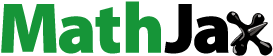
ABSTRACT
Nine distinct classes of self-cleaving ribozymes are known to date, of which the pistol ribozyme class was discovered only 5 years ago. Self-cleaving ribozymes are able to cleave their own phosphodiester backbone at a specific site with rates much higher than those of spontaneous RNA degradation. Our study focuses on a bioinformatically predicted pistol ribozyme from the bacterium Paenibacillus polymyxa. We provide a biochemical characterization of this ribozyme, which includes an investigation of the effect of various metal ions on ribozyme cleavage and a kinetic analysis of ribozyme activity under increasing Mg2+ concentrations and pH. Based on the obtained results, we discuss a possible catalytic role of divalent metal ions. Moreover, we investigated the ligation activity of the P. polymyxa pistol ribozyme – an aspect that has not been previously analysed for this ribozyme class. We determined that the P. polymyxa pistol ribozyme is almost fully cleaved at equilibrium with the ligation rate constant being nearly 30-fold lower than the cleavage rate constant. In summary, we have characterized an additional representative of this recently discovered ribozyme class isolated from P. polymyxa. We expect that our biochemical characterization of a pistol representative in a cultivatable, genetically tractable organism will support our future investigation of the biological roles of this ribozyme class in bacteria.
Graphical abstract
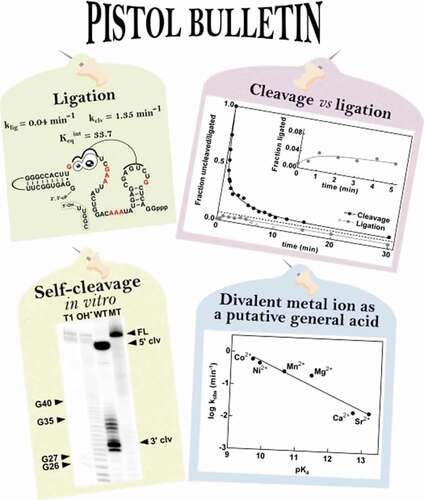
Introduction
The RNA World theory [Citation1] was hypothesized by Carl Woese [Citation2], Leslie Orgel [Citation3], and Francis Crick [Citation4] around 50 years ago. This concept assigned additional functions to RNA, converting our view of this molecule from a mere passive information transmitter to a versatile predecessor of protein enzymes. This idea has gained more attention because of the discovery of catalytically active RNAs, called ribozymes, over the past decades. Prominent early examples of such ribozymes, which fulfil essential functions in living cells, include self-splicing RNA in Tetrahymena [Citation5] and the RNA subunit of ribonuclease P [Citation6]. Later, the first class of small self-cleaving ribozymes, called hammerhead ribozymes, joined the list of possible remnants of the RNA World, revealing additional catalytic RNAs [Citation7].
Self-cleaving ribozymes perform site-specific phosphoester transfer reactions without the involvement of protein chaperones or enzymes [Citation8,Citation9]. To date, nine distinct classes of natural self-cleaving ribozymes are known. Some of them, like hammerhead, hairpin, hepatitis delta virus (HDV) or the Varkud satellite (VS) ribozymes, were discovered unintentionally, while studying the corresponding biological systems [Citation10]. In contrast to these initial findings, the remaining classes, glmS, twister, twister sister, pistol and hatchet were identified bioinformatically and then validated experimentally [Citation11–13].
The discovery of the pistol ribozyme was based on the notion that self-cleaving ribozymes in bacteria frequently associate with certain genetic elements, so that the search for conserved RNA structures was narrowed to intergenic regions close to these genetic elements [Citation12]. Pistol RNAs are commonly associated with bacteriophage-related genes, and appear to be widespread in the Firmicutes phylum and in DNA sequences collected from environmental samples [Citation14]. The unique secondary structure of this ribozyme class consists of three base-paired stems (P1-P3), an internal loop and a stabilizing pseudoknot () [Citation15]. The catalytic core of the ribozyme contains 10 highly conserved nucleotides, which are suggested to play a critical role in high-speed catalysis [Citation14].
Figure 1. Sequence, structure and activity of the P. polymyxa pistol ribozyme. (A) Sequence and secondary structure of the P. polymyxa pistol ribozyme. Nucleotides in red correspond to the highly conserved positions from the consensus model [Citation14]. Loop 3 (nucleotides coloured grey) is absent in the bimolecular construct (see Supplementary Fig. 1A for details). Altered nucleotides in the mutant construct are boxed. P1-, P2- and P3-stems are indicated. Cleavage site is indicated by a black arrowhead. (B) Self-cleavage activity of the P. polymyxa pistol ribozyme. Internally labelled cleavage products were separated by denaturing 20% PAGE. The full-length ribozyme (FL) and cleavage products (5ʹ clv; 3ʹ clv) are indicated by black arrowheads. T1: RNase T1-ladder; OH−: partial alkaline digest-ladder (see Materials and methods for details). WT: wild-type ribozyme; MT: mutant ribozyme. (C) Cleavage activity of the P. polymyxa bimolecular pistol ribozyme construct. The 32P-labelled substrate RNA (5 nM) was incubated for the times indicated in the presence (+) or absence (-) of 20 mM MgCl2 and unlabelled enzyme RNA (100 nM) (see Materials and methods for details). Cleavage products were separated by denaturing 20% PAGE. The full-length substrate (sub) and 5ʹ-cleavage product (5ʹ clv) are denoted
![Figure 1. Sequence, structure and activity of the P. polymyxa pistol ribozyme. (A) Sequence and secondary structure of the P. polymyxa pistol ribozyme. Nucleotides in red correspond to the highly conserved positions from the consensus model [Citation14]. Loop 3 (nucleotides coloured grey) is absent in the bimolecular construct (see Supplementary Fig. 1A for details). Altered nucleotides in the mutant construct are boxed. P1-, P2- and P3-stems are indicated. Cleavage site is indicated by a black arrowhead. (B) Self-cleavage activity of the P. polymyxa pistol ribozyme. Internally labelled cleavage products were separated by denaturing 20% PAGE. The full-length ribozyme (FL) and cleavage products (5ʹ clv; 3ʹ clv) are indicated by black arrowheads. T1: RNase T1-ladder; OH−: partial alkaline digest-ladder (see Materials and methods for details). WT: wild-type ribozyme; MT: mutant ribozyme. (C) Cleavage activity of the P. polymyxa bimolecular pistol ribozyme construct. The 32P-labelled substrate RNA (5 nM) was incubated for the times indicated in the presence (+) or absence (-) of 20 mM MgCl2 and unlabelled enzyme RNA (100 nM) (see Materials and methods for details). Cleavage products were separated by denaturing 20% PAGE. The full-length substrate (sub) and 5ʹ-cleavage product (5ʹ clv) are denoted](/cms/asset/b632b5fb-f20b-45d1-9288-32a94a33be37/krnb_a_1874706_f0001_oc.jpg)
A previously characterized pistol ribozyme representative from Lysinibacillus sphaericus is able to reach near-maximal activity at physiological concentrations of Mg2+ and performs more than 100 cleavages per minute under optimal reaction conditions. Based on cleavage assays in the presence of various metal ions, a suggestion was made that divalent metal ions are not absolutely required for the ribozyme cleavage activity and might serve a purely structural role [Citation14].
Possible catalytic strategies of the pistol ribozyme were discussed not long after its discovery [Citation14], but a deeper understanding of the general acid-base catalysis was gained from first structural analyses [Citation15,Citation16]. In the course of subsequent structure–function studies of the pistol ribozyme, the identity of the general base remained unchallenged [Citation15–18], whereas the putative general acid has changed from a nucleobase [Citation15,Citation16] to a hydrated metal ion [Citation17–20]. In the most recent structural analysis [Citation17], a new mechanistic proposal even included two Mg2+ ions, possibly playing a catalytic, as well as a stabilizing role.
Therefore, different roles for metal ions in the pistol ribozyme cleavage mechanism have been proposed based on biochemical and structural data. According to first biochemical experiments, metal ions were suggested to play a structural rather than a catalytic role [Citation14]. In contrast, resolving the crystal structure of the pistol ribozyme and performing additional biochemical and computational experiments resulted in the identification of a divalent metal ion playing the role of a general acid in the cleavage reaction [Citation17–20]. Thus, we expect that biochemical investigations, presented in our study, will provide additional evidence for one of the above-mentioned functions of metal ions in pistol ribozyme cleavage.
In summary, our study offers an additional point of view on the biochemistry of the pistol ribozyme class based on a representative from the bacterial species Paenibacillus polymyxa. The effect of various metal ions on pistol ribozyme cleavage is investigated and we discuss a possible catalytic role of divalent metal ions. Moreover, we addressed the ligation reaction as another aspect of pistol ribozyme biochemistry, which has not been investigated before. It was determined that cleavage activity dominates at equilibrium, which makes the P. polymyxa pistol ribozyme a more efficient nuclease than ligase. These data establish the foundation for a thorough investigation into the biological roles of this self-cleaving ribozyme class in bacteria.
Materials and methods
Assays of self-cleavage during in vitro transcription. A double-stranded DNA template encoding the wild-type pistol RNA from Paenibacillus polymyxa ATCC 842 (NZ_GL905390.1/3,135,931–3,135,862) was generated by PCR using the P. polymyxa genomic DNA as a template and synthetic DNA primers containing the T7 promoter (Biomers, Supplementary Table 1). The DNA template of the pistol motif was extended by 23 base pairs of natural sequence to obtain a longer 3ʹ-cleavage product for easier detection after in vitro transcription. A DNA template encoding a cleavage deficient ribozyme sequence was generated by PCR using overlapping synthetic DNA oligos with the intended mutation (Biomers). Mutations inhibiting ribozyme activity were introduced according to the findings of Citation14. For the production of size standards, 5ʹ 32P-labelled mutant RNA was partially digested with RNase T1 (T1-ladder) or with alkali (OH–-ladder). In vitro transcriptions of the wild-type and mutated ribozymes were performed at 37°C using T7 RNA polymerase (50 U), TIPP (2 U/µl), 100 ng of DNA template in 80 mM HEPES (pH 7.5), 25 mM MgCl2, 10% DMSO and 5 mM DTT. NTPs were present in the reaction mixture at a final concentration of 2.5 mM, supplemented with traces of [α-32P] ATP (Hartmann Analytik). Internally 32P-labelled products were separated using denaturing 20% PAGE. For detection, the Amersham Typhoon imaging system (GE Healthcare) was used.
Verification of the sequence identity. For sequence validation of the transcription template, purified PCR product was cloned with CloneJET PCR cloning kit (Thermo Scientific) using the sticky end cloning protocol according to the manufacturer’s instructions. Afterwards, Top10 E. coli chemically competent cells were transformed with the ligation product. After identification of successfully transformed clones by colony PCR, the plasmid DNA was isolated from the selected colonies using GeneJET Plasmid Miniprep Kit (Thermo Scientific). Samples were prepared for Sanger sequencing according to the Microsynth (Balgach, Switzerland) sample requirements using the standard pJET1.2-forward primer (5ʹ-CGACTCACTATAGGGAGAGCGGC-3ʹ).
Cleavage assays. To assay the cleavage activity of the pistol ribozyme, a bimolecular construct based on the representative from P. polymyxa (NZ_GL905390.1/3,135,931–3,135,862) was generated by splitting the RNA sequence in loop 3 (Supplementary Fig. 1A). The substrate strand was chemically synthesized (Biomers). The enzyme strand was prepared by in vitro transcription using T7 RNA polymerase. Substrate RNA was 5ʹ-labelled with [γ-32P] ATP (Hartmann Analytik) and T4 polynucleotide kinase (New England BioLabs) according to the manufacturer’s instructions. After transcription (enzyme strand) or labelling (substrate strand), RNA was purified by denaturing PAGE, isolated from the gel by crush-soaking in a solution containing 10 mM Tris–HCl (pH 7.5), 200 mM NaCl, and 1 mM EDTA (pH 8.0), and concentrated by precipitation with ethanol.
Bimolecular cleavage reactions contained labelled 5 nM substrate and 100 nM enzyme for single-turnover conditions. Reaction components were incubated at 23°C in a standard reaction [30 mM HEPES (pH 7.5) and 20 mM MgCl2], or under different conditions when noted. Reactions were initiated by addition of MgCl2 and halted at specific times by the addition of an equal volume of stop solution (90% formamide, 50 mM EDTA, 0.05% xylene cyanol and 0.05% bromophenol blue).
Reactions with mono- and divalent metal ions were performed similarly, but initiated by addition of the corresponding cation; for cleavage assays examining the effects of monovalent metal ions and cobalt hexammine, EDTA at the final concentration of 30 mM and 5 mM, respectively, was added to the reaction mixture. All cations tested were chloride salts.
For kinetic experiments testing the relationship between the cleavage rate and pKa of the hydrated metal ion, NaCl at a final concentration of 2 M was present in the reaction mixture, as well as 0.1 mM EDTA and 50 mM MES (pH 6.0) [reaction conditions according to Citation18]. Under these reaction conditions, the fraction of cleaved substrate in the presence of 2 M NaCl alone, was subtracted from the fraction of cleaved substrate measured in the presence of both 2 M NaCl and 1 mM divalent cation. Thus, the influence of divalent metal ions without the contribution of monovalent sodium can be analysed.
The final volume of each reaction was 10 μl. Reaction products were separated by using denaturing 20% PAGE. Gels were imaged using Amersham Typhoon imaging system (GE Healthcare). Cleavage reactions for the Mg2+-dependent activity profile were performed with 30 mM MES buffer (pH 6.0) and MgCl2 concentrations of 0.1 mM to 25 mM. Reactions were initiated by the addition of MgCl2. Aliquots of the reaction were removed at various time points and stopped by resuspension in the equal volume of stop solution (as above). Reactions for the pH-dependent activity profile were performed with 1 mM MgCl2 at a pH range of 5.25–8.75 using an appropriate buffer (either 30 mM MES, 30 mM HEPES, or 30 mM Tris). Reaction products were separated and imaged as described above.
Ligation assays. For the investigation of a cis-ligation reaction of the P. polymyxa pistol ribozyme, a ligation construct consisting of two cleavage products was designed according to Citation21 (see Supplementary Fig. 1). The 5ʹ-cleavage product was obtained from a transcription using [α-32P] ATP (Hartmann Analytik) for internal labelling and gel purified as described previously for the bimolecular construct of the cleavage reaction. The 3ʹ-cleavage product was chemically synthesized (Biomers). Both cleavage products were denatured at 70°C for 2 min in HEPES (pH 7.5) to ensure correct RNA folding [Citation22]. After cooling to room temperature, ligation reactions were initiated by the addition of MgCl2. Final concentrations of the reaction components were as follows: 200 nM 5ʹ-cleavage product, 10 µM 3ʹ-cleavage product, 30 mM HEPES (pH 7.5) and 5 mM MgCl2. 15% PEG8000 was present in the reaction mixture to stabilize the ribozyme active conformation, and therefore, improve the assay quantification [Citation23]. After different incubation times, aliquots of 10 µl were removed and quenched with an equal volume of stop solution (as above). Ligation products were resolved by denaturing 10% PAGE and visualized as described above.
Determination of rate constants for cleavage and ligation. After separation of reaction products, cleavage bands were quantified using ImageQuant software (Molecular Dynamics). In case of the cleavage reaction, apparent first-order rate constants were determined by linear curve fitting with Excel (Microsoft) using the following equation,
where fS is the remaining fraction of uncleaved substrate at a specific time point, normalized to the final extent of cleavage, t is the time, f0 is the fraction at t = 0, and kobs is the apparent first-order rate constant.
For quantification of the ligation reaction, the observed data were fitted to the same equation used for cleavage assays, with a few differences noted below,
where flig is the fraction ligated at a specific time point, normalized to the final extent of ligation, t is the time, f0 is the fraction at t = 0, and kobs is the apparent first-order rate constant.
To compare the pistol ribozyme cleavage and ligation activities, individual forward and reverse rate constants, k2 and k−2, were calculated using the following equations,
where kobs is the apparent first-order rate constant, which describes the approach to equilibrium and is, therefore, expected to reach the same value for the cleavage and ligation reaction, and feq is the fraction of uncleaved substrate/full-length ribozyme at equilibrium, which is also expected to be equal for the cleavage and ligation reactions. The internal equilibrium constant, Keqint, was calculated using
Results and discussion
Pistol ribozyme from Paenibacillus polymyxa cleaves in vitro
The biological roles of self-cleaving ribozymes in bacteria – with the exception of the glmS ribozyme – are completely unknown [Citation10]. We chose the bacterial strain Paenibacillus polymyxa ATCC 842, whose genome contains a pistol ribozyme, to initiate our investigation into the biological functions of this ribozyme class in bacteria. Paenibacillus polymyxa is a Gram-positive soil bacterium that promotes plant growth by fixing nitrogen, producing antimicrobial compounds and secreting hydrolytic enzymes [Citation24]. More importantly, P. polymyxa, which is a facultatively anaerobic species, can be easily cultured in the laboratory and is genetically tractable with the complete genome sequence being available [Citation25]. Moreover, efficient protocols for the generation of P. polymyxa knockout strains have already been developed [Citation26]. These are important factors to consider when choosing an organism for future functional studies.
Prior to an investigation of biological functions of the pistol ribozyme class in bacteria, we investigated the biochemical characteristics of the pistol ribozyme from P. polymyxa in the present study. We amplified the pistol ribozyme sequence from the genome of P. polymyxa ATCC 842, which matched consensus sequence requirements for the pistol ribozyme () [Citation12]. It should be mentioned that in a previous biochemical investigation of the pistol ribozyme [Citation14], the same representative from P. polymyxa ATCC 842 was tested for general cleavage activity as bimolecular construct. Our investigation widens the scope of existing knowledge, confirming the P. polymyxa pistol ribozyme activity in cis () and presenting the results of additional cleavage and ligation assays, discussed below.
To provide evidence for the catalytic activity of the bioinformatically predicted P. polymyxa pistol ribozyme, in vitro transcriptions using wild-type (WT) or mutant (MT) DNA templates were performed. According to the obtained results, the investigated pistol RNA appeared to perform a co-transcriptional self-cleavage reaction, whose 5ʹ- and 3ʹ-cleavage products were detected by PAGE (). Additional bands corresponding to longer or shorter 3ʹ-cleavage products are visible, probably because of the ability of the T7 RNA polymerase to add additional nucleotides at the 3ʹ-end of the transcript or terminate the reaction prematurely [Citation27]. In contrast, in vitro transcription of the DNA template with mutations of highly conserved nucleotides resulted in formation of a product, whose length matched that of the full-length ribozyme (). These results are consistent with the previous findings about sequence requirements of the pistol self-cleavage reaction [Citation14].
Generation of the pistol ribozyme bimolecular construct for cleavage
To perform a biochemical analysis of the P. polymyxa pistol ribozyme in vitro, a bimolecular construct was generated (, Supplementary Fig. 1A). In contrast to the previous assay (), where cleavage activity of the pistol ribozyme was tested using the cis – format, the bimolecular construct acts in trans (). Single-turnover conditions are ensured by adding the enzyme in excess (see Materials and methods for details). The substrate strand was cleaved only in the presence of Mg2+ and enzyme strand (). It should also be noted that we initially used KCl as a reaction component, which is thought to promote correct folding of the ribozyme. In this case, the cleavage, although not as prominent as with MgCl2, was possible in the absence of MgCl2 (Supplementary Fig. 1B). This result was verified in an assay with monovalent ions, which will be discussed in the next section.
Effects of metal ions on pistol ribozyme cleavage
Since the discovery of the first catalytic RNAs, one of the most widely discussed questions has been the possible catalytic mechanism of these molecules. Answering it appears especially challenging when taking into account the scarcity of RNA functional groups available for catalysis in comparison to proteins. For this reason, ribozymes were initially thought to be metalloenzymes rather than exploit some of their own functional groups in catalysis [Citation28]. In general, metal ions are known to fulfil two major functions in ribozymes: they can support folding of the RNA, or play catalytic roles [Citation29]. To investigate the effect of metal ions on the P. polymyxa pistol ribozyme cleavage, various divalent and monovalent ions were tested in the bimolecular format ().
Figure 2. Metal ion dependency of the P. polymyxa pistol ribozyme cleavage. (A) Pistol ribozyme cleavage assays with the P. polymyxa bimolecular construct incubated for 30 min in the absence (-) or presence of various 1 mM divalent metal ions. (B) Dependence of the P. polymyxa pistol ribozyme cleavage rate on the pKa of the hydrated divalent metal ion. Six divalent metal ions at the concentration of 1 mM, pH 6.0 and 2 M NaCl [reaction conditions according to Citation18] were tested. pKa values are taken from Citation30. (C) Reactions of the P. polymyxa bimolecular construct in the absence (-) or presence (+) of 5 mM cobalt hexammine chloride [Co(NH3)6Cl3] or MgCl2 for 60 min in the presence of 5 mM EDTA. (D) Pistol ribozyme cleavage assays with the P. polymyxa bimolecular construct incubated for 60 min in the absence (-) or presence of various 1 M monovalent metal ions. To chelate contaminating divalent metal ions, 30 mM EDTA was added to these reactions. For the positive control (PC), 25 mM MgCl2 was added
![Figure 2. Metal ion dependency of the P. polymyxa pistol ribozyme cleavage. (A) Pistol ribozyme cleavage assays with the P. polymyxa bimolecular construct incubated for 30 min in the absence (-) or presence of various 1 mM divalent metal ions. (B) Dependence of the P. polymyxa pistol ribozyme cleavage rate on the pKa of the hydrated divalent metal ion. Six divalent metal ions at the concentration of 1 mM, pH 6.0 and 2 M NaCl [reaction conditions according to Citation18] were tested. pKa values are taken from Citation30. (C) Reactions of the P. polymyxa bimolecular construct in the absence (-) or presence (+) of 5 mM cobalt hexammine chloride [Co(NH3)6Cl3] or MgCl2 for 60 min in the presence of 5 mM EDTA. (D) Pistol ribozyme cleavage assays with the P. polymyxa bimolecular construct incubated for 60 min in the absence (-) or presence of various 1 M monovalent metal ions. To chelate contaminating divalent metal ions, 30 mM EDTA was added to these reactions. For the positive control (PC), 25 mM MgCl2 was added](/cms/asset/1963f7d9-6403-4b4d-9c75-b00e4e0d2230/krnb_a_1874706_f0002_b.gif)
All tested divalent metal ions support cleavage of the P. polymyxa pistol ribozyme at a concentration of 1 mM, although to a different extent (). To explain the relationship between ribozyme activity and identity of the divalent metal ion, we performed kinetic assays. For these, reaction conditions according to Citation18, were used (see Materials and methods for details), where a clear relationship between the cleavage rate and pKa of the hydrated metal ion was detected for another pistol ribozyme example. In this kinetic analysis (), we omitted Cd2+ because it appeared to provide the weakest support for the reaction at the tested concentration of 1 mM (). Possible implications of the lower ribozyme activity in the presence of Cd2+ will be discussed below.
In our kinetic analysis of the effect of divalent metal ions on pistol ribozyme cleavage, a relationship between the cleavage rate and pKa of the hydrated metal ion was detected (R2 = 0.94) (; Supplementary Table 2). This dependency might be interpreted as biochemical evidence for the hypothesis that divalent metal ions play the role of a general acid in the pistol ribozyme cleavage mechanism [Citation17–20]. Lower pKa values lead to an increase in activity of the general acid, and therefore, higher cleavage rates could be reached by the examined ribozyme. Additionally, relationships between the ribozyme cleavage rate and radius of the divalent metal ion, as well as dependency of the ribozyme cleavage activity on the absolute hardness of the divalent cation were plotted (Supplementary Fig. 2; Supplementary Table 2). Except for Co2+, cleavage rates increase with the decreasing radius of the divalent metal ion (Supplementary Fig. 2A), whereas in case of the absolute hardness, higher cleavage rates were detected in the presence of Co2+, Ni2+ and Mn2+, which are the softest metal ions tested (Supplementary Fig. 2B). This observation seems quite plausible, if we assume that divalent metal ions are coordinated to the N7 position of a purine nucleobase, which has increased affinity for soft versus hard ligands [Citation30]. Interestingly, a different sequence of divalent metal ions in correlation with ionic radius based on the ribozyme cleavage rate was obtained, when monovalent ions were omitted in the reaction mixture (Supplementary Fig. 2C; Supplementary Table 3). A possible explanation could lie in an increased population of structurally stabilized P. polymyxa pistol ribozymes in the presence of high concentrations of monovalent metal ions, which putatively leads to a more efficient catalysis by divalent metal ions. Therefore, a correlation between the ribozyme cleavage rate and pKa of a hydrated metal ion could be detected only in the presence of high amounts of the monovalent salt. To sum up, the above made assumptions open new possibilities for additional research on the pistol ribozyme cleavage activity in the presence of different divalent metal ions.
As already mentioned, Cd2+ was excluded from the kinetic analysis because this ion provided the weakest support for cleavage in comparison with other divalent metal ions. Because the ionic radius of Cd2+ is between Co2+ and Ca2+, which each support cleavage (), we investigated if Cd2+ has an inhibitory effect on the P. polymyxa pistol ribozyme cleavage. In an assay, where Cd2+ alone (Supplementary Fig. 3A) or in combination with 0.5 mM Mg2+ (Supplementary Fig. 3B) was present in the reaction mixture, ribozyme cleavage was inhibited by Cd2+ concentrations above 0.5 mM. This could indicate that Cd2+ might dislodge Mg2+ from its binding pocket, thus inhibiting the cleavage activity, or occupy a different binding site and disrupt the active ribozyme structure. It is worth mentioning that inhibition of ribozyme activity by Cd2+ is specific for this pistol representative. When cleavage in the presence of various divalent metal ions was investigated for the L. sphaericus pistol ribozyme [Citation14], no significant differences between cleavage with Cd2+ and other metal ions, except for Zn2+ and Cu2+, were detected. To our knowledge, Cd2+ ions have not been described as having a negative effect on RNA structure or catalysis. For instance, the Schistosoma hammerhead ribozyme cleaves with highest rates in the presence of Mn2+, Co2+, Cd2+ and Zn2+ [Citation31]. Therefore, solving the crystal structure of the P. polymyxa pistol ribozyme could aid in our understanding of the effect of metal ions, particularly that of Cd2+, on the ribozyme cleavage mechanism.
As was detected in the divalent metal ion assay (), Mg2+ is not the only divalent cation which promotes the cleavage reaction. Still, it is possible that inner-sphere contacts between a divalent metal ion and RNA are essential for optimal activity. For this reason, the effects of cobalt hexammine on the P. polymyxa pistol ribozyme cleavage were assessed (). Cobalt hexammine mimics fully hydrated Mg2+, but cannot substitute for Mg2+ ions which are inner-sphere coordinated to RNA [Citation32]. Moreover, cobalt hexammine does not readily participate in proton transfer reactions [Citation33]. We found that the bimolecular P. polymyxa ribozyme construct is only modestly active in the presence of 5 mM cobalt hexammine. These results suggest that inner-sphere contacts with Mg2+ ions are not absolutely required for optimal ribozyme activity, which was previously reported for the pistol ribozyme from L. sphaericus [Citation14]. At the same time, crystal structures of the pistol ribozyme contained a hydrated divalent cation, directly coordinating the G33:N7 position [Citation15,Citation16,Citation18], which was confirmed by atomic-mutagenesis experiments [Citation20] and molecular simulation [Citation19]. Moreover, hydrated Mg2+ at this position was suggested to play the catalytic role of a general acid [Citation17–20]. Based on these results, it seems plausible that the examined pistol ribozyme exhibits a much weaker cleavage activity in the presence of cobalt hexammine, as the general acid element of catalysis in form of an optimally positioned hydrated divalent metal ion is excluded. In other words, a hydrated Mg2+ ion, which is inner-sphere coordinated to the G33:N7 position, seems to be important for optimal ribozyme cleavage activity. Another possible explanation regarding a lower cleavage rate in the presence of cobalt hexammine lies in the recruitment of a less suitable 2ʹ-OH of purine 32 as a general acid [Citation19], which was initially speculated to play this role [Citation15,Citation16]. In the presence of Mg2+ this functional group is predicted to increase the acidity of Mg2+ bound water [Citation19].
The P. polymyxa pistol ribozyme cleavage is also supported by monovalent ions (), although this support is much weaker than with most divalent cations. The lower fraction of cleaved substrate in the presence of monovalent ions might be explained with the lower charge density of monovalent ions in comparison with divalent cations, which, in turn, could mean a lower structural stability of the ribozyme [Citation34]. Ribozyme activity decreases with the increasing ionic radius (). This dependency of the ribozyme cleavage on the radius of the monovalent metal ion suggests that the corresponding binding site might have specific size requirements.
Kinetic characteristics of the pistol ribozyme cleavage activity
Next, we tested the effect of various Mg2+ concentrations (; Supplementary Table 4) and pH values (; Supplementary Table 5) on the P. polymyxa pistol ribozyme cleavage using the bimolecular construct. To quantify the obtained results, the value of the apparent first-order rate constant was determined by monitoring the progress of the substrate cleavage over time (see Materials and methods for details).
Figure 3. Kinetic characteristics of the P. polymyxa pistol ribozyme cleavage. (A) Effect of Mg2+ on the rate of P. polymyxa pistol ribozyme cleavage. The log-log plot of kobs values at pH 6.0 versus MgCl2 concentrations ranging from 0.1 mM to 25 mM is shown. (B) Effect of pH on the rate of P. polymyxa pistol ribozyme cleavage. The log-log plot of kobs values at the MgCl2 concentration of 1 mM versus pH values ranging from 5.25 to 8.75 is depicted
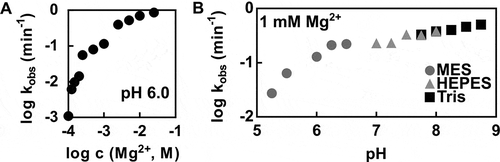
The effect of Mg2+ on the pistol ribozyme cleavage is illustrated in . In general, the ribozyme cleavage rate increases with higher Mg2+ concentrations. At low Mg2+ concentrations, the cleavage rate increases with a steep slope of ~4, suggesting that more than one metal ion is necessary for optimal ribozyme folding and activity. At higher values of Mg2+ concentration, including the physiological value of 1 mM Mg2+, the increase of cleavage rate is more gradual with the slope of ~1. Therefore, it might be possible that the examined pistol ribozyme reaches near-maximal reaction rates at physiological reaction conditions.
The P. polymyxa pistol ribozyme cleavage depends on the pH value of the reaction as well (). First, increasing pH values are associated with increasing cleavage rate, until the slope begins to level off around pH 6.5. The initial increase of cleavage rate between pH values of 5.25 and 6.5 with the slope of ~1 might be interpreted as one ionization event required for the ribozyme cleavage activity with the apparent pKa value of around 6.5. Different scenarios might be responsible for the detected relationship between the ribozyme cleavage rate and pH value. For instance, various ionizable groups not directly involved in the cleavage mechanism might have an influence on the pH-rate profile. Another factor to be considered is the shift of pKa values depending on the local RNA environment [Citation34]. That is why, a further discussion on functional groups, which might be responsible for the shape of the pH-rate profile, is difficult without resolving the crystal structure of the examined ribozyme. Nevertheless, cleavage rates under physiological (1 mM MgCl2, pH 7.5) and optimal (>50 mM MgCl2, pH 7.5–9.0) reaction conditions provide additional kinetic characteristics of the P. polymyxa pistol ribozyme, and were estimated to be 0.3 min−1 and >6 min−1, respectively. Based on these values, the pistol ribozyme from our study is considerably slower than the representative from L. sphaericus characterized by Citation14 . The L. sphaericus pistol ribozyme was predicted to accomplish more than 10 cleavages per minute under simulated physiological conditions and >100 min−1 under optimal reaction conditions. One possible explanation for such differences in reaction rates between representatives of the same ribozyme class might be that the P. polymyxa pistol ribozyme activity is additionally regulated in vivo, for instance, with the help of RNA-binding proteins or cofactors [Citation13, Citation35].
Ligation activity of the pistol ribozyme in comparison with the nucleolytic activity
Self-cleaving ribozymes have been known for their phosphodiester backbone cleavage activity. However, if the products do not immediately dissociate, the reverse reaction can occur. Early examples of ribozymes that are able to ligate their cleavage products include the hairpin [Citation36,Citation37] and hammerhead ribozymes [Citation22,Citation38]. We therefore analysed the pistol ribozyme ligation kinetics, and whether the cleavage activity dominates at equilibrium (, Supplementary Fig. 1C, Supplementary Table 6, and see Materials and methods).
Figure 4. Ligation activity of the P. polymyxa pistol ribozyme. (A) Kinetic assay using the P. polymyxa bimolecular ribozyme construct for cleavage (see Materials and methods for details) at the MgCl2 concentration of 5 mM and 30 mM Tris (pH 7.5). The corresponding time points are indicated. The cleavage products were separated by denaturing 20% PAGE. The full-length substrate (sub) and 5ʹ-cleavage product (5ʹ clv) are denoted. (B) Kinetic ligation assay of the P. polymyxa pistol ribozyme at the MgCl2 concentration of 5 mM and 30 mM HEPES (pH 7.5). 15% PEG8000 was used as an additive to improve the assay quantification. The corresponding time points are indicated. The ligation products were separated by denaturing 10% PAGE. The full-length ribozyme (FL) and 5ʹ-cleavage product (5ʹ clv) are denoted. As a positive control, hot transcription of the wild-type (WT) and mutant (MT) P. polymyxa pistol ribozyme was performed. (C) Comparison of the cleavage and ligation activities of the P. polymyxa pistol ribozyme. Approach to equilibrium at 5 mM Mg2+ and pH 7.5 is shown. The dashed line represents the mean value of the fraction of the full-length ribozyme (ligation) or uncleaved substrate (cleavage) at equilibrium, feq = 0.07. Open grey circles indicate cleavage of the ligated ribozyme, which was detected during the ligation assay. The inset shows a zoom-in of the region between 0 min and 5 min of the ligation reaction
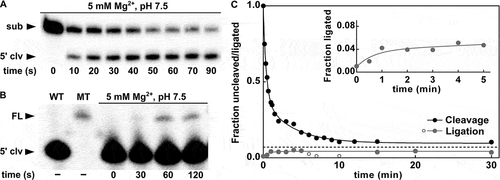
In ligation assays, we determined the kobs value of 1.39 min−1, which is the same as the kobs value for the cleavage reaction (, B). The fact that both kobs values for cleavage and ligation reach the same value means that the kobs is not describing the extent of cleavage or ligation, but the rate at which these competing reactions reach equilibrium [Citation39]. To characterize the P. polymyxa pistol ribozyme at equilibrium and directly compare its cleavage and ligation activities, k2 and k−2 rate constants for the forward (cleavage) and reverse (ligation) reactions were determined and reached 1.35 min−1 and at least 0.04 min−1, respectively (). We estimated the ligation rate constant to be at least 0.04 min−1, as the hybridization of the 3ʹ-cleavage product via four nucleotides may not provide long-term stability of the ligation complex. Nevertheless, this interaction between 5ʹ- and 3ʹ-cleavage products, however short-lived, was sufficient to observe the ligation activity with the above-mentioned rate. Although we could enhance the interaction between cleavage products through artificial extension of the P2 stem, such structural changes may have a negative effect on the ribozyme activity and, what is even more important, do not represent any natural example of a pistol ribozyme. Therefore, ligation experiments with an extended P2 stem cannot help us to understand the significance of ligation for the biology of the P. polymyxa pistol ribozyme. Taking all this into account, we conclude that the investigated pistol ribozyme is a no more than 30-fold better nuclease than ligase, with an internal equilibrium rate constant of 33.7. This might imply that the ligation activity is not of primary importance for the biological functions of pistol ribozymes in bacteria.
Although the P. polymyxa pistol ribozyme was shown to be a less efficient ligase, other self-cleaving ribozymes with even lower ligation rates have been reported. For instance, the hammerhead ribozyme construct, termed HH16, exhibits ligation rates of 0.008 min−1 [Citation38]. Consequently, in comparison with the HH16 ribozyme, the P. polymyxa pistol ribozyme is a more efficient ligase with a fivefold higher value of the ligation rate. The difference in ligation rates of these ribozymes might be even higher, as the HH16 was tested at 10 mM MgCl2, whereas for the examined pistol ribozyme, a concentration of 5 mM was used. It would be interesting to explain the preference of the P. polymyxa pistol ribozyme for the forward reaction. A possible explanation could be based on the results of a recent structure–function study, where the resulting cyclic phosphate product was proposed to be stabilized with the help of a narrowed hydrogen-bond network [Citation17].
Possible catalytic strategies of the pistol ribozyme
Four major catalytic strategies have been known to promote RNA cleavage via internal phosphoester transfer mechanism [Citation40,Citation41]. These strategies include facilitation of in-line attack (α), stabilization of the transition state (β), deprotonation of the nucleophile (γ), and protonation of the oxyanion leaving group (δ). With the help of the available structural and mutagenesis data, evidence has been provided that representatives of the pistol ribozyme class exploit all above-mentioned catalytic strategies [Citation18,Citation20]. Moreover, additional catalytic strategies, γ’ and γ’’, which lead to activation of the nucleophile through its acidification, were shown to facilitate the pistol ribozyme cleavage as well. In case of the γ’ strategy, the acidification is reached through donation of a hydrogen bond to the nucleophile. The γ’’ strategy provides the same effect through competitive hydrogen bonding to the non-bridging oxygen atom of the scissile phosphate, which prevents an inhibitory 2ʹ-OH to pro-RP interaction [Citation42]. According to the most recent ontology, γ’ and γ’’ strategies are referred to as secondary (2°) and tertiary (3°) γ catalysis, respectively [Citation43,Citation44].
The reaction rate of the P. polymyxa pistol ribozyme under optimal reaction conditions, determined here, exceeds the theoretical αγ speed limit of ~2 min−1 [Citation41]. Therefore, it is likely that other catalytic strategies, in addition to α and γ, or a completely different combination of the above-mentioned strategies are implemented by the examined pistol ribozyme.
The γ and δ strategies constitute general acid-base catalysis. Based on the nature of the general base and acid, the pistol ribozyme has been placed into the G + M – group. This makes pistol the first ribozyme employing a hydrated metal ion as general acid (M) with guanine as a general base (G) [Citation45]. For the ligation reaction, the roles of the hydrated metal ion and guanine are reversed, so that a hydrated metal ion acts as a general base, and guanine plays the role of a general acid. Our experimental data provide additional evidence that the hydrated metal ion might play the role of a general acid in the cleavage mechanism (), thus supporting the above-mentioned classification of pistol ribozymes.
In summary, the biochemical characterization of the P. polymyxa pistol ribozyme from our study sheds light on its catalytic mechanism and highlights differences to other self-cleaving ribozyme classes. We not only verified the ribozyme activity in cis, but also provided additional evidence for a divalent metal ion acting as a general acid in the cleavage mechanism. Furthermore, the ligation activity of this ribozyme class was investigated for the first time and was shown to be almost negligible in comparison with its cleavage activity. This defines pistol ribozymes as more efficient nucleases and suggests that the cleavage reaction is more important for the biological roles these ribozymes serve in bacteria. Armed with these biochemical data, we are working on elucidating the biological function of these catalytic RNAs in P. polymyxa.
Supplemental Material
Download MS Word (563.8 KB)Acknowledgments
We would like to thank all members of the Weinberg Lab, especially Richard Friedrich and V. Janett Olzog, for helpful discussions and Mario Mörl and his lab for generous support. We are also grateful to Zasha Weinberg for critical reading of this manuscript. This work was funded by the Fond of the Chemical Industry e.V. and a grant by the German Research Foundation (DFG LU1889/4-1 to C.E.W.). C.E.W. is also supported by a grant from the Peter and Traudl Engelhorn Foundation.
Disclosure statement
No potential conflict of interest was reported by the authors.
The authors report no conflict of interest.
Supplemental material
Supplemental data for this article can be accessed here.
Supplementary material
Supplemental data for this article can be accessed here.
Additional information
Funding
References
- Benner SA, Ellington AD, Tauer A. Modern metabolism as a palimpsest of the RNA world. Proc Natl Acad Sci USA. 1989;86(18):7054–7058.
- Woese C. 1967. The genetic code: the molecular basis for genetic expression. New York: Harper & Row. Available online at: http://worldcatlibraries.org/wcpa/oclc/293697
- Orgel LE. Evolution of the genetic apparatus. J Mol Biol. 1968;38(3):381–393.
- Crick FHC. The origin of the genetic code. J Mol Biol. 1968;38(3):367–379.
- Kruger K, Grabowski PJ, Zaug AJ, et al. Self-splicing RNA: autoexcision and autocyclization of the ribosomal RNA intervening sequence of tetrahymena. Cell. 1982;31(1):147–157.
- Guerrier-Takada C, Gardiner K, Marsh T, et al. The RNA moiety of ribonuclease P is the catalytic subunit of the enzyme. Cell. 1983;35(3):849–857.
- Prody GA, Bakos JT, Buzayan JM, et al. Autolytic processing of dimeric plant virus satellite RNA. Science. 1986;231(4745):1577–1580.
- Jimenez RM, Polanco JA, Lupták A. Chemistry and Biology of Self-Cleaving Ribozymes. Trends Biochem Sci. 2015;40(11):648–661.
- Lee K-Y, Lee B-J. Structural and Biochemical Properties of Novel Self-Cleaving Ribozymes. Molecules. 2017;22(4):678.
- Weinberg CE, Weinberg Z, Hammann C. Novel ribozymes: discovery, catalytic mechanisms, and the quest to understand biological function. Nucleic Acids Res. 2019;47(18):9480–9494.
- Roth A, Weinberg Z, Chen AGY, et al. A widespread self-cleaving ribozyme class is revealed by bioinformatics. Nat Chem Biol. 2014;10(1):56–60.
- Weinberg Z, Kim PB, Chen TH, et al. New classes of self-cleaving ribozymes revealed by comparative genomics analysis. Nat Chem Biol. 2015;11(8):606–610.
- Winkler WC, Nahvi A, Roth A, et al. Control of gene expression by a natural metabolite-responsive ribozyme. Nature. 2004;428(6980):281–286.
- Harris KA, Lünse CE, Sanshu L, et al. Biochemical analysis of pistol self-cleaving ribozymes. RNA. 2015;21(11):1852–1858.
- Ren A, Vušurović N, Gebetsberger J et al. (2016): Pistol ribozyme adopts a pseudoknot fold facilitating site-specific in-line cleavage. In Nat Chem Biol 12 (9), pp. 702–708. .
- Nguyen LA, Wang J, Steitz TA. Crystal structure of Pistol, a class of self-cleaving ribozyme. Proc Natl Acad Sci USA. 2017;114(5):1021–1026.
- Teplova M, Falschlunger C, Krasheninina O, et al. Crucial roles of two hydrated mg 2+ ions in reaction catalysis of the pistol ribozyme. Angew Chem. 2020;132(7):2859–2865.
- Wilson TJ, Liu Y, Li N-S, et al. Comparison of the structures and mechanisms of the pistol and hammerhead ribozymes. J Am Chem Soc. 2019;141(19):7865–7875.
- Kostenbader K, York DM. Molecular simulations of the pistol ribozyme: unifying the interpretation of experimental data and establishing functional links with the hammerhead ribozyme. RNA. 2019;25(11):1439–1456.
- Neuner S, Falschlunger C, Fuchs E, et al. Atom-specific mutagenesis reveals structural and catalytic roles for an active-site adenosine and hydrated mg2+ in pistol ribozymes. Angew Chem Int Ed Eng. 2017;56(50):15954–15958.
- Esteban JA, Banerjee AR, Burke JM. Kinetic mechanism of the hairpin ribozyme. Identification and characterization of two nonexchangeable conformations. J Biol Chem. 1997;272(21):13629–13639.
- Canny MD, Jucker FM, Pardi A. Efficient ligation of the schistosoma hammerhead ribozyme. Biochemistry. 2007;46(12):3826–3834.
- Paudel BP, Rueda D. Molecular crowding accelerates ribozyme docking and catalysis. J Am Chem Soc. 2014;136(48):16700–16703.
- Raza W, Yang W (2008): Paenibacillus polymyxa: antibiotics, hydrolytic enzymes and hazard assessment. In Int J Plant Pathol. 90 (3). Available online at https://www.researchgate.net/publication/238787533_Paenibacillus_polymyxa_Antibiotics_hydrolytic_enzymes_and_hazard_assessment.
- Jeong H, Park S-Y, Chung W-H, et al. Draft genome sequence of the paenibacillus polymyxa type strain (ATCC 842T), a plant growth-promoting bacterium. J Bacteriol. 2011;193(18):5026–5027.
- Kim S-B, Timmusk S. A simplified method for gene knockout and direct screening of recombinant clones for application in paenibacillus polymyxa. PloS One. 2013;8(6):e68092.
- Milligan JF, Uhlenbeck OC. Synthesis of small RNAs using T7 RNA polymerase. In: Dahlberg JE, Abelson JN, editors. Methods in enzymology. Vol. 180. Elsevier (Methods in Enzymology, 180); 1989. p. 51–62.
- Pyle AM. Ribozymes: a distinct class of metalloenzymes. Science. 1993;261(5122):709–714.
- Fedor MJ. The role of metal ions in RNA catalysis. Curr Opin Struct Biol. 2002;12(3):289–295.
- Feig A, Uhlenbeck O (1999): 12 The Role of Metal Ions in RNA Biochemistry. Accessed 28 Mar 2020. Available online at https://www.semanticscholar.org/paper/12-The-Role-of-Metal-Ions-in-RNA-Biochemistry-Feig-Uhlenbeck/0725633aac73939c935a328c5bbf89bd1dcde5d1.
- Boots JL, Canny MD, Azimi E, et al. Metal ion specificities for folding and cleavage activity in the schistosoma hammerhead ribozyme. RNA. 2008;14(10):2212–2222.
- Cowan JA. Metallobiochemistry of RNA. Co(NH3)63+ as a probe for Mg2+(aq) binding sites. J Inorg Biochem. 1993;49(3):171–175.
- Suga H, Cowan JA, Szostak JW. Unusual metal ion catalysis in an acyl-transferase ribozyme. Biochemistry. 1998;37(28):10118–10125.
- Luke WW, Plakos K, Derose VJ. Nucleic acid catalysis: metals, nucleobases, and other cofactors. Chem Rev. 2014;114(8):4318–4342.
- Cervera A, Marcos de LP. Eukaryotic penelope-like retroelements encode hammerhead ribozyme motifs. Mol Biol Evol. 2014;31(11):2941–2947.
- Feldstein PA, Bruening G. Catalytically active geometry in the reversible circularization of ‘mini-monomer’ RNAs derived from the complementary strand of tobacco ringspot virus satellite RNA. Nucleic Acids Res. 1993;21(8):1991–1998.
- Komatsu Y, Koizumi M, Sekiguchi A, et al. Cross-ligation and exchange reactions catalyzed by hairpin ribozymes. Nucleic Acids Res. 1993;21(2):185–190.
- Hertel KJ, Herschlag D, Uhlenbeck OC. A kinetic and thermodynamic framework for the hammerhead ribozyme reaction. Biochemistry. 1994;33(11):3374–3385.
- Nelson JA, Shepotinovskaya I, Uhlenbeck OC. Hammerheads derived from sTRSV show enhanced cleavage and ligation rate constants. Biochemistry. 2005;44(44):14577–14585.
- Breaker RR, Emilsson GM, Lazarev D, et al. A common speed limit for RNA-cleaving ribozymes and deoxyribozymes. RNA. 2003;9(8):949–957.
- Emilsson GM, Nakamura S, Roth A, et al. Ribozyme speed limits. RNA. 2003;9(8):907–918.
- Seith DD, Bingaman JL, Veenis AJ, et al. Elucidation of catalytic strategies of small nucleolytic ribozymes from comparative analysis of active sites. ACS Catal. 2018;8(1):314–327.
- Bevilacqua PC, Harris ME, Piccirilli JA, et al. An ontology for facilitating discussion of catalytic strategies of RNA-cleaving enzymes. ACS Chem Biol. 2019;14(6):1068–1076.
- Ganguly A, Weissman BP, Piccirilli JA, et al. Evidence for a catalytic strategy to promote nucleophile activation in metal-dependent RNA-cleaving ribozymes and 8-17 DNAzyme. ACS Catal. 2019;9(12):10612–10617.
- Lilley DMJ. Classification of the nucleolytic ribozymes based upon catalytic mechanism. F1000Res. 2019;8:1462.