ABSTRACT
We have previously reported that not only transcripts of RNA polymerase II (pol II), but also one type of RNA transcribed by RNA polymerase III (pol III), undergo AAUAAA-dependent polyadenylation. Such an unusual feature is inherent in Short Interspersed Elements (SINEs) from genomes of certain mammals. For polyadenylation of its transcript, SINE should contain, besides an AATAAA hexamer and a transcription terminator, two specific regions: β, located downstream of box B of a promoter, and τ, preceding AATAAA. Here, using nucleotide substitutions in SINEs B2 (mouse) and Ves (bat), we identified nucleotides of β regions necessary for polyadenylation of their transcripts. These sequences (β signals) are the following: ACCACATgg in B2 and GGGCATGT in Ves. Using this approach, we identified τ signal of SINE B2 (GCTACagTGTACTTACAT), where TGTA tetramer is most important for polyadenylation. In Ves, τ region is a long polypyrimidine motif which is able to interact with PTB protein in Ves transcripts. We demonstrated by knockdown that B2 and Ves transcript polyadenylation is performed by canonical poly(A) polymerase with the participation of proteins CSPF-160 and Fip1, the known factors of mRNA polyadenylation. We also showed that a factor CFIm partaking in polyadenylation of many mRNAs, is involved only in polyadenylation of B2 transcripts. CFIm seems to interact with τ signal of В2 RNA and thereby facilitates the recruiting of other proteins engaged in polyadenylation. Thus, SINEs utilize at least some proteins involved in polyadenylation of pol II transcripts to polyadenylate their pol III transcripts.
Graphical abstract
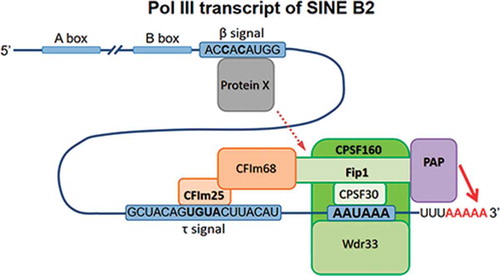
Introduction
In eukaryotic cells mRNAs and a lot of noncoding (nc) RNAs are synthesized by RNA polymerase II (pol II). Almost all mRNAs and most of these non-coding RNAs are subjected to polyadenylation (PA), i.e. poly(A) tail, up to 250 nt long, is synthesized at the 3ʹ-end of RNA [Citation1]. This reaction is carried out in the nucleus by canonical poly(A)-polymerase (PAP). PA is a complex process coupled with transcription termination, which also includes pre-mRNA cleavage at the specific site. Both cleavage and PA are directed and catalysed by a multiprotein 3ʹ-processing complex working in association with the C-terminal domain (CTD) of the largest subunit of pol II [Citation1]. Besides PAP, this complex includes multi-subunit factors such as Cleavage and Polydenylation Specifity Factor (CPSF), Cleavage Stimulation Factor (CStF) and mammalian Cleavage Factor I (CFIm) [Citation2]. The 3ʹ-processing complex recognizes specific sequences in pre-mRNA when pol II transcribes them at the end region of a gene. The main type of these sequences is a hexanucleotide polyadenylation signal (PAS), AAUAAA, which binds CPSF, namely its subunits CPSF30 and Wdr33 [Citation3]. PAS is located 10–30 nt upstream of the cleavage site. There are also auxiliary sequence elements enhancing cleavage and PA: some of them are located downstream of the cleavage site, whereas others are upstream of the PAS. These latter ones, named Upstream Sequence Elements (USEs), bind with CFIm [Citation4]. Thus, 3ʹ-processing is a subtle process directed by specific nucleotide sequences and the complex protein machinery, including pol II itself. Poly(A) plays an important role in the regulation of stability, export from the nucleus to the cytoplasm, and translation initiation of mRNAs [Citation5]. On the one hand, poly(A) protects mRNA from degradation in the cytoplasm [Citation6], and on the other hand, in the nucleus, it can direct the process of rapid decay of unwanted and spurious transcripts, mainly ncRNAs, generated by pol II [Citation7].
It is generally accepted that transcripts synthesized by two other eukaryotic RNA polymerases (pol I and pol III) cannot be polyadenylated in an above-described manner. However, as early as the 1980s, two examples of PA of RNA transcribed by pol III were reported. First, it is RNA synthesized from the 5ʹ-flanking region of the human β-globin gene [Citation8]. Second, it is RNA transcribed from a chimeric construct containing the pol III promoter of the adenoviral VAI RNA gene fused to the thymidine kinase gene [Citation9]. These transcripts have not been studied in detail and the mechanisms of their PA remain unclear. Later, we discovered a particular type of RNA generated by pol III which is polyadenylated in AAUAAA-dependent manner [Citation10,Citation11]. Pol III is a polymerase which is responsible for transcription of different small cellular RNA genes (5S rRNA, tRNAs, 7SL, 7SK, U6, Y RNAs and some others) [Citation12], as well as mobile genetic elements named Short Interspersed Elements (SINEs) [Citation13]. It turned out that pol III-generated transcripts of some SINEs can undergo AAUAAA-dependent PA.
Short Interspersed Elements (SINEs) are repetitive 80- to 400-bp sequences that are interspersed over the eukaryotic genomes and are amplified via reverse transcription (retroposition) [Citation13,Citation14]. SINEs belong to nonautonomous mobile elements since they utilize the reverse transcriptase of Long Interspersed Elements (LINEs). In mammals, usually LINE-1 is the donor of reverse transcriptase for SINE retroposition. The genome of mammalian species contains a few families of SINEs, each represented by up to a million copies. Most copies are not identical and their nucleotide sequences vary by 5–35%. A typical SINE consists of three parts: 5′-terminal ‘head,’ ‘body,’ and 3′-terminal ‘tail’ [Citation13]. The heads of SINEs demonstrate a similarity with one of the three types of cellular RNAs synthesized by pol III: tRNA, 7SL RNA, or 5S rRNA. Most SINE families originated from tRNA in the course of evolution; heads of such SINEs contain internal promoter for pol III consisting of 11 bp boxes A and B spaced by 30–40 bp. The bodies of SINEs are usually unique for each SINE family and their origins are largely unclear. The 3ʹ-terminal tails of most mammalian SINEs are variable irregular A-rich sequences.
RNAs transcribed by pol III from some SINEs play an important role in the cellular response to heat shock. SINE RNA can bind chromatin of stress response genes and block their transcription under normal conditions. Thermal stress induces rapid degradation of SINE RNA, which relieves the transcriptional block and enables the upregulation of stress-inducible genes [Citation15].
We previously found that some SINEs isolated from many mammals (e.g. mouse-like rodents, hedgehogs, bats, horses and dogs) have one or several hexamers AATAAA and a pol III terminator (TTTT) in their A-rich tails [Citation16]. Due to these T residues in the hexamer and terminator, we assigned such SINEs to class T+; SINEs without AATAAA and a pol III terminator were assigned to class T–. We showed that RNAs transcribed by pol III from T+ SINEs were polyadenylated and the replacement of T by C in the hexamer completely abolished PA [Citation10]. By examining SINEs of three families (B2 [Citation17,Citation18], Dip [Citation18] and Ves [Citation19–21]), we discovered that, besides AATAAA, two more regions were important for PA of their transcripts. The first of them (β region) was located downstream of the box B of pol III promoter, and the second (τ region) preceded an A-rich tail [Citation11]. In SINEs Dip and Ves, but not in B2, the τ region was a long polypyrimidine motif. Regions β and τ contributed nearly equally to PA of SINE transcripts and simultaneous deletions of both regions abolished the PA almost completely.
Since the position of the β and τ regions was previously determined only approximately, here we carried out a detailed analysis of the effects caused by nucleotide substitutions in the β and τ regions of SINE B2 from the mouse (Mus musculus) genome and the β region of SINE Ves from the bat (Myotis daubentonii) genome. As a result of this study, we identified nucleotide sequences which significantly contributed to the efficiency of the PA of RNA transcribed by pol III from these SINEs.
Polyadenylation of pol III-generated transcripts of T+ SINEs significantly increases their lifespan in cells [Citation10,Citation22], probably, due to the protection of RNA by poly(A)-binding protein (PABP). It has been proven that the A-rich tail of human SINE Alu (according to our classification, belongs to T – SINEs) is indispensable for its retroposition by LINE-1 reverse transcriptase [Citation23,Citation24]. This has also been confirmed for other LINE-1-mobilized SINEs [Citation25]. Due to a transcription terminator located upstream of the variable part of an A-rich tail in T+ SINEs, their transcripts lack the main part of the A-rich sequence [Citation10,Citation11]. Such RNA, apparently, cannot undergo LINE-1-dependent reverse transcription, and SINE cannot undergo retroposition. However, due to the PA of pol III-transcripts of T+ SINEs, these transcripts acquire long poly(A), and, obviously, become good templates for LINE-1 reverse transcriptase. We believe that the acquisition of their structural features by T+ SINEs that allow their RNA to be polyadenylated, enables them to overcome (bypass) some cellular defence mechanisms that inhibit the spread of SINEs throughout the genome. Interestingly, this strategy was independently ‘chosen’ by SINEs in a number of distant mammalian lineages.
In addition to canonical PAP, there are other poly(A)-polymerases in mammalian cells. The so-called Star-PAP polyadenylates mRNAs that encode proteins involved in the response of cells to stress and apoptosis induced by DNA damage [Citation26,Citation27]. These RNAs have an AUA motif upstream of a PA site and a suboptimal Downstream Sequence Element (DSE). Another enzyme, PAPD4, restores the length of shortened poly(A) tails of mRNA molecules in the cytoplasm; this process is known as cytoplasmic polyadenylation [Citation28,Citation29]. PAPD4 works in association with CPSF bound to PAS and with CPEB1 or 2 proteins that recognize specific sequences located between PAS and poly(A). In addition, there are other non-canonical PAPs that do not require a PAS in RNA [Citation30]; it is suggested that such PAPs could supply oligo(A) tails to some of the tRNA molecules that gave rise to simple ancestral SINEs in mammalian genomes [Citation31]. A priori, it cannot be ruled out that Star-PAP, PAPD4 or other non-canonical enzymes, rather than canonical PAP, implements PA of RNAs transcribed by pol III from T+ SINEs. Therefore, in the second part of this study, we tested this with the PAP knockdown and showed that SINEs B2 and Ves transcripts are polyadenylated by the canonical enzyme. Using the same approach, we found that the CPSF-160 and Fip1 proteins (CPSF subunits) are also required for PA of these transcripts. Finally, it was found that the auxiliary factor CFIm which is implicated in the 3ʹ-terminal processing of many mRNAs, is also involved in PA of pol III transcripts of SINE B2, but not Ves.
Materials and methods
Plasmid constructs
Plasmid constructs Ves-T, B2-T and Sor/B2-Т containing PAS (AAATAA), as well as Ves-C, B2-C and Sor/B2-С with inactivated PAS (AACAAA) were described previously [Citation10,Citation11]. All new constructs containing nucleotide substitutions or deletions were obtained by two-round PCR using Ves-T, B2-T or Sor/B2-Т as a template. Overlapped left and right parts of a SINE (amplified in the first PCR round) were mixed and full-size SINE with mutations were amplified in the second PCR round. Amplified DNA fragments were purified by electrophoresis in 2% agarose gel followed by elution with Cleanup Mini kit (Evrogen) and cloned into plasmid pGEM-T (Promega). Plasmids with cloned insertions were isolated by diaGene kit (3317.0250, Dia-m.ru) and subjected to DNA sequence analysis in order to exclude plasmids with random nucleotide substitutions spontaneously induced by PCR. Plasmids intended for transfection were isolated using NucleoBond PC 100 kit (Macherey-Nagel).
Cell transfections and siRNA knockdown of proteins
Plasmid constructs containing mouse SINE B2 or bat SINE Ves were transfected into human cancer cells HeLa. (Heterologous cells were used considering the convenience of hybridization detection of exogenous SINE transcripts in cellular RNA samples. Unlike normal cells, HeLa as well as many other cancer cell lines can actively express SINEs). Monolayer was grown to 80% confluency in Petri dishes (60-mm diameter). Cells were transiently transfected with 5 μg of plasmid DNA using TurboFect Transfection Reagent (ThermoFisher) following the manufacturer’s protocol. RNA was isolated 20 h post-transfection using the guanidinium thiocyanate method [Citation32].
In knockdown experiments, Hela cells were grown to 50–60% confluency in 60-mm dishes and double-stranded specific or scramble siRNAs (Table S1) were introduced into cells using Lipofectamine RNAiMax Transfection Reagent (ThermoFisher) following the manufacturer’s protocol. In 72 h, total proteins were isolated from cells (two dishes) treated with each type of siRNA or untreated at all. At the same time, siRNA-treated or control cells (one dish in each case) were transfected with one of the seven plasmid constructs (5 μg DNA) using TurboFect. Total cellular RNA was isolated in 20 h.
Northern blot analysis
Each RNA sample was treated with 60 u/ml RNase-free DNase I and 130 u/ml RiboLock RNase Inhibitor (both from ThermoFisher) in 150 μl of the mixture at 37 C for 30 min. Following inactivation of DNase (chloroform extraction and ethanol precipitation), RNA was resolved by electrophoresis in 6% polyacrylamide gel with 8 M urea (150 × 150 × 1 mm). RNA was transferred from the gel onto a Hybond-XL membrane (GE Healthcare) by semi-dry electroblotting at 3 V for 2.5 h. Hybridization probes that corresponded to 5ʹ-half of SINEs (B2 and Ves) or full-size SINE (Sor) were prepared by PCR [Citation11]. Amplified DNA fragments were purified by electrophoresis in agarose gel and eluted with Cleanup Mini kit. The probes were labelled with α[32P] dATP by PCR using only the reverse primer. The blots were hybridized in 50% formamide, 5 × Denhardt solution, 4 × SSC, 1% SDS and 0.1 mg/ml salmon sperm DNA at 42°C. Washes were performed in 0.1% SSC – 0.1% SDS at 42°C for 1 h. Then the membranes were exposed to X-ray film or/and screen (type SR) for the Phosphorimager Cyclone (Packard). The phosphorimager images were used to quantify SINE transcript polyadenylation. Radioactive signals were measured for the full-length primary SINE transcript (appearing as a distinct band of the longest RNA) and for the polyadenylated transcript (an even longer RNA appearing as a smear above the band). Finally, the percentage of polyadenylation was calculated for the transcripts of each SINE construct. Most of the transfection experiments were repeated three times. Results of typical experiments are shown in figures.
Western blot analysis
Whole cell lysate was separated by electrophoresis in 8% or 10% PAAG with 0.1% SDS. Proteins were transferred to PVDF membrane for 45–75 min using a semi-dry transfer apparatus at 15 V using CAPS buffer. The membranes were blocked with the mixture of 7% dry milk in PBS and 0.05% Tween-20 at 37°C for 45 min, and incubated with primary antibodies against the proteins studied (Table S2) at RT for 1 h. After three washes with PBS–Tween, membranes were incubated with anti-mouse secondary antibody at RT for 1 h. After three washes, proteins were detected using ECL Prime kit (GE Healthcare). The membranes were exposed to X-ray film and then ECL signals were measured with ChemiDoc MP Imager (BioRad).
Methods used for detection of association between Ves transcripts and protein PTB
We have previously described the use of this approach for detection of the binding of RNA to proteins in cells [Citation33]. Figure S1 shows three schemes for conducting these experiments with methodological details. Briefly, HeLa cells (four dishes) were transfected with the Ves-T or Ves-C plasmid, the cells were harvested in 20 h, disrupted by sonication and the cellular extract was isolated (Scheme A and B). In Scheme C, before cell disruption, they were irradiated with UV to cross-link RNAs with proteins bound to them, whereas in Scheme B, the already isolated cell extract was irradiated. Further, in all three schemes, the extracts were immunoprecipitated using antibodies against PTB (sc-56701, Santa Cruz) which were bound to Sepharose beads with protein G (Amersham). In Scheme A, the material bound and not bound to antibodies was deproteinized and RNA was separated by electrophoresis in PAAG with 8 M urea, followed by transfer of RNA to the Hybond-XL membrane. In Schemes B and C, the material bound and not bound to antibodies was separated by electrophoresis in PAAG with 0.1% SDS. Then, RNP and free RNA were transferred from the gel to Hybond-XL using semi-dry electroblotting. The final steps of the experiments carried out according to each of the three schemes were incubation of membranes with a 32P-labelled Ves probe, followed by washing and exposure of membranes with X-ray film (see Northern blot analysis).
Results
Previously, we discovered CATGG, or more rarely CATGT, pentanucleotide in the putative β regions of different T+ SINEs [Citation11], suggesting that these pentamers may play an important role in PA of T+ SINEs transcripts. To test this hypothesis, we changed CATGG in SINE B2 and CATGT in SINE Ves to other (complementary) sequences ( and ), transfected the obtained constructs to HeLa cells and analysed the isolated RNA by Northern hybridization. It turned out
Figure 1. The initial analysis of β region of SINE B2. (A) The nucleotide sequence of a B2 copy studied. Internal promoter (boxes A and B) and terminator for pol III are underlined. Two PASs are shown in italics. β and τ regions are highlighted in grey. Deletions (Δ) and substitutions (sub) introduced in constructs are shown below the corresponding regions of B2 nucleotide sequence. (B) Northern blot analysis of RNA from cells transfected by B2 with deletions and substitutions. B2-T is the original SINE; B2-C is SINE with inactivated PASs. An arrow indicates the primary transcript of B2; polyadenylated B2 RNA are marked by brace. (C) Effect of deletions and substitutions on PA of B2 transcripts. The level of PA of В2-Т is taken as 100%
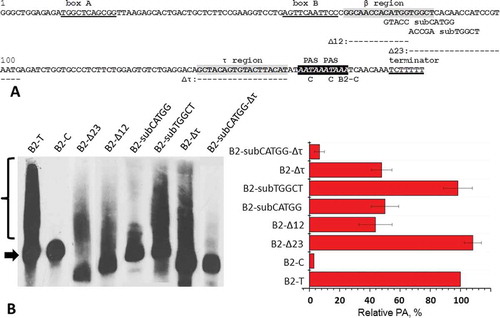
that these pentanucleotide changes reduced PA of B2 and Ves transcripts 2–2.5 times ( and ). The remaining PA level is to a large extent due to the existence of τ region, because simultaneous substitution of pentamers and deletion of τ region (Δτ) diminished PA of B2 and Ves transcripts 10 times ( and ). Thus, CATG(T/G) pentamers in β regions of B2 and Ves are indeed involved in PA of these SINEs transcripts.
In the same experiment, we examined B2 and Ves constructs with deletions of the sequences located immediately downstream of CATG(T/G) pentamer. The transcripts of these constructs (B2-Δ23 and Ves-Δ12) were polyadenylated nearly as effectively as the transcripts of the unchanged SINEs ( and ). The same applies to B2 with substitution of five nucleotides (TGGCT) immediately downstream of CATGG pentamer (). Thus, these results indicate that in both SINEs the sequence of β region involved in PA (β signal) does not extend downstream of CATG(T/G) pentamer.
Figure 2. The initial analysis of β region of SINE Ves. (A) The nucleotide sequence of a Ves copy studied. Boxes A and B, as well as a terminator for pol III are underlined. Two weak internal terminators are marked by arrows. PAS is shown in italics. β and τ regions are highlighted in grey. Deletions (Δ) and substitutions (sub) introduced in constructs are shown below the corresponding regions of Ves nucleotide sequence. (B) Northern blot analysis of RNA from cells transfected by Ves with deletions and substitutions. Ves-Т, SINE without changes; Ves-С, SINE with a mutation in PAS. Filled and open arrows mark full-size and truncated transcripts of Ves, respectively; braces show position of polyadenylated Ves RNA. (C) The influence of deletions and substitutions on PA of Ves transcripts. The level of PA of Ves-Т transcript is taken as 100%
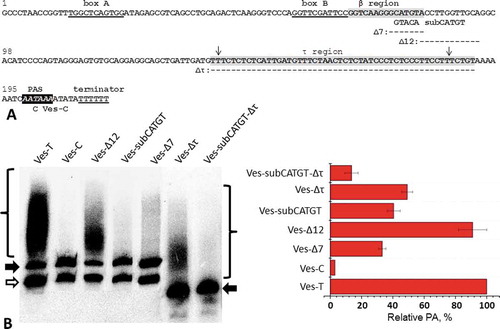
Identification of nucleotide sequence of β signal in SINE Ves
In the next experiment, we tried to determine the contribution to the PA of each of the pentamer nucleotides, as well as four upstream nucleotides in the β region of SINE Ves. The nucleotides in the sequence under study, AGGGCATGT, were numbered from 1 to 9. Based on the construct Ves-Δτ we obtained its 27 variants in which each of the nucleotides of this sequence was changed to the other three ones. These constructs were transfected to HeLa cells, and the effectiveness of PA of Ves transcripts was then quantitatively evaluated using Northern hybridization (Fig. S2). Surprisingly, many of the substitutions had little effect, i.e. the PA amounted to 80–90% of the PA level of the control construct transcripts (Ves-Δτ), or did not have the effect at all. The biggest impact was observed in the case of the constructs with 5C>A, 5C>G and 6A>C substitutions where PA amounted to 54%, 49% and 41% of the control, respectively (); this indicated the substantial effect of 5 C and 6A nucleotides on the functioning of β signal. Some substitutions of nucleotides 2G, 3G, 4G, 7T, 8G and 8T also had noticeable effect leading to a decrease of the PA to 65–75% of the control level (). Interestingly, almost all of the substitutions with marked effect were transversions, which is, apparently, due to a significant difference in size and form between purine and pyrimidine bases. That is why transversions should influence the RNA-protein interactions stronger than the transitions. The same pattern is known for regulatory DNA sequences [Citation34].
Figure 3. The influence of single-nucleotide substitutions in β region of SINE Ves on PA of its transcripts. The sequence of β region and nucleotide numbers are shown in upper and lower rows of the table. Names of constructs and their nucleotide substitutions are presented in the table. A diagram demonstrates the influence of these substitutions on PA of Ves transcript. The level of PA of the transcript containing the native β region, but lacked τ-region (Ves-Δτ) is taken as 100%. Error bars, SD, n = 3. The results of one of the transfection experiments with northern-blot analysis are shown in Fig. S2
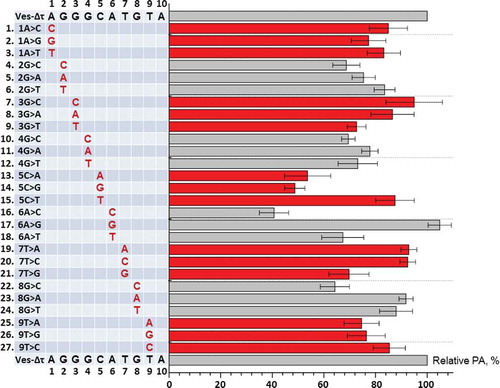
We suggested that the simultaneous change of two nucleotides slightly lowering the PA may lead to a far more pronounced effect. The double substitution will probably disturb the structure of β signal to such extent that the binding of this RNA site to hypothetical protein factors and, respectively PA, will decline significantly. In other words, the effect of the change of two nucleotides will exceed markedly the sum of the effects of each of the nucleotides. Double substitutions may probably give additional important information concerning the nucleotide sequence of the β signal. The substitution 5С>Т reducing the PA of Ves RNA to only 90% of the control level was chosen as the baseline. It should be noted that this nucleotide position is definitely important for β signal functioning, as 5 C change to A or G reduced the PA two-fold (). We also obtained the variants of Ves-Δτ construct where, apart from 5С>Т, other ineffective one-nucleotide changes located in the β region were introduced. The analysis of these constructs using HeLa cells transfection and Northern hybridization showed that many two-nucleotide mutations caused strong effects (). Thus, the substitutions 4G>T, 6A>G, 7T>G, 8G>T and 9T>G, when combined with 5С>Т, produced significant reduction of PA: 35%, 50%, 13%, 24% and 22% of the control, respectively (). This suggests an important role of GСATGT nucleotides (positions 4–9) for the performing of β signal in Ves. Similar two-nucleotide mutations affecting upstream nucleotides (1A>C, 2G> T and 3G>T) in the putative β signal produced noticeably smaller effects on PA – about 70–75% of the control (). Such a result indicates that these three nucleotides contribute to the functioning of the β signal but their impact is smaller than that of the other six nucleotides. This conclusion was confirmed by testing other paired substitutions in this site. Three such overlapping substitutions 1A>T + 2G>C, 2G>C + 3G>C and 3G>C + 4G>C lowered the PA to 80%, 65% and 40%, respectively. Thus, all the three positions are involved in the activity of the β signal and their impact increases from the first to the third nucleotide.
Figure 4. The impact of substitutions of two or more nucleotides in β region of SINE Ves. (A) Northern hybridization of RNA from cells transfected by Ves constructs with nucleotide substitutions. (B) The impact of substitutions on PA is shown in the diagram. Names of constructs and nucleotide substitutions are presented in the table. Numbers of the constructs are identical in the table and on the northern hybridization radioautograph (A). Еrror bars, SD, n = 3
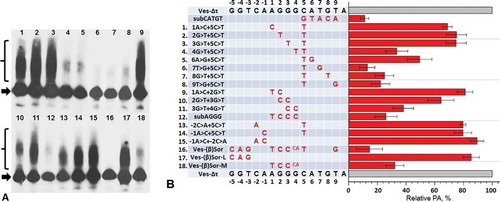
We also tested the nucleotides located upstream from the above sequence; these nucleotides were numbered from – 1 to – 5. The following three paired substitutions –1A>C + 5C>T, –2С>A + 5C>T and –1A>C (+) –2С>A lowered the PA to 80–90% of the control (). This result is difficult to interpret unambiguously and it cannot be excluded that nucleotides –1A and –2C are also involved in the functioning of β signal, which, however, seems unlikely.
We also conducted the experiments with the construct Ves-Δτ in which the whole β region or its parts were substituted with the sequences located in the same place of SINE Sor from shrew [Citation11,Citation16]. Sor belongs to class T–, i.e. its transcripts synthesized by pol III are not capable of polyadenylation [Citation11]. We suggested that the inability of Sor transcripts to PA is due, in part, to its non-functional ‘β region’. Indeed, the introduction of all of the 10 substitutions that convert the whole Ves β region to the corresponding Sor sequence reduced the PA of Ves-Δτ transcripts to 10% of the control (). The substitution (AGGG>TCCCA) affecting only the central part of Ves β region decreased PA to 30%, while the change of the left part of the Ves β region (GGT>CAG) had little effect: about 90% of the control PA (). This indicates that the nucleotides at positions – 3, – 4 and – 5 are most likely not involved in PA of Ves transcripts.
Based on all the above-presented data we conclude that the sequence GGGCATGT in the studied region makes the main contribution to PA. It is this sequence that we will continue to call the ‘core’ of β signal of SINE Ves or, for brevity, just β signal. One should take into account that the adjacent nucleotides may also be involved in the formation of the β signal and make some barely measurable contribution to PA. The full variant of SINE Ves β signal may be presented as follows: caaGGGCATGTa, where bold capital letters indicate the most important nucleotides.
Identification of nucleotide sequence of β signal in SINE B2
The study of SINE B2 β region was performed alongside with the experiments on the identification of SINE Ves β signal and was also started with the testing of the constructs with one-nucleotide substitutions. However, there were two differences in the experiment with B2. First, to minimize the effort, only transversions were introduced into B2 β region. Second, the substitutions were introduced to the full-size B2, which retains the τ region. This was done because in case of В2-Δτ the profile of the polyadenylated transcripts on the radioautograph became less distinct – it was devoid of ascension in its middle part and lacked descent immediately above the primary B2 transcript. Such profile was more difficult to analyse. However, on the other hand, the retained τ region increased the basal level of PA, which diminished the relative impact of the β region on this process.
Fourteen constructs with single substitutions involving nine nucleotides in B2 β region were obtained. HeLa cells transfection with the obtained constructs and subsequent Northern blot RNA analysis showed that almost all the single-nucleotide substitutions did not reduce the PA level of B2 transcripts (). The exception was 3С>G substitution which lowered the PA caused by β region to 60% of the control, B2-T. (Here and below we give the percentages calculated based on the fact that 50% of PA of B2 transcripts are associated with the activity of their τ-region; see the lower scale in .)
To get more pronounced effects we had to use di-, tri- and tetranucleotide substitutions. Such substitutions were introduced to the β region of B2 and the obtained constructs were tested in the experiments on the transfection of HeLa cells. Dinucleotide substitutions 1A>T + 2C> G, 3C>G + 4A>T and 5C>G + 6A>T decreased the PA caused by β region to 60%, 5% and 60%, respectively (), which indicates the importance of ACCACA nucleotides (positions 1–6) for functioning of β signal. Two overlapping trinucleotide substitutions at positions 5–7 (CAT) and 7–9 (TGG) lowered the PA to 50% and 75%, respectively. Thus, TGG also contributes to the activity of the β signal, though, apparently, its impact is less significant than the influence of the other nucleotides. Finally, the substitution of GGCA tetranucleotide located at positions from –4 to –1, that is upstream of the sequence under study, practically did not reduce the PA of the transcripts (). Taken together these results allow us to present the β signal of SINE B2 as follows: ACCACATgg, where the bold capital letters correspond to the most important for PA nucleotides.
Figure 5. The influence of nucleotide substitutions in β region of SINE B2 on PA of its transcript. (A) Northern hybridization of RNA from cells transfected by B2 constructs with substitutions. Arrows indicate the primary transcript of B2; polyadenylated B2 RNA are marked by braces. (B) Diagram demonstrated effect of substitutions. Constructs are numbered and presented in the same order as on the radioautograph. The level of PA of the B2-T transcript is taken as 100%. On the lower scale, PA of B2 transcript with the 5 bp substitution (GTACC) is taken as ‘0.’ The residual level of PA (to the left of the vertical line) is due to the activity of the τ-region (see ). Error bars, SD, n = 3
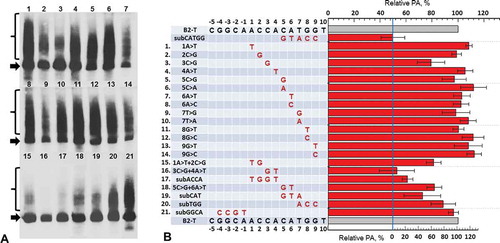
β signal of Ves is not able functionally to replace β signal in B2
To assess the degree of functional similarity (or difference) of Ves and B2 β signals we obtained five constructs based on B2-T in which three to six nucleotides in β region of B2 were replaced gradually making it identical to β region of Ves. These constructs were transfected to HeLa cells and the efficiency of PA of B2 transcripts was estimated (Fig. S3) as in the above experiments. The replacement of CCA located upstream of the pentamer with GGG lowered the PA to 35–40% of the control B2-T (the residual level of PA is due to the activity of B2 τ region). The additional substitutions of two nucleotides upstream of GGG and/or the last nucleotide in the pentamer (G > T) did not change the result significantly (Fig. S3). When all six nucleotides were changed, the 19 nt region of B2 became identical to the corresponding region of Ves. Thus, it may be concluded that the replacement of B2 β signal with Ves β signal decreases of PA of the resulting transcript. In other words, Ves β signal does not function as a part of B2 transcript, which indicates a significant functional difference between these two β signals.
Identification of nucleotide sequence of τ signal in SINE B2
In the previous work [Citation11] we found τ region in SINE B2 by adding 3ʹ-end region of B2 to the end of SINE Sor and testing transcripts PA of the obtained chimeric SINE. Sor is a SINE from the genome of the shrew (Sorex araneus) belonging to the class T– [Citation16]. Even if Sor is provided with PA signal (AATAAA) and pol III terminator, its transcripts remain incapable of PA [Citation11]. Apparently, Sor does not possess other sequences that are necessary for PA. It turned out that upon the replacement of the tail of SINE Sor with the tail of B2 together with the previous 18-nt region (τ region), the resulting transcript acquired the ability to PA [Citation11]. The use of such a chimeric SINE Sor/B2 opens a possibility for studying τ region of B2, while excluding the contribution of the β signal to the PA. Here we used this approach to find out the contribution of different nucleotides of τ region to the efficiency of the transcripts PA.
Figure 6. The impact of nucleotide substitutions in τ region of B2 on PA of transcripts of artificial chimeric element Sor/В2. (A) Northern hybridization of RNA from cells transfected by Sor/В2-T constructs with substitutions. The lane 1ʹ corresponds to the construct Sor/B2-C. Arrows indicate full-size primary transcript and braces mark the position of polyadenylated Sor/В2 RNA. (B) Diagram showing the relative level of PA of Sor/B2-Т transcripts with substitutions. Names of constructs and substitutions in them are presented in the table. Constructs are numbered in the same order as on the radioautograph. The results of an experiment with Sor/B2-sub-τMʹ construct are shown in Fig. S5. Error bars, SD, n = 3
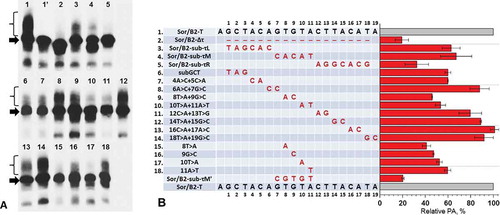
In the construct containing SINE Sor with the 3ʹ end part from B2 81 bp long (Fig. S4), the nucleotides located in the τ region of B2 were changed; all the changes were transversions. The constructs with the nucleotide changes were transfected to HeLa cells, and the isolated cellular RNA was analysed by Northern hybridization using the probe complementary to the sequence of Sor transcript (). The substitutions of the left hexamer (sub-τL) in τ region or the pentamer (sub-τM) located in the middle of this region reduced the PA of the transcripts to 60–65% of the control, Sor/B2-T (). The greatest effect (35% PA) was achieved by the replacement of the hexanucleotide located in the right part of the τ region (sub-τR). Besides, dinucleotide substitutions were introduced into the entire τ region. The replacements in the left part of this region (positions 1–3 and 4 + 5) lowered the PA to 60% of the control level (). Interestingly, the change of the two nucleotides (positions 6 + 7) did not influence the PA. The substitutions of two dinucleotides (8 + 9 and 10 + 11) located in the central part of τ had the greatest impact on PA: it dropped to 50%. Four dinucleotide substitutions corresponding to the right part of τ (positions 12 + 13, 14 + 15, 16 + 17 and 18 + 19), had a weak effect on PA, its level dropped not more than to 80% of the control (). This is quite an unexpected result taking into account that the replacement of the entire right part of τ (positions 12–18) led to the drop of PA to 35% (see above). Presumably, this region of τ is insensitive to the changes of dinucleotides which, apparently, affect only slightly the functioning of τ signal, whereas the replacement of the entire sequence (12–18) leads to significant disturbance of its work.
Since the dinucleotide substitutions showed that TGTA in the middle of the τ region (positions 8–11) contributes the most to the PA, we replaced each of the nucleotides in this tetramer separately (). All four single-nucleotide substitutions reduced PA significantly (40–60% of the control), which confirms the importance of TGTA tetramer for B2 τ signal functioning. This result allows us to suggest that τ signal may function due to the interaction with CFIm complex which is known to stimulate PA of some mRNAs by binding to UGUA tetramer in the 3ʹ-UTR of mRNA.
One contradiction may be found in the obtained results: single- and dinucleotide substitutions in the central part of τ region demonstrated a significant (two-fold) decrease of PA, while the replacement of the entire pentamer (sub-τM) led to only 1.5-fold PA reduction. Besides, this is significantly less than the effect (three-fold reduction) of substituting the right part of τ (sub-τR). While replacing GTGTA pentamer in the middle part of τ or the complementary pentamer (CACAT) we obtained by chance ACACACAT sequence in this part of τ region () which resembles the motif of B2 β signal (see above). It was suggested that this sequence can function as a β signal and thus support the PA of the transcript. We created a construct Sor/B2 with GTGTA pentamer replaced with CGCGT sequence and tested the resulting construct (sub-τMʹ) for the efficiency of PA of its transcripts. This replacement in τ region reduced PA to 24% of the control, i.e. four-fold (Fig. S5 and ). Thus, the middle part of the B2 τ region is indeed important for PA of this SINE transcripts.
The obtained results allow us to conclude that all or almost all of the 18 nucleotides of B2 τ region may be included in the τ signal, and its structure can be presented as GCTACagTGTACTTACAT, where bold capital letters correspond to the nucleotides most important for PA.
SINE Ves transcript is capable of binding to PTB protein
We have earlier found that the polypyrimidine motif (PPM) located upstream from the A-rich tail of SINEs Ves and Dip substantially contributes to the PA of their transcripts synthesized by pol III [Citation11]. Nucleotide sequences of this motif forming τ region differ significantly in Ves and Dip; PPM has a large length: 52 bp in Ves and 38 bp in Dip. It was shown that shorter (28 bp) synthetic PPM with regular nucleotide sequence also can stimulate the PA of transcripts [Citation11]. Taking into account all these data we considered it inappropriate to study the contribution of PPM to the PA using nucleotide substitutions and small deletions. Instead, we decided to test whether Ves transcripts are able to bind to PTB protein (PTBP1 – Polypyrimidine tract-binding protein 1) due to their τ region. It is known that PTB is involved both in the regulation of splicing and in the selection of polyadenylation sites [Citation35,Citation36], suggesting that PTB may take part in the PA of transcripts of Ves or other SINEs containing PPM.
The extract of HeLa cells transfected by Ves-T or Ves-C construct was subjected to immunoprecipitation using antibodies to PTB or mouse lgG2a immunoglobulin (negative control). RNA was isolated from the fraction of precipitate and supernate and subjected to Northern blot analysis using the probe complementary to Ves transcript. We found that PTB indeed binds to all types of Ves RNA that contain PPM, i.e. the full-size primary Ves transcript (212 nt) and the product of its PA, as well as to Ves RNA 185 nt long formed due to the termination of transcription on the ‘weak’ terminator located at the end of PPM ( and ). Even shorter RNA (140 nt) which is also the product of premature termination of Ves transcription does not bind to PTB (), obviously, due to the absence of PPM in this RNA ().
Figure 7. Co-immunoprecipitation of Ves RNA with PTB protein from extract of HeLa cells transfected by constructs Ves-T and Ves-С. (A) Northern hybridization RNA isolated from precipitate and supernate fractions. Precipitation was conducted by antibodies against PTB or control non-specific IgG2a (Cont.). Black, grey and white arrows indicate Ves primary transcripts 212, 185 and 140 nt long, respectively. Brace marks polyadenylated Ves RNA. (B and C) Hybridization of Ves probe to RNP complexes separated by electrophoresis in PAAG with SDS. In order to cross-link RNA with proteins, UV irradiation of the cell extract (B) or whole cells (C) was performed. Next, cell extract was fractionated by immunoprecipitation and isolated material was separated in PAAG. Hybridization at the bottom of the gel is due to Ves RNA not cross-linked to proteins. Asterisks mark major Ves RNPs that do not contain PTB
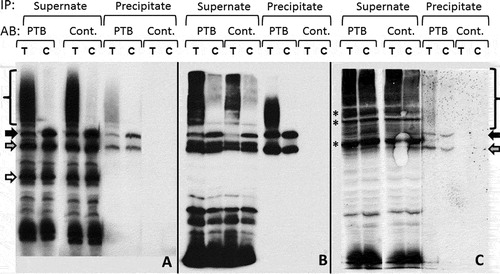
To corroborate the results obtained, the extracts of HeLa cells transfected with Ves-T or Ves-C construct were exposed to UV irradiation which causes RNA crosslinking to the proteins bound to it. Then immunoprecipitation of the extract by the antibodies to PTB was performed, after that the precipitated ribonucleoproteids (RNPs) and the material left in the solution were subjected to electrophoresis in PAAG with SDS. Blotting and the following hybridization with the Ves probe confirmed the data obtained in the previous experiment (). Moreover, this setting of the experiment revealed Ves RNA bound to PTB even better, apparently, due to UV-crosslinking that makes RNP completely resistant to the dissociation during immunoprecipitation.
To prove that the Ves RNA is bound to PTB in the living cells we conducted an experiment similar to the one described above, except for whole cells were UV-irradiated. With this setting of the experiment some fraction of Ves transcripts continued to co-precipitate with PTB, which indicates that some of these RNA molecules are associated with PTB protein in the living cells (). This experiment also indicates that Ves RNA in the cell binds to a significant number of various proteins; moreover, some of such complexes may be quite abundantly represented (marked by asterisks).
Identification of some proteins involved in PA of SINE B2 and Ves transcripts
The ability of PTB protein to bind with τ region of Ves transcript suggested that PTB could be a participant of PA of this SINE transcript. The presence in the SINE B2 τ signal of UGUA tetranucleotide which is identical to the recognition site of CFIm factor involved in PA of many mRNAs [Citation37] indicated the involvement of this factor in PA of B2 transcript. Besides, we suggested,
Figure 8. Western blot analysis of proteins subjected to knockdown. HeLe cells were transfected by specific or scramble siRNA or left untreated. In 72 h, total cellular proteins were isolated and analysed by immunoblotting. Protein hnRNP E2 (40 kDa) was used as control. Bands of proteins studied are marked by arrows. Diagram shows the efficiency of the knockdown the five proteins
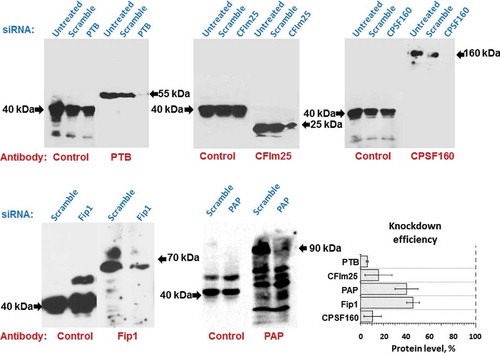
that PA of SINE transcripts generated by pol III is performed by the same protein complex that recognizes PAS in mRNA and polyadenylates it by recruiting canonical PAP. This complex mPSF (mammalian polyadenylation specificity factor) consists of four proteins: CPSF-160, WDR33, CPSF-30 and Fip1 [Citation3].
To test our hypothesis we used knockdown of the following proteins: canonical α and γPAPs, CPSF-160, Fip1, PTB and CFIm25. siRNAs specific to mRNAs of these proteins were introduced to the HeLa cells, which led to the reduction of their cellular level (). In three days of siRNA treatment, the cells were transfected with different SINE B2 and Ves constructs containing
Figure 9. Evaluation of the participation of PAP, CPSF-160, Fip1, PTB and CFIm25 proteins in PA of transcripts SINE B2 and Ves using knockdown. (A) Northern-blot analysis of RNA from cells treated or untreated with siRNA and transfected by various constructs of SINEs B2 and Ves. (B) Diagrams demonstrating the influence of the knockdown on PA of transcripts of B2 and Ves constructs (according to Northern hybridization data). Constructs B2-T and Ves-T contain both of the signals (β and τ), constructs B2-Δτ and Ves-Δτ have only β signals and constructs Sor/B2 and Ves-Δβ carry only τ signals
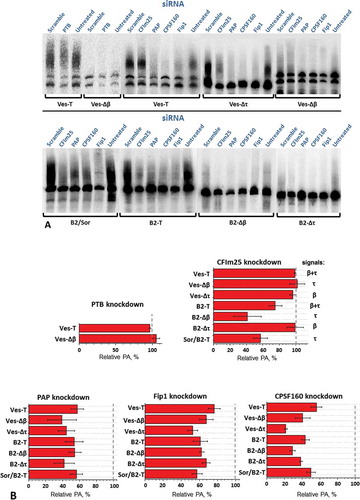
Both β and τ signals or just one of them. Finally, the level of PA of B2 and Ves transcripts was analysed by Northern hybridization (). The quantitative data presented in indicate the following. PA of transcripts of all the constructs was reduced upon knockdown of РАРs (α + γ), CPSF-160 and Fip1, i.e. canonical poly(A)-polymerase and mPSF complex are involved in PA of B2 and Ves transcripts generated by pol III. Surprisingly, the assumption about the role of PTB in the PA of Ves transcripts, apparently, turned to be incorrect: the knockdown of PTB did not diminish the PA of the SINE transcripts (). At the same time our prediction about the involvement of CFIm consisting of subunits CFIm25 and CFIm68 (or CFIm59), in the PA of B2 transcripts was confirmed: PA of the transcripts of B2 constructs containing τ signal reduced substantially upon knockdown of CFIm25 ().
Discussion
In this work, we investigated the phenomenon of PA of SINE transcripts generated by pol III. For this purpose, mouse SINE B2 and bat SINE Ves with native or altered nucleotide sequences were introduced into human HeLa cells, and the level of PA of the synthesized SINE transcripts was monitored. Since the human genome lacks B2 or Ves SINEs, HeLa cells cannot contain endogenous transcripts of these SINEs. Therefore, such heterologous cells for B2 and Ves transfection are very convenient for monitoring their transcripts. At the same time, the alterations in the proteins involved in PA in humans, mice and bats may lead to inaccurate or even erroneous results. However, this seems improbable considering that such basic processes as transcription and PA are highly conserved among mammals.
Using substitutions of the nucleotides in SINEs B2 and Ves we identified the sequences necessary for PA of these SINEs transcripts generated by pol III. These sequences called β and τ signals make approximately equal contributions to the PA efficiency; without β and τ signals pol III-depended transcripts are almost incapable of polyadenylation despite the presence of PAS (AAUAAA) in them. In both SINEs, β signals are located 5 bp downstream from box B of pol III promotor, while τ signals are located immediately upstream from the PAS.
Figure 10. The identified sequences of β and τ signals in SINE B2 and β signal in SINE Ves. The height of the bars characterizes the contribution of nucleotides to PA of SINE transcripts. Nucleotides that make up the signals are shown in black fonts, and adjacent nucleotides are present in grey. Underlined are the ‘conserved’ pentamer in β signals and the tetramer in the τ signal which is identical to a CFIm recognition site in mRNA molecules
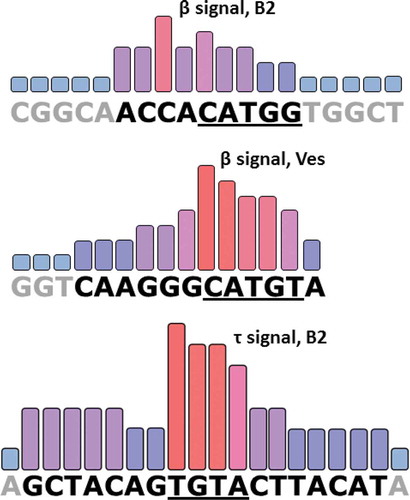
The length of β signals is 9–10 nt and they both contain conserved pentamer CATG(T/G) that we had earlier observed downstream from box B in all SINEs of class T+ [Citation11]. In the case of Ves, the nucleotides of this pentamer contribute primarily to the activity of the β signal, though the upstream three Gs also play a significant role in its function (). On the contrary, in the case of B2, the contribution of the three last nucleotides of the ‘conserved’ pentamer is comparatively small, whereas the role of the four nucleotides (ACCA) upstream from the pentamer is really significant (). Interestingly, the first two nucleotides of the pentamer (CA) in both SINEs are apparently important for the functioning of their β signals. Thus, by comparing the sequences and the impact of single nucleotides on the effectiveness of Ves and B2 β signals one may conclude that these are functionally related but significantly different signal elements. Their difference is also suggested by the fact that the Ves β signal being inserted into B2 instead of its own β signal is incapable of stimulating the PA of B2 transcript. We suggest that B2 and Ves β signals operate by binding with two different protein factors.
Comparing β signals of B2 and Ves with the putative β signals of other nine SINEs of class T+, one may notice that seven of them are similar to this B2 region whereas only two of them resemble the corresponding Ves region (Table S3). β signals of ‘type B2’ are characterized by the predominance of C and A residues in the four positions located upstream from the conserved pentamer. Those β signals that had at least two G residues in three positions upstream from the pentamer were referred to as ‘type Ves’ β signals. To prove conclusively the existence of two types of β signals additional bioinformatics and experimental studies of various SINEs are required.
The difference between the nucleotide sequences of B2 and Ves τ signals suggests that they should function in different ways. As we had shown earlier [Citation11] long polypyrimidine motifs (PPM) serve as τ signals in SINEs Ves and Dip. Such variable motifs upstream of PAS are present in 6 out of 11 SINEs of class T+ (shown in Table S4). In this work, it was demonstrated that in the cell a fraction of Ves transcripts was bound to PTB protein due to the presence of PPM in them. However, the experiments on knockdown of PTB did not confirm our suggestion about the involvement of this protein in the PA of Ves transcripts. There are two other polypyrimidine-binding proteins, PCBP1 and PCBP2 [Citation38] and all three proteins may be involved in the PA of Ves transcripts, which makes it difficult to detect the contribution of PTB to the PA of Ves transcripts in the knockdown experiment. In addition, the PA of the Ves transcript may be activated not by these polypyrimidine-binding proteins but by CstF, a major PA factor that usually binds to DSE. A rare example of stimulation of mRNA PA by the CstF interaction not with DSE but with a polypyrimidine-rich USE has been published [Citation35].
As we have shown, τ signal in SINE B2 is an 18-nt sequence of which TGTA tetramer is the most important (core) part. This tetramer corresponds to UGUA site which is recognized by CFIm – an auxiliary factor involved in the process of cleavage and polyadenylation of many pre-mRNAs [Citation4,Citation37]. CFIm consists of two subunits with a molecular mass of 25 kDa and 68 kDa (the latter may be changed to the related 59 kDa polypeptide); CFIm25 subunit binds directly to the UGUA site. Our experiments on CFIm25 knockdown showed that this protein is indeed involved in the PA of B2 transcripts and this is due to the presence of τ signal containing UGUA. Whereas UGUA tetramer is considered as the prime binding site of CFIm in the pre-mRNA molecules [Citation4,Citation37], the 6 or 7 nt sequences flanking this tetramer contribute significantly to the effectiveness of PA, as we have shown for the pol III-generated B2 transcript. In the course of evolution, the τ signal of B2 could have become an especially strong and effective binding site of CFIm25 due to the changes in the sequences flanking UGUA.
Apart from B2 other three SINEs (C, Ere-A and Ves) contain TGTA tetramer in their τ regions (Table S4). In pol III-generated transcripts of these SINEs, UGUA sites may also probably serve as τ signals. However, the experiment on CFIm knockdown showed that this is not the case for Ves transcript (), apparently, because of inappropriate nucleotide context – TGTA is located at the edge of PPM. It is noteworthy that another TGTA tetramer overlaps with the β signal of Ves. However, as revealed in the same experiment, this UGUA in the Ves transcripts does not stimulate its PA via the CFIm binding mechanism, which may be due to long distance (116 nt) between UGUA and the PAS, or unfavourable nucleotide context.
In our studies of nucleotide sequences of β and τ signals, we discovered that often single-nucleotide substitutions barely affected the PA of transcripts. This was observed for each of the nucleotides of B2 β signal (the only exception was the substitution 3C>G). For Ves β signal this phenomenon was less pronounced, so that the single-nucleotide substitutions in seven positions out of nine lowered the PA no more than to 70% of the initial level. We had to use dinucleotide substitutions in the β signals, and they turned out to be far more effective and informative mutations. However, even dinucleotide substitutions in the right part of B2 τ signal reduced the PA no more than to 80% of the control, while the replacement of all seven nucleotides decreased the PA to 35%. Such indifference of B2 β signals to the single-nucleotide substitutions and a part of B2 τ signal to dinucleotide substitutions impedes evaluation of the contribution of each nucleotide to the PA of the transcripts. Apparently, the requirements for the nucleotide sequences of these signals are quite relaxed, whereas usually functional elements in RNA and DNA are far more sensitive to single-nucleotide substitutions. For example, such changes in the PAS (AATAAA) of protein-coding genes lower its activity manifold [Citation39]. Additionally, TATA-like boxes in pol III-transcribed genes are quite ‘flexible’ and permissive sequences, however, the replacements of single nucleotides in them may reduce the transcription activity two-fold to five-fold [Citation40]. It can be assumed that nucleotide substitutions may affect the folding of B2 and Ves transcripts and thereby impact the accessibility and secondary structure of sites in RNA that bind protein factors. Indeed, many single-nucleotide substitutions had almost no effect, while longer substitutions greatly reduced the PA of transcripts.
The transcripts of class T+ SINEs are the only example of RNAs synthesized by pol III and polyadenylated in AAUAAA-dependent manner. This is quite a surprising phenomenon, because the entire mechanism of AAUAAA-dependent PA is adapted to the synthesis of poly(A) at 3ʹ-ends of the transcripts generated by pol II. The machinery of 3ʹ-end processing of pre-mRNA (cleavage/polyadenylation factors: CPSF, CstF, CFIm and CFIIm) is already associated with the C-terminal domain of the largest subunit of pol II during the gene transcription. In pol III there is no such specific long C-terminal domain adapted for the binding of these factors. Besides, the mechanisms of transcription termination performed by pol II and pol III are absolutely different. Thus, it remained unknown what factors are involved in the PA of transcripts synthesized by pol III on class T+ SINEs. Here using the knockdown of proteins we showed that CPSF-160, Fip1 and canonical poly(A)-polymerase (PAP) are involved in the PA of pol III-dependent transcripts of SINEs B2 and Ves. CPSF-160 and Fip1 are the two factors out of four forming mPSF complex responsible for the PA of pre-mRNA molecules, which is a part of an even bigger protein complex, CPSF. mPSF subunits called CPSF-30 and Wdr33 interact directly with PAS in the pre-mRNA molecules, while CPSF-160 serves as a scaffold in this complex [Citation3]. It is considered that Fip1 recruits PAP to the complex thus initiating the PA of mRNA. Our data indicate that the same mPSF complex recognizes and binds PAS in the pol III-generated transcripts of B2 and Ves, and recruits PAP which performs the PA of the transcripts.
About a half of mRNAs in human cells contain UGUA site near its PAS which is recognized by the 25 kDa subunit of CFIm factor [Citation37]. The latter stimulates the binding of mPSF (as a part of CPSF) to the PAS thus increasing the frequency of selection of this PAS by 3ʹ-processing machinery, as compared to the alternative PAS. This stimulation occurs due to the interaction of 68 kDa subunit of CFIm with Fip1 as a part of mPSF [Citation3]. We showed that the PA of pol III-generated B2 transcripts was decreased in CFIm25-depleted cells. The analogy with mRNA allows us to suggest that CFIm stimulates the PA of B2 transcript due to the binding of CFIm25 with τ signal in the RNA and simultaneous interaction of CFIm68 with Fip1. Such interaction promotes the association of mPSF with PAS and the initiation of PA. Interestingly, UGUA site (τ signal) in pol III-dependent B2 transcript is located approximately at the same distance (32 nt) from the 3ʹ-end of the RNA which appeared to be optimal for UGUA in mRNA molecules [Citation37]. This fact additionally supports the similarity between the PA of B2 transcripts and mRNA in which CFIm is involved.
We have not yet identified the proteins that have a bolstering effect on PA through interaction with β signals in B2 and Ves transcripts and τ signal of Ves transcript. Apparently, these hypothetical proteins are not involved in the PA of mRNA molecules or at least their involvement in this process has not been described before. However, one may suggest that similarly to CFIm, these proteins should act like facilitators of the binding of mPSF to PAS.
Thus, it appears that during evolution different SINEs of class T+ acquired nucleotide sequences allowing their transcripts generated by pol III to be polyadenylated. Several such sequences, PAS and τ signal in B2, are very similar to the PAS and USE that are located in the 3ʹ-terminal regions of mRNA. T+ SINEs have ‘mastered’ to use these signals and protein factors involved in the processing and PA of 3ʹ-ends of mRNA for the PA of their own transcripts. Probably, the transcripts of these SINEs recruit other proteins that are usually not involved in the PA of mRNA molecules, as facilitators of PA. From the general viewpoint, the processes of AAUAAA-dependent PA of the transcripts synthesized by pol II and pol III are similar in many ways. However, there are also clear differences. It is well known that the PA of the transcripts generated by pol II is closely linked with the processes of transcription and cleavage of the nascent RNA in a specific site near PAS. The PA of the transcripts synthesized by pol III is likely to occur post-transcriptionally. This is suggested by the presence of transcription terminators at the end of T+ SINEs and the absence of specific C-terminal domain from pol III capable of carrying the PA factors, as in the case of pol II. Further research will allow us to understand better the similarity and the difference between the mechanisms of PA of the transcripts synthesized by pol II and pol III.
Supplemental Material
Download Zip (1.5 MB)Acknowledgements
We thank Andrei Kramerov and Karina Tatosyan for the editorial assistance.
Disclosure statement
No potential conflict of interest was reported by the authors.
Supplementary material
Supplemental data for this article can be accessed here.
Additional information
Funding
References
- Proudfoot NJ. Ending the message: poly(A) signals then and now. Genes Dev. 2011;25(17):1770–1782.
- Shi Y, Manley JL. The end of the message: multiple protein-RNA interactions define the mRNA polyadenylation site. Genes Dev. 2015;29:889–897.
- Sun Y, Zhang Y, Hamilton K, et al. Molecular basis for the recognition of the human AAUAAA polyadenylation signal. Proc Natl Acad Sci U S A. 2018;115:E1419–E1428.
- Yang Q, Gilmartin GM, Doublie S. The structure of human cleavage factor I(m) hints at functions beyond UGUA-specific RNA binding: a role in alternative polyadenylation and a potential link to 5ʹ capping and splicing. RNA Biol. 2011;8:748–753.
- Nicholson AL, Pasquinelli AE. Tales of detailed poly(A) tails. Trends Cell Biol. 2019;29:191–200.
- Wigington CP, Williams KR, Meers MP, et al. Poly(A) RNA-binding proteins and polyadenosine RNA: new members and novel functions. Wiley Interdiscip Rev RNA. 2014;5:601–622.
- Wu G, Schmid M, Rib L, et al. Mechanism underlies nuclear RNA sorting by the human exosome. Cell Rep. 2020;30:2387–2401 e2385.
- Carlson DP, Ross J. Human beta-globin promoter and coding sequences transcribed by RNA polymerase III. Cell. 1983;34:857–864.
- Lewis ED, Manley JL. Polyadenylylation of an mRNA precursor occurs independently of transcription by RNA polymerase II in vivo. Proc Natl Acad Sci U S A. 1986;83:8555–8559.
- Borodulina OR, Kramerov DA. Transcripts synthesized by RNA polymerase III can be polyadenylated in an AAUAAA-dependent manner. RNA. 2008;14(9):1865–1873.
- Borodulina OR, Golubchikova JS, Ustyantsev IG, et al. Polyadenylation of RNA transcribed from mammalian SINEs by RNA polymerase III: complex requirements for nucleotide sequences. Biochim Biophys Acta. 2016;1859:355–365.
- Dieci G, Fiorino G, Castelnuovo M, et al. The expanding RNA polymerase III transcriptome. Trends Genet. 2007;23:614–622.
- Kramerov DA, Vassetzky NS. SINEs. Wiley Interdiscip Rev RNA. 2011;2:772–786.
- Ohshima K, Okada N. SINEs and LINEs: symbionts of eukaryotic genomes with a common tail. Cytogenet Genome Res. 2005;110:475–490.
- Zovoilis A, Cifuentes-Rojas C, Chu HP, et al. Destabilization of B2 RNA by EZH2 activates the stress response. Cell. 2016;167:1788–1802 e1713.
- Borodulina OR, Kramerov DA. Short interspersed elements (SINEs) from insectivores. Two classes of mammalian SINEs distinguished by A-rich tail structure. Mamm Genome. 2001;12:779–786.
- Krayev AS, Markusheva TV, Kramerov DA, et al. Ubiquitous transposon-like repeats B1 and B2 of the mouse genome: B2 sequencing. Nucleic Acids Res. 1982;10:7461–7475.
- Serdobova IM, Kramerov DA. Short retroposons of the B2 superfamily: evolution and application for the study of rodent phylogeny. J Mol Evol. 1998;46:202–214.
- Borodulina OR, Kramerov DA. Wide distribution of short interspersed elements among eukaryotic genomes. FEBS Lett. 1999;457:409–413.
- Kawai K, Nikaido M, Harada M, et al. Intra- and interfamily relationships of Vespertilionidae inferred by various molecular markers including SINE insertion data. J Mol Evol. 2002;55:284–301.
- Ray DA, Pagan HJ, Platt RN 2nd, et al. Differential SINE evolution in vesper and non-vesper bats. Mob DNA. 2015;6:10.
- Ustyantsev IG, Tatosyan KA, Stasenko DV, et al. Polyadenylation of sine transcripts generated by RNA polymerase III dramatically prolongs their lifetime in cells. Mol Biol (Mosk). 2020;54:78–86.
- Dewannieux M, Heidmann T. Role of poly(A) tail length in Alu retrotransposition. Genomics. 2005;86:378–381.
- Wagstaff BJ, Hedges DJ, Derbes RS, et al. Rescuing Alu: recovery of new inserts shows LINE-1 preserves Alu activity through A-tail expansion. PLoS Genet. 2012;8:e1002842.
- Dewannieux M, Heidmann T. L1-mediated retrotransposition of murine B1 and B2 SINEs recapitulated in cultured cells. J Mol Biol. 2005;349:241–247.
- Laishram RS. Poly(A) polymerase (PAP) diversity in gene expression–star-PAP vs canonical PAP. FEBS Lett. 2014;588:2185–2197.
- Neve J, Patel R, Wang Z, et al. Cleavage and polyadenylation: ending the message expands gene regulation. RNA Biol. 2017;14:865–890.
- Kim JH, Richter JD. Opposing polymerase-deadenylase activities regulate cytoplasmic polyadenylation. Mol Cell. 2006;24:173–183.
- Norbury CJ. Cytoplasmic RNA: a case of the tail wagging the dog. Nat Rev Mol Cell Biol. 2013;14:643–653.
- Anderson JT, Wang X. Nuclear RNA surveillance: no sign of substrates tailing off. Crit Rev Biochem Mol Biol. 2009;44:16–24.
- Roy-Engel AM. A tale of an A-tail: the lifeline of a SINE. Mob Genet Elements. 2012;2:282–286.
- Chomczynski P, Sacchi N. The single-step method of RNA isolation by acid guanidinium thiocyanate-phenol-chloroform extraction: twenty-something years on. Nat Protoc. 2006;1:581–585.
- Tatosyan KA, Koval AP, Kramerov DA. [Small noncoding 4.5SH and 4.5SI RNAs and Their binding to proteins]. Mol Biol (Mosk). 2018;52:1038–1044.
- Guo C, McDowell IC, Nodzenski M, et al. Transversions have larger regulatory effects than transitions. BMC Genomics. 2017;18:394.
- Moreira A, Takagaki Y, Brackenridge S, et al. The upstream sequence element of the C2 complement poly(A) signal activates mRNA 3ʹ end formation by two distinct mechanisms. Genes Dev. 1998;12:2522–2534.
- Romanelli MG, Diani E, Lievens PM. New insights into functional roles of the polypyrimidine tract-binding protein. Int J Mol Sci. 2013;14:22906–22932.
- Zhu Y, Wang X, Forouzmand E, et al. Molecular mechanisms for CFIm-mediated regulation of mRNA alternative polyadenylation. Mol Cell. 2018;69:62–74 e64.
- Ray D, Kazan H, Cook KB, et al. A compendium of RNA-binding motifs for decoding gene regulation. Nature. 2013;499:172–177.
- Sheets MD, Ogg SC, Wickens MP. Point mutations in AAUAAA and the poly (A) addition site: effects on the accuracy and efficiency of cleavage and polyadenylation in vitro. Nucleic Acids Res. 1990;18:5799–5805.
- Tatosyan KA, Stasenko DV, Koval AP, et al. TATA-like boxes in RNA polymerase III promoters: requirements for nucleotide sequences. Int J Mol Sci. 2020;21:3706.