ABSTRACT
The yellow fever mosquito Aedes aegypti is an obligatory blood feeder and a major arboviral disease vector, evoking severe public health concerns worldwide. In adult female mosquitoes, the gut is critical for blood digestion and pathogen entry. We aimed for a systematic exploration of microRNA expression dynamics in the gut during the gonadotrophic cycle. Small RNA libraries were constructed from female mosquito gut tissues at five time points. Unsupervised hierarchical clustering revealed three expression clusters (early, mid and late) peaking at sequential time points – 24, 48 and 72 h posteclosion. Differentially expressed miRNAs were identified at 24 h post-blood meal (PBM). Depletions of Methoprene-tolerant [Met; the juvenile hormone (JH) receptor] and Ecdysone receptor [EcR; the receptor to 20-hydroxyecdysone (20E)] were performed using dsRNA to these genes to investigate impacts on microRNA expressions. Our results suggest that Met-mediated signalling downregulates miRNA expression from the early cluster and upregulates that from the late cluster. EcR signalling either up- or downregulated miRNA levels at 24 h PBM, indicating a differential effect of this receptor in miRNA gene expression. Furthermore, miR-281, which is the most abundant miRNA in the gut tissue, is induced and repressed by Met- and EcR-mediated signalling, respectively. Systematic depletion using synthetic antagomir and phenotype examinations indicate that miR-281 is obligatory for the normal progression of blood digestion, ovarian development and reproduction. Collectively, this study unveils expression dynamics of microRNAs in the female gut tissue during the gonadotrophic cycle and demonstrates that they are affected by JH and 20E signalling.
Introduction
The yellow fever mosquito Aedes aegypti is the major vector that transmits arboviral pathogens causing yellow fever, dengue fever, chikungunya fever and Zika [Citation1–4]. Elucidation of mosquito biology provides invaluable resources for developing novel mosquito management practice [Citation5].
Throughout the life cycle, mosquitoes undergo a complete metamorphosis. The transition from pupa to adult marks changes in living habitat and food resources. Posteclosion (PE) maturation is required of female mosquitoes to prime for a gonadotrophic cycle, and juvenile hormone (JH) is a key factor controlling this phase of the gonadotrophic cycle [Citation6]. JH titre, which is high during the PE phase, rapidly drops after acquisition of a blood meal and is later re-elevated to prepare mosquitoes for a subsequent gonadotrophic cycle [Citation7]. In contrast, following a blood meal, 20-hydroxyecdysone (20E) titre rises to a peak by 18 h post-blood meal (PBM) and returns to a basal level around 48 h PBM [Citation8]. Drastic shifts of protein-coding gene expression have been revealed before and after a blood meal, and JH and 20E modulate these gene expression dynamics during mosquito gonadotrophic cycles [Citation6,Citation9].
Intake of a blood meal is required for female reproduction and facilitates pathogen dissemination. The gut is critical for blood digestion to provide nutrients for ovarian development and egg production [Citation10]. Importantly, it is also the pathogen entry site in mosquitoes [Citation11]. Thus, it has been a focal tissue for elucidating biological functions of digestive enzymes, ammonia-detoxification mechanisms and host–pathogen interactions [Citation12–14]. MicroRNAs (miRNAs) are a group of regulatory RNAs executing post-transcriptional regulation, and their physiological significance has been widely recognized in plants and animals [Citation15,Citation16]. Previous results demonstrated that in Ae. aegypti, several miRNAs are indispensable for normal progression of blood digestion in the gut; these include miR-1174, miR-1890 and miR-275 [Citation17–20]. miRNAs are recognized as a partial host toolkit against infection [Citation21], and their levels in mosquito guts fluctuate in response to Plasmodium parasites and viruses [Citation22]. In addition, conserved responses of Ae. aegypti miRNAs against infection of Dengue, West Nile and Zika viruses have been noted [Citation23].
In order to understand the possible coordination of miRNA expression dynamics in the mosquito gut with gonadotropic cycles, we constructed small RNA libraries to depict miRNA dynamics in the gut tissue of female adults before and after a blood meal. Expression profiles of miRNAs in this tissue were characterized, and novel miRNA candidate loci were revealed based on the analysis of gut small RNA libraries. Receptor-mediated JH and 20E signalling cascades were noted as two substantial forces modulating miRNA expression during the gonadotrophic cycle in the gut. Finally, characterization of miR-281, the most abundant miRNA in the gut, revealed its essential role in blood digestion and, consequently, egg maturation. This study expands our understanding of miRNAs regulated by two major developmental hormones.
Materials and methods
Animals and tissues
The UGAL/Rockefeller strain of Ae. aegypti was maintained under laboratory conditions, as described previously [Citation24]. Mosquitoes were reared and maintained at 27°C. Gut tissues were collected at 0–6 h, 24 h, 48 h and 72 h PE. Blood meals were provided from white Leghorn chickens, and fully engorged mosquitoes were subjected to gut collection at 24 h PBM. For phenotype examination, only fully engorged female mosquitoes were analysed. The animal use protocol was approved by the Institutional Animal Care and Use Committee of the University of California, Riverside.
Preparation and bioinformatics analysis of small RNA library
Biological material from the gut tissue of 20 female mosquitoes was collected for each library. The gut tissue from three independent collections was stored in TRIZOL (Thermofisher) at −80°C. Total RNA was extracted according to the manufacturer’s instructions and shipped to BGI Americas for small RNA library construction and sequencing. In total, 15 small RNA libraries were sequenced, with 3 independent biological replicates at each of the 5 time points: 0–6 h PE, 24 h PE, 48 h PE, 72 h PE and 24 h PBM.
Individual FASTQ files were separated based on indices. The systemPipeR pipeline was applied with modifications to obtain miRNA absolute read counts (https://github.com/tgirke/systemPipeR). Briefly, 3ʹ adaptor sequences (AGTCGGAGGCCAAGCGGTCTTAGGAAGACAA) were trimmed so that inserts were kept between 18 and 32 nt in length. Reads were mapped to the AaegL5 genome assembly of Ae. aegypti (VectorBase: https://www.vectorbase.org/) using Bowtie2 [Citation25]. Sequences were downloaded from miRBase for Ae. aegypti mature miRNAs and precursors (v22.1, http://mirbase.org/). Genomic coordinates were collected using BLAT [Citation26] on AaegL5 and converted to the GFF3 format. Mature miRNA profiling coordinates were obtained by 2-nt upstream and 5-nt downstream extensions, similar to that of miRDeep2 [Citation27]. Absolute read counts were subsequently extracted using the function of ‘summarizeOverlaps’ under the IntersectionStrict mode [Citation28], normalized to genome-mapped read numbers in each library, and presented as normalized expression in reads per million (RPM). The pipeline miRDeep2 was used with default parameters to identify novel miRNA candidates [Citation27]. Candidate loci were listed with a minimal miRDeep2 score of 1.
Antagomir and dsRNA treatment
The miR-281-5p antagomir was designed similarly to that previously described [Citation29] and purchased from Dharmacon (5ʹ mA.*.mC.*.mU.mG.mU.mC.mG.mA.mC.mG.mG.mA.mU.mA.mG.mC.mU.mC.*.mU.*.mC.*.mU.*.mU.3ʹ-Chl 3ʹ. m, 2ʹ-OMe modified base; *, phosphorothioate linkage; 3ʹ-Chl, cholesterol motif at 3ʹ end). A scrambled oligo (5ʹ mG.*.mU.*.mA.mA.mG.mU.mA.mG.mG.mU.mC.mC.mU.mC.mU.mA.mC.mU.*.mC.*.mG.*.mU.*.mC.3ʹ-Chl 3ʹ) was synthesized as a control antagomir. DsRNA was synthesized using MEGAscript kit (Ambion) and purified with MEGAclear transcription clean-up kit (Ambion). The DsRNA templates for Met and EcR were obtained by amplification using Superscript III reverse-transcription product of the gut tissue total RNA, while the template for Luciferase was amplified from psiCheck-2 vector (Promega). Template sequences were verified by Sanger sequencing before dsRNA synthesis. Primer sequences are included in Table S3. After injection, mosquitoes were allowed a recovery of up to 4 days at 27°C. For antagomir inoculation, 50 pmol was injected into each female thorax one day PE. For Met knockdown, 1 µg dsRNA was injected per mosquito at 6 h PE, and gut tissues were collected for analysis 4 days post-injection. For EcR knockdown, 2.5 µg dsRNA was injected per mosquito one day PE, and mosquitoes were provided a blood meal post-recovery; gut tissues were then collected for analysis at 24 h PBM.
Haemoglobin quantification in mosquito guts
Female mosquitoes were provided a blood meal, and fully engorged individuals were dissected for midgut at 24 h PBM without removing blood. For each group, one biological replicate consisted of three to six midguts placed in a 1.5-ml tube, snap-frozen and stored at −80°C until further processing. Tissues were homogenized in 500 µl Drabkin’s reagent (Sigma-Aldrich) using tube pestles and incubated at room temperature for 30 min. Tubes were centrifuged at 5,000 g for 5 min at room temperature and supernatant was transferred to a new tube. Then, 200 µl reaction mix was loaded per well in 96-well plates and readings were obtained at 540 nm in a Perkin Elmer Wallac Victor 2 Plate Reader. Serial dilution of haemoglobin (Sigma-Aldrich) was performed in Drabkin’s reagent and processed as described above for a standard curve.
Reverse transcription and quantitative PCR
qPCR was performed for miRNA and mRNA quantification, as described previously [Citation30]. Briefly, total RNA was extracted from biological materials stored in TRIZOL and treated with DNase I (Thermofisher) to remove genomic DNA contamination. Reverse transcription was performed using miScript II RT kit (Qiagen) and SuperScript III Reverse Transcriptase with Oligo(dT)12–18 primer (Thermofisher) for small RNAs and mRNAs, respectively. qPCR was conducted using QuantiFast SYBR Green PCR kit (Qiagen) in CFX 96 (BioRad). Primer sequences are included in Table S3. Expression data were normalized to that of U6 snRNA and RPS7 using the method of 2−ΔCt for small RNAs and protein-coding genes, respectively [Citation31].
Results
miRNA expression dynamics in the gut of adult female mosquitoes
To systematically investigate fluctuations of microRNA levels before and after a blood meal, we collected gut samples from 20 female mosquitoes at 5 time points: 0–6 h PE, 24 h PE, 48 h PE, 72 h PE, and 24 h PBM. Using these samples, we constructed and sequenced small RNA libraries. Three independent biological replicates were completed for each time point. From 15 libraries, we obtained a total of 355 million reads, of which 338 million (95.32%) were mapped to the AaegL5 genome (Table S1). Expression levels of 164 Ae. aegypti mature miRNAs were quantified, as registered in miRBase v22.1 [Citation32]. To evaluate consistency among three biological replicates, we first applied Principal Component Analysis (PCA) using DESeq2 package (Fig. S1) [Citation33] and, based on PC1 separation, three biological replicates correlated well for each time point. Thus, the average of normalized expression levels (reads per million, RPM) of three biological replicates was taken to represent miRNA abundance; this was done for each unique time point. We inspected the most abundant miRNAs in the gut tissue at 3 time points: 24 h PE, 72 h PE and 24 h PBM (). Among the top 10 miRNAs, seven were present at each time point: miR-281-5p, miR-1174, miR-184, miR-8-3p, miR-998, miR-14 and miR-276-3p. Of these seven, miR-281-5p, miR-184, miR-8-3p and miR-276-3p have been commonly noted as top abundant miRNAs in female adults of Ae. aegypti, Ae. albopictus, Anopheles gambiae, An. stephensi and Culex quinquefasciatus, suggesting their significance in regulation of mosquito physiology [Citation34–37]. In addition, miR-281-5p and miR-1174 have previously been characterized as gut-specific miRNAs in Ae. albopictus, Ae. aegypti and An. gambiae [Citation18,Citation38,Citation39], and they were identified as the two most abundant miRNAs in our small RNA libraries (Dataset S1).
Figure 1. miRNA expression dynamics in the gut tissue posteclosion at the adult stage. (A) Top 10, most abundant Ae. aegypti miRNAs at 24 h PE, 72 h PE and 24 h PBM in the gut tissue. (B) Unsupervised hierarchical clustering (Pearson correlation and complete linkage) of mature miRNAs during posteclosion (PE) time phase; expression levels are indicated by averages of normalized expression (RPM) from three independent biological replicates of small RNA libraries at unique time points. (C) Expression trend lines of three clusters peaking at 24 h (early; clade II and VI), 48 h (mid; clade III), and 72 h (late; clade IV) PE. For each miRNA, expression profiles were converted to represent percentages of normalized reads at each time point. Averages were taken at each time point within clusters to display trend lines. Cluster colours are consistent with those in panel B
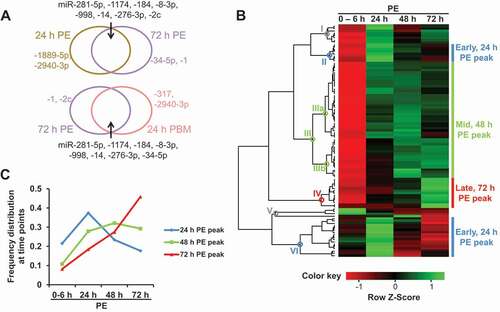
A cut-off of 5 RPM was applied for summed abundance at 5 time points, and profiles of 100 mature miRNAs were subjected to unsupervised hierarchical clustering analysis at the PE stage (). Based on monophyletic grouping, these miRNAs fell into six clades and there existed three major expression clusters: early (25, number of miRNA individuals included; clades II and VI), mid (50; clade III), and late (13; clade IV) (Table S2). Trend lines of three clusters disclose different miRNA expression patterns in the gut before the blood meal (): early, mid and late clusters illustrate individual miRNA expressions peaking at 24 h, 48 h and 72 h PE, respectively.
Gut samples were collected, independently from small RNA library construction, for validation by means of quantitative PCR (qPCR). Two miRNAs were selected from each major cluster, and their expression levels were quantified relative to that of U6 and compared between 0–6 h PE and the corresponding peak time points (Fig. S2A). Expression levels reached a statistical significance between 0–6 h PE and peak time points for miR-1175-3p and miR-2945-3p (early, 24 h PE peak), miR-8-3p and miR-281-5p (mid, 48 h PE peak), as well as miR-317 and miR-34-5p (late, 72 h PE peak), which matched expression patterns of these miRNAs suggested by the small RNA library analysis.
To evaluate impacts of a blood meal on miRNA expression, differential expression analysis was performed using edgeR package comparing gut miRNA levels at 72 h PE and 24 h PBM [Citation40]. In total, statistical significance (FDR<0.05) was reached for 17 miRNAs, including 14 upregulated and 3 downregulated at 24 h PBM (). Among them, levels of miR-184 and miR-275-3p had previously been shown to be elevated in the gut at 24 h PBM by means of northern blot and qPCR, respectively [Citation20,Citation41]. In addition, the level of miR-317 was upregulated at 24 h PBM in the Ae. aegypti gut based on small RNA library analysis [Citation41]. To support the differential expression analysis, the gut tissue was collected at 72 h PE and 24 h PBM and subjected to qPCR quantification independently from small RNA library construction. Two additional miRNAs – miR-305-5p and miR-11,899 – were validated to be upregulated at 24 h PBM in the gut relative to that of U6 (Fig. S2B). Two miRNAs – miR-281-5p and miR-2a-3p – were selected for qPCR examination by comparing relative levels at 72 h PE and 24 h PBM in small RNA libraries. When normalized to that of U6, their levels indeed were significantly lower at 24 h PBM, matching fluctuations suggested by small RNA library analysis (Fig. S2B; Dataset S1). Thus, by means of small RNA library and qPCR quantifications, we determined three major expression patterns of miRNAs in the gut during the PE stage and differentially expressed miRNAs at 24 h PBM compared with those at 72 h PE.
Figure 2. Differential expression of miRNAs in the gut at 72 h PE and 24 h PBM. List of differentially expressed miRNAs acquired with edgeR package (FDR<0.05), based on three independent biological replicates of small RNA libraries. The list was plotted with normalized expression (RPM) in each biological replicate
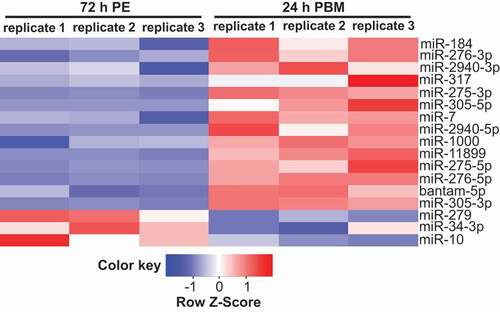
Deep sequencing of small RNA libraries advances novel miRNA discovery [Citation32]. Utilizing miRDeep2 package with default parameters and Ae. aegypti miRNAs in miRBase v22.1 [Citation27], we disclosed 155 candidate loci for novel miRNAs (Dataset S2). Small RNA sequencing in the gut tissue and our prediction presented additional support for 33 novel miRNA candidate loci identified in previous studies in the Ae. aegypti genome [Citation30,Citation36,Citation42,Citation43]. This provides a solid foundation for future confirmation as genuine novel miRNAs and for elucidating their biological functions.
JH receptor Methoprene-tolerant (Met) mediates the JH signalling cascade in regulation of miRNA expression in the mosquito gut during the PE stage
In mosquitoes, JH/Met signalling is crucial for the preparation of female adults for subsequent blood feeding and vitellogenic events [Citation6]. Our small RNA sequencing analysis has revealed miRNA clusters showing temporary correlation with either low or high titres of JH. This suggests that JH could be a defining factor controlling miRNA expression clusters at the PE phase. To test this hypothesis, we explored the effect of JH/Met signalling on miRNA expression by means of Met RNA interference (RNAi). Double-stranded RNAs (dsRNA) were synthesized for Met and Luciferase genes and injected into female mosquitoes at 6 h PE. After a 3-day recovery, gut tissues were concurrently collected from non-manipulated (WT), injection control (iLuc) and Met-depleted (iMet) mosquitoes and subjected to qPCR quantification. We first quantified the level of Met in iMet mosquitoes. When normalized to the expression level of the ribosomal protein S7 (RPS7), that of Met was shown to be significantly lower in the iMet group than in WT or iLuc (Fig. S3A). The gene Ornithine decarboxylase (AAEL000044, ODC) was previously identified as one repressed by JH/Met signalling [Citation6]. We examined ODC gene level as a positive control and, when normalized to that of RPS7, it was significantly higher in the iMet group than in WT and iLuc groups (Fig. S3A), supporting effective Met depletion by dsRNA.
Expression levels of mature miRNAs were quantified in response to the Met knockdown. Within the early cluster (24 h PE peak), levels of miR-1889-5p and miR-275-3p were significantly higher in the iMet group than in WT and iLuc groups, normalized to the U6 level (), suggesting that JH/Met signalling represses the expression of miRNAs from the early cluster. For the late cluster (72 h PE peak), Met depletion elicited statistically significantly lower levels of miR-317 and miR-279 compared with those in WT and iLuc groups (), and the results indicated that the rising titre of JH promotes miRNA expression in the late cluster. Regarding the mid cluster (48 h PE peak), a mixed response was noted where inhibition of JH signalling caused higher levels of miR-2a-3p and miR-100 as well as lower levels of miR-276-3p and miR-1000 (). A closer look reveals two sub-patterns in the mid cluster, corresponding to clades IIIa (early-mid) and IIIb (late-mid) (; Table S2). Mature miRNAs exhibited higher levels at 24 h PE and 48 h PE in clade IIIa (including miR-2a-3p and miR-100), while those in clade IIIb (containing miR-276-3p and miR-1000) displayed higher levels at the later time points, 48 h PE and 72 h PE. Within the 48 h PE peak cluster, our results imply that JH/Met signalling suppresses and induces miRNA expressions in the early-mid and late-mid sub-patterns, respectively. Collectively, these experiments illustrate that JH/Met signalling is a distinct driving force controlling miRNA expression dynamics during the PE reproductive phase.
Figure 3. Met-mediated JH signalling modulates miRNA expression. Non-manipulated mosquitoes (WT) and those injected with luciferase gene dsRNA (iLuc) were treated as control groups. Relative expression levels of mature miRNAs, normalized to U6, were surveyed using qPCR in the gut tissue responsive to Met knockdown (A, 24 h PE peak; B, 72 h PE peak; and C, 48 h PE peak). Three independent biological replicates were performed, and statistical significance was calculated using unpaired t-tests (*P-value <0.05; **P-value <0.01). Data are presented as mean ± SEM. Colours are consistent with those of expression patterns in , C
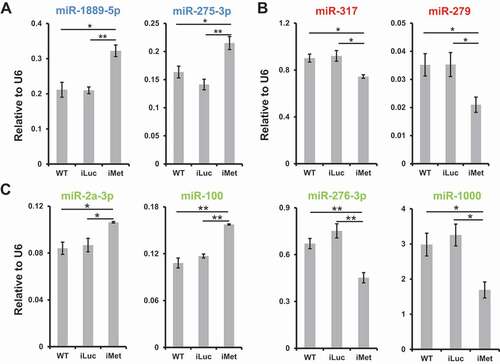
Ecdysone receptor (EcR)-mediated 20E signalling modulates miRNA expression at post-blood meal phase
Intake of a blood meal invokes drastic fluctuations of protein-coding gene expression in female mosquitoes, and 20E has previously been demonstrated to be the major hormone orchestrating unique expression patterns during the PBM phase of mosquito gonadotrophic cycles [Citation9]. To investigate its effect on miRNA expression levels, we applied an RNAi approach. Systematic depletion of EcR (iEcR) was performed using dsRNA, while non-manipulated (WT) and Luciferase dsRNA (iLuc) groups of mosquitoes served as negative controls. DsRNA injections were conducted at 1 day PE and a blood meal was provided after a 4-day recovery. Gut tissues were dissected at 24 h PBM and subjected to qPCR quantification. To evaluate the knockdown effect, we first examined the expression level of EcR and it was significantly lower in the iEcR group than in WT and iLuc (Fig. S3B). In addition, the gene Oxidoreductase (AAEL010075) was shown previously to be inhibited by 20E/EcR signalling in the fat body [Citation9], and we treated it as a positive control. Normalized to RPS7, the expression level of Oxidoreductase in the iEcR group was significantly higher than in WT and iLuc groups (Fig. S3B).
Guided by miRNAs up- or downregulated at 24 h PBM in the gut tissue, we next examined the impact of EcR depletion on their expression. Among miRNAs elevated at 24 h PBM, one miRNA (miR-11,899) was observed to be significantly reduced with EcR knockdown (), suggesting that its elevation occurs concurrently with a higher titre of 20E. For other upregulated miRNAs at 24 h PBM (), EcR depletion caused no significant reductions of miRNA levels compared with WT or iLuc groups, illustrated by examples of miR-317 and miR-275-3p (). We suggest that other factors contribute to increased levels of these miRNAs at 24 h PBM in the gut. A more consistent pattern was perceived for miRNAs downregulated at 24 h PBM where miR-279, miR-281-5p and miR-2a-3p were elevated significantly in response to EcR RNAi depletion (). This suggests that after a blood meal, a higher titre of 20E imposes distinguishably opposite effects on expression of studied miRNAs.
Figure 4. EcR-mediated 20E signalling modulates miRNA expression. Non-manipulated mosquitoes (WT) and those injected with luciferase gene dsRNA (iLuc) were treated as control groups. Relative expression levels of mature miRNAs, normalized to U6, were surveyed using qPCR in gut tissue responsive to EcR knockdown at 24 h PBM (A, upregulated miRNAs at 24 h PBM, listed in ; B, downregulated miRNAs at 24 h PBM, listed in and S2B). Three independent biological replicates were performed and statistical significance was calculated using unpaired t-tests (*P-value <0.05; **P-value <0.01). Data are presented as mean ± SEM
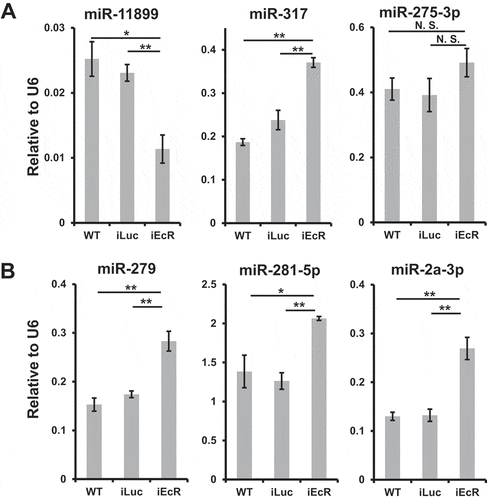
RNAi depletion of miR-281(−5p) adversely impacts blood digestion and reproduction in female Ae. aegypti mosquitoes
We identified miR-281 as the most abundant miRNA in the gut throughout the reproductive cycle (Dataset S1). The abundance of miR-281 was examined using qPCR relative to that of U6 in female adult tissues (fat body, ovary and gut) at 72 h PE and 24 h PBM (). It revealed that miR-281 is a gut-specific miRNA in female Ae. aegypti adults, similarly as described in two other mosquito species, An. gambiae and Ae. albopictus [Citation38,Citation39]. To explore functions of this miRNA, miR-281-specific antagomir was injected 1-day PE (prior to blood feeding). Non-manipulated (WT) mosquitoes and those injected with scrambled antagomir (Ant-Scr) served as controls. Four days after injection, mosquitoes were given a blood meal for phenotype examination. To assess knockdown effects, gut tissues were collected post-injection recovery and levels of miR-281 were inspected using qPCR relative to that of U6. The abundance of miR-281 was significantly lower in the gut of those with miR-281 antagomir injection than in WT and Ant-Scr injection controls (). To test the specificity of miR-281 knockdown, levels of miR-275-3p were similarly examined (), and no significant difference was observed in miR-275-3p levels after miR-281 antagomir injection when compared with WT or Ant-Scr group. In addition, miR-281 levels were quantified in the gut tissue at 24 h PBM and were significantly lower in the miR-281 antagomir group than in the WT or Ant-Scr groups, implying the sustained depletion of miR-281 ().
Figure 5. miR-281(−5p) is indispensable for the normal progression of blood digestion and gonadotrophic cycle. Non-manipulated mosquitoes (WT) and those injected with a scrambled antagomir (Ant-Scr) were treated as control groups. Statistical significance was calculated using unpaired t-tests (*P-value <0.05; **P-value <0.01). (A) Expression levels of miR-281 normalized to U6 were examined in female adult tissues of fat body (FB), ovary and gut at 72 h PE and 24 h PBM. (B-C) Expression levels of miR-281 normalized to U6 were examined at 72 h PE (B) and 24 h PBM (C) to confirm reduced expression due to the antagomir treatment. A randomly selected miRNA, miR-275-3p (B), was surveyed to affirm the specificity of the miR-281 antagomir. For illustration of panel B, means and SEMs are linearly scaled by treating average expression levels of WT as 1. (D) Digestive status was monitored by measuring haemoglobin (Hb) levels at 24 h PBM. Three to six fully engorged guts were homogenized per group in each biological replicate and three independent biological replicates were quantified. Results are illustrated as mean ± SD. (E) Follicle size was measured at 24 h PBM under the Leica M165 FC stereo microscope in groups of WT, Ant-Scr and Ant-281. In each group, one biological replicate consisted of readings from a minimum of 6 females and three independent biological replicates were performed. Data are presented as mean ± SEM. (F) Female fecundity was assessed by counting average number of eggs laid in WT, Ant-Scr and Ant-281 groups. In one biological replicate, eggs were counted from 15–20 females in each group, and a total of six independent biological replicates were performed per group. Data are presented as mean ± SD
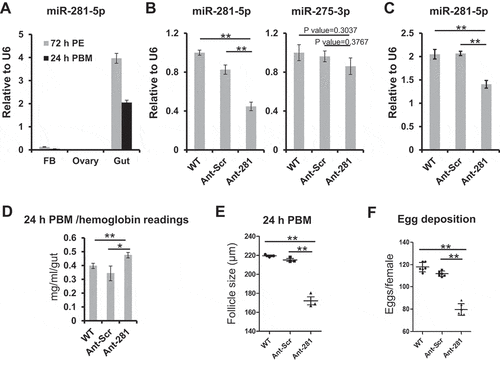
Mosquitoes were then screened for phenotype manifestations. First, blood digestion status was inspected by evaluation of haemoglobin (Hb) concentration using a cyanmethemoglobin-based method [Citation44]. Upon treatment with the miR-281 antagomir, the amount of Hb was significantly higher than that in the WT and Ant-Scr groups at 24 h PBM, implying that reduced levels of miR-281 hinder blood digestion in the gut (). Then, ovarian development was examined, and the primary follicle length was recorded at 24 h PBM. With miR-281 antagomir treatment, blood-fed mosquitoes failed to display fully developed vitellogenic ovaries, and their primary follicle lengths were significantly reduced to an average of 172 µm compared with 210–220 µm exhibited in WT and Ant-Scr controls (). Next, we assessed the effect of miR-281 depletion on mosquito fecundity. After miR-281 depletion, female mosquitoes laid significantly fewer eggs (80 eggs per mosquito on average) than either WT (118 eggs per mosquito) or Ant-Scr injected (112 eggs per mosquito) controls (). This implies the need to sustain the expression of miR-281 in the gut for female reproduction during the gonadotrophic cycle.
Discussion
Throughout the life cycle of mosquitoes, the adult stage is the most devastating for human health. Intake of a blood meal facilitates female reproduction and disease transmission; thus, adult females are a focal point in mosquito research. One particular group of microRNAs – miR-275, miR-1174, miR-8, miR-1890, miR-309 and miR-277 – are considered essential for female mosquito reproduction [Citation17–20,Citation45–47]. Our comparison of small RNA libraries during the period 72 h PE and 24 h PBM provides an updated list of differentially expressed miRNAs in the gut of Ae. aegypti (; Dataset S1). Compared with a previous list based on 454 sequencing [Citation41], our results independently confirm expression trends of 10 (from a total of 16) miRNAs: miR-11, −1175-3p, −184, −281-5p, −283, −317, −34, −8, −989, and −998. Other than miR-8, their endogenous gene targets remain unknown and future functional studies are required to elucidate their functions in the gut of female mosquitoes. In addition, microbiota abundance has been shown to increase at 24 h PBM and reduced levels of miR-275 and miR-305, in Ae. aegypti and An. gambiae respectively, elicit suppression of microbiota expansion [Citation20,Citation48]. It is interesting to note that, in our study, miR-275 and miR-305 were upregulated in the gut at 24 h PBM (), but it remains to be seen whether other up- or downregulated miRNAs contribute to increased gut microbiota abundance.
Our study also revealed systematic profiling of mature miRNAs in the gut tissue at 24-h intervals PE, and three distinct expression clusters were observed peaking at sequential time points (), C. In Ae. aegypti, there are 14 miRNA clusters where neighbouring precursors are located within 10 kb of the genome, and clustered miRNAs are generally believed to undergo polycistronic transcription [Citation36]. We noted cases in which clustered miRNAs fell into the same expression pattern in gut tissue. For instance, miR-2 family members miR-2a-3p, miR-2b, miR-2 c and miR-13-3p were categorized in clade IIIa; miR-11-3p and miR-998 were placed in clade III; and clustered mature miRNAs miR-1175-5p and miR-1174 in clade III (Table S2). Another clustered pair, miR-275-3p and miR-305-5p, exhibited significant upregulation at 24 h PBM in both the gut () and fat body [Citation30]. We speculate that temporal separation of miRNA expression patterns provides an additional layer to fine-tuning gene expression, and this hypothesis can be solved in future by in silico target prediction and transcriptome analysis of gut genes during adult maturation. Another solution is to utilize Argonaute 1 cross-linking and immunoprecipitation followed by high-throughput sequencing (AGO1 CLIP-seq) to uncover genome-wide downstream targets in the gut tissue [Citation49].
JH and 20E are widely considered as two critical hormones in insect development, and selected miRNAs are responsive to treatment with JH or 20E [Citation50]. Our results suggest that the Met-mediated JH signalling cascade is a significant driving force orchestrating miRNA expression. It suppresses miRNA levels from early and early-mid clusters while activating those in late-mid and late clusters PE ( and Table S2). The miRNA miR-281-5p was identified as the most abundant miRNA in the gut at each time point in small RNA libraries (Dataset S1). During the PE reproductive phase, the increasing level of miR-281-5p coincides with the JH rising titre (Fig. S4), supported by the observation that JH/Met signalling induces the expression of miR-281-5p (Fig. S5A). Treatment of miR-281-5p antagomir resulted in abnormal phenotypes, such as delayed blood digestion, reduced average follicle size and lower fecundity (). These phenotype manifestations clearly demonstrate the importance of this miRNA in regulating essential physiological functions in the mosquito.
Female mosquito reproduction exhibits a cyclical feature and the vitellogenic phase is elicited by each intake of a blood meal. Previous results suggested that certain protein-coding genes are cyclically regulated by JH/Met signalling during the PE and late PBM phases [Citation9]. Our results show the activation effect of JH/Met signalling on miR-281-3p and miR-279 ( and Fig. S5B). Inclusion of results from 48 h PBM and qPCR examination shows an increased abundance of these two miRNAs and miR-281-5p relative to that at 24 h PBM in the gut (Fig. S4). This observation indicates that JH/Met signalling imposes a cyclical regulation on microRNAs in the gut tissue to prepare female adults for subsequent gonadotrophic cycles.
Previous studies have demonstrated that the 20E titre peaks close to 18 h post-blood feeding [Citation8]; however, most of genes involved in egg maturation events exhibit their peaks at 24 h PBM [Citation9]. Thus, we selected the latter time point for the miRNA expression investigation. Among the miRNAs downregulated at 24 h PBM relative to 72 h PE, we found that in EcR RNAi mosquito guts, many displayed significantly higher levels responsive to EcR depletion including miR-281-5p, miR-279, miR-2a-3p, and miR-281-3p ( and Fig. S5). For miRNAs with significant upregulation at 24 h PBM (), miR-11,899 exhibited a significantly lower level after EcR knockdown (). Similarly, a previous study identified miR-1890 induced by 20E/EcR signalling in the gut [Citation19]. Collectively, the results imply that the 20E rising titre imposes opposite effects on miRNA expression in gut tissue post-blood feeding. We observed no additional miRNAs with significantly lower levels in EcR RNAi gut samples and thus speculate that other factors contribute to increased levels of miRNAs in the gut at 24 h PBM. For instance, serine proteases are considered major digestive enzymes in mosquito guts, among which late-phase serine proteases (profile peaking at 24 h PBM in the gut) have been shown to be directly stimulated by insulin-like peptide 3 [Citation51]. In addition, previous results demonstrated activation impacts of amino acids and nuclear receptor HR3 on protein-coding genes at distinct time points (6 h and 36 h, respectively) post-blood feeding in the fat body [Citation9].
In insects, miRNAs have been shown to link various signalling cascades and interact with pathway components as authentic targets [Citation50]. Interestingly, evolutionarily conserved 20E effects on individual miRNAs, including miR-8, miR-34, miR-2 and miR-281-3p, have been observed in various insect species ( and Fig. S5) [Citation52–55]. Our results expand the current view of miRNA expression associated with JH and 20E and provide insights into the interactions of miRNAs and developmental hormones. For instance, miR-2 family members were previously shown to bind the 3ʹ UTR of Kruppel homolog 1 (Krh1) directly and regulate developmental transition in the cockroach Blattella germanica [Citation56]. JH activation of miR-2 and Krh1 implies that miR-2 fine-tunes Krh1 expression through post-transcriptional regulation. Our results indicate antagonistic effects of JH and 20E on six selected miRNAs: miR-281-5p, −281-3p, −279, −317, −1000 and −278-3p in the mosquito gut (, , and Fig. S5). Future efforts are essential to elucidate the mechanistic basis of JH and 20E effects on miRNA gene expression.
The mosquito gut is the gateway for the pathogen entry. Thus, elucidation of gut functions is of paramount importance for designing novel approaches for a mosquito control. Significance of miRNA has been recognized in mosquito physiological processes such as development, metabolism, reproduction, innate immunity and pathogen interactions [Citation48]. Our present study has revealed the miRNA dynamics and their hormonal regulations in the gut of Aedes mosquito females, the life stage involved in acquisition and transmission of human disease pathogens. This information provides a solid foundation for the exploration of miRNA functions in the gut. Moreover, we have identified that the most abundant miRNA, miR-281, in the female mosquito gut is critical for the regulation of blood digestion and subsequently ovarian development and fecundity, offering further evidence that miR-281 may serve as a feasible direct target for developing control approaches leading to the collapse of mosquito populations.
Disclosure of potential conflicts of interest
No potential conflicts of interest were disclosed.
Supplemental Material
Download Zip (99.7 KB)Supplementary material
Supplemental data for this article can be accessed here.
Additional information
Funding
References
- Barrett ADT, Higgs S. Yellow fever: a disease that has yet to be conquered. Annu Rev Entomol. 2007;52:209–229.
- McFee RB. Selected mosquito borne illnesses - dengue. Disease-a-month. 2018;64:246–274.
- Tsetsarkin KA, Chen RB, Weaver SC. Interspecies transmission and chikungunya virus emergence. Curr Opin Virol. 2016;16:143–150.
- Weaver SC, Costa F, Garcia-Blanco MA, et al. Zika virus: history, emergence, biology, and prospects for control. Antiviral Res. 2016;130:69–80.
- Shaw WR, Catteruccia F. Vector biology meets disease control: using basic research to fight vector-borne diseases. Nat Microbiol. 2019;4:20–34.
- Zou Z, Saha TT, Roy S, et al. Juvenile hormone and its receptor, methoprene-tolerant, control the dynamics of mosquito gene expression. Proc Natl Acad Sci U S A. 2013;110:E2173–2181.
- Shapiro AB, Wheelock GD, Hagedorn HH, et al. Juvenile-hormone and juvenile-hormone esterase in adult females of the mosquito aedes-aegypti. J Insect Physiol. 1986;32:867–877.
- Hagedorn HH, Shapiro JP, Hanaoka K. Ovarian ecdysone secretion is controlled by a brain hormone in an adult mosquito. Nature. 1979;282:92–94.
- Roy S, Saha TT, Johnson L, et al. Regulation of gene expression patterns in mosquito reproduction. PLoS Genet. 2015;11:e1005450.
- Attardo GM, Hansen IA, Raikhel AS. Nutritional regulation of vitellogenesis in mosquitoes: implications for anautogeny. Insect Biochem Mol Biol. 2005;35:661–675.
- Salazar MI, Richardson JH, Sanchez-Vargas I, et al. Dengue virus type 2: replication and tropisms in orally infected Aedes aegypti mosquitoes. BMC Microbiol. 2007;7:9.
- Noriega FG, Wells MA. A molecular view of trypsin synthesis in the midgut of Aedes aegypti. J Insect Physiol. 1999;45:613–620.
- Petchampai N, Scaraffia PY. Nitrogen metabolism in mosquitoes new insights into the nitrogen metabolism in blood-fed mosquitoes. Prog Mosq Res. 2016;51:363–391.
- Bartholomay LC, Michel K. Mosquito Immunobiology: the Intersection of Vector Health and Vector Competence. Annu Rev Entomol. 2018;63:145–167.
- Skvortsova K, Iovino N, Bogdanovic O. Functions and mechanisms of epigenetic inheritance in animals. Nat Rev Mol Cell Biol. 2018;19:774–790.
- Yu Y, Zhang YC, Chen XM, et al. Plant noncoding RNAs: hidden players in development and stress responses. Annu Rev Cell Dev Biol. 2019;35:407–431.
- Bryant B, Macdonald W, Raikhel AS. microRNA miR-275 is indispensable for blood digestion and egg development in the mosquito Aedes aegypti. Proc Natl Acad Sci U S A. 2010;107:22391–22398.
- Liu S, Lucas KJ, Roy S, et al. Mosquito-specific microRNA-1174 targets serine hydroxymethyltransferase to control key functions in the gut. Proc Natl Acad Sci U S A. 2014;111:14460–14465.
- Lucas KJ, Zhao B, Roy S, et al. Mosquito-specific microRNA-1890 targets the juvenile hormone-regulated serine protease JHA15 in the female mosquito gut. RNA Biol. 2015;12:1383–1390.
- Zhao B, Lucas KJ, Saha TT, et al. MicroRNA-275 targets sarco/endoplasmic reticulum Ca2+ adenosine triphosphatase (SERCA) to control key functions in the mosquito gut. PLoS Genet. 2017;13:e1006943.
- Samuel GH, Adelman ZN, Myles KM. Antiviral immunity and virus-mediated antagonism in disease vector mosquitoes. Trends Microbiol. 2018;26:447–461.
- Feng X, Zhou S, Wang J, et al. microRNA profiles and functions in mosquitoes. PLoS Negl Trop Dis. 2018;12:e0006463.
- Saldana MA, Etebari K, Hart CE, et al. Zika virus alters the microRNA expression profile and elicits an RNAi response in Aedes aegypti mosquitoes. PLoS Negl Trop Dis. 2017;11:e0005760.
- Hays AR, Raikhel AS. A novel protein produced by the vitellogenic fat body and accumulated in mosquito oocytes. Roux’s Arch Dev Biol. 1990;199:114–121.
- Langmead B, Salzberg SL. Fast gapped-read alignment with Bowtie 2. Nat Methods. 2012;9:357–359.
- Kent WJ. BLAT–the BLAST-like alignment tool. Genome Res. 2002;12:656–664.
- Friedlander MR, Mackowiak SD, Li N, et al. miRDeep2 accurately identifies known and hundreds of novel microRNA genes in seven animal clades. Nucleic Acids Res. 2012;40:37–52.
- Lawrence M, Huber W, Pages H, et al. Software for computing and annotating genomic ranges. PLoS Comput Biol. 2013;9:e1003118.
- Velu CS, Grimes HL. Utilizing antagomiR (antisense microRNA) to knock down microRNA in murine bone marrow cells. Methods Mol Biol. 2012;928:185–195.
- Zhang X, Aksoy E, Girke T, et al. Transcriptome-wide microRNA and target dynamics in the fat body during the gonadotrophic cycle of Aedes aegypti. Proc Natl Acad Sci U S A. 2017;114:E1895–E1903.
- Schmittgen TD, Livak KJ. Analyzing real-time PCR data by the comparative C(T) method. Nat Protoc. 2008;3:1101–1108.
- Kozomara A, Griffiths-Jones S. miRBase: annotating high confidence microRNAs using deep sequencing data. Nucleic Acids Res. 2014;42:D68–D73.
- Love MI, Huber W, Anders S. Moderated estimation of fold change and dispersion for RNA-seq data with DESeq2. Genome Biol. 2014;15:550.
- Biryukova I, Ye T, Levashina E. Transcriptome-wide analysis of microRNA expression in the malaria mosquito Anopheles gambiae. BMC Genomics. 2014;15:557.
- Gu J, Hu W, Wu J, et al. miRNA genes of an invasive vector mosquito, Aedes albopictus. PloS One. 2013;8:e67638.
- Hu W, Criscione F, Liang S, et al. MicroRNAs of two medically important mosquito species: aedes aegypti and Anopheles stephensi. Insect Mol Biol. 2015;24:240–252.
- Skalsky RL, Vanlandingham DL, Scholle F, et al. Identification of microRNAs expressed in two mosquito vectors, Aedes albopictus and Culex quinquefasciatus. BMC Genomics. 2010;11:119.
- Lampe L, Levashina EA. MicroRNA tissue atlas of the malaria mosquito anopheles gambiae. G3. 2018;8:185–193.
- Zhou Y, Liu Y, Yan H, et al. miR-281, an abundant midgut-specific miRNA of the vector mosquito Aedes albopictus enhances dengue virus replication. Parasit Vectors. 2014;7:488.
- Robinson MD, McCarthy DJ, Smyth GK. edgeR: a bioconductor package for differential expression analysis of digital gene expression data. Bioinformatics. 2010;26:139–140.
- Li S, Mead EA, Liang S, et al. Direct sequencing and expression analysis of a large number of miRNAs in Aedes aegypti and a multi-species survey of novel mosquito miRNAs. BMC Genomics. 2009;10:581.
- Akbari OS, Antoshechkin I, Amrhein H, et al. The developmental transcriptome of the mosquito Aedes aegypti, an invasive species and major arbovirus vector. G3. 2013;3:1493–1509.
- Nouzova M, Etebari K, Noriega FG, et al. A comparative analysis of corpora allata-corpora cardiaca microRNA repertoires revealed significant changes during mosquito metamorphosis. Insect Biochem Mol Biol. 2018;96:10–18.
- Shah AP, Patel PT, Patel BP, et al. Evaluation of microtitre plate-based Haemoglobin estimation. Int J Lab Hematol. 2018;40:196–200.
- Ling L, Kokoza VA, Zhang C, et al. MicroRNA-277 targets insulin-like peptides 7 and 8 to control lipid metabolism and reproduction in Aedes aegypti mosquitoes. Proc Natl Acad Sci U S A. 2017;114:E8017–E8024.
- Lucas KJ, Roy S, Ha J, et al. MicroRNA-8 targets the Wingless signaling pathway in the female mosquito fat body to regulate reproductive processes. Proc Natl Acad Sci U S A. 2015;112:1440–1445.
- Zhang Y, Zhao B, Roy S, et al. microRNA-309 targets the Homeobox gene SIX4 and controls ovarian development in the mosquito Aedes aegypti. Proc Natl Acad Sci U S A. 2016;113:E4828–4836.
- Dennison NJ, BenMarzouk-Hidalgo OJ, Dimopoulos G. MicroRNA-regulation of Anopheles gambiae immunity to Plasmodium falciparum infection and midgut microbiota. Dev Comp Immunol. 2015;49:170–178.
- Darnell RB. HITS-CLIP: panoramic views of protein-RNA regulation in living cells. Wiley Interdiscip Rev RNA. 2010;1:266–286.
- Luhur A, Chawla G, Sokol NS. MicroRNAs as components of systemic signaling pathways in drosophila melanogaster. Dev Timing. 2013;105:97–123.
- Gulia-Nuss M, Robertson AE, Brown MR, et al. Insulin-like peptides and the target of rapamycin pathway coordinately regulate blood digestion and egg maturation in the mosquito Aedes aegypti. PloS One. 2011;6:e20401.
- Chen J, Liang Z, Liang Y, et al. Conserved microRNAs miR-8-5p and miR-2a-3p modulate chitin biosynthesis in response to 20-hydroxyecdysone signaling in the brown planthopper, Nilaparvata lugens. Insect Biochem Mol Biol. 2013;43:839–848.
- Jiang J, Ge X, Li Z, et al. MicroRNA-281 regulates the expression of ecdysone receptor (EcR) isoform B in the silkworm, Bombyx mori. Insect Biochem Mol Biol. 2013;43:692–700.
- Jin H, Kim VN, Hyun S. Conserved microRNA miR-8 controls body size in response to steroid signaling in Drosophila. Genes Dev. 2012;26:1427–1432.
- Xiong XP, Kurthkoti K, Chang KY, et al. miR-34 modulates innate immunity and ecdysone signaling in Drosophila. PLoS Pathog. 2016;12:e1006034.
- Lozano J, Montanez R, Belles X. MiR-2 family regulates insect metamorphosis by controlling the juvenile hormone signaling pathway. Proc Natl Acad Sci U S A. 2015;112:3740–3745.