ABSTRACT
MicroRNAs play a central role in responses to biotic stressors through their interactions with their target mRNAs. Tea plant (Camellia sinensis L.), an important beverage crop, is vulnerable to tea geometrid and anthracnose disease that causes considerable crop loss and tea production worldwide. Sustainable production of tea in the current scenario to biotic factors is major challenges. To overcome the problem of biotic stresses, high-throughput sequencing (HTS) with bioinformatics analyses has been used as an effective approach for the identification of stress-responsive miRNAs and their regulatory functions in tea plant. These stress-responsive miRNAs can be utilized for miRNA-mediated gene silencing to enhance stress tolerance in tea plant. Therefore, this review summarizes the current understanding of miRNAs regulatory functions in tea plant responding to Ectropis oblique and Colletotrichum gloeosporioides attacks for future miRNA research. Also, it highlights the utilization of miRNA-mediated gene silencing strategies for developing biotic stress-tolerant tea plant.
Introduction
Tea plant [Camellia sinensis (L.) O. Kuntze; family Theaceae] is an economically important beverage crop, native to southwestern China which is widely cultivated in the tropical and subtropical regions around the world [Citation1]. Camellia sinensis (L.) O. Kuntze (China type), Camellia assamica (Assam or India type) and Camellia assamica subsp. lasiocalyx (Planchon ex Watt.) (Cambod type) are three main worldwide natural tea plant hybrids [Citation2–Citation4]. The apical bud and two leaves immediately below the bud on the tender shoots of tea plants are processed into the most widely consumed healthy non-alcoholic beverage for its bioactive ingredients and potential beneficial health effects [Citation5]. It is well known that tea plant leaves have been recognized as a good source for producing major types of tea, including green tea (non-fermented), black tea (fully fermented) and oolong tea (semi-fermented) [Citation6]. Among these, green tea is one of the most popular and commonly consumed beverages in China, which is manufactured from young tea leaves and sold for consumption without fermentation after withering, steaming, drying and grading. It contains various biologically active components, such as catechins, caffeine, theanine, saponins, fluorine and γ-aminobutyric acid [Citation1]. Black tea is a stimulating drink produced from the leaves of the tea plant by the process of oxidation and fermentation. During black tea production, the process of oxidation and fermentation degrade the polyphenols of tea leaves and form different substances, like theaflavins, thearubigins, caffeine and proanthocyanidins [Citation7]. Oolong tea is an intermediate product between the process for green and black tea, produced by a partial oxidation of tea leaves. It is rich in caffeine, theaflavins, theanine, thearubigins and catechins [Citation8]. Altogether, the different types of tea contain polyphenols and other beneficial properties, which has therapeutic effects on health by reducing the risk of chronic diseases including cancers, cardiovascular diseases, arthritis and diabetes disease [Citation6,Citation9,Citation10].
Tea plants are plantation crops that continuously growing in the field for a very long time (>50 years) at the same site. As sessile organisms, tea plants are suffering from various biotic (living organisms) and abiotic stresses (non-living factors) under natural conditions, which affect normal growth and development, and cause considerable crop loss and tea production worldwide [Citation11,Citation12]. For biotic stress management, farmers use the agrochemicals extensively for the control of pathogens and pests, and this has led to the development of stress resistance by these biotic factors against agrochemicals. Therefore, the development of crop plants resistant to pathogens and pests with increased yields using novel alternative approaches has become the major area of focus to meet the needs of the growing population. The healthy survival of the tea plants in the same field depends on the use of different plant defence responses to detrimental biotic stresses [Citation13]. In response to biotic stress, tea plants express various functional gene product (protein) and non-protein coding regulatory molecules such as ribosomal RNA (rRNA), transfer RNA (tRNA) and small nuclear RNA (snRNA) [Citation14]. Among these, snRNAs are recently discovered small non-coding RNAs consisted of 20–24 nucleotides (nt) that control the expression of defence responsive protein-coding genes at the transcriptional and posttranscriptional levels.
In plants, small RNAs (sRNAs) are classified into two major classes, including microRNAs (miRNAs) and short interfering RNAs (siRNAs) based on their biogenesis and functions: miRNAs are derived from single-stranded stem-loop precursor structures, while siRNAs are processed from perfect double-stranded RNA transcripts [Citation15]. It is well known that miRNAs are an extensive class of small endogenous, 20–24 nucleotides-long non-coding RNA molecules that regulate gene expression at post-transcriptional levels through perfect or near-perfect complementarities with their specific messenger RNA (mRNA) targets for cleavage, degradation or translational inhibition [Citation16,Citation17]. The plant miRNAs are mainly involved in several regulatory pathways related to plant developments, metabolisms, signal transductions and stress responses [Citation18,Citation19]. The plant adaptive/defence mechanisms under different types of stress mainly depend on the defence responsive gene expression. Investigations have shown that miRNAs have emerged as important regulators that highly involved in the reprogramming of gene expression during stress condition. The manipulation of miRNAs and their target genes can be used for the development of stress-resistant cultivars by RNA interference-based strategies. RNA interference (RNAi) techniques, including hairpin gene silencing, transcriptional gene silencing, artificial miRNAs, virus-induced gene silencing (VIGS) and co-suppression, have been applied to protect several agricultural crop species from various stress factors [Citation20]. More recently, RNAi-based disease control techniques, including host-induced gene silencing (HIGS) and spray-induced gene silencing (SIGS) have been developed to assess pathogen gene function in plants using double-stranded RNAs (dsRNAs) and small RNAs (sRNAs) [Citation21,Citation22]. In this review, we outline the knowledge on miRNA biogenesis and functions in plants and describe shortly about the identified miRNAs and their targets in tea plant. Then, we summarize the current understanding of miRNAs mediated regulatory functions and adaptive/defence mechanisms in tea plant response to biotic stresses especially, insect herbivory and fungal pathogen attack in tea plants. Finally, we conclude the innovative role of miRNA-mediated gene silencing and its application for biotic stress improvement in tea plant.
Plant miRNAs biogenesis and functions
Mostly, plant miRNAs are found in the intergenic region and are transcribed from non-coding nuclear genes by RNA polymerase II into primary transcripts (pri-miRNAs). During this process, the 5′ end of pri-miRNA is capped, the 3′ end is polyadenylated and then it forms an imperfect fold-back structure [Citation23]. The pri-miRNAs are then processed into a stem-loop precursor miRNAs (Pre-miRNAs) by an enzyme complex, which includes DICER-LIKE-1 RNase III endonucleases (DCL1), double-stranded RNA (dsRNA) binding protein HYPONASTIC LEAVES1 (HYL1) and C2H2-zinc finger protein SERRATE (SE) in nuclear processing centres called D-bodies or SmD3/SmB-bodies [Citation24,Citation25]. The stem-loop-structured pre-miRNAs are further processed into miRNA-miRNA* duplex by DCL1. To prevent uridylation and exonucleases activity, the miRNA-miRNA* duplex is methylated by S-adenosyl methionine-dependent methyltransferase HUA ENHANCER 1 (HEN1) at 3′ terminal nucleotides of each strand [Citation26,Citation27]. The methylated miRNA-miRNA* duplex is exported into the cytoplasm with the help of HASTY (HST, an Exportin-5 homology protein). In cytoplasm, the methylated miRNA-miRNA* duplex is separated into miRNA (guide strand) and miRNA* (passenger strand) by the small RNA degrading nuclease (SDN) class of exonucleases [Citation26,Citation27]. The guide strand of the duplex or miRNA is next loaded into RNA induced silencing complex (RISC). The most important and key component of the miRNA-RISC complex is Arganoute (AGO1) family protein, which contains RNA binding domain (PAZ) and RNaseH-like P-element-induced wimpy testis (PIWI) domain. Once miRNAs incorporated into the cytoplasmic RISC, it will regulate their specific target gene expression either by mRNA cleavage at the 10th or 11th nucleotide from the 5′ end of the miRNA or repress the target mRNA translation [Citation28] ().
Figure 1. The model of miRNA biogenesis and functions in plants. RNA polymerase II (RNA Pol II), primary transcripts (pri-miRNAs), stem-loop precursor miRNAs (Pre-miRNAs), DICER-LIKE-1 RNase III endonucleases (DCL1), double-stranded RNA (dsRNA) binding protein, HYPONASTIC LEAVES1 (HYL1), C2H2-zinc finger protein SERRATE (SE), s-adenosyl methionine-dependent methyltransferase HUA ENHANCER 1 (HEN1), exportin-5 homology protein (HASTY), small RNA degrading nuclease (SDN) class of exonucleases, RNA induced silencing complex (RISC), argonaute family protein (AGO1).
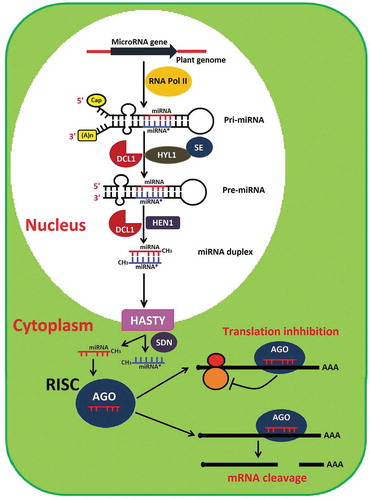
Identification of miRNAs and their target genes in tea plants
There are two approaches, including bioinformatics and experimental methods can be utilized for the identification and characterization of miRNAs and their mRNA targets in plants. The finding of homology sequence of identified known mature and precursor miRNAs from other species represents the computational approach to identify conserved miRNAs. In tea plant, for the first time, Prabu and Mandal, (2010) identified four candidate tea miRNAs from four families by EST-based comparative genomics and 30 potential target genes for 11 miRNA families by psRNATarget [Citation15]. Subsequently, using the same method, Zhu and Luo, (2013) further identified 14 new tea miRNAs and their 50 potential target genes. In addition, these predicted target genes were further analysed by gene ontology (GO) and Kyoto Encyclopedia of Genes and Genomes (KEGG) pathway for the understanding of tea miRNAs function [Citation29]. In 2012, the experimental approach has been employed for the identification of six novel sRNAs and these sRNAs were experimentally validated in young and old leaves during dormant and non-dormant growth phases [Citation17]. In recent years, highly efficient next-generation high-throughput sequencing (HTS) technology with bioinformatics analyses was employed to identify large numbers of conserved and novel miRNAs involved in the regulation of apical bud development [Citation30–Citation32], catechin biosynthesis [Citation33] and terpenoid biosynthesis [Citation34], and in responses to cold stress [Citation35], chilling and freezing stress [Citation36], drought stress [Citation37,Citation38], Ectropis oblique attack [Citation39] and Colletotrichum gloeosporioides infection [Citation40] in tea plant. In addition, several studies have investigated the expression levels of miRNAs in different tissues of tea plant [Citation5,Citation41,Citation42]. In order to identify a complex and dynamic network of tea miRNAs involved in the regulation of stress responses, growth and development, target genes of miRNA cleavage sites have been identified by degradome sequencing of mRNA combined with gene functional annotation [Citation30,Citation35–Citation40]. Most of the reported miRNAs have been found to contribute essential functions in the plant growth and development, and in the responses to biotic and abiotic stresses through the regulation of transcription factor genes. A major category of plant miRNA target genes consists of transcription factors or other regulatory proteins that function in plant development, signal transduction and stress response [Citation30–Citation32,Citation35-Citation40].
Biotic stresses
Biotic stress is mainly triggered by various living organisms, specifically bacteria, fungi, nematodes, arthropod pests and weeds. Tea plants have evolved sophisticated strategies to adapt and cope with the diverse biotic stresses, including arthropod pests and plant diseases. Some of the major tea pests, such as tea mosquito bug, red spider mites, tea geometrid and shot hole borer cause serious damage to tea plantations [Citation43]. Plant diseases are one of the most important biotic stress factors, which have direct and indirect effects on tea plant by influencing photosynthetic and transpiration rates which in turn affect the quality of tea production [Citation44]. There are 400 disease-causing pathogens have been reported from tea plant; of which, the majorities of them are fungi and a few are bacteria, viruses and algae [Citation45]. Three most common bacterial diseases are bacterial canker, bacterial shoot blight and crown gall [Citation43]. The fungal diseases are categorized into three major classes based on the plant parts affected by pathogens, such as root, stem and leaf diseases. Leaf diseases are a more major problem in tea plant because the tea plant is cultivated for its young succulent leaves. The most important foliar diseases of tea include blister blight, brown blight, grey blight, black rot, bird’s eye spot, anthracnose and red rust [Citation43,Citation46]. The study of physiological and molecular mechanisms of tea plants in response to various biotic stresses will provide a better understanding for the development of stress-tolerant varieties in the future. The tolerance of tea plants against biotic stress can be achieved by the activation of cascades of molecular networks related to stress signal perception, signal transduction, the over-expression of specific stress-responsive genes and the synthesis of metabolites [Citation47]. Apart from the development of resistant plant varieties against biotic stress by overexpression of specific stress-responsive genes, recently discovered stress-responsive miRNAs in plants are designated as effective gene regulatory small RNA molecules in various developmental pathways and are also efficiently involved in both abiotic and biotic stress responses [Citation48].
Role of tea miRNAs in response to insect herbivory
Plant–herbivore interaction is one of the most important in plant biology research. Tea is an economically important beverage crop, which is often exposed to biotic stresses from high insect populations [Citation49]. The most common insect pest of tea in China is tea geometrid (Ectropis oblique, a chewing insect), which causes severe infestation of the tea plant that leads to complete defoliation, greatly reducing tea production [Citation50,Citation51]. Generally, plant responds to insect-induced stress via both direct and indirect mechanisms. Direct defence mechanisms refer to plant characteristics that provide mechanical protection (thicker leaves, hairs, trichomes, thorns and spines) and produce toxic chemicals (terpenoids, alkaloids, anthocyanins, phenols and quinines) to kill or retard the development of herbivores [Citation52]. Indirect defences refer to the release of a blend of volatile substances that attract natural predators of the herbivores by providing food (extrafloral nectar) and shelter to predators [Citation53]. Host-derived miRNAs play important roles in regulating plant defence responses to insect herbivory. Recently, a study by Jeyaraj et al. (2017b) has shown that a total of 130 known miRNAs and 512 novel miRNAs were identified using high-throughput sequencing-based miRNAs identification approach; of these, a total of 80 conserved and 356 novel miRNAs were commonly expressed in mechanical wound (MW), geometrid attack (GA) and health control (CK) samples, whereas 18 (8 conserved and 10 novel miRNAs), 18 (4 conserved and 14 novel miRNAs) and 21 (9 conserved and 12 novel miRNAs) miRNAs were only detected in MW, GA, CK libraries, respectively [Citation39]. It is interested that 4 conserved (csn-miR169b, csn-miR827a, csn-miR1318 and csn-miR2629a) and 14 novel (csn-miRn19, csn-miRn57, csn-miRn83, csn-miRn96, csn-miRn123, csn-miRn126, csn-miRn173-5P, csn-miRn190, csn-miRn246, csn-miRn334, csn-miRn335, csn-miRn387, csn-miRn392 and csn-miRn427) miRNAs were only detected in the GA library. In addition to these miRNAs, 19 novel miRNAs were differentially expressed between GA and CK libraries, including 14 up-regulated (csn-miRn30, csn-miRn36, csn-miRn40, csn-miRn87, csn-miRn99, csn-miRn169, csn-miRn236-3P, csn-miRn287, csn-miRn301, csn-miRn381, csn-miRn396, csn-miRn406, csn-miRn421 and csn-miRn467-5P) and 5 down-regulated (csn-miRn68, csn-miRn109, csn-miRn168-5P, csn-miRn198, csn-miRn275) novel miRNAs. These identified miRNAs firmly play a critical role in the response to oral secretion (OS) induced stress in tea plant ().
Table 1. Potential target transcripts of E. oblique-induced stress-responsive miRNAs in C. sinensis reported by Jeyaraj et al. (2017b).
However, the identification of miRNA targets is essential for the analysis of the functions of miRNAs in stress response regulatory networks. The elicitors that existing in the oral secretions of Ectropis oblique can enter into tea plants during insect herbivory. These elicitors act as molecular cues for tea plant defence, which are essential for the insect recognition and receptor-mediated perception, and they deceive the invader to the tea plant’s surveillance system. The ability to recognize these elicitors and rapidly initiate defence responses is vital to the basal resistance of tea plants to this herbivory. The identification of receptors involved in the perception of elicitors and elicitor-induced defences in plant–pathogen interactions plays a major role in stress response [Citation54]. Regulation of these receptor kinases by tea miRNA and their identification is essential for defence in plant-insect interaction [Citation49]. In tea plant, Jeyaraj et al. (2017b) study had focused that the elicitors of Ectropis oblique were highly recognized by various plant receptors, like Leucine-rich repeat receptor-like protein kinase (LRR-RLKs), Cysteine-rich receptor-like protein kinase (CRR-RLKs), serine/threonine protein kinase (Ser/Thr kinase) and mitogen-activated protein kinase (MAPKs) for the activation of signal transduction pathways [Citation55,Citation56], and thereby regulate the expression of resistant genes and enhance the production of defence-related metabolites in response to OS-induced stress in tea plant [Citation57]. Also, this study has highlighted that receptors and kinase, like LRR-RLKs, CRR-RLKs, Ser/Thr kinase and MAPKs were highly regulated by OS responsive miRNAs. LRR-RLKs are targeted by three novel miRNAs (csn-miRn19, csn-miRn57 and csn-miRn421), whereas CRR-RLKs targeted by csn-miRn387 and csn-miRn421. Furthermore, Ser/Thr_kinase regulated by three novel miRNAs, including csn-miRn19, csn-miRn57 and csn-miRn335 while MAPKs regulated by csn-miRn190 [Citation39]. These tea miRNAs were potentially targeting LRR-RLKs and Ser/Thr-kinase receptors, which have further influence its biological effects by triggering Ca2+ influxes and Ca2+–binding protein activation through MAPK cascades, followed by a activation of various phytohormone synthesis and ROS biosynthesis pathways against OS-induced stresses in tea plant. Studies have shown that Ca2+-related proteins and ROS-related enzymes are involved in modulating plant defence responses against insect herbivory [Citation58,Citation59]. Notably, glutathione S-transferase (GST) and calcium-binding protein (CBP) were identified to be potential targets of csn-miRn335 and csn-miRn57, respectively [Citation39]. Altogether, these miRNAs are involved in protein kinase induced signal events, thereby triggering the miRNA-mediated defence responses against OS-induced stresses in tea plant ().
Transcription factors are proteins that involved in the process of converting genetic information from DNA to messenger RNA by binding with the promoter regions of specific genes present in DNA. Several transcription factors, namely, Nuclear transcription factor Y subunit A (NF-YAs), basic leucine zipper (bZIP) transcription factor (VIP1), basic helix-loop-helix (bHLHs) transcription factor, NAC domain transcription factor (NACs) and ethylene-responsive transcription factor (ERFs) act as major transcription factors concerned with biotic as well as abiotic stress responses across various plant species [Citation60]. It is well known that transcription factors are the predominant targets of miRNAs in both animals and plants [Citation61].
After OS-induced stress in the tea plant, it has been clearly revealed that csn-miRn19, csn-miRn301, csn-miRn392 and csn-miRn421 are significantly involved in the expression of bHLHs transcription factor [Citation39]. Transcription factor bHLH plays a versatile role in plant development and especially in plant-defence responses. These miRNAs may regulate the combinatorial interaction between MYBs and bHLHs, and thereby regulate defence-related secondary metabolite production in tea plant. This interaction controls multiple enzymatic steps in the flavonoid (anthocyanin and proanthocyanidin) biosynthesis pathway, phytochrome A (phyA) signalling and activation of abscisic acid (ABA)-inducible gene expression; it also regulates defence-related secondary metabolite production, such as glucosinolate biosynthesis [Citation62–Citation64]. These indicate that these miRNAs were likely to act serious roles in response to herbivory attack, and it is of interest to elucidate their potential biological functions against OS-induced stresses in tea plant.
ERFs are identified to be potential targets of two tea miRNAs, csn-miRn57 and csn-miRn335 after herbivory attack. Ethylene (ET) is essential for many developmental processes and act as a key mediator of biotic stress responses in plants. Expression of ERFs as downstream components of the ethylene signalling and response pathway can be induced by ethylene as well as biotic stress. It is well known that ERFs regulate molecular response to pathogen attack by binding to sequences containing AGCCGCC motifs (the GCC box), a cis-acting element and act as a key regulatory hub in plant responses to stresses [Citation65]. The crosstalk between ERFs and hormones like auxin, cytokinin, gibberellin, salicylic acid and brassinosteroid increases the stress adaptation [Citation66]. Thus, these tea miRNAs significantly act against the OS-induced stress by inducing ERF.
In addition, VIP1, NACs and NF-YAs are found to be targeted by csn-miRn36, csn-miRn57 and csn-miR169b, respectively [Citation43]. NAC genes comprise one of the largest families of plant transcription regulators that are known to play crucial roles in various developmental processes and stress responses [Citation67]. Data from various studies indicate that NAC transcription factor plays an important role in the regulation of plant defence responses to various stresses. The application of exogenous phytohormones, such as JA, SA and ET has also been shown to induce NAC genes in several species [Citation68–Citation75]. Hence, NACs can possibly modulate the phytohormonal regulation of the biotic stress cellular network for convergent and divergent adaptive pathways.
Consequently, the identified up-regulated OS-induced miRNAs significantly involved in the regulation of four main pathways, namely receptor recognition, signal perception, signal transduction and activation of transcription factors or defence genes in plant-insect interaction in tea plant to cope with this insect stress response. A hypothetical model of OS-induced miRNA regulatory pathways and their target genes in tea plant derived from this study were illustrated schematically (). The identification and characterization of these miRNAs will help uncover the molecular mechanisms of stress resistance to herbivores in the tea plant. The prospects of these present novel outcomes provide insights into miRNA-mediated regulatory mechanisms underlying the response to GA stress, and also offer valuable information for development of pest resistance using RNAi-based strategies in tea plants ().
Figure 2. The hypothetical model of regulatory networks of Ectropis oblique stress-responsive miRNA in tea plant. Leucine-rich repeat receptor-like protein kinase (LRR-RLKs), Cysteine-rich receptor-like protein kinase (CRR-RLKs), serine/threonine protein kinase (Ser/Thr kinase), glutathione S-transferase (GST), calcium-binding protein (CBP), mitogen-activated protein kinase (MAPKs), basic helix-loop-helix (bHLHs) transcription factor, ethylene-responsive transcription factor (ERFs), basic leucine zipper (bZIP) transcription factor (VIP1), NAC domain transcription factor (NACs) and Nuclear transcription factor Y subunit A (NF-YAs).
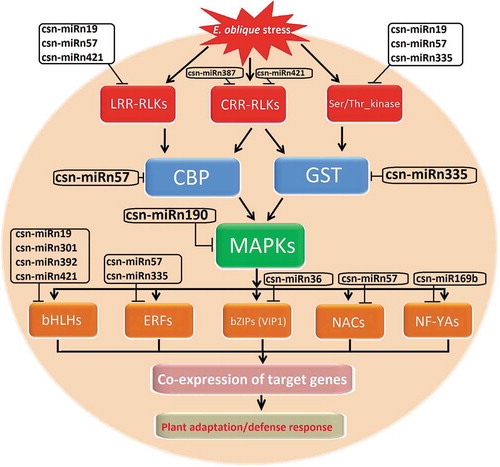
Role of tea miRNAs in response to Colletotrichum gloeosporioides
Colletotrichum gloeosporioides (C. gloeosporioides) is one of the dominant endophytic taxa of C. sinensis in worldwide. It is considered as the world’s eighth largest plant pathogen [Citation76] and widely distributed in tropical and subtropical crops [Citation77,Citation78]. C. gloeosporioides causes anthracnose disease in tea plant. This disease is very destructive and has become highly limiting for tea cultivation and the tea industry [Citation79]. It is a type of hemibiotrophic fungus, which displays biotrophic and necrotrophic phases to infect tea plant leaves [Citation80]. During the biotrophic phase, the host’s immune system and cell death are actively suppressed and allow invasive hyphae to spread throughout the infected tissues. During the necrotrophic phase, toxins secreted by the pathogen are introduced into the host cell and induce cell death [Citation40]. A study by Jeyaraj et al. (2019) identified a total of 485 conserved and 761 novel miRNAs in tea plant. Of those, 239 known and 369 novel miRNAs exhibited significantly differential expression under C. gloeosporioides stress. These C. gloeosporioides-responsive miRNAs and their targets are involved in defence response, regulation of transcription and MAPK signalling pathway in tea plant susceptible to this fungus [Citation44].
Recently, it has been found that diverse miRNAs are involved in both pathogen-associated molecular patterns to regulate various gene expressions during plant fungal infection [Citation40,Citation81,Citation82]. In regards to C. gloeosporioides infection in the tea plant, earlier this fungus enters into the biotrophic phase which eventually activates pathogen-associated molecular patterns (PAMPs) triggered immunity (PTI) in tea plant. PTI defence mechanism in plants depends on the activity of pattern-recognition receptors (PRRs), which is located on the cell surface that recognizes pathogenic organisms by interaction with pathogen-specific PAMPs (elicitors). Perception of PAMPs triggers plant resistance to most of the pathogens [Citation83]. If pathogens have evolved to counteract PTI defence system by delivering PTI interfering effector proteins into the plant cells [Citation84]; then, plant resistance initiates a second layer of innate immunity, effector-triggered immunity (ETI).
In this section, we review a study of miRNAs regulating the PTI in tea plant against of C. gloeosporioides [Citation40]. PTI is triggered by recognition of C. gloeosporioides PAMPs that activate PRRs, such as leucine-rich repeat (LRR) protein kinase, serine/threonine (Ser/Thr) protein kinase and LRR receptor-like Ser/Thr protein kinase. The phosphorylation and activation of LRR lead to activate a number of downstream signalling components, including calcium-dependent protein kinase (CDPK), mitogen-activated protein kinase (MAPK), transcription factors and defence-related genes [Citation83].
Initially, C. gloeosporioides fungus must obtain nutrients from tea plant cells and tissues and secrete limited amounts of effectors to suppress the host immune system. PTI is the first layer of innate immunity and is initiated by plants in response to pathogen infection, which utilizes receptor-like protein kinase (RLKs). RLKs play a role in the plant’s recognition of the pathogen [Citation85]. Mostly, plants use a gene-for-gene system for pathogen recognition and its resistance. These receptors are found to be involved in communication between the cell wall and the cell membrane during pathogen attack, which is probably crucial for the plant’s ability to respond to wounding or pathogen invasion [Citation86,Citation87]. Recently, Jeyaraj et al. (2019) found that the LRR protein kinase regulated by csn-miR396b-p3/p5_2ss18AT19CT (down-regulated) and csn-miR5368-p5_1ss(3/4)TC (up-regulated), Ser/Thr protein kinase targeted by csn-miR5368-p5_2ss15TC18CT, PC-3p-106557_16, and PC-3p- 70583_26 (down-regulated), and LRR receptor-like Ser/Thr protein kinase regulated by csn-miR156a-p3_1ss10TA (up-regulated) and PC-3p- 1581_1049 (down-regulated) after C. gloeosporioides infection in tea plant. Thus, these miRNAs function to target PRRs and activate the perception of the pathogen attack and thereby induce CDPK and MAPK signalling cascade pathways for PTI defence responses against C. gloeosporioides stress in tea plant [Citation40]. This receptor signalling is thought to require the formation of complexes upon ligand binding, prompting the activation of the protein kinase domain and associated proteins [Citation85,Citation88]. This mode of passing signals across the plasma membrane through signalling complexes which causes defence response and disease resistance in the plant–fungus interaction.
Protein phosphorylation, mediated by protein kinases and removed by protein phosphatases is probably the most abundant post-translational modification and plays important functions in signal transduction [Citation89,Citation90]. Numerous phosphorylation events occur upon pathogen recognition, which may play a pre-eminent role in PTI signalling [Citation91]. The contribution of MAPKs and CDPKs during stress resistance is noteworthy, they both act as critical regulators by modulating multiple defence responses in plant disease resistance [Citation92–Citation94]. One of the earliest known physiological responses to MAMP/DAMP perception is an influx of extracellular Ca2+ in the cytosol (Ca2+ burst). The extracellular calcium signals generated after pathogen attacks are transmitted to calmodulins and calmodulin-binding proteins (CBP), which receive signals from the calmodulins to activate CDPKs. Ca2+-induced CDPKs phosphorylate a large spectrum of substrates leading to ET and SA biosynthesis and transcription factors [Citation95]. Studies have clearly reported that CDPKs were targeted by two up-regulated miRNAs (csn-miR482b-p5_2ss3TA19 T and PC-3p-134668_11) and one down-regulated miRNA (csn-miR169e_R-3). These miRNAs are likely involved in calcium-activated SA biosynthesis in tea plant. CDPKs activated by these miRNAs might elevate the SA accumulation, increased expression of the defence genes which may enhance resistance to C. gloeosporioides.
After the generation of these Ca2+ signals, MAPK cascade is activated through phosphorylation. MAPKs induce notably the activation of defence genes and ET biosynthesis, which may contribute to plant immunity. Most of the MAPK substrates are transcription factors induced upon ET are possibly thought to be involved in pathogen response. The PAMP receptor LRR protein kinase and ROS production cause downstream activation by phosphorylating a complete MAPK cascade [Citation96]. Two up-regulated tea miRNAs (csn-miR6173-p3_1ss4CT and PC-5p-167506_9) are found to target MAPKs. These tea miRNAs are believed to regulate MAPK induced by Ca2+ signal events and ROS burst, thereby triggering the miRNA-mediated transcription of specific defence genes during C. gloeosporioides infection in C. sinensis [Citation40].
Several regulatory genes including transcription factors, the key regulators of plant development and plant responses to biotic and abiotic stresses are the targets of the miRNAs families. It was reported that tea miRNAs target multiple transcription factors, such as WRKY, NAC, NF-YA, ARF and MYB in response to C. gloeosporioides infection in C. sinensis. WRKY is a well-known transcription factor family in plants, which is involved in various stress response networks [Citation97]. Activated MAPK and CDPKs regulate stress responses by phosphorylation of specific MYB and WRKY transcription factors [Citation98]. During C. gloeosporioides infection, WRKY has been found to be targeted by one down-regulated novel tea miRNA (PC-5p-80764_22); NACs were targeted by two down-regulated conserved miRNAs (csn-miR164a and csn-miR164a_L + 1_1/2); NF-YAs were targeted by four up-regulated miRNAs (csn-miR169i-p3_1ss6 GA, csn-miR169e_1ss21GC, csn-miR169(a/q)_R-2 and csn-miR169x_R + 1_2ss11TC21AC) and three down-regulated miRNAs (csn-miR169 g-p3_1ss10CT, csn-miR169b and csn-miR169e_R-3); ARFs were targeted by an up-regulated miRNA (csn-miR7733-p3/p5_2ss12CT17CA) and five down-regulated miRNAs (csn-miR167k_R-3_1ss9CT, csn-miR160a, csn-miR160a_L + 1, csn-miR160b and csn-miR160c); MYBs were targeted by four up-regulated miRNAs (csn-miR828a, csn-miR858b_R-3, csn-miR858a_L-1 R + 1 and csn-miR6300-p3_1ss10AG) [Citation40]. Thus, this study implies that regulation of transcription factor by miRNAs might play important roles in regulating a wide range of molecular events in response to C. gloeosporioides infection in C. sinensis ().
Table 2. Potential target transcripts of C. gloeosporioides-induced stress-responsive miRNAs in C. sinensis reported by Jeyaraj et al. (2019).
A second layer of innate immunity, ETI was initiated after the fungi enter the necrotrophic phase. During this phase, plant cells recognize the pathogen and develop its resistance through the proteins encoded by resistance (R) genes, which are typically nucleotide-binding site leucine-rich repeat (NBS-LRR) proteins [Citation99]. Studies have shown that various miRNA families target genes encoding NBS-LRR plant innate immune receptors [Citation100,Citation101]. NBS-LRR proteins are normally associated with ETI [Citation102]. Binding of NBS-LRR protein to fungal effector molecules triggers different downstream defence signalling cascades that lead to the hypersensitivity response (HR) in plants. HR is a rapid apoptotic reaction that functions to remove the availability of cytoplasmic nutrients to pathogens in infected cells, and thereby restricts fungal growth and spread [Citation103]. In response to C. gloeosporioides infections, various down-regulated tea miRNAs, like csn-miR482b-p5_2ss9TA19AC and csn-miR3633a-3p_R-3_1ss7TA were found to target NBS-LRR [Citation40]. These tea miRNAs regulations on NBS-LRR protein may result in a mechanism for C. gloeosporioides fungal pathogen-induced expression of NBS-LRR defence-related genes. Thereby, C. gloeosporioides enter the necrotrophic phase and activate R protein-mediated ETI to cause HR cell death, which leads to effector-triggered susceptibility (ETS).
In Jeyaraj et al. (2019) study it has been reported that in addition to PTI and ETI response, C. gloeosporioides also induce classical plant defence responses, such as the accumulation of ROS and phenolic compounds [Citation40]. Rapid production of ROS in response to pathogen infection in plants causes the development of the antioxidant system through ROS-related enzymes such as peroxidase (POD), superoxide dismutase (SOD), glutathione S-transferases (GST) and ascorbate peroxidase (APX) [Citation104]. It was reported that target genes of several miRNAs were confirmed to regulate various antioxidant genes and intensely induced H2O2. Catalase isozyme1 (CAT) was targeted by three down-regulated miRNAs (csn-miR8757b-p5_1ss1TG, csn-miR396b-p3_2ss18AT19CT and csn-miR396b-p5_2ss18AT19CT); L-ascorbate peroxidase (L-APX) was targeted by two up-regulated miRNAs (csn-miR408_L-1 R + 1 and csn-miR408-p3_2ss18GT19GT); Glutathione reductase (GR) was targeted by an up-regulated miRNA (PC-3p-58653_32); Glutathione S-transferase (GST) was targeted by a down-regulated csn-miR166 g-5p_2ss5GA8GT; Cytochrome c oxidase (COX subunit V) was targeted by up-regulated csn-miR398a; Peroxidase was targeted by three conserved miRNAs (csn-miR166e-p5_2ss10GT17CT, csn-miR8005a-p5_1ss11AG and csn-miR821b-p5_2ss14TG20AT) and two novel miRNAs (PC-3p-180347_8 and PC-3p-184859_7); Phenylalanine ammonia lyase (PAL) was targeted by up-regulated miR477 family (csn-miR477 g-p5, csn-miR477 g-p5_1ss1CT and csnmiR477 g-p5_2ss1CT22CT) in tea plant against C. gloeosporioides [Citation40]. Thus, ROS scavenging system also plays an important role in C. gloeosporioides stress responses mediated by regulating the glycosylating hormones and secondary metabolites in tea plant. We speculate that during plant infection, the production and recognition of specific ligand peptides might act as pathogen effectors to facilitate the suppression of the plant’s immune system and orchestrate the reprogramming of the infected tissue so that it becomes a source of nutrients that are required by the pathogen to support its growth and development, which could contribute to the enhanced susceptibility. Thus, fungi might have adapted to these plant processes to improve the invasion of the host.
Consequently, in all these essential phases, the involvement of identified miRNA was precisely found to regulate the various target genes (), which specifically implies its crucial role in tea plant against C. gloeosporioides stress [Citation40] (). The miRNAs have potential action on targeting various genes involved in the regulation of auxin pathway, ROS scavenging pathway, SA mediated pathway, receptor kinases and transcription factors; this in turn actively intricate to various biological responses against C. gloeosporioides stress.
Figure 3. The hypothetical model of regulatory networks of Colletotrichum gloeosporioides–responsive miRNAs and their target genes in tea plant. Leucine-rich repeat protein kinase (LRR-PKs), serine/threonine protein kinase (Ser/Thr-PKs), LRR receptor-like Ser/Thr protein kinase (LRR-Ser/Thr-PKs), calcium-dependent protein kinase (CDPKs), mitogen-activated protein kinase (MAPKs), WRKY family transcription factor (WRKYs), NAC domain transcription factors (NACs), nuclear transcription factor Y (NF-YAs), auxin response factor (ARFs), Myb domain proteins (MYBs), nucleotide-binding site leucine-rich repeat (NBS-LRRs), catalases isozyme1 (CAT), L-ascorbate peroxidase (L-APX), glutathione reductase (GR), glutathione S-transferase (GST), cytochrome c oxidase (COX), peroxidase (POX), phenylalanine ammonia-lyase (PAL).
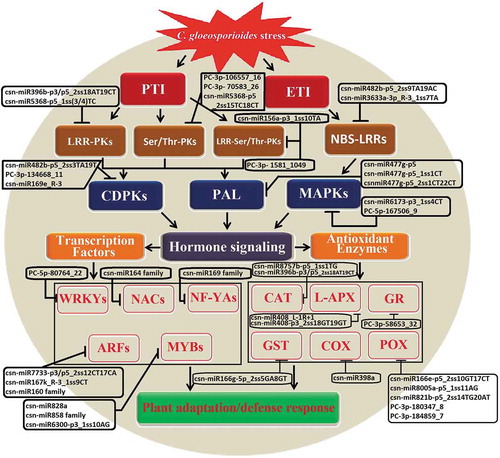
miRNA-mediated gene silencing approaches in the improvement and protection of plants
The cumulative mandate for safe approaches for crop improvement and plant defence management makes RNAi technology as a highly important and exciting field of research in plant kingdom. Generally, plant uses RNA-mediated gene silencing (RNAi) or post-transcriptional gene silencing as a mechanism of gene regulation and natural defence responses against various biotic and abiotic stresses. These mechanisms are mainly mediated by different kinds of small RNAs, among which the most widely studied are miRNAs and siRNAs. Extensive genomic studies on miRNAs effective involvement in the regulation of plant growth and development, signal transduction, protein degradation, and response to biotic and abiotic stresses made it an attractive in the field of gene silencing.
Biotic stress has become a major problem in tea plant due to constant and wild development of biotic agents, such as viruses, bacteria, fungus, insects and nematodes. Such biotic interactions evolved the tea plants in modifying stress response by expressing or regulating stress-responsive genes and transcription factors. The well-conserved miRNA biogenesis pathway is a crucial factor for proper growth and development of plants. A large number of targets and miRNAs from tea plants involved in diverse biological processes/pathways were identified in two types of biotic stresses, Ectropis oblique and C. gloeosporioides attacks. Additionally, in both studies, systemic analysis of miRNA-mRNA regulatory network from tea leaves and during time courses was done, which could provide a better understanding of gene regulatory network in stress response pathways in tea plant. Moreover, these studies also highlighted the diverse regulatory patterns of target gene expression. In addition to alteration by miRNAs, correlations between miRNAs and their target mRNAs provide an important layer of gene regulatory networks and facilitate a comprehensive understanding of biotic stress in the tea plant, and help to elucidate miRNA-mediated molecular mechanisms underlying tea plant responses to biotic stress and provide insights into the functional role of miRNAs and their targets in tea plant resistance to biotic stress.
Typically, different studies have confirmed the vital roles of miRNAs in multiple developmental as well as signalling pathways in plants. Abnormal expression patterns of these small molecules due to stress might suggest their potential use as a target for genetic improvement [Citation105]. Most recent developments of genome-wide high-throughput sequence data of many important crop species and also the emerging role in targeting regulatory genes involved in various phases of plant life cycle claimed miRNA an essential one in the transgenic area [Citation106–Citation108]. Recent advances in miRNA research brought about the idea of manipulating the specific silencing efficiency of miRNAs to silence the gene of interest. miRNA-mediated gene silencing in plants fulfills the function of positive or negative immune response regulation and/or activator of a positive or negative regulator of immune defence.
miRNA-mediated gene silencing has been employed to defend numerous agricultural crop species against numerous biotic stresses in plants [Citation109,Citation110]. One of the main miRNA-mediated gene silencing approaches is overexpression or suppression of one or more specific miRNAs, depending upon whether the target gene(s) has a desirable or an undesirable effect in the host (). Over-expressing miRNA are done by using endogenous processing machinery (DCL1) to process pri- and/or pre-form of miRNA into a mature form, which has its effect on its target gene. Studies have shown that over-expression of miRNA has resulted in undesirable traits, such as pleiotropic phenotypes. Strong over-expression impairs the fine-tuning of several biological pathways, which might be overcome by using specific promoters (eg tissue-specific, stress-induced or developmental stage-specific promoters) [Citation111–Citation113]. Over-expression of miRNAs alter specifically the expression of their target mRNAs and participated in oxidative stress response [Citation114], plant architecture [Citation115], pollen degradation [Citation116], root growth [Citation117], flower and fruit development [Citation118,Citation119]. Studies have shown that the over-expression of Osa-miR7696 confers resistance to blast infection in rice [Citation120]. Pseudomonas syringae (P. syringae) bacterial flagellin-derived peptide has found to be induced miR393 in Arabidopsis, which undesirably regulates mRNAs expressing F-box auxin receptors, causes increased resistance to P. syringae. Over-expression of miR393 reduces the plant bacterial titre by approximately five-fold, providing a tool for plant immunity to disease [Citation121]. Over-expression of miR160a directly regulates MAMP-induced callose deposition, whereas miR398b and miR773 adversely regulate MAMP-induced callose deposition and resistance to bacterial infection [Citation122]. Over-expression of two miRNAs (miR319 g and miRStv_11) in leaves of Stevia rebaudiana using Agrobacterium-mediated transient transformation method revealed that co-expression of anti-miR319 g and miRStv_11 enhanced steviol glycoside content through steviol glycosides biosynthetic pathway [Citation123]. A study by Mishra et al. (2018) showed that over-expression of can-miRn37a in susceptible variety of Capsicum annuum L induced expression of defence-related genes, and enhanced resistance to Colletotrichum truncatum by repressing ethylene response factors (ERFs) and preventing fungal growth and colonization [Citation124]. Over-expression of a set of cotton miRNAs (miR398 and miR2950) in Gossypium hirsutum var. HS6 effectively generated resistance against cotton leaf curl disease (CLCuD) by targeting multiple ORFs (genome) of cotton leaf curl multan virus (CLCuMuV) and associated beta-satellite (CLCuMB) [Citation125]. Transient over-expression of miR398b showed enhanced fungal lesions in Nicotiana benthamiana leaves that infected by Sclerotinia sclerotiorum [Citation114].
Figure 4. The general mechanism of miRNAs (A), amiRNAs (B) and target mimicry (C) in plants. RNA polymerase II (RNA Pol II), stem-loop precursor miRNAs (Pre-miRNAs), DICER-LIKE-1 RNase III endonucleases (DCL1), double-stranded RNA (dsRNA) binding protein, HYPONASTIC LEAVES1 (HYL1), s-adenosyl methionine-dependent methyltransferase HUA ENHANCER 1 (HEN1), argonaute family protein (AGO1).
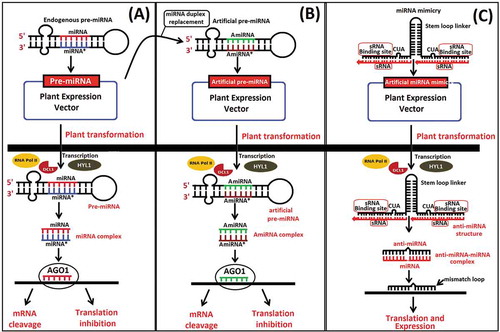
Although many genes were employed to improve plant tolerance to drought and salinity through over-expressing their expression levels using transgenic technology, the majority of these transgenic plants exhibited a very small increase and even no increase on the improvement of plant tolerance to drought and salinity stresses [Citation126,Citation127]. For instances, over-expression of Sly-miR169 confers enhanced drought tolerance in tomato by regulating nuclear factor Y subunit genes (SlNF-YA1/2/3) and one multidrug resistance-associated protein gene (SlMRP1) [Citation128], and over-expression of osa-miR396 c decreases salt and alkali stress tolerance by regulating its multiple targets [Citation129]. It was identified that over-expression of GhmiRNA157 precursor in cotton (Gossypium hirsutum) targets five SQUAMOSA promoter-binding protein-like (SPL) genes, and affect floral growth and size formation through regulating MADS-box genes and auxin signal transduction [Citation119]. Ethylene is one of the most important hormones for plant developmental processes and stress responses. Zhang et al. (2016) demonstrated that over-expression of miR319b predominantly decreased MYB33 transcript levels and enhanced ethylene-responsive root phenotype in Arabidopsis, suggesting ethylene mediated phosphorylation of cap-binding protein 20 (CBP20) is involved in root growth through the regulation of miR319b and its target MYB33 [Citation117]. Ding et al. (2016) have found that miR169 family (pag-miR169a, n and r) identified to target dormancy-specific gene, Heme Activator Protein2 (HAP2)/Nuclear factor Y-A (NF-YA) transcription factor (PagHAP2-6) in poplar (Populus alba). This study demonstrates over-expression of PagHAP2-6 in Arabidopsis and transient co-expression assays in Nicotiana benthamiana, suggesting that miR169 and its target PagHAP2-6 regulated by exogenous abscisic acid (ABA) involved in poplar cambium dormancy [Citation130]. Exogenous ABA induces miR394 expression while its target, Leaf Curling Responsiveness (LCR) expression depressed. MiR394 and LCR regulated by ABA could involve in salt and drought stress responses in Arabidopsis [Citation131].
Several studies on the over-expression of miRNA in plant development and architecture are observed. In Brassica campestris ssp. chinensis, over-expression of bra-miR158a1 and bra-miR158a2 significantly target pentatricopeptide repeat-containing protein (bra027656) and cause pollen content degradation and lead to unsound pollen intine and shrinking pollen grains [Citation116]. Yao et al. (2016) demonstrated that over-expression of miR172 in transgenic tomato (Solanum lycopersicum) alters flower and fruit development by controlling the expression of Apetala2 (AP2) gene [Citation118]. Zhu et al. (2009) reported that over-expression of miR172 causes loss of spikelet determinacy and floral organ abnormalities in rice (Oryza sativa) [Citation132]. In addition, Wang et al. (2016) also demonstrated that GIGANTEA ortholog (GmGIa) promotes gma-miR172a metabolism and represses Glyma03g3347. Over-expression of gma-miR172a in Glycine max exhibited an early flowering phenotype in transgenic lines and showed increased expression flowering-related genes [Citation133]. Shi et al. (2015) found that over-expression of Tristetraproline (TTP) affects target gene of miR160, auxin response factor (ARF17) expression and leads to male sterility in Arabidopsis [Citation134]. Over-expression of microRNA171 affects phase transitions and floral meristem determinacy in barley [Citation135]. Over-expression of sly-miR156a in tomato regulates squamosa promoter-binding protein (SBP)-box transcription factor genes and involved in multiple vegetative and reproductive trait alterations including reduced height, numerous smaller leaves and smaller fruit [Citation136]. In Oryza sativa, over-expression of OsmiR396d control plant architecture (plant height and leaf angle) by repressing the expression of growth-regulating factors, OsGRF4 and OsGRF6 though brassinosteroid (BR) gibberellin signalling pathways, respectively [Citation115]. Over-expressing a miRNA is a very tedious and time-consuming process. In this process, the plant’s own endogenous machinery has to process the miRNA biogenesis to form a mature miRNA. During this process, the production of mature miRNA level might be very less.
In addition, artificial miRNA (amiRNA) is another robust biotechnology used in plants for silencing of genes, and engineering of amiRNAs has been widely applied for the targeted down-regulation of endogenous genes in various plants (). Artificial miRNA/miRNA mimic is generally used directly as a mature form. It does not require Drosa and Dicer processing. There are few reports of the successful use of amiRNA technology for developing plants resistant to biotic stresses. For example, virus-resistant transgenic Arabidopsis [Citation114,Citation137], tobacco and tomato [Citation138–Citation141] plants have been developed using amiRNA approach. More recently, Guo et al. (2014) reported that transgenic tobacco plants expressing amiRNA showed stronger resistance against aphids. Transgenic plants targeting the acetylcholinesterase 2 transcript of the aphid Myzus persicae using an artificial miRNA show stronger resistance than plants expressing the same gene within an artificial virus-specific hairpin vector (hpRNAs) [Citation142]. Through artificial miRNA-mediated gene silencing, the invading Phytophthora infestans withdraws the amiRNAs from the host cell leading to the silencing of the Avr3a gene expression causing pathogen death and/or loss of virulence [Citation143].
Even though in recent years, the development of plant resistant to biotic stresses by amiRNA-based strategies is a more efficient technology to knock out/down the expression of specific target genes because of their higher specificity and fewer undesirable off-target effects [Citation110,Citation144]. Generally, artificial miRNA can lead to several 1000 fold induction which might not give an accurate result. Because, it can randomly bind to any mRNA at 3′-UTRs regions that contains its binding site and down-regulate that gene. On the other hand, high concentration of mimic can also interfere with the function of other miRNAs that naturally target those genes through competition with binding sites in UTR or endogenous processing machinery.
Another technique which is useful to suppress a particular miRNA is through anti-miR [short tandem target mimic (STTM)] to understand the suppression and/or activation of target mRNA (). The artificial target mimicry technology, STTM is specifically used to block or suppress the functions of specific endogenous mature miRNAs in plants [Citation145]. STTM is an artificial short (∼100 nt) non-coding RNA composed of two sRNA binding site and a spacer at the central region, which can be expressed through genetic engineering. The sRNA binding sites are complementary to target small RNAs except for the central three-nucleotide bulge [Citation146]. STTMs have been successfully used in various monocots and dicots to block miRNA functions [Citation147]. From the detail of above all studies implies that the use of these approaches like over-expressed miRNA, or anti-miRNAs or miRNA mimics in the host plant to target the specific genes involved in defence and immune response might potentially act as a useful technology for crop improvement and protection.
Conclusion and future perspectives
Enhancement of plant tolerance to biotic stress factors is essential in order to confirm quality and safe food resources. Tea research has been considered as one of the promising research areas due to its greater extent of health benefits on human. Progress in this area is also firmed by the degree to which we have identified the molecular mechanisms of stress resistance to tea plant. Recently, it has been well understand that miRNAs are considered as a key player in tea plant responses to pathogen attack, knowledge of their functional role and regulation of their expression will be able to improve crop tolerance to biotic stress factors.
The tea geometrid (E. oblique, a chewing insect) is one of the most common pests of tea in China. Generally, plants and insect interaction is a complex process [Citation148]. Plants need to defend themselves against dangerous insect herbivore while stimulating insect pollinators and beneficial insects. Applying the contrary genetics approach to individually characterize functions of the components of RNAi demonstrated a key role in the regulation of stress-responsive genes, including genes responsive to herbivory [Citation149–Citation154]. Silencing RNA-dependent RNA polymerase 1 (RDR1) in Nicotiana attenuata impairs plant’s ability to activate JA-mediated responses and makes the plant highly susceptible to insects [Citation149]. Research has shown that miRNA molecules play a critical role in the genome responses of tea plants to E. oblique. Many stress-regulated genes have been found to be regulated by miRNAs. While approaching to miRNA, foliar feeding insect E. Oblique releases its cellular contents during tissue ingestion which might trigger delivery of tea plant-derived miRNAs. Studies have shown that insect herbivore genes expressed in the insect midgut cells are important targets that have the potential to disrupt feeding or detoxification of plant-specialized metabolites or insecticides. Plant defence responses against insect herbivory are well modified through distinct receptor signalling and perception and activation of various phytohormone signalling pathways through transcription factors all these interact to produce an outcome that specifically targets the herbivore. Study shows that by regulating the expression of a particular type of miRNA molecule in plant tolerance or increase in tolerance to a well-defined stress factor may be increased or decreased. Thus, miRNA-mediated gene silencing in tea plant insects might cause the suppression of a critical insect-gene through insect feeding. Using a specific over-expressed miRNA or artificial miRNA or anti-miR can prompt the defensive gene against the E. oblique attack. Also, the herbivorous insect gene silencing efficiency can be stimulated by ingestion of transgenic miRNA producing plants that are gene-specific, which proved effective against E. oblique damage.
Plant-fungi interactions mandatory elaborate plant defence systems, including non-host resistance via physical barriers, pathogen-associated molecular pattern (PAMP)-triggered immunity (PTI), and effector-triggered immunity (ETI). Anthracnose disease caused by C. gloeosporioides is one of the most destructive fungal diseases in the tea plant. Host-induced gene silencing (HIGS) is the widely used RNAi technology against a fungal pathogen. HIGS has been emphasized in order to prevent yield losses and to cultivate strong plants. Till now, it has been showed that fungal pathogen infection is partially or completely inhibited using HIGS. The study has shown that several conserved and novel miRNAs have been identified that regulate fungal resistance to tea plant. The identification of miRNA in C. gloeosporioides infection indicated the essential regulatory roles of specific miRNAs in tea plant immunity and also in altering the expression of defence-associated genes might be devoted to plant defence or tolerance. Because of its biphasic life cycle, C. gloeosporioides is considered as a hemibiotrophic fungus with a biotrophic life phase and a necrotrophic phase in aerial of plant tissues. We strongly believe that the silencing of a specific gene involved in the pathogenicity of plant fungal pathogens by miRNA-mediated gene silencing approaches and can be transferred more easily to plants will make these genes applicable in practice. Recently, a study by Liu et al. (2019) found that csn-miR319 c and its target gene, CsnTCP2 (TEOSINTE BRANCHED/CYCLOIDEA/PROLIFERATING CELL FACTOR 2) exhibited opposite expression patterns from the quiescent bud to burst bud. Further, this study confirmed that csn-miR319 c suppresses the functions of CsnTCP2 by co-transformation experiment in a model plant, tobacco [Citation31]. Although studies have proposed that miRNA modification may play an important role in miRNA regulation of insect and fungi stress resistance in the tea plant, till now no experimental study has been performed to elucidate the function of individually modified tea plant miRNAs against this stress. Thus, functional analysis of miRNA modifications may provide new insights into plant miRNA biogenesis and ultimately may aid in generating transgenic tea plants with enhanced tolerance to biotic stress ().
Figure 5. Hypothetical model of miRNA identification and its strategies that target mRNA for the improvement of tea plant against biotic stress factors. Invading Ectropis oblique elicitor or Colletotrichum gloeosporioides pathogen can trigger the production of tea plant endogenous miRNAs that can target their respective mRNA for degradation were determined by HTS and degradome sequencing. Although tea plant-Ectropis oblique or Colletotrichum gloeosporioides derived miRNA-mediated gene silencing have not yet been discovered, we illustrate hypothetical model that identified miRNAs and target will be used to generate transgenic plants with miRNA-mediated gene silencing approaches, namely, over-expression of endogenous miRNA, artificial miRNA, miRNA mimic which will induce or suppress of the host and pathogen transcripts to generate biotic stress tolerance in tea plant.
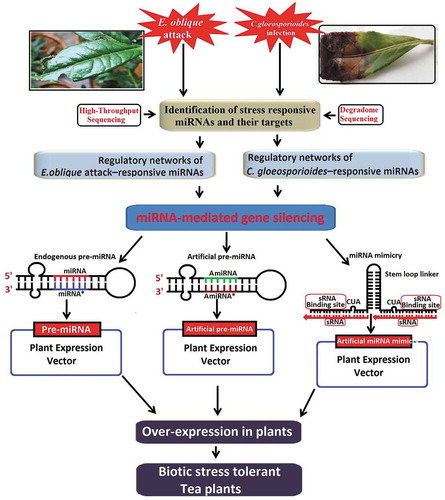
This review summarizes the complications of biotic stress in tea plants, miRNA biogenesis and functions, identification of biotic stress-responsive miRNAs in the tea plant and also explained the hypothetical model of tea miRNA-mediated target gene regulation in response to E. oblique feeding and C. gloeosporioides infection for the improvement of biotic stress tolerance. The discovery of plant miRNAs can trigger miRNA-mediated gene silencing in pathogen cells is of great interest and provides a novel layer of regulation for the interplay between plant hosts and pathogens. There are three approaches in this process: over-expression of particular miRNA to silence or decrease the specific target genes, synthesizing artificial miRNAs for silencing particular gene of interest (amiRNAs) and producing specific artificial miRNA mimics to repress the particular miRNA function, which are efficiently used for miRNA-based genetic manipulation to control gene expression and used for the interrogation of individual miRNA functions in vivo. Knowledge concerning the transportation and molecular mechanisms of miRNA-mediated gene silencing in pathogen cells is vital. Genome-wide expression analysis also revealed the precise action of miRNA, suggesting it to be an accurate gene silencing method. The transformation of targeted miRNAs into crops may provide insights into the functional role of miRNAs which can be used as an alternative to the existing approaches being used for generating stress-resistant crop plants. Finally, we conclude that these data would offer new insights for understanding the action of miRNAs and their potential to be used to engineer enhanced biotic stress tolerance in the tea plant and also these miRNAs might indirectly helpful in controlling abiotic stress as well as useful in tea plant growth and development.
Disclosure statement
No potential conflict of interest was reported by the authors.
Correction Statement
This article has been republished with minor changes. These changes do not impact the academic content of the article.
Additional information
Funding
References
- Chacko SM, Thambi PT, Kuttan R, et al. Beneficial effects of green tea: a literature review. Chin Med. 2010;5:13.
- Wight W. Tea classification revised. Curr Sci. 1962;31:298–299.
- Banerjee B. Botanical classification of tea. In: Wilson KC, Clifford MN, editors. Tea cultivation to consumption. London: Chapman and Hall; 1992. p. 25–51.
- Mondal TK. Genetics and Breeding. In: Breeding and biotechnology of tea and its wild species. New Delhi: Springer. 2014. p. 9–34.
- Jeyaraj A, Chandran V, Gajjeraman P. Differential expression of microRNAs in dormant bud of tea [Camellia sinensis (L.) O. Kuntze]. Plant Cell Rep. 2014;33(7):1053–1069.
- Khan N, Mukhtar H. Tea and health: studies in humans. Curr Pharm Des. 2013;19(34):6141–6147.
- Waugh DT, Godfrey M, Limeback H, et al. Black tea source, production, and consumption: assessment of health risks of fluoride intake in New Zealand. J Environ Public Health. 2017;2017:5120504.
- Boros K, Jedlinszki N, Csupor D. Theanine and caffeine content of infusions prepared from commercial tea samples. Pharmacogn Mag. 2016;12(45):75–79.
- Yang CS, Landau JM. Effects of tea consumption on nutrition and health. J Nutr. 2000;130(10):2409–2412.
- Negishi H, Xu JW, Ikeda K, et al. Black and green tea polyphenols attenuate blood pressure increases in stroke-prone spontaneously hypertensive rats. J Nutr. 2004;134(1):38–42.
- Mukhopadhyay M, Mondal TK, Chand PK. Biotechnological advances in tea (Camellia sinensis [L.] O. Kuntze): a review. Plant Cell Rep. 2001;35(2):255–287.
- Zhang Z, Feng X, Wang Y, et al. Advances in research on functional genes of tea plant. Gene. 2019;711:143940.
- Zhou Y, Liu Y, Wang S, et al. Molecular cloning and characterization of galactinol synthases in Camellia sinensis with different responses to biotic and abiotic stressors. J Agric Food Chem. 2017;65(13):2751–2759.
- Xia EH, Zhang HB, Sheng J, et al. The tea tree genome provides insights into tea flavor and independent evolution of caffeine biosynthesis. Mol Plant. 2017;10(6):866–877.
- Prabu GR, Mandal AK. Computational identification of miRNAs and their target genes from expressed sequence tags of tea (Camellia sinensis). Genomics Proteomics Bioinformatics. 2010;8(2):113–121.
- Unver T, Namuth-Covert DM, Budak H. Review of current methodological approaches for characterizing microRNAs in plants. Int J Plant Genomics. 2009;2009:262463.
- Mohanpuria P, Yadav SK. Characterization of novel small RNAs from tea (Camellia sinensis L.). Mol Biol Rep. 2012;39(4):3977–3986.
- Zamore PD, Haley B. Ribo-gnome: the big world of small RNAs. Science. 2005;309(5740):1519–1524.
- Chen X. Small RNAs - secrets and surprises of the genome. Plant J. 2010;61(6):941–958.
- Zheng LL, Qu LH. Application of microRNA gene resources in the improvement of agronomic traits in rice. Plant Biotechnol J. 2015;13(3):329–336.
- Wang M, Jin H. Spray-induced gene silencing: a powerful innovative strategy for crop protection. Trends Microbiol. 2017;25(1):4–6.
- Qi T, Guo J, Peng H, et al. Host-induced gene silencing: a powerful strategy to control diseases of wheat and barley. Int J Mol Sci. 2019;20(1):pii: E206.
- Voinnet O. Origin, biogenesis, and activity of plant microRNAs. Cell. 2009;136:669–687.
- Kurihara Y, Takashi Y, Watanabe Y. The interaction between DCL1 and HYL1 is important for efficient and precise processing of pri-miRNA in plant microRNA biogenesis. RNA. 2006;12:206–212.
- Fang Y, Spector DL. Identification of nuclear dicing bodies containing proteins for microRNA biogenesis in living arabidopsis plants. Curr Biol. 2007;17:818–823.
- Li J, Yang Z, Yu B, et al. Methylation protects miRNAs and siRNAs from a 3ʹ-end uridylation activity in arabidopsis. Curr Biol. 2005;15:1501–1507.
- Yang Z, Ebright YW, Yu B, et al. HEN1 recognizes 21–24 nt small RNA duplexes and deposits a methyl group onto the 2ʹ OH of the 3ʹ terminal nucleotide. Nucleic Acids Res. 2006;34:667–675.
- Jones-Rhoades MW, Bartel DP, Bartel B. MicroR3and their regulatory roles in plants. Annu Rev Plant Biol. 2006;57:19–53.
- Zhu QW, Luo YP. Identification of miRNAs and their targets in tea (Camellia sinensis). J Zhejiang Univ Sci B. 2013;14(10):916–923.
- Jeyaraj A, Zhang X, Hou Y, et al. Genome-wide identification of conserved and novel microRNAs in one bud and two tender leaves of tea plant (Camellia sinensis) by small RNA sequencing, microarray-based hybridization and genome survey scaffold sequences. BMC Plant Biol. 2017a;17(1):212.
- Liu S, Mi X, Zhang R, et al. Integrated analysis of miRNAs and their targets reveals that miR3Sc/TCP2 regulates apical bud burst in tea plant (Camellia sinensis). Planta. 2019;250(4):1111–1129.
- Zhao L, Chen C, Wang Y, et al. Conserved microRNA act boldly during sprout development and quality formation in Pingyang Tezaocha (Camellia sinensis). Front Genet. 2019;10:237.
- Sun P, Cheng C, Lin Y, et al. Combined small RNA and degradome sequencing reveals complex microRNA regulation of catechin biosynthesis in tea (Camellia sinensis). PLoS One. 2017;12(2):e0171173.
- Zhao S, Wang X, Yan X, et al. Revealing of microRNA involved regulatory gene networks on terpenoid biosynthesis in Camellia sinensis in different growing time points. J Agric Food Chem. 2018;66(47):12604–12616.
- Zhang Y, Zhu X, Chen X, et al. Identification and characterization of cold-responsive microRNAs in tea plant (Camellia sinensis) and their targets using high-throughput sequencing and degradome analysis. BMC Plant Biol. 2014;14:271.
- Zheng C, Zhao L, Wang Y, et al. Integrated RNA-Seq and sRNA-Seq analysis identifies chilling and freezing responsive key molecular players and pathways in tea plant (Camellia sinensis). PLoS One. 2015;10(4):e0125031.
- Liu SC, Xu YX, Ma JQ, et al. Small RNA and degradome profiling reveals important roles for microRNAs and their targets in tea plant response to drought stress. Physiol Plant. 2016;158(4):435–451.
- Guo Y, Zhao S, Zhu C, et al. Identification of drought-responsive miRNAs and physiological characterization of tea plant (Camellia sinensis L.) under drought stress. BMC Plant Biol. 2017;17(1):211.
- Jeyaraj A, Liu S, Zhang X, et al. Genome-wide identification of microRNAs responsive to Ectropis oblique feeding in tea plant (Camellia sinensis L.). Sci Rep. 2017b;7(1):13634.
- Jeyaraj A, Wang X, Wang S, et al. Identification of regulatory networks of microRNAs and their targets in response to Colletotrichum gloeosporioides in tea plant (Camellia sinensis L.). Front Plant Sci. 2019;10:1096.
- Hou YH, Jeyaraj A, Zhang X, et al. Absolute quantification of microRNAs in green tea (Camellia sinensis) by stem-loop quantitative real-time PCR. J Sci Food Agric. 2017;97(9):2975–2981.
- Song H, Zhang X, Shi C, et al. Selection and verification of candidate reference genes for mature microRNA expression by quantitative Rt-Pcr in the tea plant (Camellia sinensis). Genes (Basel). 2016;7(6):pii: E25.
- Borchetia S, Handique G, Roy S, et al. Genomics approaches for biotic and abiotic stress improvement in tea. In: Han WY, Li X, Ahammed G. editors. Chapter 13. Stress physiology of tea in the face of climate change. Singapore: Springer. p. 289–312.
- Ponmurugan P, Baby UI, Rajkumar R. Growth, photosynthetic and biochemical responses of tea cultivars infected with various diseases. Photosyn. 2007;45:143–146.
- Tewari A. To check the bio-efficiency of deuce 2.8 (deltamethrin) against Helopeltis theivora or more commonly known as the tea mosquito. Int J Innov Appl Sci Eng. 2016;2:31–35.
- Ponmurugan P, Manjukarunambika K, Gnanamangai BM. Impact of various foliar diseases on the biochemical, volatile and quality constituents of green and black teas. Aust Plant Pathol. 2016;45:175.
- Huang GT, Ma SL, Bai LP, et al. Signal transduction during cold, salt, and drought stresses in plants. Mol Biol Rep. 2012;39:969–987.
- Khraiwesh B, Zhu JK, Zhu J. Role of miRNAs and siRNAs in biotic and abiotic stress responses of plants. Biochim Biophys Acta. 2012;1819(2):137–148.
- Wang YN, Tang L, Hou Y, et al. Differential transcriptome analysis of leaves of tea plant (Camellia sinensis) provides comprehensive insights into the defense responses to Ectropis oblique attack using RNA-Seq. Funct Integr Genomics. 2016;16:383–398.
- Chen YG, Huang MD. Biological control progress of Ectropis oblique Prout. J Environ Entomol. 2001;4:181–184.
- Chen ZM, Sun XL, Dong WX. Genetics and chemistry of the resistance of tea plant to pests. In: Chen L, Apostolides Z, Chen Z-M, editors. Advanced topics in Science and Technology in China. Global Tea Breeding. Berlin, Heidelberg: Springer; 2012. p. 343–360.
- Hanley ME, Lamont BB, Fairbanks MM, et al. Plant structural traits and their role in antiherbivore defense. Perspec Plant Ecol Evol Syst. 2007;8:157–178.
- Arimura GI, Matsui K, Takabayashi J. Chemical and molecular ecology of herbivore-induced plant volatiles: proximate factors and their ultimate functions. Plant Cell Physiol. 2009;50:911–923.
- Nurnberger T, Kemmerling B. Receptor protein kinases-pattern recognition receptors in plant immunity. Trends Plant Sci. 2006;11:519–522.
- Artico S, Ribeiro-Alves M, Oliveira-Neto OB, et al. Transcriptome analysis of Gossypium hirsutum fower buds infested by cotton boll weevil (Anthonomus grandis) larvae. BMC Genomics. 2014;15:854.
- Hill MG, Wurms KV, Davy MW, et al. Transcriptome analysis of kiwifruit (Actinidia chinensis) bark in response to armoured scale insect (Hemiberlesia lataniae) feeding. PLoS One. 2015;10:e0141664.
- Kuhns EH, Seidl-Adams I, Tumlinson JH. Heliothine caterpillars differ in abundance of a gut lumen aminoacylase (-ACY-1)-suggesting a relationship between host preference and fatty acid amino acid conjugate metabolism. J Insect Physiol. 2012;58:408–412.
- Kiep V, Vadassery J, Lattke J, et al. Systemic cytosolic Ca2+ elevation is activated upon wounding and herbivory in Arabidopsis. New Phytol. 2015;207:996–1004.
- Pavani A, Chaitanya RK, Chauhan VK, et al. Differential oxidative stress responses in castor semilooper. Achaea janata. J Invertebr Pathol. 2015;132:157–164.
- Das A, Pramanik K, Sharma R, et al. In-silico study of biotic and abiotic stress-related transcription factor binding sites in the promoter regions of rice germin-like protein genes. PLoS One. 2019;14(2):e0211887.
- Kumar R. Role of microRNAs in biotic and abiotic stress responses in crop plants. Appl Biochem Biotechnol. 2014;174:93–115.
- Nakata M, Mitsuda N, Herde M, et al. A bHLH-type transcription factor, ABA-INDUCIBLE BHLH-TYPE TRANSCRIPTION FACTOR/JA-ASSOCIATED MYC2-LIKE1, acts as a repressor to negatively regulate jasmonate signaling in arabidopsis. Plant Cell. 2013;25:1641–1656.
- Sasaki-Sekimoto Y, Jikumaru Y, Obayashi T, et al. Basic helix-loop-helix transcription factors JASMONATE-ASSOCIATED MYC2-LIKE1 (JAM1), JAM2, and JAM3 are negative regulators of jasmonate responses in Arabidopsis. Plant Physiol. 2013;163:291–304.
- Xu W, Dubos C, Lepiniec L. Transcriptional control of flavonoid biosynthesis by MYB-bHLH-WDR complexes. Trends Plant Sci. 2015;20:176–185.
- Berrocal-Lobo M, Molina A, Solano R. Constitutive expression of ETHYLENE- RESPONSE-FACTOR1 in arabidopsis confers resistance to several necrotrophic fungi. Plant J. 2002;29:23–32.
- Chen YF, Etheridge N, Schaller GE. Ethylene signal transduction. Ann Bot (Lond). 2005;95:901–915.
- Olsen AN, Ernst HA, Leggio LL, et al. NAC transcription factors: structurally distinct, functionally diverse. Trends Plant Sci. 2005;10:79–87.
- Hegedus D, Yu M, Baldwin D, et al. Molecular characterization of Brassica napus NAC domain transcriptional activators induced in response to biotic and abiotic stress. Plant Mol Biol. 2003;53:383–397.
- Tran LS, Nakashima K, Sakuma Y, et al. Isolation and functional analysis of arabidopsis stress-inducible NAC transcription factors that bind to a drought-responsive cis-element in the early responsive to dehydration stress 1 promoter. Plant Cell. 2004;16:2481–2498.
- He XJ, Mu RL, Cao WH, et al. AtNAC2, a transcription factor downstream of ethylene and auxin signaling pathways, is involved in salt stress response and lateral root development. Plant J. 2005;44:903–916.
- Yoshii M, Shimizu T, Yamazaki M, et al. Disruption of a novel gene for a NAC-domain protein in rice confers resistance to rice dwarf virus. Plant J. 2009;57:615–625.
- Seo PJ, Kim MJ, Park JY, et al. Cold activation of a plasma membrane-tethered NAC transcription factor induces a pathogen resistance response in arabidopsis. Plant J. 2010;61:661–671.
- Xia N, Zhang G, Sun YF, et al. TaNAC8, a novel NAC transcription factor gene in wheat, responds to stripe rust pathogen infection and abiotic stresses. Physiol Mol Plant Pathol. 2010b;74:394–402.
- Nuruzzaman M, Manimekalai R, Sharoni AM, et al. Genome-wide analysis of NAC transcription factor family in rice. Gene. 2010;465:30–44.
- Nuruzzaman M, Sharoni AM, Kikuchi S. Roles of NAC transcription factors in the regulation of biotic and abiotic stress responses in plants. Front Microbiol. 2013;4:248.
- Dean R, Van Kan JAL, Pretorius ZA, et al. The top 10 fungal pathogens in molecular plant pathology. Mol Plant Pathol. 2015;16:414–430.
- Copes WE, Thomson JL. Survival analysis to determine the length of the incubation period of camellia twig blight caused by Colletotrichum gloeosporioides. Plant Dis. 2008;92:1177–1182.
- Liu F, Weir BS, Damm U, et al. Unravelling Colletotrichum species associated with Camellia: employing ApMat and GS loci to resolve species in the C. gloeosporioides complex. Persoonia. 2015;35:63–86.
- Fang WP, Yang LC, Zhu XJ, et al. Seasonal and habitat dependent variations in culturable endophytes of Camellia sinensis. J Plant Pathol Microbiol. 2013;4:169.
- Münch S, Lingner U, Floss DS, et al. The hemibiotrophic lifestyle of Colletotrichum species. J Plant Physiol. 2008;165:41–51.
- Weiberg A, Wang M, Bellinger M, et al. Small RNAs: a new paradigm in plant-microbe interactions. Annu Rev Phytopathol. 2014;52:495–516.
- Chen Y, Dong J, Bennetzen JL, et al. Integrating transcriptome and microRNA analysis identifies genes and microRNAs for AHO-induced systemic acquired resistance in N. Tabacum Sci Rep. 2017;7:12504.
- Jones JD, Dangl JL. The plant immune system. Nature. 2006;444:323–329.
- Chisholm ST, Coaker G, Day B, et al. Host microbe interactions: shaping the evolution of the plant immune response. Cell. 2006;124:803–814.
- Shiu SH, Bleecker A. Plant receptor-like kinase gene family: diversity, function and signaling. Science’s STKE. 2001a;113:re22.
- Song WY, Wang GL, Chen LL, et al. A receptor kinase-like protein encoded by the rice disease resistance gene, Xa21. Science. 1995;270:1804–1806.
- Dangl J, Jones J. Plant pathogens and integrated defence responses to infection. Nature. 2002;411:826–833.
- Shiu SH, Bleecker A. Receptor-like kinases from arabidopsis form a monophyletic gene family related to animal receptor kinases. Proc Natl Acad Sci USA. 2001b;98:10763–10768.
- Minguez P, Parca L, Diella F, et al. Deciphering a global network of functionally associated posttranslational modifications. Mol Syst Biol. 2012;8:599.
- Olsen JV, Mann M. Status of large-scale analysis of posttranslational modifications by mass spectrometry. Mol Cell Proteomics. 2013;12:3444–3452.
- Rayapuram N, Bonhomme L, Bigeard J, et al. Identification of novel PAMP triggered phosphorylation and dephosphorylation events in Arabidopsis thaliana by quantitative phosphoproteomic analysis. J Proteome Res. 2014;13:2137–2151.
- Nuhse TS, Peck SC, Hirt H, et al. Microbial elicitors induce activation and dual phosphorylation of the arabidopsis thaliana MAPK 6. J Biol Chem. 2000;275:7521–7526.
- Meng X, Xu J, He Y, et al. Phosphorylation of an ERF transcription factor by arabidopsis MPK3/MPK6 regulates plant defense gene induction and fungal resistance. Plant Cell. 2013;25:1126–1142.
- Kandoth PK, Ranf S, Pancholi SS, et al. Tomato MAPKs LeMPK1, LeMPK2, and LeMPK3 function in the systemin-mediated defense response against herbivorous insects. Proc Natl Acad Sci USA. 2007;104:12205–12210.
- Dodd AN, Kudla J, Sanders D. The language of calcium signaling. Annu Rev Plant Biol. 2010;61:593–620.
- Suarez-Rodriguez MC, Adams-Phillips L, Liu Y, et al. MEKK1 is required for flg22-induced MPK4 activation in arabidopsis plants. Plant Physiol. 2007;143:661–669.
- Banerjee A, Roychoudhury A. WRKY proteins: signaling and regulation of expression during abiotic stress responses. Sci World J. 2015;2015:807560.
- Andreasson E, Jenkins T, Brodersen P, et al. The MAP kinase substrate MKS1 is a regulator of plant defense responses. Embo J. 2005;24:2579–2589.
- Cui H, Tsuda K, Parker JE. Effector-triggered immunity: from pathogen perception to robust defense. Annu Rev Plant Biol. 2015;66:487–511.
- Zhai J, Jeong DH, De Paoli E, et al. MicroRNAs as master regulators of the plant NB-LRR defense gene family via the production of phased, trans-acting siRNAs. Genes Dev. 2011;25:2540–2553.
- Li F, Pignatta D, Bendix C, et al. MicroRNA regulation of plant innate immune receptors. Proc Natl Acad Sci USA. 2012;109:1790–1795.
- Shivaprasad PV, Chen HM, Patel K, et al. A microRNA superfamily regulates nucleotide binding site-leucine-rich repeats and other mRNAs. Plant Cell. 2012;24:859–874.
- Oren M, Barela Hudge MA ll, Golconda P, et al. Genomic instability and shared mechanisms for gene diversification in two distant immune gene families: the echinoid 185/333 and the plant NBS-LRR. In: Malagoli Deditor. The evolution of the immune system: conservation and diversification. London, UK: Elsevier-Academic Press; 2016. p. 295–310.
- Silveira PR, Nascimento KJT, Andrade CCL, et al. Physiological changes in tomato leaves arising from Xanthomonas gardneri infection. Physiol Mol Plant Pathol. 2015;92:e130–e138.
- Zhang B, Wang Q. MicroRNA-based biotechnology for plant improvement. J Cell Physiol. 2015;230:1–15.
- Sun G. MicroRNAs and their diverse functions in plants. Plant Mol Biol. 2012;80:17–36.
- Zhou M, Luo H. MicroRNA-mediated gene regulation: potential application for plant genetic engineering. Plant Mol Biol. 2013;83:59–75.
- Zhang B, Wang Q. MicroRNA, a new target for engineering new crop varieties. Bioengineered. 2016;7:7–10.
- Tiwari M, Sharma D, Trivedi PK. Artificial microRNA mediated gene silencing in plants: progress and perspectives. Plant Mol Biol. 2014;86:1–18.
- Khalid A, Zhang Q, Yasir M, et al. Small RNA based genetic engineering for plant viral resistance: application in crop protection. Front Microbiol. 2017;8:43.
- Lu Y, Feng Z, Bian L, et al. miR398 regulation in rice of the responses to abiotic and biotic stresses depends on CSD1 and CSD2 expression. Funct Plant Biol. 2010;38:44–53.
- Yang C, Li D, Mao D, et al. Over-expression of microRNA319 impacts leaf morphogenesis and leads to enhanced cold tolerance in rice (Oryza sativa L.). Plant Cell Environ. 2013;36:2207–2218.
- Niu J, Wang J, Hu H, et al. Cross‐talk between freezing response and signaling for regulatory transcriptions of MIR475b and its targets by miR475b promoter in Populus suaveolens. Sci Rep. 2016;6:1–11.
- Naya L, Paul S, Valdés-López O, et al. Regulation of copper homeostasis and biotic interactions by microRNA 398b in common bean. PLoS One. 2014;9(1):e84416.
- Tang Y, Liu H, Guo S, et al. OsmiR396d affects gibberellin and brassinosteroid signaling to regulate plant architecture in rice. Plant Physiol. 2018;176(1):946–959.
- Ma Z, Jiang J, Hu Z, et al. Over-expression of miR158 causes pollen abortion in Brassica campestris ssp. Chinensis Plant Mol Biol. 2017;93(3):313–326.
- Zhang F, Wang L, Lim JY, et al. Phosphorylation of CBP20 Links microRNA to root growth in the ethylene response. PLoS Genet. 2016;12(11):e1006437.
- Yao JL, Tomes S, Xu J, et al. How microRNA172 affects fruit growth in different species is dependent on fruit type. Plant Signal Behav. 2016;11(4):e1156833.
- Liu N, Tu L, Wang L, et al. MicroRNA 157-targeted SPL genes regulate floral organ size and ovule production in cotton. BMC Plant Biol. 2017;17(1):7.
- Campo S, Peris-Peris C, Sire C, et al. Identification of a novel microRNA (miRNA) from rice that targets an alternatively spliced transcript of the Nramp6 (Natural resistance-associated macrophage protein 6) gene involved in pathogen resistance. New Phytol. 2013;199:212–227.
- Navarro L, Dunoyer P, Jay F, et al. A plant miRNA contributes to antibacterial resistance by repressing auxin signalling. Science. 2006;312:436–439.
- Li Y, Zhang QQ, Zhang J, et al. Identification of microRNAs involved in pathogen-associated molecular pattern-triggered plant innate immunity. Plant Physiol. 2010;152:2222–2231.
- Saifi M, Yogindran S, Nasrullah N, et al. Co-expression of anti-miR319g and miRStv_11 lead to enhanced steviol glycosides content in stevia rebaudiana. BMC Plant Biol. 2019;19(1):274.
- Mishra R, Mohanty JN, Chand SK, et al. Can-miRn37a mediated suppression of ethylene response factors enhances the resistance of chilli against anthracnose pathogen Colletotrichum truncatum L. Plant Sci. 2018;267:135–147.
- Akmal M, Baig MS, Khan JA. Suppression of cotton leaf curl disease symptoms in Gossypium hirsutum through over expression of host-encoded miRNAs. J Biotechnol. 2017;263:21–29.
- Bartels D, Sunkar R. Drought and salt tolerance in plants. Crit Rev Plant Sci. 2005;24:23–58.
- Sunkar R, Li YF, Jagadeeswaran G. Functions of microRNAs in plant stress responses. Trends Plant Sci. 2012;17:196–203.
- Zhang X, Zou Z, Gong P, et al. Over-expression of microRNA169 confers enhanced drought tolerance to tomato. Biotechnol Lett. 2011;33(2):403–409.
- Gao P, Bai X, Yang L, et al. Over-expression of osa-MIR396c decreases salt and alkali stress tolerance. Planta. 2010;231(5):991–1001.
- Ding Q, Zeng J, He XQ. MiR169 and its target PagHAP2-6 regulated by ABA are involved in poplar cambium dormancy. J Plant Physiol. 2016;198:1–9.
- Song JB, Gao S, Sun D, et al. miR394 and LCR are involved in arabidopsis salt and drought stress responses in an abscisic acid-dependent manner. BMC Plant Biol. 2013;13:210.
- Zhu QH, Upadhyaya NM, Gubler F, et al. Over-expression of miR172 causes loss of spikelet determinacy and floral organ abnormalities in rice (Oryza sativa). BMC Plant Biol. 2009;9:149.
- Wang T, Sun MY, Wang XS, et al. Over-expression of GmGIa-regulated soybean miR172a confers early flowering in transgenic arabidopsis thaliana. Int J Mol Sci. 2016;17(5):pii: E645.
- Shi ZH, Zhang C, Xu XF, et al. Overexpression of AtTTP affects ARF17 expression and leads to male sterility in arabidopsis. PLoS One. 2015;10(3):e0117317.
- Curaba J, Talbot M, Li Z, et al. Over-expression of microRNA171 affects phase transitions and floral meristem determinancy in barley. BMC Plant Biol. 2013;13:6.
- Zhang X, Zou Z, Zhang J, et al. Over-expression of sly-miR156a in tomato results in multiple vegetative and reproductive trait alterations and partial phenocopy of the sft mutant. FEBS Lett. 2011;585(2):435–439.
- Duan CG, Wang CH, Fang RX, et al. Artificial microRNAs highly accessible to targets confer efficient virus resistance in plants. J Virol. 2008;82:11084e11095.
- Ai T, Zhang L, Gao Z, et al. Highly efficient virus resistance mediated by artificial microRNAs that target the suppressor of PVX and PVY in plants. Plant Biol. 2011;13:304e316.
- Kung YJ, Lin SS, Huang YL, et al. Multiple artificial microRNAs targeting conserved motifs of the replicase gene confer robust transgenic resistance to negative-sense single-stranded RNA plant virus. Mol Plant Pathol. 2012;13:303e317.
- Ali I, Amin I, Briddon RW, et al. Artificial microRNA-mediated resistance against the monopartite begomovirus cotton leaf curl burewala virus. Virol J. 2013;10:231e238.
- Vu TV, Choudhury NR, Mukherjee SK. Transgenic tomato plants expressing artificial microRNAs for silencing the pre-coat and coat proteins of a begomovirus, tomato leaf curl New Delhi virus, show tolerance to virus infection. Virus Res. 2013;172:35e45.
- Guo H, Song X, Wang G, et al. Plant generated artificial small RNAs mediated aphid resistance. PLoS One. 2014;9:e97410.
- Thakur A, Sanju S, Siddappa S, et al. Artificial microRNA mediated gene silencing of Phytophthora infestans single effector avr3a gene imparts moderate type of late blight resistance in potato. Plant Pathol J. 2015;14:1–12.
- Yogindran S, Ghosh A, Rajam MV. Artificial miRNAs for specific gene silencing and engineering virus resistance in plants. Cell Dev Biol. 2015;4:e137.
- Wang Y, Wang Z, Yang W, et al. Degradation of fungal microRNAs triggered by short tandem target mimics is via the small-RNA-degrading nuclease. Appl Environ Microbiol. 2019;85:9.
- Yan J, Gu Y, Jia X, et al. Effective small RNA destruction by the expression of a short tandem target mimic in arabidopsis. Plant Cell. 2012;24(2):415–427.
- Teotia S, Zhang D, Tang G. Knockdown of rice microRNA166 by short tandem target mimic (STTM). Methods Mol Biol. 2017;1654:337–349.
- Lucas-Barbosa D. Integrating studies on plant–pollinator and plant–herbivore interactions. Trends Plant Sci. 2016;21:125–133.
- Pandey SP, Baldwin IT. RNA-directed RNA polymerase 1 (RdR1) mediates the resistance of Nicotiana attenuata to herbivore attack in nature. Plant J. 2007;50:40–53.
- Pandey SP, Shahi P, Gase K, et al. Herbivory-induced changes in the small-RNA transcriptome and phytohormone signaling in Nicotiana attenuata. Proc Natl Acad Sci USA. 2008;105:4559–4564.
- Liu Y, Gao Q, Wu B, et al. NgRDR1, an RNA-dependent RNA polymerase isolated from Nicotiana glutinosa, was involved in biotic and abiotic stresses. Plant Physiol Biochem. 2009;47:359–368.
- Ruiz-Ferrer V, Voinnet O. Roles of plant small RNAs in biotic stress responses. Annu Rev Plant Biol. 2009;60:485–510.
- Yang H, Wang M, Gao Z, et al. Isolation of a novel RNA-dependent RNA polymerase 6 from Nicotiana glutinosa, NgRDR6, and analysis of its response to biotic and abiotic stresses. Mol Biol Rep. 2011;38:929–937.
- Bozorov TA, Pandey SP, Dinh ST, et al. DICER-like proteins and their role in plant-herbivore interactions in Nicotiana attenuata. J Integr Plant Biol. 2012;54(3):189–206.