ABSTRACT
Transcription factor p53 is activated in response to numerous stress stimuli in order to promote repair and survival or death of abnormal cells. For decades, regulatory mechanisms and downstream targets that execute the many biological functions of tumour suppressor p53 largely focused on the products of protein-coding genes. Recently, an entirely new class of molecules, termed long non-coding RNAs (lncRNAs), were discovered as key regulatory players in shaping p53 activity and biological outcomes. Many p53-regulated lncRNAs are now reported to either directly or indirectly intervene in p53-regulatory networks, generally in fine-tuning p53’s tumour surveillance programme. Recent studies reveal that signals that converge upon p53 to regulate its activity, and molecules that implement downstream p53-response include both proteins and lncRNAs. In this review, we discuss the non-proteomic component of p53-regulatory networks, focusing on lncRNAs regulated by p53 and/or that regulate p53 activity, and their impact on biological outcomes.
Introduction
The gene TP53 (Trp53 in mice), which encodes p53, is the most frequently mutated tumour suppressor gene in human cancers and, as a result, one of the most extensively studied regulatory networks [Citation1,Citation2]. Loss of normal p53-transcriptional activity, by mutations in TP53 or activation of pathways that negatively regulate p53, is a major contributing factor to malignant transformation. p53 responds to a variety of stress signals, including DNA damage, replicative stress, hypoxia, oxidative stress, and nutrient deprivation, by regulating the expression of a myriad of genes, to safeguard genomic stability and suppressing tumour development [Citation3]. In response to stress, p53 is post-translationally modified at specific residues to disrupt negative feedback loops that lead to p53 protein degradation. As p53 becomes more stable, it binds to consensus p53-response elements (p53RE) in order to regulate the expression of target genes in the execution of p53 stress and surveillance response [Citation4,Citation5]. The major biological processes downstream of p53 include cell cycle arrest, apoptosis, senescence and autophagy, metabolic reprogramming, ferroptosis, and inhibition of ‘stemness’, as part of p53’s broader roles in cellular homoeostasis and tumour suppression [Citation3,Citation6]. The molecular mechanisms regulating these processes are just as diverse and continue to unfold. Collective efforts of multiple laboratories over past three decades have revealed several elements and regulatory aspects of the p53-pathway such as, the stress stimuli that regulate p53, post-translational modifications of p53, proteins that regulate p53 protein stability, protein partners that co-regulate p53’s transcriptional output, global chromatin localization of p53 and transcriptomes regulated by p53 in various cellular contexts [Citation6–8]. These genome-wide transcriptomic analyses led to the identification of numerous, previously unknown p53-gene targets and revealed novel activities of p53; however, these studies focused largely on protein-coding genes.
Advancements in large-scale, high-throughput genomic techniques and sequencing of the human genome revealed that only 2% of our genes encode proteins and the remaining 98% was referred to as ‘junk DNA’ [Citation9,Citation10]. However, subsequent investigations supported by consortiums, e.g. ENCODE and others, revealed that three-quarters of the human genome may potentially be transcribed [Citation11,Citation12] into non-coding RNA molecules (ncRNA) that not only regulate fundamental biological processes such as growth and development, but also play critical roles in several diseases, including cancer [Citation13]. An important subset of ncRNAs is long non-coding RNA (lncRNA), characterized as transcripts greater than 200 base pairs, transcribed by RNA Polymerase II, capped, polyadenylated, tissue-specific, and poorly conserved among species. In a majority of cases, lncRNAs are spliced through canonical mechanisms similar to mRNAs [Citation14–16]. LncRNAs regulate key cellular processes, including proliferation, metastasis, genomic stability, and differentiation and development, and often interface with epigenetic machinery as effector molecules that fine-tune transcriptional output in a context-dependent manner [Citation17,Citation18]. LncRNAs can operate through different mechanisms, as flexible molecular scaffolds for protein–protein interactions, molecular decoys, or guides to target elements in the genome and can function either in cis or trans to regulate gene expression [Citation19,Citation20]. One of the well-characterized mechanisms of lncRNA activity is guiding RNA/protein complexes to specific genomic loci to influence gene expression [Citation19]. It is essential to understand the expression of lncRNAs in different cellular contexts and how their integration with the transcription machinery impacts the response of a transcription factor, especially one as critical in the regulation of cellular surveillance and homoeostasis as p53.
Recently, by utilizing cutting-edge high-throughput genomic approaches, several laboratories, including ours, reported an expanded p53-regulated transcriptome that includes numerous p53-lncRNA targets, which can functionally integrate in the p53 pathway [Citation21–Citation23]. LncRNAs, some of which are themselves transcriptionally regulated by p53, form a regulatory network to either amplify or counter p53 response (). Many of these p53-lncRNA targets augment canonical p53-transcription activity and play significant roles in managing the tumour progression. These studies are a shift from a solely proteomic view regarding the regulatory aspects of the p53 pathway. Here, we review the functional significance and mechanism of action of lncRNA components in the p53 network, highlighting specific lncRNAs that are transcriptionally regulated by p53 and/or regulate p53-transcriptional activity.
Table 1. LncRNAs that interface with p53-pathway.
LncRNAs transcriptionally regulated by p53
p53 exerts its transcriptional activity by directly binding to p53REs, either in the promoter or enhancer, to regulate expression of target genes [Citation4,Citation24]. The historical view of p53 controlling the only expression of protein-coding genes to execute its response changed after identification of a number of lncRNAs that are a direct transcriptional target of p53 (example: LincRNA-p21, LINC-PINT, NORAD, DINO, TUG1, PINCR, LED) [Citation21,Citation22], a majority of which amplify p53-response and tumour suppression ( and ).
Figure 1. P53-regulated lncRNAs control diverse biological processes. Active p53 in response to DNA damage or other stimuli transcriptionally regulates the expression of several lncRNAs. LincRNA-p21 interacts with hnRNP K to promote gene repression and p53-mediated apoptosis. DINO stabilizes p53 protein in order to mediate and amplify p53-response. PR-lncRNA1 and PR-lncRNA-10 inhibit cell survival and promote apoptosis. NEAT1 forms nuclear paraspeckles and regulate gene expression to achieve context-specific tumour suppression or proliferation. PANDA exert pro-survival functions by sequestering transcription factor NF-YA away from the promoter of pro-apoptotic genes and recruits SAFA/PRC2 complex on senescence-promoting genes. TUG1 can promote or inhibit cell proliferation depending on cellular context or tumour type. Human and murine orthologues of PINT have distinct functions. LED and PINCR promote p53 activity at a subset of p53 targets by enhancing recruitment of p53 at specific enhancers. LNCPRESS1 is a pluripotency-specific p53-repressed lncRNA that sequesters histone deacetylase, SIRT6 away from promoters of pluripotency genes in order to maintain stemness. NORAD maintains genomic stability by sequestering PUMILIO proteins. GUARDIN stabilizes BRCA1 and increase expression of TRF2 to maintain genomic stability.
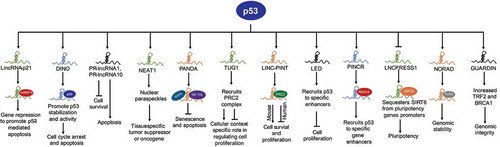
LincRNA-p21
LincRNA-p21, named such because of its proximity to the CDKN1A gene (encodes p21), is the first lncRNA characterized for its role as a core component of a p53-transcriptional network. LincRNA-p21 was identified using tiling microarrays designed to detect the expression of lncRNAs among transcripts induced by p53 in mouse embryonic fibroblasts (MEFs) in response to DNA damage, as well as with p53 restoration in KRAS/p53 mutant lung cancer cells [Citation25]. Downstream expression analysis after depletion of lincRNA-p21 revealed that a significant proportion of genes repressed by lincRNA-p21 and p53 overlap, suggesting that lincRNA-p21 participates in downstream p53-mediated transcriptional repression. Functionally, lincRNA-p21 interacts with hnRNP K (heterogeneous nuclear ribonucleoprotein K), an RNA binding and chromatin-associated factor, to repress target genes in trans and regulate p53-mediated apoptosis [Citation25] (). LincRNA-p21, by interacting with hnRNP K, SETDB1, and other chromatin modifiers, maintain repressive chromatin on pluripotency genes to inhibit reprogramming of somatic cells to induced-pluripotent cells (iPSCs), a function also blocked by p53 [Citation26]. Besides these initial findings, many studies in various experimental models reveal that, in addition to the core p53 network, lincRNA-p21 plays a variety of roles and is associated with many different types of cancers (reviewed in [Citation27]). LincRNA-p21 can also regulate genes at a post-transcriptional level: i) interacting with MDM2 to regulate p53 levels, and ii) inhibiting degradation of HIF1-α under hypoxia to regulate glycolysis, cell proliferation, and apoptosis [Citation27]. The functional significance and integration of p53-regulated lincRNA-p21 into the p53-network set the stage for subsequent identification of additional lncRNAs that play important roles in p53 regulation and tumour suppression.
DINO
DINO (Damage induced non-coding) is a conserved ~1kb mono-exonic lncRNA, transcribed divergently from the CDKN1A promoter during p53-dependent response to DNA damage [Citation28]. A majority of DINO-regulated genes contain canonical p53-binding site(s) but fail to respond to DNA damage in the absence of DINO. DINO regulates chromatin accessibility and p53 enrichment at cognate p53-target genes, such as CDKN1A, and is required for cell cycle arrest in response to DNA damage. Further, DINO physically interacts with the C-terminus of p53 and DINO depletion blocked the ability of DNA damage to induce p53 protein levels and p21 induction, suggesting that DINO is required for p53 stability in DNA damage response (). DINO and p53 co-localize at p53REs of a subset of p53-targets, including GADD45A, CDKN1A, and DDB2 [Citation28]. Mice lacking Dino are viable, fertile, and without apparent developmental defects. However, Dino-null MEFs exhibited substantially dampened induction of canonical p53-target genes [Citation28]. Thus, DINO is unique among the group of p53-regulated lncRNAs, since DINO creates a feedback loop with p53 to execute and amplify p53-response.
PANDA
PANDA (p21 associated ncRNA DNA damage activated, also known as PANDAR) is a human-specific mono-exonic lncRNA encoded within a few kilobases upstream of the CDKN1A promoter [Citation29]. PANDA is induced upon DNA damage and is involved in cell cycle progression, senescence, and apoptosis [Citation29,Citation30]. PANDA exerts anti-apoptotic functions in response to DNA damage, as depletion of PANDA sensitizes cells to DNA damage-induced apoptosis [Citation29]. Functionally, PANDA physically interacts with and sequesters NF-YA (nuclear transcription factor Y, alpha) away from promoters of a subset of pro-apoptotic p53-target genes, including CCNB1, FAS, BBC3 (PUMA protein), and PMAIP1 (NOXA protein) to inhibit cell death () [Citation29]. PANDA interacts with SAFA (Scaffold Attachment Factor A) and recruits PRC (Polycomb Repressive Complex) to repress transcription of senescence-promoting genes in order to maintain cells in a proliferative state [Citation30]. Subsequent studies reveal that PANDA is overexpressed in many tumours and may act as a biomarker for cancer diagnosis [Citation31].
LINC-PINT
LncRNA LINC-PINT (p53-induced transcript), formally known as lincMkln1, was also identified as a direct p53-transcriptional target in the same study that reported lincRNA-p21 [Citation25]. LINC-PINT is conserved between mouse and human; however, its functions are distinct. Murine linc-Pint acts as a pro-survival gene by interacting with the PRC2 complex and repressing a subset of p53-target genes to avoid an uncontrolled p53 response, whereas human LINC-PINT functions as a tumour suppressor gene that opposes cell proliferation and is downregulated in many cancers () [Citation32,Citation33]. A highly conserved sequence element in LINC-PINT mediates specific interactions with PRC2, which is necessary for LINC-PINT-dependent repression of a group of pro-invasion genes, regulated by the transcription factor EGR1 in colorectal cancer [Citation33]. LINC-PINT suppresses cell proliferation and migration of melanoma cells by recruiting EZH2, a member of the PRC2 complex, resulting in suppression of target genes [Citation34], and inhibits early-stage pancreatic ductal adenocarcinoma (PDAC) growth through activation of TGF-β pathway [Citation35]. A short 87-amino acid peptide encoded by the circular form of LINC-PINT was recently reported that directly interacts with polymerase associated factor complex (PAF1 c) and inhibits transcription elongation of multiple oncogenes and suppresses glioblastoma cell proliferation [Citation36].
GUARDIN
LncRNA GUARDIN (RP3-510D11.2–1) was identified as a direct p53-target gene by array profiling studies in TP53-null H1299 lung adenocarcinoma cells carrying an inducible wild-type p53 expression [Citation37]. GUARDIN is important for maintaining genomic integrity, since its silencing triggered apoptosis and senescence, enhanced cytotoxicity of additional genotoxic stress and inhibited cancer xenograft growth (). GUARDIN functions to regulate TRF2 a critical component of the shelterin complex through sequestering miR-23a37. GUARDIN also stabilizes breast cancer 1 (BRCA1) stability by acting as an RNA scaffold to facilitate the heterodimerization of BRCA1 and BRCA1-associated RING domain protein 1 (BARD1) [Citation37]. Thus, GUARDIN protects genomic integrity through TRF2 and BRCA1.
NEAT1
NEAT1 (Nuclear Enriched Abundant Transcript 1) was identified as a direct p53-target gene using ChIP-seq and RNA-seq in wild-type mouse embryonic fibroblasts (MEFs) treated with the DNA-damaging agent doxorubicin to activate p53 [Citation38]. NEAT1 is an essential structural component of paraspeckles, which are nuclear substructures that participate in controlling gene expression through nuclear retention of hyper-edited RNAs () [Citation39]. NEAT1 acts as both tumour suppressor and oncogene, although later functions are more pronounced (reviewed in [Citation40]). Several studies have shown that NEAT1 drives tumour initiation and progression by modulating the expression of genes involved in the regulation of tumour cell growth, epithelial-to-mesenchymal transition, stem cell-like phenotype, and chemoresistance [Citation41]. NEAT1 dampens the oncogene-dependent activation of p53, and thus engages in a negative feedback loop with p53 [Citation42]. Depletion of NEAT1 sensitizes established human cancer cells to both chemotherapy and p53 reactivation therapy [Citation42], suggesting the potential for NEAT1 to be a novel diagnostic biomarker and therapeutic target.
PVT1
PVT1 is evolutionary conserved p53-regulated lncRNA with a chromosomal location within a recognized cancer risk locus, downstream of the MYC oncogene in human, and co-amplification of PVT1 is mandatory for MYC-driven cancers [Citation43,Citation44]. PVT1 acts as an oncogene and promotes proliferation, which is in contrast to p53’s role in tumour suppression. Interestingly, p53 binds to a p53RE within intron 1 of PVT1 to induce expression of both PVT1 and miR-1204, which in contrast has anti-proliferative effects [Citation43]. How these two p53-induced ncRNAs expressed from the same locus exert distinct effects on proliferation to impact cell survival remains unknown.
In addition to the examples described above, there are many DNA damage-induced p53-lncRNA targets that are not extensively studied but play significant roles in executing p53’s response in tumour suppression ( and ). PR-lncRNA1 and PR-lncRNA-10 regulate the expression of several genes to inhibit cell survival and proliferation and promote apoptosis. PR-lncRNA1 and PR-lncRNA-10 are also required for efficient enrichment of p53 at its target gene sites, but the mechanism(s) remains unknown [Citation45]. TUG1 (Taurine Upregulated Gene 1) was initially reported as a tumour suppressor gene that limits cell proliferation, most likely by recruiting the PRC2 complex to repress expression of the HOXB7 gene [Citation46]. However, subsequent studies suggested TUG1 promotes proliferation in multiple cancer types [Citation21], thus TUG1 functions in a cell type-specific manner, a characteristic of many lncRNAs. PINCR (p53-Induced Noncoding RNA) is a direct target of p53 and induced in response to DNA damage [Citation47]. PINCR regulates a subset of p53-target genes and exerts pro-survival functions in response to DNA damage. A few tumour suppressor lncRNAs, including LED and linc-475, are produced from p53-bound enhancer regions in a p53-dependent manner and are required for p53-mediated G1 arrest [Citation24,Citation48]. A recent report proposed a negative feedback loop between p53 and oncogenic lncRNA HOTAIR in non-small cell lung cancer cells (NSCLC) [Citation49]. p53 enrichment at the promoter represses expression of HOTAIR, and overexpression of HOTAIR results in increased histone H3 lysine 27 trimethylation (H3K27me3), resulting in suppression of p53 expression [Citation49]. LNCPRESS1 (p53 Regulated and ESC Associated 1) was identified by profiling p53-targets in human embryonic stem cells (ESCs) undergoing differentiation [Citation23]. LNCPRESS1 is a pluripotency-specific transcript, which is repressed by p53 during differentiation. Mechanistically, LNCPRESS1 sequesters SIRT6, rendering it unable to repress core-pluripotency genes and maintain stemness, suggesting a novel mechanism of p53-mediated suppression of reprograming [Citation23]. In general, a majority of p53-regulated lncRNAs act as safeguards against cancer development by preventing proliferation, promoting apoptosis, activating expression of tumour suppressor genes, or maintaining genomic stability. These studies reveal that p53 regulates a wide variety of lncRNA genes, a majority of which feedback into p53-regulated networks to execute p53’s response in tumour suppression.
LncRNAs that regulate p53
The spectrum of non-coding genes that integrate with p53-transcriptional machinery continues to unfold. Fine tuning of p53 protein levels or its transcriptional activity in tumours or cells undergoing stress is critical in cellular life or death decisions. In addition to lncRNAs that are regulated by p53 and amplify p53’s response, many lncRNAs participate in the p53 network by directly or indirectly regulating p53 levels and transcriptional activity ( and ).
Figure 2. LncRNAs regulate p53 activity. Several lncRNAs, some themselves regulated by p53 modulate the p53-response by multiple mechanisms including, destabilizing p53-interactions with its negative regulator MDM2 (MEG3, PSTAR), increasing p53 levels by increased translation (MT1JP), phosphorylation of p53 (LOC572558) or direct interactions with p53 to stabilize the protein (DINO), and downregulate p53 levels via miRNAs or reduced the transcript stability (LincRNA-ROR, H19) or downregulating p53 acetylation and stabilization (MALAT1).
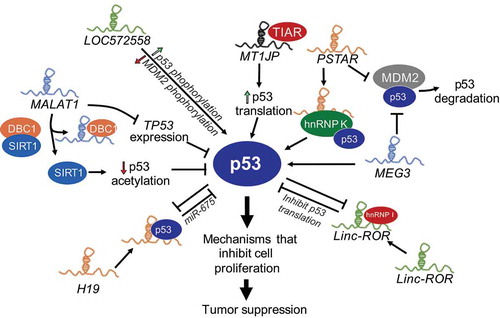
MEG3
LncRNA MEG3 (Maternally Expressed Gene 3) is downregulated in many cancers and is one of the most well-characterized tumour suppressor lncRNAs [Citation13]. Several studies demonstrated its role as a tumour suppressor gene by stimulating p53 activation [Citation50]. Mechanistically, MEG3 acts by physically interacting with p53 and disabling p53-MDM2 complexes, downregulating MDM2 expression, and thus neutralizing the inhibitory effects of MDM2 on p53 () [Citation50,Citation51]. MEG3 selectively stimulate the expression of a subset of p53-target genes by enhancing p53 binding to their promoter [Citation50]. Recently, structural and mutational analyses revealed an evolutionary conserved region of MEG3 that forms mutually exclusive pseudoknot structures that are required for proper p53-stimulation in vivo [Citation52]. How MEG3 counteracts p53-MDM2 complex and how it directs p53 to specific sites in the genome needs further investigation.
MALAT1
LncRNA MALAT1 (Metastasis Associated Lung Adenocarcinoma Transcript 1) is a highly abundant nuclear RNA overexpressed in many cancers, which correlates with hyperproliferation and metastasis [Citation53]. MALAT1 controls cell cycle progression by regulating the expression of pro-proliferative genes such as E2F1 and affecting p53 activity. Depletion of MALAT1 in normal diploid human lung fibroblasts activates DNA damage response, resulting in the activation of p53 and its target genes such as CDKN1A [Citation54]. In addition, MALAT1 expression negatively correlates with p53, since disruption of p53 by DNA oncoviruses led to strong upregulation of MALAT1 [Citation55]. A defined mechanism by which MALAT1 regulates p53 activity is not clear; however, it has been proposed that MALAT1 can repress p53 activity at both transcriptional and post-transcriptional level () [Citation56,Citation57]. MALAT1 directly represses p53 transcription by binding to the minimal MALAT1-responsive region in the P1 promoter of TP53. MALAT1 binds and competes with DBC1 to release sirtuin1 (SIRT1) and increase its deacetylation activity, which results in decreased acetylation of p53, and reduces p53-transcriptional activity [Citation57]. This intricate relationship between MALAT1 and p53 suggests the existence of a negative feedback loop between an oncogenic lncRNA and a tumour suppressor protein.
Linc-ROR
LincRNA-ROR (Regulator of Reprogramming) is a cytoplasmic lncRNA that is activated and stimulates reprogramming of differentiated cells to iPSCs [Citation58]. Further studies revealed that lincRNA-ROR negatively intervenes in p53 signalling, since its depletion led to upregulation of genes involved in p53 response, oxidative stress, and DNA damage [Citation59]. Additional evidence suggests that lincRNA-ROR is overexpressed in several cancers and is a strong negative regulator of p53 [Citation59]. Mechanistically, lincRNA-ROR binds to heterogeneous nuclear ribonucleoprotein I (hnRNP I) to interfere with hnRNP I binding to p53 mRNA resulting in repression of p53 translation (). In addition, p53 transcriptionally induces lincRNA-ROR expression to form an auto-regulatory feedback loop [Citation59], much like its negative regulator MDM2 [Citation60], suggesting a lincRNA-ROR-p53 axis that constitutes an additional surveillance mechanism for cells to counteract sustained p53 response and maintain cellular homoeostasis.
H19
H19 transcribed from imprinted loci has oncogenic properties and is deregulated in several cancers. H19 up-regulation in cells in response to stresses, such as genomic instability and hypoxia, activates a survival mode by accelerating cellular proliferation and increasing overall cellular resistance to stress [Citation61]. H19 and p53 also negatively regulate each other: p53 represses H19 expression by promoting DNA methylation of the imprinting control region upstream of H19 [Citation62] and suppressing the H19 promoter [Citation63]. H19 directly interacts with p53 to partially inactivate p53, and H19 derived miR-675 reduces p53 expression () [Citation64,Citation65]. These studies reveal a negative regulatory relationship between p53 and H19.
PSTAR
LncRNA PSTAR (p53-Stabilizing and Activating RNA) was discovered in a large-scale omics analysis of mRNA and lncRNA expression profiles in primary tissues of hepatocellular carcinoma (HCC) patients [Citation66]. PSTAR is an evolutionarily conserved lncRNA, which is significantly downregulated in tissues and cell lines derived from HCC. Low levels of PSTAR predict poor prognosis in HCC patients, especially those harbouring wild-type p53. PSTAR induces cell cycle arrest, inhibits HCC cell proliferation, and tumorigenesis. PSTAR interacts with hnRNP K, induces its SUMOylation and thus enhances hnRNP K interactions with p53, which in turn results in increased stability of p53 and trans-activation of p53-target genes (). In addition, overexpression of PSTAR inhibits MDM2-mediated p53 ubiquitination and degradation [Citation66]. PSTAR is induced in response to DNA damage such as gamma irradiation (IR) and Doxorubicin, although whether this is p53 dependent is not known. These studies reveal that PSTAR contributes to amplifying p53 response in order to achieve tumour suppression.
Conclusion
Despite decades of studies on p53’s significance in tumour suppression, the determination of pathways that negatively regulate p53 in tumours, and the downstream effectors of p53 response, there remains much to learn about the roles and regulation of p53. In recent years, a growing body of evidence, aided by the development of new technologies, has revealed that p53-regulatory networks are composed of both proteins and non-coding genes, including lncRNAs. LncRNAs are poorly conserved and, although expressed at significantly lower levels compared to the protein-coding genes, exhibit extensive mechanistic diversity, emerging as significantly relevant biological molecules [Citation20]. In fact, an explosion of studies focused on lncRNAs have shown that they represent a diverse group of ncRNAs, including both oncogenes and tumour suppressors [Citation13]. LncRNas can function either in cis or trans to regulate gene expression at all levels, epigenetic, transcriptional, and post-transcriptional. LncRNAs can assemble as flexible molecular scaffolds to foster interactions with a large variety of regulatory proteins necessary to sustain cellular activity [Citation67]. Many lncRNas are deregulated in a variety of cancers and thus can serve as diagnostic biomarkers and therapeutic targets [Citation13]. Since p53 is one of the most studied and highly mutated tumour suppressors, therefore understanding the functions of any lncRNA component in the p53 network is essential. A compendium of p53-regulated lncRNAs were recently discovered that regulate p53’s transcriptional outcomes in cellular surveillance and regulation of cellular homoeostasis. Some lncRNAs directly regulated by p53 are critical regulators of cell cycle arrest, genomic stability, DNA damage repair, and apoptosis, contributing to tumour-suppressor functions of p53 [Citation21,Citation22]. Conversely, lncRNAs not regulated by p53, intervene in p53-signalling networks to directly or indirectly control p53 protein levels and transcriptional activity. These p53 regulatory lncRNAs alter p53 functions, terminate p53-signalling, and/or maintain p53 at low levels tolerable by normal, unstressed cells. Further identification and definition of the regulatory mechanisms by which lncRNAs impinge upon p53 are needed to better understand p53’s activities and its surveillance mechanisms.
Utilizing the knowledge of p53-lncRNA networks offers an alternative to overcome the challenges of translation to clinical applications that historically focused on targeting proteins that negatively regulate p53. Molecules that either target MDM2 to restore p53 [Citation68], or that target mutant p53, either by changing its confirmation to wild-type p53 or promoting its degradation [Citation69] show some promise but none have been approved for the treatment of cancers. Since many lncRNAs that intervene in p53 signalling are deregulated in cancer, targeting oncogenic lncRNAs that suppress p53 levels in order to restore p53 functions holds considerable therapeutic promise. In fact, the development of effective therapies to silence (for oncogenes) or overexpress (for tumour suppressors) ncRNA has been an active area of investigation [Citation70]. In comparison to small chemical moieties, targeting RNA with ‘RNA-based therapeutics’ may be more facile since RNA drugs are based on nucleotide hybridization, which may increase specificity and affinity, although delivery methods could offer complications [Citation13]. If or when RNA drugs are utilized to restore p53 functions to combat cancers, is an exciting area for further research. Together, the discovery of lncRNAs has opened up a new chapter in p53 biology, one that broadens the reach of p53 networks, increases complexity in p53 regulation, and promises to revolutionize cancer drug discovery.
Acknowledgments
We thank Dr Michelle Barton for critically reading this manuscript and all current and former members of our laboratory for helpful discussions. We regret for not being able to cite all the work related to this review due to space limitations.
Disclosure statement
The author declares no conflict of interest.
References
- Bouaoun L, Sonkin D, Ardin M, et al. TP53 variations in human cancers: new lessons from the IARC TP53 database and genomics data. Hum Mutat. 2016;37(9):865–876.
- Vousden KH, Prives C. Blinded by the light: the growing complexity of p53. Cell. 2009;137(3):413–431.
- Vousden KH, Lu X. Live or let die: the cell’s response to p53. Nat Rev Cancer. 2002;2(8):594–604.
- Beckerman R, Prives C. Transcriptional regulation by p53. Cold Spring Harb Perspect Biol. 2010;2(8):a000935.
- Menendez D, Inga A, Resnick MA. The expanding universe of p53 targets. Nat Rev Cancer. 2009;9(10):724–737.
- Jain AK, Barton MC. p53: emerging roles in stem cells, development and beyond. Development. 2018;145(8). DOI:10.1242/dev.158360.
- Kruse JP, Gu W. Modes of p53 regulation. Cell. 2009;137:609–622.
- Sullivan KD, Galbraith MD, Andrysik Z, et al. Mechanisms of transcriptional regulation by p53. Cell Death Differ. 2018;25(1):133–143.
- Mattick JS, Makunin IV, Non-coding RNA. Hum Mol Genet. 2006;15. Spec No 1: R17-29. DOI: 10.1093/hmg/ddl046.
- Slack FJ. Regulatory RNAs and the demise of ‘junk’ DNA. Genome Biol. 2006;7(9):328.
- Djebali S, Davis CA, Merkel A, et al. Landscape of transcription in human cells. Nature. 2012;489(7414):101–108.
- Gerstein MB, Kundaje A, Hariharan M, et al. Architecture of the human regulatory network derived from ENCODE data. Nature. 2012;489:91–100.
- Slack FJ, Chinnaiyan AM. The role of non-coding RNAs in oncology. Cell. 2019;179(5):1033–1055.
- Iyer MK, Niknafs YS, Malik R, et al. The landscape of long noncoding RNAs in the human transcriptome. Nat Genet. 2015;47(3):199–208.
- Derrien T, Johnson R, Bussotti G, et al. The GENCODE v7 catalog of human long noncoding RNAs: analysis of their gene structure, evolution, and expression. Genome Res. 2012;22(9):1775–1789.
- Cabili MN, Trapnell C, Goff L, et al. Integrative annotation of human large intergenic noncoding RNAs reveals global properties and specific subclasses. Genes Dev. 2011;25(18):1915–1927.
- Schmitt AM, Chang HY. Long noncoding RNAs in cancer pathways. Cancer Cell. 2016;29(4):452–463.
- Rinn JL. lncRNAs: linking RNA to Chromatin. Cold Spring Harb Perspect Biol. 2014;6(8):a018614.
- Guttman M, Rinn JL. Modular regulatory principles of large non-coding RNAs. Nature. 2012;482(7385):339–346.
- Wang KC, Chang HY. Molecular mechanisms of long noncoding RNAs. Mol Cell. 2011;43(6):904–914.
- Chaudhary R, Lal A. Long noncoding RNAs in the p53 network. Wiley Interdiscip Rev RNA. 2017;8(3). DOI:10.1002/wrna.1410.
- Grossi E, Sanchez Y, Huarte M. Expanding the p53 regulatory network: lncRNAs take up the challenge. Biochim Biophys Acta. 2016;1859(1):200–208.
- Jain AK, Xi Y, McCarthy R, et al. LncPRESS1 is a p53-regulated LncRNA that safeguards pluripotency by disrupting SIRT6-mediated de-acetylation of histone H3K56. Mol Cell. 2016;64(5):967–981.
- Leveille N, Melo CA, Rooijers K, et al. Genome-wide profiling of p53-regulated enhancer RNAs uncovers a subset of enhancers controlled by a lncRNA. Nat Commun. 2015;6(1):6520.
- Huarte M, Guttman M, Feldser D, et al. A large intergenic noncoding RNA induced by p53 mediates global gene repression in the p53 response. Cell. 2010;142(3):409–419.
- Bao X, Wu H, Zhu X, et al. The p53-induced lincRNA-p21 derails somatic cell reprogramming by sustaining H3K9me3 and CpG methylation at pluripotency gene promoters. Cell Res. 2015;25(1):80–92.
- Chen S, Liang H, Yang H, et al. LincRNa-p21: function and mechanism in cancer. Med Oncol. 2017;34(5):98.
- Schmitt AM, Garcia JT, Hung T, et al. An inducible long noncoding RNA amplifies DNA damage signaling. Nat Genet. 2016;48(11):1370–1376.
- Hung T, Wang Y, Lin MF, et al. Extensive and coordinated transcription of noncoding RNAs within cell-cycle promoters. Nat Genet. 2011;43(7):621–629.
- Puvvula PK, Desetty RD, Pineau P, et al. Long noncoding RNA PANDA and scaffold-attachment-factor SAFA control senescence entry and exit. Nat Commun. 2014;5(1):5323.
- Zou Y, Zhong Y, Wu J, et al. Long non-coding PANDAR as a novel biomarker in human cancer: A systematic review. Cell Prolif. 2018;51(1):e12422. DOI:10.1111/cpr.12422.
- Marin-Bejar O, Marchese FP, Athie A, et al. Pint lincRNA connects the p53 pathway with epigenetic silencing by the polycomb repressive complex 2. Genome Biol. 2013;14(9):R104.
- Marin-Bejar O, Mas AM, Gonzalez J, et al. The human lncRNA LINC-PINT inhibits tumor cell invasion through a highly conserved sequence element. Genome Biol. 2017;18(1):202.
- Xu Y, Wang H, Li F, et al. Long Non-coding RNA LINC-PINT suppresses cell proliferation and migration of melanoma via recruiting EZH2. Front Cell Dev Biol. 2019;7:350.
- Lu H, Yang D, Zhang L, et al. Linc-pint inhibits early stage pancreatic ductal adenocarcinoma growth through TGF-beta pathway activation.. Oncol Lett. 2019;17(5):4633–4639.
- Zhang M, Zhao K, Xu X, et al. A peptide encoded by circular form of LINC-PINT suppresses oncogenic transcriptional elongation in glioblastoma. Nat Commun. 2018;9(1):4475.
- Hu WL, Jin L, Xu A, et al. GUARDIN is a p53-responsive long non-coding RNA that is essential for genomic stability. Nat Cell Biol. 2018;20(4):492–502.
- Mello SS, Sinow C, Raj N, et al. Neat1 is a p53-inducible lincRNA essential for transformation suppression. Genes Dev. 2017;31(11):1095–1108.
- Hutchinson JN, Ensminger AW, Clemson CM, et al. A screen for nuclear transcripts identifies two linked noncoding RNAs associated with SC35 splicing domains. BMC Genomics. 2007;8(1):39.
- Ghafouri-Fard S, Taheri M. Nuclear Enriched Abundant Transcript 1 (NEAT1): a long non-coding RNA with diverse functions in tumorigenesis. Biomed Pharmacother. 2019;111:51–59.
- Dong P, Xiong Y, Yue J, et al. Long non-coding RNA NEAT1: a novel target for diagnosis and therapy in human tumors. Front Genet. 2018;9:471.
- Adriaens C, Standaert L, Barra J, et al. p53 induces formation of NEAT1 lncRNA-containing paraspeckles that modulate replication stress response and chemosensitivity. Nat Med. 2016;22(8):861–868.
- Barsotti AM, Beckerman R, Laptenko O, et al. p53-dependent Induction of PVT1 and miR-1204. J Biol Chem. 2012;287(4):2509–2519.
- Tseng YY, Moriarity BS, Gong W, et al. PVT1 dependence in cancer with MYC copy-number increase. Nature. 2014;512:82–86.
- Sanchez Y, Segura V, Marin-Bejar O, et al. Genome-wide analysis of the human p53 transcriptional network unveils a lncRNA tumour suppressor signature. Nat Commun. 2014;5(1):5812.
- Zhang EB, Yin DD, Sun M, et al. P53-regulated long non-coding RNA TUG1 affects cell proliferation in human non-small cell lung cancer, partly through epigenetically regulating HOXB7 expression. Cell Death Dis. 2014;5:e1243.
- Chaudhary R, Gryder B, Woods WS, et al. Prosurvival long noncoding RNA PINCR regulates a subset of p53 targets in human colorectal cancer cells by binding to Matrin 3. Elife. 2017;6:e23244. DOI: 10.7554/eLife.23244.
- Melo CA, Leveille N, Rooijers K, et al. A p53-bound enhancer region controls a long intergenic noncoding RNA required for p53 stress response. Oncogene. 2016;35(33):4399–4406.
- Zhai N, Xia Y, Yin R, et al. A negative regulation loop of long noncoding RNA HOTAIR and p53 in non-small-cell lung cancer. Onco Targets Ther. 2016;9:5713–5720.
- Zhou Y, Zhong Y, Wang Y, et al. Activation of p53 by MEG3 non-coding RNA. J Biol Chem. 2007;282(34):24731–24742.
- Zhu J, Liu S, Ye F, et al. Long noncoding RNA MEG3 interacts with p53 protein and regulates partial p53 target genes in hepatoma cells. PLoS One. 2015;10(10):e0139790.
- Uroda T, Anastasakou E, Rossi A, et al. Conserved Pseudoknots in lncRNA MEG3 are essential for stimulation of the p53 pathway. Mol Cell. 2019;75:982–95 e9.
- Gutschner T, Hammerle M, Diederichs S. MALAT1 – a paradigm for long noncoding RNA function in cancer. J Mol Med (Berl). 2013;91:791–801.
- Tripathi V, Shen Z, Chakraborty A, et al. Long noncoding RNA MALAT1 controls cell cycle progression by regulating the expression of oncogenic transcription factor B-MYB. PLoS Genet. 2013;9(3):e1003368.
- Jeffers LK, Duan K, Ellies LG, et al. Correlation of transcription of MALAT-1, a novel noncoding RNA, with deregulated expression of tumor suppressor p53 in small DNA tumor virus models. J Cancer Ther. 2013;4(3). DOI:10.4236/jct.2013.43094.
- Tano K, Onoguchi-Mizutani R, Yeasmin F, et al. Identification of minimal p53 promoter region regulated by MALAT1 in human lung adenocarcinoma cells. Front Genet. 2017;8:208.
- Chen R, Liu Y, Zhuang H, et al. Quantitative proteomics reveals that long non-coding RNA MALAT1 interacts with DBC1 to regulate p53 acetylation. Nucleic Acids Res. 2017;45(17):9947–9959.
- Loewer S, Cabili MN, Guttman M, et al. Large intergenic non-coding RNA-RoR modulates reprogramming of human induced pluripotent stem cells. Nat Genet. 2010;42:1113–1117.
- Zhang A, Zhou N, Huang J, et al. The human long non-coding RNA-RoR is a p53 repressor in response to DNA damage. Cell Res. 2013;23(3):340–350.
- Jain AK, Barton MC. Making sense of ubiquitin ligases that regulate p53. Cancer Biol Ther. 2010;10(7):665–672.
- Raveh E, Matouk IJ, Gilon M, et al. The H19 Long non-coding RNA in cancer initiation, progression and metastasis - a proposed unifying theory. Mol Cancer. 2015;14(1):184.
- Park IY, Sohn BH, Choo JH, et al. Deregulation of DNA methyltransferases and loss of parental methylation at the insulin-like growth factor II (Igf2)/H19 loci in p53 knockout mice prior to tumor development. J Cell Biochem. 2005;94:585–596.
- Dugimont T, Montpellier C, Adriaenssens E, et al. The H19 TATA-less promoter is efficiently repressed by wild-type tumor suppressor gene product p53. Oncogene. 1998;16(18):2395–2401.
- Yang F, Bi J, Xue X, et al. Up-regulated long non-coding RNA H19 contributes to proliferation of gastric cancer cells. Febs J. 2012;279(17):3159–3165.
- Liu C, Chen Z, Fang J, et al. H19-derived miR-675 contributes to bladder cancer cell proliferation by regulating p53 activation. Tumour Biol. 2016;37(1):263–270.
- Qin G, Tu X, Li H, et al. Long noncoding RNA p53-stabilizing and activating RNA promotes p53 signaling by inhibiting heterogeneous nuclear ribonucleoprotein K deSUMOylation and suppresses hepatocellular carcinoma. Hepatology. 2020;71(1):112–129.
- Kopp F, Mendell JT. functional classification and experimental dissection of long noncoding RNAs. Cell. 2018;172(3):393–407.
- Vassilev LT, Vu BT, Graves B, et al. In vivo activation of the p53 pathway by small-molecule antagonists of MDM2. Science. 2004;303:844–848.
- Parrales A, Iwakuma T. Targeting oncogenic mutant p53 for cancer therapy. Front Oncol. 2015;5:288.
- Arun G, Diermeier SD, Spector DL. Therapeutic targeting of long non-coding RNAs in cancer. Trends Mol Med. 2018;24(3):257–277.
- Dimitrova N, Zamudio JR, Jong RM, et al. LincRNA-p21 activates p21 in cis to promote Polycomb target gene expression and to enforce the G1/S checkpoint. Mol Cell. 2014;54(5):777–790.
- Yoon JH, Abdelmohsen K, Srikantan S, et al. LincRNA-p21 suppresses target mRNA translation. Mol Cell. 2012;47(4):648–655.
- Lee S, Kopp F, Chang TC, et al. Noncoding RNA NORAD regulates genomic stability by sequestering PUMILIO proteins. Cell. 2016;164(1–2):69–80.
- Paci P, Colombo T, Farina L. Computational analysis identifies a sponge interaction network between long non-coding RNAs and messenger RNAs in human breast cancer. BMC Syst Biol. 2014;8(1):83.
- Khalil AM, Guttman M, Huarte M, et al. Many human large intergenic noncoding RNAs associate with chromatin-modifying complexes and affect gene expression. Proc Natl Acad Sci U S A. 2009;106(28):11667–11672.
- Zhang Y, He Q, Hu Z, et al. Long noncoding RNA LINP1 regulates repair of DNA double-strand breaks in triple-negative breast cancer. Nat Struct Mol Biol. 2016;23(6):522–530.
- Yang Y, Wang C, Zhao K, et al. TRMP, a p53-inducible long noncoding RNA, regulates G1/S cell cycle progression by modulating IRES-dependent p27 translation. Cell Death Dis. 2018;9(9):886.
- Sharma V, Khurana S, Kubben N, et al. A BRCA1-interacting lncRNA regulates homologous recombination. EMBO Rep. 2015;16:1520–1534.
- Matouk IJ, DeGroot N, Mezan S, et al. The H19 non-coding RNA is essential for human tumor growth. PLoS One. 2007;2(9):e845.
- Li XL, Subramanian M, Jones MF, et al. Long noncoding RNA PURPL suppresses basal p53 levels and promotes tumorigenicity in colorectal cancer. Cell Rep. 2017;20(10):2408–2423.
- Gutschner T, Hammerle M, Eissmann M, et al. The noncoding RNA MALAT1 is a critical regulator of the metastasis phenotype of lung cancer cells. Cancer Res. 2013;73(3):1180–1189.
- Ji Q, Zhang L, Liu X, et al. Long non-coding RNA MALAT1 promotes tumour growth and metastasis in colorectal cancer through binding to SFPQ and releasing oncogene PTBP2 from SFPQ/PTBP2 complex. Br J Cancer. 2014;111(4):736–748.
- Zhang X, Gejman R, Mahta A, et al. Maternally expressed gene 3, an imprinted noncoding RNA gene, is associated with meningioma pathogenesis and progression. Cancer Res. 2010;70(6):2350–2358.
- Sun L, Li Y, Yang B. Downregulated long non-coding RNA MEG3 in breast cancer regulates proliferation, migration and invasion by depending on p53’s transcriptional activity. Biochem Biophys Res Commun. 2016;478(1):323–329.
- Liu L, Yue H, Liu Q, et al. LncRNA MT1JP functions as a tumor suppressor by interacting with TIAR to modulate the p53 pathway. Oncotarget. 2016;7(13):15787–15800.
- Zhu Y, Dai B, Zhang H, et al. Long non-coding RNA LOC572558 inhibits bladder cancer cell proliferation and tumor growth by regulating the AKT-MDM2-p53 signaling axis. Cancer Lett. 2016;380:369–374.