ABSTRACT
Treatment of central nervous system (CNS) demyelination is greatly hindered by lack of the knowledge regarding to underlying molecular mechanisms as well as therapeutic agents. Here, we report a novel small molecule agent, gastrodin (GAS), which can significantly promote CNS myelination in in vivo mice models. By using high-throughput sequencing analysis, we discover a key long non-coding RNA Gm7237 that can enhance CNS myelination and is up-regulated by GAS. Through using bioinformatic analysis and experimental validations, we further unravel that microRNA-142a (miR-142a) and its target myelin gene regulatory factor (MRF) is under the direct regulation by Gm7237. Finally, we demonstrate that Gm7237/miR-142a/MRF axis is the key pathway involved in CNS myelination mediated by GAS. Overall, our results provide not only a novel agent for therapeutic treatment of CNS demyelination but also a molecular basis responsible for GAS-promoted CNS myelination.
KEYWORDS:
Introduction
Central nervous system (CNS) demyelinating diseases, such as multiple sclerosis, neuromyelitis optic and acute disseminated encephalomyelitis, affect millions of people in the worldwide [Citation1]. The major causes of CNS demyelination include genetic abnormalities and inflammatory damages [Citation2]. During the pathological process of demyelination in CNS, myelin sheaths degenerate and detach from around axons, giving rise to severe impairment in CNS structure and function [Citation3]. Although a regenerative process of remyelination spontaneously follows after CNS demyelination, remyelination normally occurs in an inadequate or failed manner [Citation4,Citation5]. Therefore, developing effective therapies for CNS demyelination and understanding the underlying myelination process to facilitate this development are urgent. Extensive efforts towards this goal have thus been made, leading to the discovery of a series of biochemical factors involved in CNS myelination [Citation2,Citation6]. At length, trial therapies using antibodies that target inhibitors of remyelination [Citation7] and stem cells that promote regeneration [Citation8] have been developed. However, these strategies are still far from meeting the standards of safety and efficacy [Citation9]. Moreover, the major challenges for this field remain not only the discovery of therapeutic drugs but also their delivery into CNS.
Small molecules hold great promise in the treatment of CNS demyelination due to their excellent ability in crossing the blood-brain barrier to reach therapeutic targets in CNS [Citation9]. Hence, high-throughput screens using small molecules or US Food and Drug Administration (FDA)-approved drugs to examine the responses of oligodendrocyte behaviour that is considered as the key stage of CNS myelination have been performed [Citation10–12]. These screens result in the discovery of several small molecules that are able to promote CNS myelination. Nevertheless, the underlying molecular mechanisms responsible for CNS myelination mediated by some of these small molecules are still elusive. In addition, given the highly complex context of CNS and exclusive targeting of oligodendrocyte by these identified small molecules, screens against other or the whole stages of myelination are also required [Citation9]. Thus, diverse small molecules that have the capability to promote CNS myelination are still highly demanded.
Gastrodin (GAS, ) is a phenolic glucoside derived from the rhizome of Gastrodia elata Blume, which is a Chinese herbal medicine plant and has been used to treat various disorders such as headache, dizziness, stoke, and amnesia for centuries[Citation13]. Since its first isolation in 1978, extensive investigations on the biological activity of GAS have been performed. As a result, GAS is found to protect cardiomyocytes from hypoxia-induced injury [Citation14] and is also able to alleviate ethanol-induced liver injury [Citation15]. Notably, in light of its ability to cross the blood-brain barrier [Citation16], GAS has also been extensively studied for its potential in treating CNS diseases including epilepsy, neurodegenerative disease, and cerebral ischaemia-reperfusion injury [Citation13,Citation17,Citation18]. Considering the lack of potential small molecules in the treatment of CNS demyelination and the broad neuroprotective activity of GAS, whether GAS can promote CNS myelination as well as what is the underlying molecular mechanism responsible for the biological activity of GAS deserves further exploration.
Long noncoding RNAs (lncRNAs) are RNA transcripts with more than 200 nucleotides but without protein-coding role [Citation19]. It has been reported that lncRNAs are universally distributed in different organs and the number of human lncRNAs exceeds that of protein-coding RNAs [Citation20,Citation21]. Therefore, lncRNAs are an important class of naturally occurring biomolecules and have attracted much attention from scientists. Plenty of studies on the discovery and function of lncRNAs have thus been performed, which reveal that lncRNAs play important regulatory roles in not only physiological processes but also pathological processes [Citation22]. One significant working mechanism for lncRNAs is that lncRNAs can function as competing endogenous RNAs (ceRNAs) to bind with endogenous microRNAs (miRNAs) [Citation23], which are short single-stranded non-coding RNAs that negatively regulate expressions of target mRNAs[Citation24]. The competitive interaction between lncRNAs and miRNAs finally alters expression levels of target mRNAs, inducing crosstalk among lncRNAs, miRNAs and target mRNAs. It is worth noting that lncRNAs are also implicated in CNS myelination. For example, the loss of lncOL-1, an oligodendrocyte-restricted lncRNA, induces remarkable defects in CNS myelination following injury [Citation25]. Another long noncoding RNA, 2700046G09Rik, is shown to regulate CNS myelination via binding to miR-23 [Citation26]. Based on these previous findings, lncRNAs are valuable targets for the treatment of CNS demyelination.
Here, we report GAS as a novel agent that can promote CNS myelination. The biological activity of GAS comes from its function in up-regulation of a lncRNA, Gm7237. Our results further indicate that Gm7237 serves as a ceRNA to bind with miR-142a and increases the expression of miR-142a target, myelin gene regulatory factor (MRF), to achieve the enhancement of myelination.
Materials and methods
In vivo animal models
All mouse protocols were conducted in accordance with the guidelines set forth by Zunyi medical university. We developed to control, demyelination, remyelination and GAS-treatment model mice by using C57/Bl/6 background mice (n = 10 for each group). All the C57/Bl/6 background mice were obtained from Model animal Research Center of Nanjing University (Nanjing, China). Demyelination model mice were developed by feeding mice with a diet containing 0.2% (w/w) cuprizone for 5 weeks. Remyelination model mice were developed by feeding the demyelination model mice with a normal diet for 4 weeks. For GAS-treatment model mice, we further fed the demyelination model mice with a diet containing 0.2% (w/w) cuprizone and daily 100 mg/kg GAS by gavage for 4 weeks.
Tissue preparation
The mice of each group were sacrificed and brain tissue was taken out. The hippocampus of the mouse brain was isolated and stored in a – 80 C refrigerator for high-throughput second-generation sequencing. Mouse brain tissue was stored in a − 80 C refrigerator for protein extraction and total RNA extraction experiments. The mouse brain tissue was fixed with 4% paraformaldehyde and the tissue was used for pathological examination experiments.
Electron microscope
The mice of each group were sacrificed and brain tissue was taken out. The hippocampus tissue of the brain tissue was separated and fixed in 1% OsO4, the tissue was dehydrated by a graded ethanol series, immersed in propylene oxide and embedded in the resin. Electron microscopy was taken to analyse different tissues.
LFB staining
The tissue of the brain fixed by paraformaldehyde was made into paraffin sections, and the tissue was dehydrated by a graded ethanol series. The solid blue dye solution was preheated in a 60 C oven for 30 min, sliced into the dye solution for 3–4 h, cooled for 10–15 min, and rinsed with water. The sections were alternately differentiated in 70% ethanol and lithium carbonate solution, and then differentiated with water, and the degree of differentiation was controlled under the microscope. Sections from the corpus callosum region of brains were observed under a Nikon inverted microscope and images were acquired.
Immunofluorescence
Cells climbing samples were placed in EDTA antigen retrieval buffer (pH 9.0) for antigen retrieval. After cooling naturally, the slides were placed in PBS (pH 7.4) and washed three times for 5 min each time on a decolorizing shaker. The PBS was added dropwise to the sections and incubated at a certain ratio (1:100) with the MBP primary antibody (Abcam, ab40390, England) overnight at 4°C. The slides were placed in PBS (pH 7.4) and washed three times on a decolorizing shaker for 5 min each time. Samples were incubated with Alexa Fluor® 488 conjucted secondary antibody (Abcam, ab150117, England) for 50 min at room temperature in the dark. The slides were placed in PBS (pH 7.4) and washed 3 times on a decolorizing shaker for 5 min each time. DAPI (Synthgene, China) was added dropwise and incubated for 10 min at room temperature. The sections were mounted with an anti-fluorescence quenching sealer. Sections were observed under a Nikon inverted fluorescence microscope and images were acquired.
Western blot analysis
To isolate the protein, tissue samples or cell samples were homogenized in 500 uL lysis buffer. Lysis buffer containing 10 mM HEPES, pH 8.0, 150 mM NaCl, 0.02% sodium azide, 0.1% SDS, 1% NP40 (Synthegene, China), 0.5% deoxycholic acid, 50 mM NaF, 0.2 mM PMSF and 1:100 dilution Protease inhibitor cocktail (Sigma, America). After homogenizing the tissue or cell sample, it was allowed to stand on ice for 30 minutes. The sample was centrifuged at 10,000 g for 10 minutes at 4°C and the supernatant was collected. Protein concentration was determined using the BCA protein assay (Synthegene, China). Loading buffer was added to the protein lysate and the sample was boiled for 5 minutes. The proteins were then labelled with the following primary antibodies at 4°C overnight: MBP (1:500, abcam, Ab40390, England), MRF (1:500, Abcam, ab213681, England) and GAPDH (1:2000, Abcam, ab181602, England). GAPDH served as a loading control and protein intensities were quantified using Image J Software.
RNA extraction and qRT-PCR
Total RNA was extracted according to the protocol of the Trizol (Life Technologies, USA) specification. cDNA was synthesized using a reverse transcription kit ((Takara Biotechnology, China)). Real-time quantitative PCR (RT-qPCR) was performed using an ABI Prism 7300 Sequence Detection System (Applied Biosystems, USA). The qRT-PCR primers used for mouse gene sequences are: MRF-F: 5ʹ-AGGTGGTGGACGAGACCGAAGC-3ʹ MRF-R: 5ʹ -GGAGGTGGCGGTGGTGGTG-3ʹ. MBP-F: 5ʹ-TCACAGAAGAGACCCTCAC-A-3ʹ; MBP-R: 5ʹ-GCCGTAGTGGGTAGTTCTTG-3ʹ; MOG-F: 5ʹ-GGGTAGTTTAGAGTGATAGGATTAAGATAT-3ʹ. MOG-R: 5ʹ-TAAAAATAAACCACCCTAAAAAAAA-3ʹ. GAPDH-F: 5ʹ-GATATTGTTGACATC-AATGAC-3ʹ; GAPDH-R: 5ʹ-TTGATTTTGGAGGGATCTCG-3ʹ. All primers are designed by primer5 software.
Northern blot
Total RNA was extracted from the hippocampus of the mouse brain. For each sample, 1 μg of RNA was used and separated on a 10% denatured TBE gel and electrophoretically transferred onto a Hybond-NC membrane. Hybridization was carried out overnight at 50 C using a predesigned ncRNA Gm7237 probe (DIG-labelled DNA oligonucleotide probe). After wash, the NC membrane was further incubated with the anti-DIG antibody, and finally, the nucleic acid was visualized using the DIG luminescence detection kit. U6 was used as an internal reference control.
Cell culture and Transfection
Isolation and culture of primary OPCs: Primary OPCs were isolated from the cerebral cortex of neonatal mice using differential separation. After removal of microglia and astrocytes by shaking and differential adherence, cultured in OPC growth medium (10 ng/ml PDGFAA and 20 ng/ml bFGF) for 7–10 days, bipolar or tris Extreme OPC cell morphology. The medium of multi-polarity was found in the cells by changing the medium of differentiation medium (15 nM/T3, 10 ng/ml ciliary neurotrophic factor) for 2–3 days. The functional verification part of this experiment is the growth medium. The cell-level drug concentration of gastrodin in the different treatment groups of cells was: 50 uM.
Transfection reagents (Invitrogen, USA) Lipofectamine 2000 was used according to the manufacturer’s protocol. 50 nM miRNA mimics/anti-miRNAs and 0.75 μg/ml plasmid were transfected into cells in six well plates containing 2 mL medium.
Luciferase reporter assay
The 3ʹ-untranslated region (3ʹ-UTR) sequence of MRF was obtained from the NCBI database. The binding sequence of MRF and lncRNA Gm7237 that interacted with miR-142a was obtained by BiBiServ2–RNAhybrid website. The entire 3ʹ-UTR of MRF and lncRNA Gm7237 containing the predicated target sites for miR-142a were synthesized by Synthgene (China). To investigate the binding specificity, the binding sequences that interacted with miR-142a were mutated and mutant MRF 3ʹ-UTRs/lncRNA Gm7237 were then inserted into an equivalent luciferase reporter plasmid. In order to perform the luciferase reporter assay, the cells were plated in 24-well plates and 1 µg luciferase reporter plasmid and 1 µg β-galactosidase plasmid (internal control) were transfected by using Lipofectamine 2000. After 4 h, the cells were further transfected with 100 pmole of miR-142a mimics, anti-miR-142a using Lipofectamine 2000. The luciferase activity was measured using a Dual-Luciferase® Reporter Assay System in accordance with the manufacturer’s protocol (Promega Corporation, Madison, WI, USA).
RNA pulldown
LncRNA Gm7237 was transcribed from the pGEM-TlncRNA Gm7237 vector in vitro. Like the pGEM-T transcribed transcript (used as a negative control), lncRNA Gm7237 was biotinylated with biotin RNA. Labelling kits (Roche, Switzerland) and T7 RNA polymerase (Roche, Switzerland) were treated with RNase-free DNase I (Roche, Switzerland) and purified using the RNeasy Mini Kit (Qiagen, USA). Scramble sequence with 3ʹ-biotin was used as a negative control. 1 mg of whole cell lysate from OPC cells was incubated with 3 micrograms of purified biotinylated transcript for 1 hour at 25°C. The complex was isolated by streptavidin agarose beads (Invitrogen, USA). Any miRNA present in the pulldown material is detected by real-time PCR analysis.
Immunoprecipitation
OPCs were transfected with miR-142a. After 48 hours, RIP experiments were performed using anti-Ago2 or anti-IgG antibody (Millipore, USA) and Magna RIPTM RNA binding protein immunoprecipitation kit (Millipore, USA). RNA was isolated from the immunoprecipitated product and the amount of mRNA expressed therein was detected by real-time PCR analysis.
RNA-seq and data analysis
We isolated RNA in the hippocampus of the brain of demyelination, remyelination with GAS-treatment model mice and placed the sample in RNA-seq. RNA-seq libraries were prepared using the Illumina RNA-seq Preparation Kit (Illumina) and sequenced on a HiSeq 2000 sequencer. RNA integrity numbers for RNA quality assessment were passed through an Agilent RNA 6000 Nano kit (Agilent 2100 Bioanalyzer system, Agilent Technologies, Inc.). Sequencing coverage depth is ~20 million readings per sample. Map the RNA-seq readings using TopHat using the default settings (http://tophat.cbcb.umd.edu). The TopHat output data were then analysed by Cufflinks to calculate a fragment of the per thousand base transcript/million map reading (FPKM) value of the known transcript in the mouse genome reference. Heat maps of gene differential expression were generated using Gene cluster and Java Treeview. Use DAVID for gene ontology functional classification (http://david.abcc.ncifcrf.gov) and Ingenuity Pathway analysis (Supported by Synthgene, China).
Statistical analysis
The results are expressed as the mean ± standard deviation of the mean of three independent experiments. Comparisons were determined using Student’s t-test and a p < 0.05 was considered statistically significant.
Results
GAS is able to promote CNS myelination
To investigate whether GAS can enhance CNS myelination, we developed demyelination, remyelination and GAS-treatment model mice. Demyelination model mice were developed by feeding mice with a diet containing 0.2% (w/w) cuprizone for 5 weeks [Citation27]. Remyelination model mice were developed by feeding the demyelination model mice with a normal diet for 4 weeks [Citation10,Citation27]. For GAS-treatment model mice, we further fed the demyelination model mice with a diet containing 0.2% (w/w) cuprizone and daily 100 mg/kg GAS by gavage for 4 weeks. To evaluate the myelination, we harvested hippocampus of brain tissues from these model mice. Electron microscopy (EM) was first used to observe myelin surrounding spinal cords. As shown in , comparing with that in the control group, significant degeneration of myelin was observed in demyelination group and remyelination happened after cuprizone withdrawal but in an inadequate manner. In contrast, GAS remarkably promoted myelination (), which demonstrated our initial hypothesis that GAS possesses the potential for the treatment of CNS demyelination. We then stained these tissues with Luxol fast blue (LFB) (), showing consistent results with EM images (). To verify these results, we also measured the expression levels of myelin proteins, including myelin basic protein (MBP) and myelin oligodendrocyte glycoprotein (MOG) [Citation10,Citation28], in these dissected tissues. As shown in , a dramatic decrease in protein levels of MBP and MOG was detected in demyelination group, which confirmed successful demyelination by cuprizone. In comparison, levels of these myelin proteins increased after remyelination and GAS significantly restored their expressions. In addition, we have also verified the mRNA expression levels of MBP and MOG via RT-qPCR in Figure S1, and the results of MBP and MOG were consistent at the mRNA level and protein level. Taken together, these results indicate that GAS can promote CNS myelination.
Figure 2. GAS promotes CNS myelination. (A, B) (A) Electron microscopy (EM) images of spinal cords isolated from control, demyelination, remyelination and GAS-treatment model mice. Scale bar: 5 µm. (B) Quantification. (C, D) (C) Luxol fast blue (LFB) staining of hippocampus of brain tissues isolated from control, demyelination, remyelination and GAS-treatment model mice. Scale bar: 100 µm. (D) Quantification. (E, F) (E) Western blotting analysis of MBP and MOG in hippocampus of brain tissues isolated from control, demyelination, remyelination and GAS-treatment model mice. (F) Quantification. Scale bar: 100 µm. Data are shown as mean ± SEM (n = 3). *P< 0.05
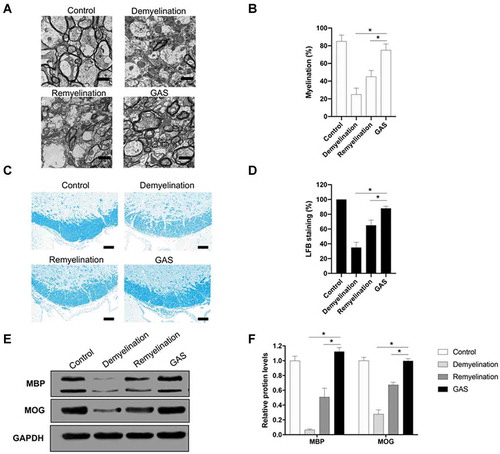
LncRNA Gm7237 is up-regulated by GAS
Since lncRNAs are extensively involved in CNS myelination and also potential therapeutic targets for the treatment of CNS demyelination [Citation25,Citation26], we next tried to check whether GAS promotes CNS myelination via modulating lncRNA. We isolated total RNA from the dissected hippocampus of brain tissues that were derived from demyelination and GAS-treatment model mice. The isolated RNA samples were further analysed by next-generation sequencing (Supplementary data). In total, 34 lncRNAs were found to be differentially expressed in brain tissues derived from demyelination and GAS-treatment model mice, of which 11 were up-regulated and 23 were down-regulated. The expression patters of lncRNAs were analysed by hierarchical clustering (). Through verifying expression levels of significantly regulated lncRNAs in these dissected tissues via real-time quantitative PCR (RT-qPCR), we found that a lncRNA Gm7237 significantly increased in the GAS group (). We also used the northern blotting analysis method to confirm the expressions of Gm7237 in the dissected hippocampus of brain tissues that were isolated from control, demyelination, remyelination and GAS-treatment model mice. As shown in , Gm7237 markedly decrease in demyelination group, whereas GAS significantly enhanced its expression. These results demonstrate that GAS can up-regulate lncRNA Gm7237 in CNS and suggest that Gm7237 is a potential target for GAS to promote CNS myelination.
Figure 3. LncRNA Gm7237 is up-regulated by GAS. (A) Hierarchical clustering analysis of lncRNAs that were differentially expressed in hippocampus of brain tissues isolated from demyelination and GAS-treatment model mice. (B) RT-qPCR analysis of up-regulated lncRNAs. (C, D) (C) Northern blotting analysis of Gm7237 in hippocampus of brain tissues isolated from control, demyelination, remyelination and GAS-treatment model mice. U6 served as internal control. (D) Quantification. Data are shown as mean ± SEM (n = 3). *P< 0.05
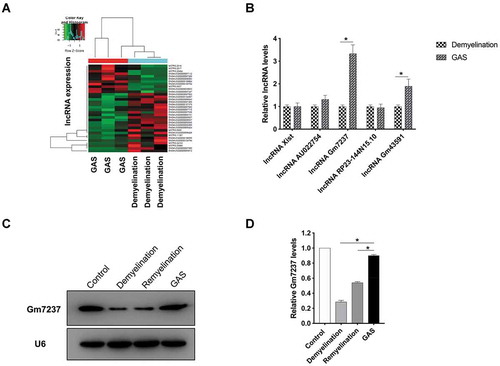
LncRNA Gm7237 is a positive regulator of myelination
Next, we continued to examine the role of Gm7237 in CNS myelination. For this goal, we isolated and cultured oligodendrocyte precursor cells (OPCs) for further assays. First, we used the Cy3-labelled fluorescence probe to determine the location of Gm7237 in OPCs via fluorescence in-situ hybridization (FISH) analysis. As shown in , Gm7237 mainly located in the cytoplasm, where lncRNAs can bind to and regulate mature miRNAs[Citation19]. To evaluate the function of Gm7237, we designed a plasmid to over-express Gm7237 and a short-interfering RNA (siRNA) to knock down Gm7237. After transfection of the over-expression plasmid or siRNA into OPCs, we measured the expression levels of Gm7237 in OPCs through RT-qPCR. confirmed successful over-expression or down-regulation of Gm7237 in OPCs. We then measured the expressions of myelin protein, MBP[Citation28], in OPCs following over-expression or down-regulation of Gm7237. We observed that overexpression of Gm7237 significantly enhanced the expression of MBP whereas knockdown of Gm7237 markedly decreased the expression of MBP (). Immunofluorescence staining of MBP using MBP antibody confirmed the Western blotting results (). These results suggest that the lncRNA, Gm7237, can promote CNS myelination. Since GAS was able to promote CNS myelination () and up-regulate Gm7237 (), we wondered whether GAS promoted CNS myelination through modulating Gm7237. To test this hypothesis, we respectively or simultaneously treated OPCs with Gm7237 siRNA and GAS. The expression levels of MBP in treated OPCs were then determined, showing that GAS increased the expression of MBP while Gm7237 siRNA decreased the expression of MBP (). These results coincided well with previous data. However, upon knockdown of Gm7237, the activity of GAS in up-regulation of MBP decreased (), suggesting that GAS promotes CNS myelination via Gm7237. We also confirmed these results through immunofluorescence staining of MBP (). Taken together, Gm7237 is a positive regulator of CNS myelination and a key biomolecule involved in CNS myelination promoted by GAS.
Figure 4. LncRNA Gm7237 is a positive regulator of CNS myelination. (A) Localization of Gm7237 in OPCs through FISH analysis. Cy3-labelled probes targeting Gm7237 or 18S were used. Hoechst was used to stain nucleus. (B) RT-qPCR analysis of Gm7237 levels in OPCs after transfection with Gm7237 plasmid or Gm7237 siRNA. (C, D) (C) Western blotting analysis of MBP protein levels in OPCs after transfection with Gm7237 plasmid or Gm7237 siRNA. GAPDH served as internal control. (D) Quantification. (E) Immunofluorescence staining of MBP in OPCs after transfection with Gm7237 plasmid or Gm7237 siRNA. DAPI was used to stain nucleus. (F, G) (F) Western blotting analysis of MBP protein levels in OPCs after treatment with GAS, transfection with Gm7237 siRNA, or co-treatment with GAS and Gm7237 siRNA. (G) Quantification. (H) Immunofluorescence staining of MBP in OPCs after treatment with GAS, transfection with Gm7237 siRNA, or co-treatment with GAS and Gm7237 siRNA. DAPI was used to stain nucleus. Scale bar: 20 µm. Data are shown as mean ± SEM (n = 3). *P< 0.05
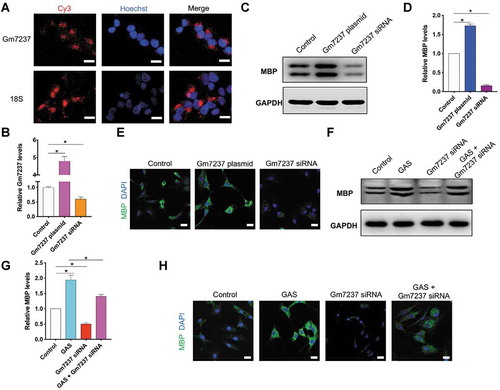
MiR-142 is a direct target of lncRNA Gm7237
As one significant function of lncRNAs is their inhibition of endogenous miRNAs via a ceRNA mechanism [Citation19,Citation29], we thus predicted the downstream targets of Gm7237, including miRNAs and mRNAs (), through an mRNA-ceRNA analysis. To verify the bioinformatic analysis results and find out which miRNA is the direct target of Gm7237, we used 3ʹ-biotinylated Gm7237 as the probe to pull down interacted miRNA ()[Citation30]. Briefly, 3ʹ-biotinylated Gm7237 was prepared and incubated with cell lysis of OPCs, followed by pull-down of Gm7237/miRNAs via streptavidin beads. We then measured the levels of predicted miRNAs in the pull-down samples via RT-qPCR. We used scramble RNA (nc) with 3ʹ-biotin as the control probe. As shown in , miR-142a was significantly enriched in the pull-down samples, indicating that miR-142a may be a direct target of Gm7237. To verify this, we compared the expression levels of miR-142a in OPCs after over-expression or down-regulation of Gm7237 using the same method described above. The over-expression of Gm7237 could obviously decrease miR-142a levels while the down-regulation of Gm7237 markedly increased miR-142a levels (). In order to determine whether Gm7237 regulates miR-142a through direct binding, we inserted Gm7237 into the 3ʹ-untranslated region (3ʹ-UTR) of the luciferase reporter gene (). After co-transfection of the engineered luciferase gene and miR-142a into OPCs, miR-142a could recognize and bind with the 3ʹ-UTR of luciferase and blocked its expression in a typical miRNA-mediated gene regulation manner[Citation31]. Therefore, we could simply tell the interaction between miR-142a and Gm7237 through measuring luciferase signals. As expected, miR-142a significantly repressed the expression of luciferase, whereas antisense miR-142a (anti-miR-142a) markedly increased luciferase signals (). When we used a mutated sequence of Gm7237 to engineer luciferase reporter gene and performed the same experiment, we found that miR-142a could not regulate luciferase expression (). These results demonstrate that Gm7237 can modulate miR-142a expression and miR-142a is a direct target of Gm7237.
Figure 5. The direct target of Gm7237 is miR-142a. (A) mRNA-ceRNA analysis of Gm7237 targets. (B) Schematic illustration of using biotinylated Gm7237 to pull down miRNA targets for Gm7237. (C) RT-qPCR analysis of miRNA levels in pull-down samples that were cell lysis of OPCs transfected with biotinylated nc probe or biotinylated Gm7237 probe and enriched by streptavidin magnetic beads. (D) RT-qPCR analysis of miR-142a levels in OPCs after transfection with Gm7237 plasmid or Gm7237 siRNA. (E) Design of luciferase reporter gene containing Gm7237 or mutated Gm7237. (F) Relative luciferase signals from OPCs that were transfected with luciferase gene for Gm7237 or mutated Gm7237 and miR-142a or anti-miR-142a. Data are shown as mean ± SEM (n = 3). *P< 0.05
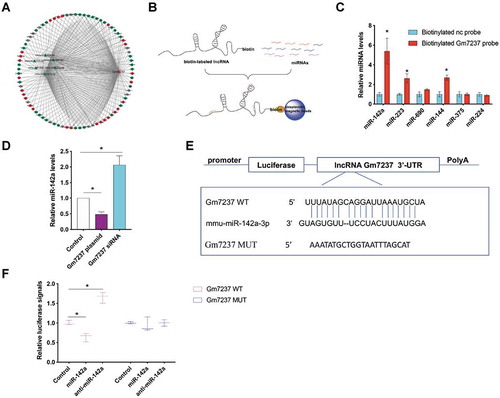
The direct mRNA target of miR-142a is MRF
To screen the target mRNAs of miR-142a (), we used immunoprecipitation method to pull down potential miR-142a targets[Citation32]. In brief, Argonaute 2 (AGO2) antibody was used to pull down the RNA-induced silencing complex (RISC) [Citation33,Citation34], which contained miR-142a and its target mRNAs (). We then verified the predicted target mRNAs by using RT-qPCR and the results were shown in . IgG antibody was used as a negative control. Among the predicted targets, MRF, which is a critical transcriptional regulator for CNS myelination [Citation35,Citation36], was markedly enriched in the pull-down samples, indicating that MRF may be a direct target of miR-142a. To demonstrate the direct interaction between miR-142a and MRF, we inserted the 3ʹ-UTR of MRF into the 3ʹ-UTR of the luciferase reporter gene (). Upon co-transfection of engineered luciferase reporter gene and miR-142a mimic or antisense miR-142a (anti-miR-142a) into OPCs, over-expression of miR-142a significantly suppressed luciferase expression while down-regulation of miR-142a by anti-miR-142a increased luciferase expression (). In contrast, when we mutated the binding region of miR-142a in the engineered luciferase reporter gene (), miR-142a could not regulate luciferase expression (). We also measured the endogenous MRF protein levels in OPCs post over-expression or knockdown of miR-142 by using miR-142a mimic or anti-miR-142a (). To validate whether the miR-142a/MRF axis involved in CNS myelination, we also evaluated the protein level of myelin protein, MBP, in OPCs post over-expression or knockdown of miR-142. We found that over-expression of miR-142a decreased the MBP expression while down-regulation of miR-142a significantly enhanced MBP expression. When we simultaneously over-expressed miR-142a and MRF by respectively using a miR-142a mimic and a plasmid expressing MRF in OPCs, we observed that the down-regulation of MBP by miR-142 could be alleviated by over-expression of MRF (). These results demonstrate that MRF is the direct target of miR-142a in OPCs and the miR-142a/MRF pathway directly involves in CNS myelination.
Figure 6. MRF is the direct target of miR-142a. (A) Schematic illustration of immunoprecipitation methods to capture RISC. (B) RT-qPCR analysis of target mRNAs in pull-down samples that were cell lysis of OPCs purified by IgG or AGO2 antibody. (C) Design of luciferase reporter gene containing 3ʹ-UTR of MRF or mutated 3ʹ-UTR of MRF. (D) Relative luciferase signals from OPCs that were transfected with luciferase gene containing 3ʹ-UTR of MRF or mutated 3ʹ-UTR of MRF and miR-142a or anti-miR-142a. (E, F) (E) Western blotting analysis of MRF and MBP protein levels in OPCs that were transfected with miR-142, anti-miR-142a or miR-142a plus MRF plasmid. (F) Quantification. Data are shown as mean ± SEM (n = 3). *P< 0.05
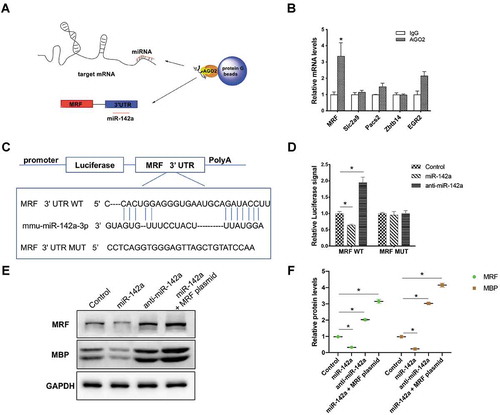
GAS promotes myelination through a lncRNA Gm7237/miR-142a/MRF pathway
Till now, we have demonstrated that GAS could promote CNS myelination via Gm7237 and miR-142a/MRF that involves in CNS myelination was the downstream pathway of Gm7237. Finally, we validated whether GAS promoted CNS myelination through the Gm7237/miR-142a/MRF pathway. We checked the expression levels of miR-142a and MRF in the dissected hippocampus of brain tissues that were isolated from control, demyelination, remyelination and GAS-treatment model mice. RT-qPCR analysis results showed that miR-142a significantly decreased and MRF markedly up-regulated in brain tissues of mice treated with GAS (). Together with the evidences that GAS could up-regulate Gm7237 level and Gm7237 directly regulates miR-142a/MRF (), these data support that GAS can regulate the Gm7237/miR-142/MRF axis. To determine the key roles of Gm7237/miR-142/MRF pathway in CNS myelination promoted by GAS, we treated OPCs with GAS, Gm7237 over-expression plasmid, Gm7237 over-expression plasmid plus miR-142a mimic, GAS plus miR-142 mimic, or GAS with Gm7237 over-expression plasmid plus miR-142a mimic. The protein levels of MRF and MBP were then measured, which were shown in . As expected, GAS and Gm7237 could up-regulate MRF and MBP protein levels. While simultaneous overexpression of miR-142a neutralized the effect of GAS or Gm7237. Immunostaining of MBP in treated OPCs confirmed these results (). Collectively, these results validate that GAS promotes CNS myelination via the Gm7237/miR-142a/MRF pathway.
Figure 7. GAS promotes CNS myelination via the Gm7237/miR-142a/MRF pathway. (A, B) RT-qPCR analysis of (A) miR-142a and (B) MRF levels in hippocampus of brain tissues isolated from control, demyelination, remyelination and GAS-treatment model mice. (C, D) Western blotting analysis of MRF and MBP protein levels in OPCs that were treated with GAS, or transfected with Gm7237 plasmid, or co-treated with Gm7237 plasmid plus miR-142a, or co-treated with GAS plus miR-142a, or co-treated with GAS plus Gm7237 plasmid plus miR-142a. (D) Quantification. (E) Immunostaining of MBP in OPCs that were treated with GAS, or transfected with Gm7237 plasmid, or co-treated with Gm7237 plasmid plus miR-142a, or co-treated with GAS plus miR-142a, or co-treated with GAS plus Gm7237 plasmid plus miR-142a. DAPI was used to stain nucleus. Scale bar: 20 µm. Data are shown as mean ± SEM (n = 3). *P< 0.05
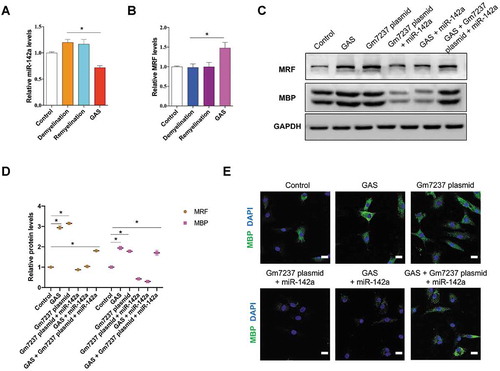
Discussion and conclusion
CNS demyelination, which can be induced by genetic abnormalities that impact glia and inflammatory damages to myelin and oligodendrocytes, has posed a great threat to human [Citation2]. Slow progress towards the treatment of CNS demyelination has been made due to the lack of global understanding of complex networks and practical therapeutic agents [Citation9]. Although antibody- or stem cell-based therapies have been developed to treat CNS demyelination [Citation7,Citation8], their delivery into CNS, safety and efficacy are still the major concerns for clinical use. Small molecules are promising alternatives to overcome these limitations and several small molecules identified by in vitro assays have been discovered as potential therapeutic agents.10−12 However, the complicated CNS microenvironment requires diversified small molecules that have the capability to promote CNS myelination in vivo, and understanding the underlying process may further facilitate the development of therapeutic strategies for CNS demyelination. Here, we reported GAS as a novel agent to promote CNS myelination. During this process, GAS up-regulated a lncRNA Gm7237, which acted as a ceRNA to compete with miR-142a and relieved expression of MRF that is a transcriptional regulator for CNS myelination [Citation35], to induce enhancement on CNS myelination ().
Figure 8. A schematic diagram of GAS-promoted CNS myelination. GAS upregulates the lncRNA Gm7237, which directly regulates miR-142/MRF pathway
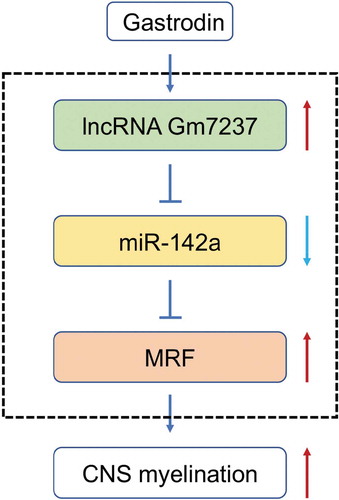
GAS is the major bioactive component of Gastrodia elata Blume, which is a Chinese traditional herbal plant for treatment of disorders, such as headache and dizziness[Citation37]. The biological activities of GAS have been extensively investigated, including its role in the treatment of CNS diseases[Citation13]. For instance, GAS has been used to treat Chronic epilepsy, Parkinson’s disease combined with mild cognitive impairment, post-stroke disease, vascular dementia [Citation13,Citation38–40]. The broad neuroprotective activity of GAS then inspired us that it might also have the capability to treat CNS demyelination. To this end, we developed demyelination mice models and treated the model mice with GAS through gavage. Gratifyingly, we observed that GAS significantly promoted CNS myelination (). This result suggests that we have discovered a novel agent for the treatment of CNS demyelination and a new pharmacological activity of GAS. The molecular basis for the role of GAS in the treatment of CNS demyelination that may further facilitate or enhance the therapeutics was then investigated by us.
Non-coding RNAs, including lncRNAs and miRNAs, have big roles in regulating gene expression and are widely involved in different human diseases [Citation22,Citation41]. For regulation of myelination, a series of studies have also been performed to reveal the roles of lncRNAs or miRNAs. For example, a lncRNA lnc158 is found to promote the differentiation of neural precursor cells into oligodendryocytes[Citation42]. Moreover, lncRNAs lncOL1 and 2700046G09Rik are discovered as regulators of CNS myelination [Citation25,Citation26]. During our exploration of the molecular mechanism for GAS’s activity in CNS myelination, we identified a lncRNA Gm7237 as the target for GAS through sequencing (). We further demonstrated that Gm7237 is a positive regulator of CNS myelination (). Thus, we identified a novel factor, the lncRNA Gm7237, in CNS myelination, which may serve as a future therapeutic target for CNS demyelination.
As a significant class of gene regulators, miRNAs contribute to a variety of both physiological and pathological processes, including CNS disorders, through regulating the expression of target mRNAs [Citation24,Citation31,Citation43]. For example, miR-23a can enhance oligodendrocyte differentiation in vitro and promote CNS myelination in vivo [Citation44]. MiR-125a is found to inhibit oligodendrocyte maturation and contributes to the development of multiple sclerosis [Citation45]. Since the significant function for lncRNAs is as ceRNAs to impede miRNA function [Citation19], we further tried to unravel the downstream pathways for Gm7237. Through a combination of bioinformatic methods and experimental validations, we found that miR-142a was the direct target of Gm7237 and MRF was the direct target of miR-142a (). Moreover, we also validated that miR-142a/MRF axis was involved in CNS myelination. miR-142a is previously discovered as an important regulator of immune responses [Citation46] and vascular development [Citation47]. Besides, miR-142 is up-regulated during multiple sclerosis and can modulate inflammation and T cell differentiation [Citation48]. Here, we found that miR-142a was a negative regulator of CNS myelination and repressed by Gm7237 during myelination. Moreover, MRF, the direct target of miR-142a as demonstrated by us, is a critical regulator of CNS myelination [Citation35,Citation36]. The relief of MRF expression through suppression of miR-142a by Gm7237 further enhanced myelination (), which coincided well with the previous report. Thus, we finally verified that GAS promoted CNS myelination through regulating the Gm7237/miR-142a/MRF axis ().
In summary, we have reported a novel therapeutic agent, GAS, for treatment of CNS demyelination. The biological activity of GAS was resulted from its up-regulation of the lncRNA Gm7237, which directly modulated miR-142a/MRF pathway. Therefore, our results provide not only a source of the agent but also a molecular basis for further treatment of CNS demyelination. The combined use of GAS and genetic methods that regulate Gm7237 or miR-142a to treat CNS demyelination is now underway in our group.
Supplemental Material
Download Zip (4.5 MB)Acknowledgments
We wish to thank Synthgene Biotech Cloud for technical assistance.
Disclosure statement
The authors declare no competing financial interest.
Supplementary Material
Supplemental data for this article can be accessed here.
Additional information
Funding
References
- Matas SL, Glehn F, Fernandes GB, et al. Cerebrospinal fluid analysis in the context of CNS demyelinating diseases. Arq Neuropsiquiatr. 2013;71(9B):685–688.
- Franklin RJ, Ffrench-Constant C. Remyelination in the CNS: from biology to therapy. Nat Rev Neurosci. 2008;9:839–855.
- Felts PA, Baker TA, Smith KJ. Conduction in segmentally demyelinated mammalian central axons. J Neurosci. 1997;17(19):7267–7277.
- Patani R, Balaratnam M, Vora A, et al. Remyelination can be extensive in multiple sclerosis despite a long disease course. Neuropathol Appl Neurobiol. 2007;33(3):277–287.
- Patrikios P, Stadelmann C, Kutzelnigg A, et al. Remyelination is extensive in a subset of multiple sclerosis patients. Brain. 2006;129:3165–3172.
- Bercury KK, Macklin WB. Dynamics and mechanisms of CNS myelination. Dev Cell. 2015;32(4):447–458.
- Cadavid D, Balcer L, Galetta S, et al. Safety and efficacy of opicinumab in acute optic neuritis (RENEW): a randomised, placebo-controlled, phase 2 trial. Lancet Neurol. 2017;16(3):189–199. .
- Wang S, Bates J, Li X, et al. Human iPSC-derived oligodendrocyte progenitor cells can myelinate and rescue a mouse model of congenital hypomyelination. Cell Stem Cell. 2013;12(2):252–264. .
- Franklin RJM, Ffrench-Constant C. Regenerating CNS myelin - from mechanisms to experimental medicines. Nat Rev Neurosci. 2017;18(12):753–769.
- Deshmukh VA, Tardif V, Lyssiotis CA, et al. A regenerative approach to the treatment of multiple sclerosis. Nature. 2013;502(7471):327–332. .
- Najm FJ, Madhavan M, Zaremba A, et al. Drug-based modulation of endogenous stem cells promotes functional remyelination in vivo. Nature. 2015;522(7555):216–220. .
- Mei F, Fancy SPJ, Shen YA, et al. Micropillar arrays as a high-throughput screening platform for therapeutics in multiple sclerosis. Nat Med. 2014;20(8):954–960. .
- Liu Y, Gao J, Peng M, et al. A review on central nervous system effects of gastrodin. Front Pharmacol. 2018;9:24/1-/18.
- Xing Y, Li L. Gastrodin protects rat cardiomyocytes H9c2 from hypoxia-induced injury by up-regulation of microRNA-21. Int J Biochem Cell Biol. 2019;109:8–16.
- Zhang Y, Wang C, Yu B, et al. Gastrodin protects against ethanol-induced liver injury and apoptosis in HepG2 Cells and animal models of alcoholic liver disease. Biol Pharm Bull. 2018;41(5):670–679.
- Lin LC, Chen YF, Lee WC, et al. Pharmacokinetics of gastrodin and its metabolite p-hydroxybenzyl alcohol in rat blood, brain and bile by microdialysis coupled to LC-MS/MS. J Pharm Biomed Anal. 2008;48(3):909–917.
- Luo L, Kim S-W, Lee H-K, et al. Gastrodin exerts robust neuroprotection in the postischemic brain via its protective effect against Zn2+-toxicity and its anti-oxidative effects in astrocytes. Animal Cells Systems. 2018;22(6):429–437. .
- Zhao X, Zou Y, Xu H, et al. Gastrodin protect primary cultured rat hippocampal neurons against amyloid-beta peptide-induced neurotoxicity via ERK1/2-Nrf2 pathway. Brain Res. 2012;1482:13–21.
- Quinn JJ, Chang HY. Unique features of long non-coding RNA biogenesis and function. Nat Rev Genet. 2016;17(1):47–62.
- Mattick JS, Taft RJ, Faulkner GJ. A global view of genomic information–moving beyond the gene and the master regulator. Trends Genet. 2010;26(1):21–28.
- Djebali S, Davis CA, Merkel A, et al. Landscape of transcription in human cells. Nature. 2012;489(7414):101–108. .
- Wu T, Du Y. LncRNAs: from basic research to medical application. Int J Biol Sci. 2017;13(3):295–307.
- Tay Y, Rinn J, Pandolfi PP. The multilayered complexity of ceRNA crosstalk and competition. Nature. 2014;505(7483):344–352.
- Bartel DP. Metazoan MicroRNAs. Cell. 2018;173(1):20–51.
- He D, Wang J, Lu Y, et al. lncRNA functional networks in oligodendrocytes reveal stage-specific myelination control by an lncOL1/Suz12 complex in the CNS. Neuron. 2017;93(2):362–378.
- Lin ST, Heng MY, Ptacek LJ, et al. Regulation of myelination in the central nervous system by nuclear lamin B1 and non-coding RNAs. Transl Neurodegener. 2014;3(1):4.
- Steelman AJ, Thompson JP, Li J. Demyelination and remyelination in anatomically distinct regions of the corpus callosum following cuprizone intoxication. Neurosci Res. 2012;72(1):32–42.
- Wahl SE, McLane LE, Bercury KK, et al. Mammalian target of rapamycin promotes oligodendrocyte differentiation, initiation and extent of CNS myelination. J Neurosci. 2014;34(13):4453–4465.
- Song YX, Sun JX, Zhao JH, et al. Non-coding RNAs participate in the regulatory network of CLDN4 via ceRNA mediated miRNA evasion. Nat Commun. 2017;8:289.
- Seo JS, Chua NH. Identification of long noncoding RNA-protein interactions through In Vitro RNA pull-down assay with plant nuclear extracts. Methods Mol Biol. 2019;1933:279–288.
- Bartel DP. MicroRNAs: target recognition and regulatory functions. Cell. 2009;136(2):215–233.
- Imig J, Brunschweiger A, Brummer A, et al. miR-CLIP capture of a miRNA targetome uncovers a lincRNA H19-miR-106a interaction. Nat Chem Biol. 2015;11:107–114.
- Liu J, Carmell MA, Rivas FV, et al. Argonaute2 is the catalytic engine of mammalian RNAi. Science. 2004;305(5689):1437–1441.
- Hutvagner G, Simard MJ. Argonaute proteins: key players in RNA silencing. Nat Rev Mol Cell Biol. 2008;9(1):22–32.
- Emery B, Agalliu D, Cahoy JD, et al. Myelin gene regulatory factor is a critical transcriptional regulator required for CNS myelination. Cell. 2009;138(1):172–185.
- Koenning M, Jackson S, Hay CM, et al. Myelin gene regulatory factor is required for maintenance of myelin and mature oligodendrocyte identity in the adult CNS. J Neurosci. 2012;32(36):12528–12542.
- Chinese Pharmacopoeia Commission. 2015. Beijing: Chinese Medical Science and Technology Press.
- Lu Y, Xu D. The study of EEG changes in epilepsy patients treated with gastrodin. Pharmac Clin Chin Mat Med. 2015;31:114.
- Chen L, Liu X, Wang H, et al. Gastrodin attenuates pentylenetetrazole-induced seizures by modulating the mitogen-activated protein kinase-associated inflammatory responses in mice. Neurosci Bull. 2017;33(3):264–272.
- Wang XL, Xing GH, Hong B, et al. Gastrodin prevents motor deficits and oxidative stress in the MPTP mouse model of Parkinson’s disease: involvement of ERK1/2-Nrf2 signaling pathway. Life Sci. 2014;114:77–85.
- Garzon R, Marcucci G, Croce CM. Targeting microRNAs in cancer: rationale, strategies and challenges. Nat Rev Drug Discov. 2010;9:775–789.
- Li Y, Guo B, Yang R, et al. A novel long noncoding RNA lnc158 promotes the differentiation of mouse neural precursor cells into oligodendrocytes by targeting nuclear factor-IB. Neuroreport. 2018;29(13):1121–1128.
- Rupaimoole R, Slack FJ. MicroRNA therapeutics: towards a new era for the management of cancer and other diseases. Nat Rev Drug Discovery. 2017;16(3):203–222.
- Lin S-T, Huang Y, Zhang L, et al. MicroRNA-23a promotes myelination in the central nervous system. Proc Natl Acad Sci U S A. 2013;110(43):17468–17473. S/1-S/11.
- Lecca D, Marangon D, Coppolino GT, et al. MiR-125a-3p timely inhibits oligodendroglial maturation and is pathologically up-regulated in human multiple sclerosis. Sci Rep. 2016;6(1):34503.
- Fan H-B, Liu Y-J, Wang L, et al. miR-142-3p acts as an essential modulator of neutrophil development in zebrafish. Blood. 2014;124(8):1320–1330.
- Lalwani MK, Sharma M, Singh AR, et al. Reverse genetics screen in zebrafish identifies a role of miR-142a-3p in vascular development and integrity. PLoS One. 2012;7(12):e52588.
- Talebi F, Ghorbani S, Chan WF, et al. MicroRNA-142 regulates inflammation and T cell differentiation in an animal model of multiple sclerosis. J Neuroinflammation. 2017;14(1): 55/1-/14. doi:10.1186/s12974-017-0832-7.