miRNA-mediated renal fibrosis was reviewed by some scholars from different perspectives [Citation8,Citation13–Citation15]. Moreover, as new research hotspots, other types of ncRNAs such as long ncRNAs and circle ncRNAs are also involved in miRNA-mediated mechanisms of renal fibrosis, such as competing endogenous RNAs (ceRNA) [Citation16,Citation17]. It is necessary to provide a supplementary summary of the relevant research in the recent 5 years. Therefore, we hereby summarized the latest research progress in miRNAs in renal fibrosis and evaluate the biological functions of some miRNAs in different stages of renal fibrosis. Furthermore, we also expound clinical application prospects for miRNAs to provide new insights into the treatment of renal fibrosis disease.
Research advances in miRNA expression profiles in renal fibrosis
Considerable new evidence reveals multiple families of miRNAs implicated in renal disease in the last 5 years. These miRNAs are summarized in , and those with more in-depth studies are described in detail below. There is also considerable evidence supporting the therapeutic applications of miRNAs, for example, treatment for renal fibrosis disorders, and as biomarkers in body fluid for the early detection of renal fibrosis diseases. With the development of detection technology in the future, it can be expected that miRNAs as biomarkers have important clinical value in the diagnosis and treatment of renal fibrosis disease.
Table 1. miRNAs implicated in renal fibrosis.
Current studies of therapeutic applications of miRNAs in renal fibrosis diseases mainly focus on the DN model. The use of locked nucleic acid (LNA) technology designing a shorter anti-miR NA to inhibit specific miRNAs is the most commonly used method. In addition, other genetic techniques can be applied for therapeutic applications with miRNAs in the animal renal fibrosis model, including mimic miRNA, antago miRNA, recombinant adeno-associated virus (rAAV)-miRNA, and the less common ultrasound-micro bubble-mediated miRNA [Citation18–Citation27]. It is worth mentioning that C66, as a novel curcumin analogue, can ameliorate DN in mice by increasing the expression of miR-200a and inhibiting the expression of miR-21 [Citation28], which can also be considered a therapeutic application of miRNAs. A few studies have also examined therapeutic applications of miRNAs in other renal fibrosis diseases, inducing AKI-induced later renal fibrosis and ureteral obstruction-induced ON. A study has shown that deletion of miR-150 in mice protects the kidney from myocardial infarction-induced AKI and late fibrosis [Citation29]. Additionally, CsA-induced renal damage and fibrosis have been confirmed to be restored by miR-181c mimics in Nrf2-/- mice [Citation30]. Similarly, the research has demonstrated that miR-34c attenuates EMT transition and kidney fibrosis with ureteral obstruction [Citation31]. In conclusion, all these data support the notion that miRNA may serve as an important factor for protecting renal fibrosis from diverse types of nephropathy. All these therapeutic applications with miR-based gene tools to treat renal fibrosis in recent years are presented in .
Figure 1. Main miRNAs as biomarkers involved in various kidney diseases: 12 miRNAs expression are up-regulated (red) and 4 miRNAs expression is down-regulated (blue) by detecting blood and urine or kidney tissue biopsy in renal disease patients.
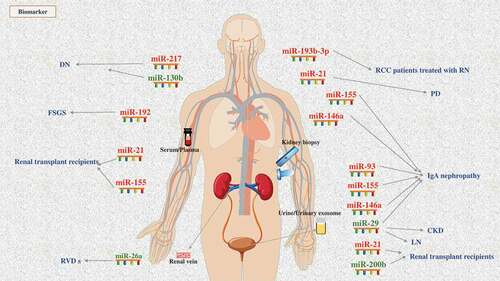
Regardless of the kind of renal fibrosis disease, early diagnosis is very important for prevention, treatment and prognosis. At present, diagnosis of the severity level of renal fibrosis is mainly evaluated by urinary protein, creatinine, urea nitrogen and other indicators, as well as invasive renal tissue biopsy. Despite being the gold standard for diagnosis, innovative practices of renal biopsy still limit its use. Renal biopsy is relatively harmful to patients, while the sensitivity and accuracy of other non-invasive indicators are not satisfactory in clinical practice. Therefore, it is of great clinical significance to seek more accurate, sensitive and non-invasive biomarkers to reflect the degree of renal injury and fibrosis level for the diagnosis and treatment of renal fibrosis disease. shows known miRNAs to date that act as biomarkers for renal fibrosis disease.
miR-21
As one of the first human miRNA genes, miR-21 has been extensively studied in various diseases, including renal fibrosis [Citation32–Citation35]. miR-21 is very highly expressed in kidney tissue and is closely linked to TGF-β signalling and consequently fibrosis [Citation36,Citation37]. It has been widely studied as a marker of renal fibrosis in patients receiving kidney transplantation and has a good positive correlation with the degree of renal fibrosis not only in kidney tissue but also in blood or urine [Citation7,Citation38–Citation40]. In addition, a good association between the expression of miR-21 and renal fibrosis degree has been demonstrated in patients undergoing peritoneal dialysis [Citation41], and miR-21-5p expression in the kidney is associated with fibrosis and renal survival in patients with IgAN [Citation15]. A large number of studies have demonstrated that miR-21 is significantly upregulated in several classic animal models of renal fibrosis, including obstructive nephropathy (ON), diabetic nephropathy (DN), kidney transplantation, and drug toxicity [Citation18–Citation20,Citation35,Citation42]. TGF-β is currently recognized as a key signalling pathway of renal fibrosis, and many scholars have also observed the upregulated expression of miR-21 in vitro in multiple cell lines induced by TGF-β [Citation18,Citation41,Citation43].
miR-23
The miR-23 family regulates proliferation, differentiation and apoptosis in different cell types. The level of miR-23b is significantly decreased in HK2 treated with high glucose (HG) and in kidney tissues of DN mice [Citation44]. These findings were also confirmed in the peripheral blood of diabetes patients and in a DN model in vivo and in vitro [Citation45]. However, another isomiR of mir-23, miR-23a, seems to have the opposite expression trend. miR-23a was recently found to be upregulated in renal tissues of diabetic patients and HK2 cells treated with HG [Citation46].
miR-29
Accumulating studies have demonstrated that the miR-29 (miR-29a, −29b, −29c) family participates in anti-fibrosis in the kidney. miR-29 family expression is increased in the kidney of Smad3 knockout mice with UUO, and it is reduced in proximal tubular cells treated with TGF-β1 [Citation47]. Moreover, miR-29 family expression is decreased in unilateral ureter obstruction (UUO) model rats and NRK52E cells treated with TGF-β [Citation48]. However, different subtypes and isomers of miR-29 show complexity in different models. miR-29b-3p is significantly decreased in a mouse model of UUO and ON patients [Citation49]. miR-29b is largely downregulated in Dahl salt-sensitive rats and responds to diabetic conditions or TGF-β1 treatment [Citation21,Citation50]. miR-29c expression is also remarkably reduced in patients with renal fibrosis disease and in models of renal fibrosis in vivo and vitro [Citation51,Citation52]. Furthermore, all three miRNA isomers are decreased in diabetic mice, but only the changes in miR-29a and miR-29c were significant increased [Citation53]. miR-29a is overexpressed after IRI and subsequently downregulated in the kidney of rats, but it is only detected to be decreased in diabetic kidney [Citation54–Citation56]. In the classic model of hypertension, Ang II induces a downregulation of miR-29a-3p in tubules of rats, while no significant changes are observed in glomeruli [Citation57]. In an interesting study, Yu, Y. suggested that leukaemia inhibitory factory (LIF) increases the expression of miR-29c in kidney fibroblast cells [Citation58]. In addition, miRNAs are known as a potential biomarker for renal fibrosis disease. For example, urinary miR-29b and miR-29c are significantly reduced in IgA nephropathy patients compared with healthy controls [Citation40]. miR-29c in urinary exosomes is regarded as a predictor of early renal fibrosis in lupus nephritis (LN) and correlated with both renal function and the degree of histological fibrosis in other CKD patients [Citation59,Citation60].
miR-34
miRNA-34a has been shown to be downregulated in hypoxic renal PTCs and IRI kidney of mice [Citation55,Citation61,Citation62], but the opposite evidence demonstrates that miR-34a is markedly upregulated in tubulointerstitial spaces and microvesicles isolated from kidney in UUO mice and TGF-β1-treated fibroblasts [Citation63]. Moreover, high glucose upregulates miRNA-34a-5p to aggravate fibrosis in HK-2 cells [Citation64], and miR-34c is upregulated by ureteral obstruction-induced renal fibrosis and TGF-β-induced EMT in mMTCs [Citation31].
miR-146
As a known anti-inflammatory microRNA, miR-146a expression has been observed in kidney samples of DN, IgAN patients and kidney transplant recipients [Citation65–Citation67]. miR-146a expression is also increased in both peritoneal and intrarenal macrophages in diabetic mice and kidney of IRI mice [Citation68]. In vitro, miR-146a is transcriptionally upregulated by ligands of IL-1 receptor/Toll–like receptor family members in cultured renal PTCs [Citation69].
miR-155
Hypoxia has been considered to be a significant microenvironmental factor in promoting renal fibrosis, which has been confirmed to induce overexpression of miR-155 in PTCs [Citation70]. Significantly upregulated expression of miR-155 has been detected in plasma samples of renal transplant recipients [Citation38]. Interestingly, studies indicate that mice and rats have different miR-155 expression profiles in kidneys in response to different models [Citation71,Citation72].
miR-192
The expression and role of miR-192 in kidney disease appear to be more complex than other miRNAs. miR-192 is significantly upregulated in the kidney of animals with UUO or in a remnant kidney model, and in the kidney of patients with hypertensive nephrosclerosis or IgA nephropathy. It also exhibits higher levels in serum of focal segmental glomerulosclerosis (FSGS) patients, which act as markers to differentiate FSGS and minimal change disease (MCD) [Citation73]. miR-192 expression is induced by TGF-β1 in a time- and dosage-dependent manner and is also upregulated in renal proximal tubule cells (PTCs) treated with aristolochic acid (AA) [Citation50,Citation74,Citation75]. However, the reverse expression trend is observed in DN disease. Although miR-192 is upregulated in TGF-β-treated mouse mesothelial cells (MMCs) and in the glomeruli of diabetic mice and contributes to the increased accumulation of collagen in MCs [Citation76,Citation77], other scholars have noted that it is significantly reduced in the late stage of DN fibrosis, with low expression of miR-192 correlated with tubulointerstitial fibrosis. Furthermore, decreased expression of miR-192 has also been detected in proximal tubular cells, tubular cells, and mesangial cells in vitro [Citation22,Citation78,Citation79].
miR-200
Early studies have indicated that miR-200b and miR-200c are upregulated not only in MMCs induced by TGF-β1 but also in the glomeruli of diabetic mice [Citation80]. However, subsequent studies have provided opposite results showing that miR-200 is downregulated in different renal fibrosis models in vivo and in vitro. For example, the miR-200 family would be downregulated in the glomeruli of adult-programmed rats with a maternal low protein diet that may subsequently cause glomeruli epithelial-mesenchymal transformation (EMT). Downregulation of miR-200a has been detected in the kidney in DN and UUO models [Citation28,Citation81–Citation84], as well as inNRK52E cells treated with TGF-β1or TGF-β2, which was shown to induce EMT and fibrogenesis. Moreover, hepatocyte nuclear factor 1 homeobox B (HNF-1β) regulates epithelial differentiation in the mammalian nephron and plays a central role in cystic kidney disease. The expression of miR-200 is decreased in kidneys from HNF-1β knockout mice and renal epithelial cells with HNF-1β knockdown [Citation85]. In addition, the expression of miR-200a and miR-200b also are decreased following 48 h of hypoxic conditions in HK-2 cells [Citation86]. From the point of view of diagnosis and markers, the downregulated urinary miR-200b expression levels are accompanied by renal allograft dysfunction [Citation39].
miR-214
miR-214 has been shown to be not only upregulated in the kidney of UUO mice and the renal cortex of type 1 diabetic mice accompanied with fibronectin expression [Citation87] but also augmented in mesangial and PTECs exposed to high glucose [Citation88]. Furthermore, miR-214-3p is significantly upregulated in IgAN biopsy specimens [Citation89], as well as hypoxia-treated HK-2 cells, UUO model animals and CKD patients [Citation90].
miR-let7
miR-let7b and miR-let7d and fibrogenesis are downregulated by TGF-β1 in NRK52E cells, and miR-let7c is also downregulated in HK2 cells under fibrotic conditions [Citation91]. miR-let7b expression is consistent in different animal model of renal fibrosis [Citation11,Citation92], and decreases in miR-let7a-5p have been observed in UUO model mice, contributing to the excessive deposition of collagen and tissue fibrosis in the kidney [Citation46].
Others miRs
In addition to the miRNA described above, a number of miRNAs have been confirmed to have significant changes in the expression of renal fibrotic models in vitro and in vivo or in human renal fibrotic diseases in recent years [Citation6,Citation10,Citation23,Citation38,Citation58,Citation63,Citation93–Citation112]. For example, studies of miR-30 in renal fibrosis disease focus on DN models, and these results show that miR-30c and miR-30e are all significantly decreased in DN models and are downregulated in NRK-52E cells treated with TGF-β1 [Citation24,Citation25,Citation113,Citation114]. Treatment of human renal epithelial cells with TGF-β1 results in the upregulation of miR-382, which targets SOD2 and induces a loss of epithelial characteristics [Citation115]. The same research team has also observed significantly increased miR-382 in the obstructed kidney at 3 and 7 days in the UUO model [Citation116,Citation117]. Some studies have reported a significant increase in miR-433 in mouse kidneys after UUO for 5 days and in NRK-52E cells treated with TGF-β [Citation118,Citation119]. Even more noteworthy is that miR-217, miR-93, miR-150 and miR-26 are found to have opposite trends compared with the existing literature [Citation29,Citation40,Citation114,Citation120–Citation124]. Decreased miR-26a expression in urine may be act as a good biomarker for renal vascular disease (RVD) [Citation124]. miR-193b-3p is also upregulated as a predictive biomarker of CKD in patients undergoing radical nephrectomy for renal cell carcinoma [Citation102]. Furthermore, other downregulated miRNAs can serve as biomarkers. For example, downregulation of miR-130b in serum may be a new biomarker for the early diagnosis of DN [Citation103]. Aside from the different models or renal diseases, our knowledge about the field of miRNAs in renal fibrosis remains scarce.
As mentioned above, numerous miRNAs are involved in various types of renal fibrosis diseases. However, they may present different or even opposite effects in different kidney fibrosis diseases, different models and even different species or cells. For a clearer presentation, we present a summary of miRNA targets in common types of renal fibrosis disease.
As the most common cause of CKD, DN is one of the most deeply concerning types of renal fibrosis diseases in mi RNA research. miR-192 was early reported to be involved in DN [Citation62]. Interestingly, however, the major findings of Krupa showed that the loss of miR192 is correlated with tubulointerstitial fibrosis and reduction in renal function in patients with DN [Citation22]. In contrast, the specific reduction of miR-192 was found to decrease renal fibrosis to block kidney disease progression in DN model mice [Citation61]. The other miRNA involved in fibrotic disorders is miR-21. Numerous studies have demonstrated that inhibition of miR-21 may be an effective therapy for DN [Citation18–Citation20,Citation26,Citation28,Citation125]. However, miR-30 seems to have different effects than miR-21, and downregulation or overexpression of miR-30 has been found to also ameliorate renal fibrosis in different models [Citation24,Citation114]. miRNAs are known to play important roles in fibrosis pathogenesis of DN, as shown in [Citation10,Citation21,Citation23,Citation27,Citation66,Citation72,Citation73,Citation79,Citation82,Citation88,Citation94,Citation100].
ON is another common cause of chronic renal failure, and the most popular model of this condition is UUO. Morizane found that miR-34c attenuates TGF-β-induced EMT in mouse tubular cells, and miR-34c plasmid decreases the kidney fibrosis degree in UUO mice [Citation79]. Other research has revealed that miR-let7a and miR-29b are involved in the development of fibrosis in ureteropelvic junction obstruction via the control of DTX4 [Citation46].
As the principal cause of ESRD worldwide and one of most common forms of primary glomerulonephritis, IgAN has of course been a recent focus of the miRNA field. Intrarenal expression of miR-200c, miR-141, miR-205 and miR-192 are diversely regulated and correlated with severity and progression in IgAN patients [Citation126]. Moreover, intrarenal and urinary levels of miR-146a and miR-155 are also significantly positively correlated with the clinical and histological severity of IgAN [Citation80]. Furthermore, inhibition of miR-21 prevents fibrogenic activation in podocytes and tubular cells in IgAN [Citation127]. miR-21-5p, miR-214-3p and miR-199a-5p are the three ‘fibro-miRANs’ involved in renal fibrosis in the course of IgAN, and the first two miRNAs are closely associated with the renal survival rate [Citation89].
Angiotensin (Ang) II is a renal growth factor and the major multifactorial peptide, involved in the inactivation of mesangial and tubular cells and interstitial fibroblasts. The miR-221/Ets-1 axis plays an important role in mediating AngII-induced interstitial fibroblast activation and renal fibrosis [Citation108]. Some research has shown that miR-29a-3p plays an important role in renal fibrosis targeted to MMP-2, and the expression of miR-29a-3p in renal tubules treated with AngII is different from that in glomeruli [Citation54].
LN is a highly complex autoimmune disease caused by systemic lupus erythematosus (SLE), and a significant proportion of patients with LN will develop ESRD [Citation128]. miR-150 promotes renal fibrosis in LN by increasing profibrotic molecules [Citation123], and miR-410 suppresses the expression of IL-6 as well as the progression of renal fibrosis in the pathogenesis of LN [Citation110].
AKI is associated with the activation of immune inflammation, which leads to progression from short-term injury to long-term fibrosis. miRNA-21 and miR-155 have been shown to play a critical role in the pathogenesis of kidney injury and the tissue repair process [Citation85], and miR-192 and miR-155 also mediate cisplatin and aristolochic acid-induced nephrotoxicity [Citation59,Citation86]. Subsequently, the deficiency of miR-150 was found to protect the kidney from myocardial infarction-induced AKI [Citation120]. In addition, miR-146a, miR-204, miR-1247, miR-29a and miR-34a mediate fibrosis-associated AKI induced by IR, alcohol and cyclosporine A [Citation30,Citation51,Citation81,Citation96,Citation101].
New advances in therapeutic applications of miRNA in renal fibrosis
Among the various mechanisms of miRNAs involved in renal fibrosis, signalling pathways are undoubtedly the most noteworthy. We summarize recent research progress on miRNA-mediated signalling pathways in .
Figure 2. miRNAs targeted in renal fibrosis diseases (diabetic nephropathy, AKI-induced nephropathy and obstructive nephropathy): targeting on different miRNAs can reduce ECM accumulation, inflammation, apoptosis, and so on, and thus reduce the formation of renal fibrosis in the four different animal models.
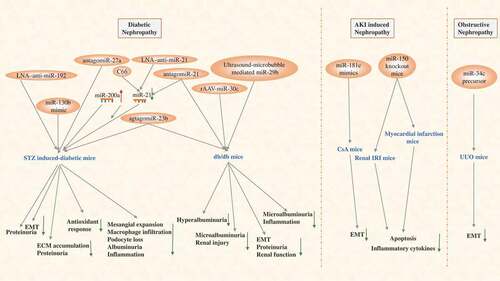
The TGF-β signalling pathway is known as the master regulator of fibrosis [Citation16]. Hence, TGF-β plays a core role in the signalling pathway mechanism of miRNAs involved in renal fibrosis disease. miR-135a is markedly upregulated in DN mice and appears to be affected by high glucose and TGF-β1, followed by promotion of renal fibrosis by regulating the transient receptor potential cation channel, subfamily C, member 1 (TRPC1), during renal injury [Citation94]. TGF-β1 decreases miR-192 expression in PTCs, and overexpression of miR-192 suppresses E-Box repressors Zeb1/2, thereby opposing TGF-β1-mediated downregulation of E-cadherin [Citation22]. Interestingly, another study confirmed that inhibition of miR-192 significantly increases the expression of Zeb1/2 and decreases that of TGF-β in the kidneys of diabetic mice [Citation61]. In addition, some studies have shown that miR-26a and mir-30c play cooperative roles in TGF-β-induced EMT [Citation114]. Therefore, TGF-β and multiple miRNAs regulate each other and positively or negatively regulate the process of renal fibrosis. miRNAs also coregulate the progression of renal fibrosis through the balance between Smad3 and Smad7. For example, it has been verified that overexpression of miR-21 promotes EMT induced by TGF-β1 by inhibiting the target Smad7 [Citation18]. while genetic deletion of miR-21 significantly upregulates the expression of Smad7, suppresses the phosphorylation of Smad3, and then attenuates renal fibrosis in mice [Citation129].
Phosphatase and tensin homologue (PTEN) are tumour suppressor proteins that are widely recognized as a negative regulator of the phosphoinositide 3-kinase (PI3K)/Akt signalling pathway. In fact, multiple miRNAs regulate the fibrosis process by mediating the PTEN or PI3K/AKT signalling pathways in renal fibrosis disease, especially in DN. This study demonstrated that miR-214 targets PTEN via Akt activation, resulting in high-glucose-induced mesangial and proximal tubular cell hypertrophy and fibronectin expression [Citation88]. The fibrotic changes associated with increased miR-21 levels also regulate PI3K-dependent signalling pathways and PTEN [Citation19]. Although the miR-23-mediated PTEN signalling pathway has not yet been observed, some studies have shown that miR-23 acts as a suppressor of EMT in DN by repressing PI3K/Akt signalling pathway activation [Citation72]. Another study has suggested that inhibition of miR-21 prevents fibrogenic activation in podocytes and tubular cells by preventing PTEN/Akt pathway activation in IgAN [Citation127]. PTEN has direct or indirect effects on some signalling pathways, such as PI3K, MAPK and p53, which interact with each other. Among the complicated regulatory nets, miRNAs act as a connecting point to play an important interactive role in the signalling mechanism of renal fibrosis.
NF-κB (nuclear factor kappa light chain enhancer of activated B cells) is a family of highly conserved transcription factors that regulate many important cellular behaviours, in particular, inflammatory responses, which are known to play an important role in renal fibrosis. NF-κB-driven renal inflammation may be an important mechanism associated with miR-29b treatment in db/db mice [Citation21]. Under proinflammatory conditions, miR-146a is transcriptionally upregulated by ligands of IL-1 receptor/Toll–like receptor family members through the activation of NF-kB in cultured renal PTCs [Citation81].
Another signalling pathway, p53, is also very active in the mechanism of miR-mediated renal fibrosis. The expression levels of p53 and miR-192 are increased in the renal cortex of diabetic mice, and the miR-192-mediated p53 signalling pathway enhances renal glomerular expansion and fibrosis [Citation62]. Moreover, hyperglycaemia regulates pathogenic processes in DN through a miR-23b/G3BP2 feedback circuit involving p53 [Citation73]. In addition, signalling pathways, such as notch, MAPK, among others, have also been shown to be involved in the mechanism of miRNA-mediated renal fibrosis [Citation30,Citation114]. Furthermore, overwhelming evidence now indicates the phenotype mechanism of renal fibrosis disease with a focus on excessive deposition of ECM, EMT, and the excessive inflammatory response, among others. As shown in , most miRNAs eventually mediate the above phenotypes through various signalling pathways resulting in renal fibrosis. A further understanding of these various phenotype-mediated miRNAs through the signalling pathway mechanism of renal fibrosis will contribute to the development of novel therapeutics to halt the most damaging processes in CKD.
Conclusion
Over the last few years, there is still no effective treatment to halt the progression of CKD other than supportive care, and this public health problem is a heavy economic burden on society. There is an urgency to identify an effective treatment for renal fibrosis disease. Compared with protein-coding genes, miRNAs with greater functionality provide great hope. However, they also introduce challenges. With further research, as shown in and , we have found that the exact mechanism by which miRNAs regulate renal fibrosis is quite complex. The same miRNA may have different or even opposite effects in different types of renal diseases. Thus, it is very useful to summarize the commonality and specificity of the roles of miRNAs in renal fibrosis, as well as the regulatory mechanisms at different levels of expression, including transcriptional and posttranscriptional levels, and even to investigate miRNA-mediated fibrosis in other organs. Thus, it is important to systematically illuminate different types and causes of renal fibrosis as much as possible. In conclusion, all these data support the notion that miRNAs may serve as an important factor in protecting renal fibrosis from diverse types of nephropathy.
Figure 3. Renal fibrosis mechanism of diabetic nephropathy: miRNAs and signalling pathways are involved in the pathogenesis of renal fibrosis. Ten miRNAs expression is up-regulated (red) and seven miRNAs expression is down-regulated (blue), and fibronectin, ECM, hypertrophy, EMT, collagen, and inflammatory are the main factors leading to renal fibrosis.
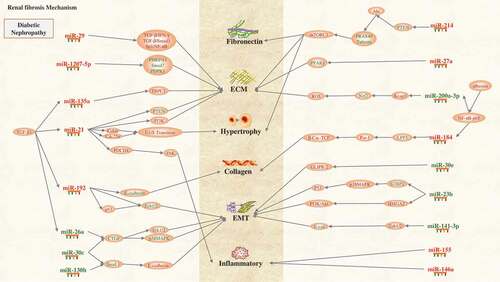
Figure 4. Renal fibrosis mechanism of AKI-induced nephropathy, hypertensive nephropathy, obstructive nephropathy, IgA nephropathy and lupus nephropathy: miRNAs and signalling pathways are involved in the pathogenesis of renal fibrosis. Four miRNAs expression is up-regulated (red) and seven miRNAs expression is down-regulated (blue), and inflammatory, ECM, EMT, αSMA, fibronectin, collagen, and are the main factors leading to renal fibrosis.
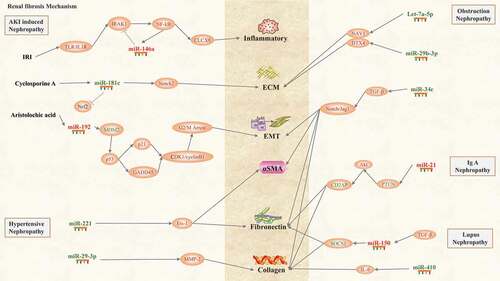
With the further study of the mechanism of renal fibrosis, some new research directions, such as ferroptosis and pyroptosis, deserve our attention. Whether or not miRNAs are related to these mechanisms may also become a new research hotspot. In recent years, the important physiological functions of ncRNAs such as lncRNA and circRNA in the pathophysiology of various systems have attracted much attention. However, the research on renal fibres is still in its infancy. With the in-depth study of the mechanism of renal fibrosis involving lncRNA and circRNA, the role of miRNAs closely related to them in renal fibres will also be necessary to further clarify. In addition, with more and more thorough understanding of the signalling function of exosomes in recent years, the study of miRNAs exerting physiological functions through exosomes in renal fibres will be more extensive. Finally, the origin of fibroblasts in the process of renal fibrosis is still controversial, and the relationship between miRNAs and the origin of fibroblasts, EMT and fibroblasts proliferation and differentiation will be more thoroughly elucidated. In the future, we believe that these findings will aid in understanding the specific functions of miRNAs in the process of renal fibrosis and application of the knowledge of miRNAs to the clinical treatment of renal fibrosis.
Disclosure statement
No potential conflict of interest was reported by the authors.
Additional information
Funding
References
- Hyun J, Jung Y. MicroRNAs in liver fibrosis: focusing on the interaction with hedgehog signaling. World J Gastroenterol. 2016;22(29):6652–6662.
- O’Reilly S. MicroRNAs in fibrosis: opportunities and challenges. Arthritis Res Ther. 2016;18:11.
- Zou XZ, Liu T, Gong Z-C, et al. MicroRNAs-mediated epithelial-mesenchymal transition in fibrotic diseases. Eur J Pharmacol. 2017;796:190–206.
- Lui JC. Regulation of body growth by microRNAs. Mol Cell Endocrinol. 2017;456:2–8.
- He Y, Huang C, Lin X, et al. MicroRNA-29 family, a crucial therapeutic target for fibrosis diseases. Biochimie. 2013;95(7):1355–1359.
- Macconi D, Tomasoni S, Romagnani P, et al. MicroRNA-324-3p promotes renal fibrosis and is a target of ACE inhibition. J Am Soc Nephrol. 2012;23(9):1496–1505.
- Glowacki F, Savary G, Gnemmi V, et al. Increased circulating miR-21 levels are associated with kidney fibrosis. PLoS One. 2013;8(2):e58014.
- Chung AC, Lan HY. MicroRNAs in renal fibrosis. Front Physiol. 2015;6:50.
- Gomez IG, Nakagawa N, Duffield JS. MicroRNAs as novel therapeutic targets to treat kidney injury and fibrosis. Am J Physiol Renal Physiol. 2016;310(10):F931–F944.
- Zanchi C, Macconi D, Trionfini P, et al. MicroRNA-184 is a downstream effector of albuminuria driving renal fibrosis in rats with diabetic nephropathy. Diabetologia. 2017;60(6):1114–1125.
- Wang Y, Le Y, Xue J-Y, et al. Let-7d miRNA prevents TGF-beta1-induced EMT and renal fibrogenesis through regulation of HMGA2 expression. Biochem Biophys Res Commun. 2016;479(4):676–682.
- Zhang A, Li M, Wang B, et al. miRNA-23a/27a attenuates muscle atrophy and renal fibrosis through muscle-kidney crosstalk. J Cachexia Sarcopenia Muscle. 2018;9(4):755–770.
- Srivastava SP, Koya D, Kanasaki K. MicroRNAs in kidney fibrosis and diabetic nephropathy: roles on EMT and EndMT. Biomed Res Int. 2013;2013:125469.
- Wang B, Ricardo S. Role of microRNA machinery in kidney fibrosis. Clin Exp Pharmacol Physiol. 2014;41(8):543–550.
- Van der Hauwaert C, Savary G, Hennino M-F, et al. MicroRNAs in kidney fibrosis. Nephrol Ther. 2015;11(6):474–482.
- Meng XM, Nikolic-Paterson DJ, Lan HY. TGF-beta: the master regulator of fibrosis. Nat Rev Nephrol. 2016;12(6):325–338.
- Kota SK, Kota SB. Noncoding RNA and epigenetic gene regulation in renal diseases. Drug Discov Today. 2017;22(7):1112–1122.
- Wang JY, Gao Y-B, Zhang N, et al. miR-21 overexpression enhances TGF-beta1-induced epithelial-to-mesenchymal transition by target smad7 and aggravates renal damage in diabetic nephropathy. Mol Cell Endocrinol. 2014;392(1–2):163–172.
- McClelland AD, Herman-Edelstein M, Komers R, et al. miR-21 promotes renal fibrosis in diabetic nephropathy by targeting PTEN and SMAD7. Clin Sci (Lond). 2015;129(12):1237–1249.
- Kolling M, Kaucsar T, Schauerte C, et al. Therapeutic miR-21 silencing ameliorates diabetic kidney disease in mice. Mol Ther. 2017;25(1):165–180.
- Chen HY, Zhong X, Huang XR, et al. MicroRNA-29b inhibits diabetic nephropathy in db/db mice. Mol Ther. 2014;22(4):842–853.
- Krupa A, Jenkins R, Luo DD, et al. Loss of microRNA-192 promotes fibrogenesis in diabetic nephropathy. J Am Soc Nephrol. 2010;21(3):438–447.
- Wu L, Wang Q, Guo F, et al. MicroRNA-27a induces mesangial cell injury by targeting of PPARgamma, and its in vivo knockdown prevents progression of diabetic nephropathy. Sci Rep. 2016;6:26072.
- Zhao Y, Yin Z, Li H, et al. MiR-30c protects diabetic nephropathy by suppressing epithelial-to-mesenchymal transition in db/db mice. Aging Cell. 2017;16(2):387–400.
- Zhao D, Jia J, Shao H. miR-30e targets GLIPR-2 to modulate diabetic nephropathy: in vitro and in vivo experiments. J Mol Endocrinol. 2017;59(2):181–190.
- Wang J, Gao Y, Ma M, et al. Effect of miR-21 on renal fibrosis by regulating MMP-9 and TIMP1 in kk-ay diabetic nephropathy mice. Cell Biochem Biophys. 2013;67(2):537–546.
- Bai X, Geng J, Zhou Z, et al. MicroRNA-130b improves renal tubulointerstitial fibrosis via repression of snail-induced epithelial-mesenchymal transition in diabetic nephropathy. Sci Rep. 2016;6:20475.
- Wu H, Kong L, Tan Y, et al. C66 ameliorates diabetic nephropathy in mice by both upregulating NRF2 function via increase in miR-200a and inhibiting miR-21. Diabetologia. 2016;59(7):1558–1568.
- Ranganathan P, Jayakumar C, Tang Y, et al. MicroRNA-150 deletion in mice protects kidney from myocardial infarction-induced acute kidney injury. Am J Physiol Renal Physiol. 2015;309(6):F551–F558.
- Sun W, Min B, Du D, et al. miR-181c protects CsA-induced renal damage and fibrosis through inhibiting EMT. FEBS Lett. 2017;591(21):3588–3599.
- Morizane R, Fujii S, Monkawa T, et al. miR-34c attenuates epithelial-mesenchymal transition and kidney fibrosis with ureteral obstruction. Sci Rep. 2014;4:4578.
- Xu X, Kriegel AJ, Jiao X, et al. miR-21 in ischemia/reperfusion injury: a double-edged sword? Physiol Genomics. 2014;46(21):789–797.
- Zhou TB, Jiang ZP. Role of miR-21 and its signaling pathways in renal diseases. J Recept Signal Transduct Res. 2014;34(5):335–337.
- Loboda A, Sobczak M, Jozkowicz A, et al. TGF-beta1/Smads and miR-21 in renal fibrosis and inflammation. Mediators Inflamm. 2016;2016:8319283.
- Schauerte C, Hübner A, Rong S, et al. Antagonism of profibrotic microRNA-21 improves outcome of murine chronic renal allograft dysfunction. Kidney Int. 2017;92(3):646–656.
- Denby L, Baker AH. Targeting non-coding RNA for the therapy of renal disease. Curr Opin Pharmacol. 2016;27:70–77.
- Zhong X, Chung ACK, Chen H-Y, et al. Smad3-mediated upregulation of miR-21 promotes renal fibrosis. J Am Soc Nephrol. 2011;22(9):1668–1681.
- Zununi Vahed S, Poursadegh Zonouzi A, Ghanbarian H, et al. Differential expression of circulating miR-21, miR-142-3p and miR-155 in renal transplant recipients with impaired graft function. Int Urol Nephrol. 2017;49(9):1681–1689.
- Zununi Vahed S, Omidi Y, Ardalan M, et al. Dysregulation of urinary miR-21 and miR-200b associated with interstitial fibrosis and tubular atrophy (IFTA) in renal transplant recipients. Clin Biochem. 2017;50(1–2):32–39.
- Wang G, Kwan BC-H, Lai FM-M, et al. Urinary miR-21, miR-29, and miR-93: novel biomarkers of fibrosis. Am J Nephrol. 2012;36(5):412–418.
- Lopez-Anton M, Lambie M, Lopez-Cabrera M, et al. miR-21 promotes fibrogenesis in peritoneal dialysis. Am J Pathol. 2017;187(7):1537–1550.
- Chen J, Zmijewska A, Zhi D, et al. Cyclosporine-mediated allograft fibrosis is associated with micro-RNA-21 through AKT signaling. Transpl Int. 2015;28(2):232–245.
- Liu X, Hong Q, Wang Z, et al. Transforming growth factor-beta-sphingosine kinase 1/S1P signaling upregulates microRNA-21 to promote fibrosis in renal tubular epithelial cells. Exp Biol Med (Maywood). 2016;241(3):265–272.
- Liu H, Wang X, Liu S, et al. Effects and mechanism of miR-23b on glucose-mediated epithelial-to-mesenchymal transition in diabetic nephropathy. Int J Biochem Cell Biol. 2016;70:149–160.
- Zhao B, Li H, Liu J, et al. MicroRNA-23b targets Ras GTPase-activating protein SH3 domain-binding protein 2 to alleviate fibrosis and albuminuria in diabetic nephropathy. J Am Soc Nephrol. 2016;27(9):2597–2608.
- Xu H, Sun F, Li X, et al. Down-regulation of miR-23a inhibits high glucose-induced EMT and renal fibrogenesis by up-regulation of SnoN. Hum Cell. 2018;31(1):22–32.
- Qin W, Chung ACK, Huang XR, et al. TGF-beta/Smad3 signaling promotes renal fibrosis by inhibiting miR-29. J Am Soc Nephrol. 2011;22(8):1462–1474.
- Ramdas V, McBride M, Denby L, et al. Canonical transforming growth factor-beta signaling regulates disintegrin metalloprotease expression in experimental renal fibrosis via miR-29. Am J Pathol. 2013;183(6):1885–1896.
- Papadopoulos T, Casemayou A, Neau E, et al. Systems biology combining human- and animal-data miRNA and mRNA data identifies new targets in ureteropelvic junction obstruction. BMC Syst Biol. 2017;11(1):31.
- Chung AC, Dong Y, Yang W, et al. Smad7 suppresses renal fibrosis via altering expression of TGF-beta/Smad3-regulated microRNAs. Mol Ther. 2013;21(2):388–398.
- Fang Y, Yu X, Liu Y, et al. miR-29c is downregulated in renal interstitial fibrosis in humans and rats and restored by HIF-alpha activation. Am J Physiol Renal Physiol. 2013;304(10):F1274–F1282.
- Jiang L, Zhou Y, Xiong M, et al. Sp1 mediates microRNA-29c-regulated type I collagen production in renal tubular epithelial cells. Exp Cell Res. 2013;319(14):2254–2265.
- Wang B, Komers R, Carew R, et al. Suppression of microRNA-29 expression by TGF-beta1 promotes collagen expression and renal fibrosis. J Am Soc Nephrol. 2012;23(2):252–265.
- Rodrigues CE, Capcha JM, de Braganca AC, et al. Human umbilical cord-derived mesenchymal stromal cells protect against premature renal senescence resulting from oxidative stress in rats with acute kidney injury. Stem Cell Res Ther. 2017;8(1):19.
- Lin CL, Lee P-H, Hsu Y-C, et al. MicroRNA-29a promotion of nephrin acetylation ameliorates hyperglycemia-induced podocyte dysfunction. J Am Soc Nephrol. 2014;25(8):1698–1709.
- Hsu YC, Chang P-J, Ho C, et al. Protective effects of miR-29a on diabetic glomerular dysfunction by modulation of DKK1/Wnt/beta-catenin signaling. Sci Rep. 2016;6:30575.
- Castoldi G, Di Gioia C, Giollo F, et al. Different regulation of miR-29a-3p in glomeruli and tubules in an experimental model of angiotensin II-dependent hypertension: potential role in renal fibrosis. Clin Exp Pharmacol Physiol. 2016;43(3):335–342.
- Yu Y, Wang Y, Niu Y, et al. Leukemia inhibitory factor attenuates renal fibrosis through Stat3-miR-29c. Am J Physiol Renal Physiol. 2015;309(7):F595–F603.
- Sole C, Cortés-Hernández J, Felip ML, et al. miR-29c in urinary exosomes as predictor of early renal fibrosis in lupus nephritis. Nephrol Dial Transplant. 2015;30(9):1488–1496.
- Lv LL, Cao Y-H, Ni H-F, et al. MicroRNA-29c in urinary exosome/microvesicle as a biomarker of renal fibrosis. Am J Physiol Renal Physiol. 2013;305(8):F1220–F1227.
- Du R, Sun W, Xia L, et al. Hypoxia-induced down-regulation of microRNA-34a promotes EMT by targeting the notch signaling pathway in tubular epithelial cells. PLoS One. 2012;7(2):e30771.
- Lu X, Chen Z, Liang H, et al. Thyroid hormone inhibits TGFbeta1 induced renal tubular epithelial to mesenchymal transition by increasing miR34a expression. Cell Signal. 2013;25(10):1949–1954.
- Yang Z, Xiong M, Niu J, et al. Secreted fibroblast miR-34a induces tubular cell apoptosis in fibrotic kidney. J Cell Sci. 2014;127(20):4494–4506.
- Xue M, Li Y, Hu F, et al. High glucose up-regulates microRNA-34a-5p to aggravate fibrosis by targeting SIRT1 in HK-2cells. Biochem Biophys Res Commun. 2018;498(1):38–44.
- Huang Y, Liu Y, Li l, et al. Involvement of inflammation-related miR-155 and miR-146a in diabetic nephropathy: implications for glomerular endothelial injury. BMC Nephrol. 2014;15:142.
- Wang G, Kwan BC-H, Lai FM-M, et al. Elevated levels of miR-146a and miR-155 in kidney biopsy and urine from patients with IgA nephropathy. Dis Markers. 2011;30(4):171–179.
- Amrouche L, Desbuissons G, Rabant M, et al. MicroRNA-146a in human and experimental ischemic AKI: CXCL8-dependent mechanism of action. J Am Soc Nephrol. 2017;28(2):479–493.
- Bhatt K, Lanting LL, Jia Y, et al. Anti-inflammatory role of MicroRNA-146a in the pathogenesis of diabetic nephropathy. J Am Soc Nephrol. 2016;27(8):2277–2288.
- Morishita Y, Imai T, Yoshizawa H, et al. Delivery of microRNA-146a with polyethylenimine nanoparticles inhibits renal fibrosis in vivo. Int J Nanomedicine. 2015;10:3475–3488.
- Xie S, Chen H, Li F, et al. Hypoxia-induced microRNA-155 promotes fibrosis in proximal tubule cells. Mol Med Rep. 2015;11(6):4555–4560.
- Saikumar J, Hoffmann D, Kim T-M, et al. Expression, circulation, and excretion profile of microRNA-21, −155, and −18a following acute kidney injury. Toxicol Sci. 2012;129(2):256–267.
- Kathryn L, Pellegrini TH, Bijol V, et al. MicroRNA-155 deficient mice experience heightened kidney toxicity when dosed with cisplatin. ToxSci Adv Access. 2014;141(2):484–492.
- Cai X, Xia Z, Zhang C, et al. Serum microRNAs levels in primary focal segmental glomerulosclerosis. Pediatr Nephrol. 2013;28(9):1797–1801.
- Jenkins RH, Davies LC, Taylor PR, et al. miR-192 induces G2/M growth arrest in aristolochic acid nephropathy. Am J Pathol. 2014;184(4):996–1009.
- Hong JP, Li X-M, Li M-X, et al. VEGF suppresses epithelial-mesenchymal transition by inhibiting the expression of Smad3 and miR192, a Smad3-dependent microRNA. Int J Mol Med. 2013;31(6):1436–1442.
- Putta S, Lanting L, Sun G, et al. Inhibiting microRNA-192 ameliorates renal fibrosis in diabetic nephropathy. J Am Soc Nephrol. 2012;23(3):458–469.
- Deshpande SD, Putta S, Wang M, et al. Transforming growth factor-beta-induced cross talk between p53 and a microRNA in the pathogenesis of diabetic nephropathy. Diabetes. 2013;62(9):3151–3162.
- Wang B, Herman-Edelstein M, Koh P, et al. E-cadherin expression is regulated by miR-192/215 by a mechanism that is independent of the profibrotic effects of transforming growth factor-beta. Diabetes. 2010;59(7):1794–1802.
- Jenkins RH, Martin J, Phillips AO, et al. Pleiotropy of microRNA-192 in the kidney. Biochem Soc Trans. 2012;40(4):762–767.
- Kato M, Arce L, Wang M, et al. A microRNA circuit mediates transforming growth factor-beta1 autoregulation in renal glomerular mesangial cells. Kidney Int. 2011;80(4):358–368.
- Wei J, Zhang Y, Luo Y, et al. Aldose reductase regulates miR-200a-3p/141-3p to coordinate Keap1-Nrf2, Tgfbeta1/2, and Zeb1/2 signaling in renal mesangial cells and the renal cortex of diabetic mice. Free Radic Biol Med. 2014;67:91–102.
- Xiong M, Jiang L, Zhou Y, et al. The miR-200 family regulates TGF-beta1-induced renal tubular epithelial to mesenchymal transition through Smad pathway by targeting ZEB1 and ZEB2 expression. Am J Physiol Renal Physiol. 2012;302(3):F369–F379.
- Gong Y, Qin Z, Zhou B, et al. MicroRNA-200a inhibits transforming growth factor beta1-induced proximal tubular epithelial-mesenchymal transition by targeting beta-catenin. Nephron. 2017;137(3):237–249.
- Wang B, Koh P, Winbanks C, et al. miR-200a prevents renal fibrogenesis through repression of TGF-beta2 expression. Diabetes. 2011;60(1):280–287.
- Hajarnis SS, Patel V, Aboudehen K, et al. Transcription factor Hepatocyte Nuclear Factor-1beta (HNF-1beta) regulates microRNA-200 expression through a long noncoding RNA. J Biol Chem. 2015;290(41):24793–24805.
- Bai J, Xiao X, Zhang X, et al. Erythropoietin inhibits hypoxia-induced epithelial-to-mesenchymal transition via upregulation of miR-200b in HK-2 cells. Cell Physiol Biochem. 2017;42(1):269–280.
- Denby L, Ramdas V, Lu R, et al. MicroRNA-214 antagonism protects against renal fibrosis. J Am Soc Nephrol. 2014;25(1):65–80.
- Bera A, Das F, Ghosh-Choudhury N, et al. Reciprocal regulation of miR-214 and PTEN by high glucose regulates renal glomerular mesangial and proximal tubular epithelial cell hypertrophy and matrix expansion. Am J Physiol Cell Physiol. 2017;313(4):C430–C447.
- Hennino MF, Buob D, Van der Hauwaert C, et al. miR-21-5p renal expression is associated with fibrosis and renal survival in patients with IgA nephropathy. Sci Rep. 2016;6:27209.
- Liu M, Liu L, Bai M, et al. Hypoxia-induced activation of Twist/miR-214/E-cadherin axis promotes renal tubular epithelial cell mesenchymal transition and renal fibrosis. Biochem Biophys Res Commun. 2018;495(3):2324–2330.
- Brennan EP, Nolan KA, Börgeson E, et al. Lipoxins attenuate renal fibrosis by inducing let-7c and suppressing TGFbetaR1. J Am Soc Nephrol. 2013;24(4):627–637.
- Wang B, Jha JC, Hagiwara S, et al. Transforming growth factor-beta1-mediated renal fibrosis is dependent on the regulation of transforming growth factor receptor 1 expression by let-7b. Kidney Int. 2014;85(2):352–361.
- Bijkerk R, de Bruin RG, van Solingen C, et al. Silencing of microRNA-132 reduces renal fibrosis by selectively inhibiting myofibroblast proliferation. Kidney Int. 2016;89(6):1268–1280.
- He F, Peng F, Xia X, et al. MiR-135a promotes renal fibrosis in diabetic nephropathy by regulating TRPC1. Diabetologia. 2014;57(8):1726–1736.
- Trevisani F, Ghidini M, Larcher A, et al. MicroRNA 193b-3p as a predictive biomarker of chronic kidney disease in patients undergoing radical nephrectomy for renal cell carcinoma. Br J Cancer. 2016;115(11):1343–1350.
- Chen SJ, Wu P, Sun L-J, et al. miR-204 regulates epithelial-mesenchymal transition by targeting SP1 in the tubular epithelial cells after acute kidney injury induced by ischemia-reperfusion. Oncol Rep. 2017;37(2):1148–1158.
- Noel Faherty SPC, O’Donovan H, Martin F, et al. CCN2/CTGF increases expression of miR-302 microRNAs, which target the TGFβ type II receptor with implications for nephropathic cell phenotypes. J Cell Sci. 2012;125(23):5621–5629.
- Sun J, Yin A, Zhao F, et al. Protection of tubular epithelial cells during renal injury via post-transcriptional control of BMP7. Mol Cell Biochem. 2017;435(1–2):141–148.
- Mohan A, Singh RS, Kumari M, et al. Urinary exosomal microRNA-451-5p is a potential early biomarker of diabetic nephropathy in rats. PLoS One. 2016;11(4):e0154055.
- Alvarez ML, Khosroheidari M, Eddy E, et al. Role of microRNA 1207-5P and its host gene, the long non-coding RNA Pvt1, as mediators of extracellular matrix accumulation in the kidney: implications for diabetic nephropathy. PLoS One. 2013;8(10):e77468.
- Dreval K, de Conti A, Furuya S, et al. miR-1247 blocks SOX9-mediated regeneration in alcohol- and fibrosis-associated acute kidney injury in mice. Toxicology. 2017;384:40–49.
- Xiao L, Zhou X, Liu F, et al. MicroRNA-129-5p modulates epithelial-to-mesenchymal transition by targeting SIP1 and SOX4 during peritoneal dialysis. Lab Invest. 2015;95(7):817–832.
- Lv C, Zhou Y-H, Wu C, et al. The changes in miR-130b levels in human serum and the correlation with the severity of diabetic nephropathy. Diabetes Metab Res Rev. 2015;31(7):717–724.
- Yin S, Zhang Q, Yang J, et al. TGFβ-incurred epigenetic aberrations of miRNA and DNA methyltransferase suppress Klotho and potentiate renal fibrosis. Biochim Biophys Acta Mol Cell Res. 2017;1864(7):1207–1216.
- Xu P, Guan M-P, Bi J-G, et al. High glucose down-regulates microRNA-181a-5p to increase pro-fibrotic gene expression by targeting early growth response factor 1 in HK-2 cells. Cell Signal. 2017;31:96–104.
- Xue R, Li Y, Li X, et al. miR-185 affected the EMT, cell viability and proliferation via DNMT1/MEG3 pathway in TGF-beta1-induced renal fibrosis. Cell Biol Int. 2018;43(10):1152–1162.
- Meng J, Li L, Zhao Y, et al. MicroRNA-196a/b mitigate renal fibrosis by targeting TGF-β receptor 2. J Am Soc Nephrol. 2016;27(10):3006–3021.
- Di J, Jiang L, Zhou Y, et al. Ets-1 targeted by microrna-221 regulates angiotensin II-induced renal fibroblast activation and fibrosis. Cell Physiol Biochem. 2014;34(4):1063–1074.
- Chen C-H, Cheng C-Y, Chen Y-C, et al. MicroRNA-328 inhibits renal tubular cell epithelial-to-mesenchymal transition by targeting the CD44 in pressure-induced renal fibrosis. PLoS One. 2014;9(6):e99802.
- Liu D, Zhang N, Zhang J, et al. miR-410 suppresses the expression of interleukin-6 as well as renal fibrosis in the pathogenesis of lupus nephritis. Clin Exp Pharmacol Physiol. 2016;43(6):616–625.
- Zhang K, Zhang G-Q, Zhang R-Y, et al. miRNA589 regulates epithelial-mesenchymal transition in human peritoneal mesothelial cells. J Biomed Biotechnol. 2012;2012:673096.
- Liu T, Nie F, Yang X, et al. MicroRNA-590 is an EMT-suppressive microRNA involved in the TGFbeta signaling pathway. Mol Med Rep. 2015;12(5):7403–7411.
- Wang J, Duan L, Guo T, et al. Downregulation of miR-30c promotes renal fibrosis by target CTGF in diabetic nephropathy. J Diabetes Complications. 2016;30(3):406–414.
- Zheng Z, Lin Z, Buawangpong N, et al. The coordinated roles of miR-26a and miR-30c in regulating TGFbeta1-induced epithelial-to-mesenchymal transition in diabetic nephropathy. Sci Rep. 2016;6:37492.
- Kriegel AJ, Fang Y, Liu Y, et al. MicroRNA-target pairs in human renal epithelial cells treated with transforming growth factor beta 1: a novel role of miR-382. Nucleic Acids Res. 2010;38(22):8338–8347.
- Kriegel AJ, Liu Y, Cohen B, et al. MiR-382 targeting of kallikrein 5 contributes to renal inner medullary interstitial fibrosis. Physiol Genomics. 2012;44(4):259–267.
- Fang Y, Xie T, Xue N, et al. miR-382 contributes to renal tubulointerstitial fibrosis by downregulating HSPD1. Oxid Med Cell Longev. 2017;2017:4708516.
- Kato M. TGF-beta-induced signaling circuit loops mediated by microRNAs as new therapeutic targets for renal fibrosis? Kidney Int. 2013;84(6):1067–1069.
- Li R, Chung ACK, Dong Y, et al. The microRNA miR-433 promotes renal fibrosis by amplifying the TGF-beta/Smad3-Azin1 pathway. Kidney Int. 2013;84(6):1129–1144.
- Han F, Konkalmatt P, Chen J, et al. MiR-217 mediates the protective effects of the dopamine D2 receptor on fibrosis in human renal proximal tubule cells. Hypertension. 2015;65(5):1118–1125.
- Shao Y, Ren H, Lv C, et al. Changes of serum Mir-217 and the correlation with the severity in type 2 diabetes patients with different stages of diabetic kidney disease. Endocrine. 2017;55(1):130–138.
- Ma J, Zhang L, Hao J, et al. Up-regulation of microRNA-93 inhibits TGF-beta1-induced EMT and renal fibrogenesis by down-regulation of Orai1. J Pharmacol Sci. 2018;136(4):218–227.
- Zhou H, Hasni SA, Perez P, et al. miR-150 promotes renal fibrosis in lupus nephritis by downregulating SOCS1. J Am Soc Nephrol. 2013;24(7):1073–1087.
- Zhu XY, Ebrahimi B, Eirin A, et al. Renal vein levels of microRNA-26a are lower in the poststenotic kidney. J Am Soc Nephrol. 2015;26(6):1378–1388.
- Zhong X, Chung ACK, Chen HY, et al. miR-21 is a key therapeutic target for renal injury in a mouse model of type 2 diabetes. Diabetologia. 2013;56(3):663–674.
- Wang G, Kwan BC-H, Lai F-M-M, et al. Intrarenal expression of microRNAs in patients with IgA nephropathy. Lab Invest. 2010;90(1):98–103.
- Bao H, Hu S, Zhang C, et al. Inhibition of miRNA-21 prevents fibrogenic activation in podocytes and tubular cells in IgA nephropathy. Biochem Biophys Res Commun. 2014;444(4):455–460.
- Tachaudomdach C, Kantachuvesiri S, Wongpraphairot S, et al. High collagen I gene expression as an independent predictor of adverse renal outcomes in lupus nephritis patients with preserved renal function. Arch Pathol Lab Med. 2015;139(3):378–387.
- Wu X, Ding X, Ding Z, et al. Total flavonoids from leaves of carya cathayensis ameliorate renal fibrosis via the miR-21/Smad7 signaling pathway. Cell Physiol Biochem. 2018;49(4):1551–1563.