ABSTRACT
Extracellular vesicles (EV), such as exosomes, are emerging biologic entities that mediate important newly recognized functional effects. Exosomes are intracellular endosome-originating, cell-secreted, small nano-size EV. They can transfer cargo molecules like miRNAs to act intracellularly in targeted acceptor cells, to then mediate epigenetic functional alterations. Exosomes among EV, are universal nanoparticles of life that are present across all species. Some critics mistakenly hold exosomes to concepts and standards of cells, whereas they are subcellular nanospheres that are a million times smaller, have neither nuclei nor mitochondria, are far less complex and currently cannot be studied deeply and elegantly by many and diverse technologies developed for cells over many years. There are important concerns about the seeming impossibility of biologically significant exosome transfers of very small amounts of miRNAs resulting in altered targeted cell functions. These hesitations are based on current canonical concepts developed for non-physiological application of miRNAs alone, or artificial non-quantitative genetic expression. Not considered is that the natural physiologic intercellular transit via exosomes can contribute numerous augmenting carrier effects to functional miRNA transfers. Some of these are particularly stimulated complex extracellular and intracellular physiologic processes activated in the exosome acceptor cells that can crucially influence the intracellular effects of the transferred miRNAs. These can lead to molecular chemical changes altering DNA expression for mediating functional changes of the targeted cells. Such exosome mediated molecular transfers of epigenetic functional alterations, are the most exciting and life-altering property that these nano EV bring to virtually all of biology and medicine. .
Abbreviations: Ab, Antibody Ag Antigen; APC, Antigen presenting cells; CS, contact sensitivity; DC, Dendritic cells; DTH, Delayed-type hypersensitivity; EV, extracellular vesicles; EV, Extracellular vesicle; FLC, Free light chains of antibodies; GI, gastrointestinal; IP, Intraperitoneal administration; IV, intravenous administration; OMV, Outer membrane vesicles released by bacteria; PE, Phos-phatidylethanolamine; PO, oral administration.
Introduction
There are vast numbers of total extracellular vesicles (EVs) and perhaps trillions of exosome subtypes and billions of EV like exosomes per millilitre of mammalian blood. Exosomes and other related EVs, occur in all species; down to and including bacteria. They participate in an ever increasingly identified vast array of biologic and clinical functions. It is proposed here that exosome transfers of small amounts of miRNAs, that seem impossible according to current concepts developed for miRNAs alone, are dependent on carrier effects of the exosome themselves that have not been considered previously.
Carrier effects of exosomes on their miRNA transferred biologic activities is the central point discussed here
We hold that exosome transfers of functioning polyribonucleotides like miRNAs, should in most instances not be considered just at the level of transfers of the RNAs alone. Instead, they should be considered in the context of what we call the ‘exosome carrier effects’. Thus far, small EV exosomes provide the dominant mechanisms for intercellular transfer of molecules like miRNAs. This compares to much less frequent reports of transfer of function by exosome-free RNA complexed with chaperones like Argonauts [Citation1,Citation2].
In this review, we will focus on the effects of exosome miRNA transfers to target cells, that often cause epigenetic changes to mediate resulting in functional alterations. We will especially focus on the controversial transfer of very small amounts of exosome miRNA. We point out that this can be ascribed to the carrier properties of the involved exosomes that are needed to therefore accomplish non-canonical mechanisms of miRNA transfers.
Carrier effects on intercellular miRNA transfers are both carrier-delivering and carrier-acting
There are in fact at least two broad areas subsumed under the umbrella term of ‘exosome carrier effects’. Firstly, there is are ‘carrier-delivering effects’ in the sense of exosomes actually carrying their contents to targeted cells. These are postulated effects on such acceptor cell delivery that are enabled by survival of exosomes produced under very harsh conditions not suitable for cells; including extracellular milieu like hypoxia and highly acidic and digestive enzyme environments like in the stomach. These are considered ‘carrier-delivering effects’, since without the resistance to harsh conditions the exosomes would not be able to deliver their miRNA.
Secondly there are exosome ‘carrier-acting effects’ on the miRNA molecular-acting intracellular transfers, often leading to epigenetic functional effects on the targeted cells; like altering DNA expression viva codon conjugations such as by methylation. These are hypothesized to participate in resulting special functional aspects of these especially hardy exosomes, enabling them to affect the target cells beyond just mere RNA molecular delivery. Thus, external cell to cell ‘carrier-delivering effects’ and intracellular ‘carrier-acting effects’ of exosome transfers are able to actively participate in the biologic intracellular events of transferred miRNAs in targeted cells.
Thus, these carrier-acting aspects are predicted to promote the transfer and subsequent molecular function of their delivered miRNA, and likely other cargo like proteins and lipids, on the targeted cells. This can account for unexpected greater effects of exosome low-level miRNA delivery and actions, that are not covered by established canonical miRNA-only concepts. Thus, exosome transfers of low amounts of miRNA, acting via both ‘carrier-delivery’ and ‘carrier-acting’ modes are the “Elephant in the Room because these exosome carrier effects have been overlooked by both the miRNA Cognoscente clinging to conventional miRNA transfer concepts not considering the effects of the exosomes on the targeted cells and the few remaining anti-exosome Luddites, who doubt the significance of EVs per se ().
Figure 1. Obvious carrier effects on intercellular miRNA transfers have been vastly overlooked and thus are ‘The Elephant in the Room’. The carrier-delivering and carrier-acting aspects of exosomal transfer of miRNAs to targeted cells are predicted to promote the transfer and subsequent molecular functions of their delivered miRNA on the targeted cells. This can account for unexpected greater effects of exosome miRNA delivery and subsequent intracellular actions, than conceptualized by established canonical concepts of miRNA of only direct addition to targeted cells or genetic expression of miRNAs. Thus, exosome transfers of what are considered low amounts of miRNA, acting via both ‘carrier-delivery’ and ‘carrier-acting’ modes are the ‘Elephant in the Room’. This is because these obvious exosome carrier effects have been overlooked by both the miRNA Cognoscente clinging to conventional miRNA transfer concepts and thus, not considered as crucial to miRNA transfers to acceptor cells, and by the few remaining anti-exosome Luddites, who doubt the significance of EV per se. These carrier ‘Elephant in the Room’ aspects of these issues should be obvious, but are overlooked because it makes some uncomfortable, who are clinging to conventional canonical concepts; as out of this box (non- canonical). The point is that something as conspicuous as an elephant can be overlooked amid codified canonical concepts of such interactions
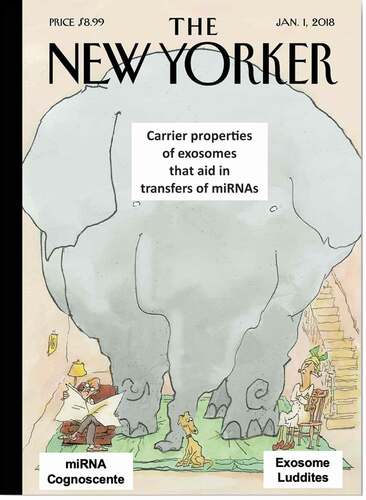
Terminology for of the various EV subsets like exosomes and micro vesicles
A major recognized broad subset of EV are microvesicles (MV) that originate by pinching off singly from the donor cell surface membrane resulting in release of individual vesicles. These large MV are 200–1500 nanometres in diameter, pellet with centrifugation at only 10,000 g, and thus far mediate a minority of EV transferred effects. In comparison, there are a broad, and very large group of many and multiple subsets that have been called classical exosomes or small EV, in comparison to MV [Citation3], that are responsible for the vast majority of transferred effects of EV. Exosomes are nanovesicles pinched off from intracellular endosome membranes that are 50–150 nanometres in diameter and pellet at 100,000 g. Their cargo can contain many subtypes of RNAs (miRNAs, mRNAs, lncRNAs, tRNAs, rRNA and tRNA fragments, etc.) [Citation4] and perhaps RNA-binding proteins as well [Citation5]. Also contained and potentially transferred to also mediate biologic effects are contained diverse lipids and a variety of bioactive proteins like enzymes and signalling molecules.
The RNAome of MVs can resemble the intracellular profile, whereas the exosome RNA profile is quite different from that of the donor cells; reflecting distinct intracellular sorting processes to be more fully unravelled. Prior to their release, increasing numbers of exosomes pinching off of the endosomal membranes are in expanding groups that accumulate in the progressively dilated multivesicular endosomal body (MVB) near the cell surface. They then are released extracellularly in bunches via compound sequential exocytosis of the MVB; and thus were named exosomes. Although it is thought that despite many overlaps between MV and exosomes, there should be distinguishing markers and properties between them. This has not emerged until a recent deeper study that fractionated vesicles by multiple means found that the surface molecule Annexin A-1 was characteristic of the MVs compared to classical exosome small EVs [Citation3].
There is great heterogeneity of exosomes and the possibility that no two are exactly alike; thus contributing to a potential great variety of carrier effects
As noted, exosomes and MV are quite separate in cell genesis and cell compartment of origin, but their surface molecule arrays and contained cargo can have great overlap. It is emerging that there is a continuum of many EV types [Citation6,Citation7]; with the largest peaking at MV, next classical small nano-sized exosomes, and then the smaller exomeres [Citation8]. It thus seems that there are millions of EV subsets [Citation3,Citation6,Citation7]. This brings up the possibility that in fact no two exosomes are precisely alike for the billions of subsets, analogous to what now seems true for cells considering recent findings employing single cell analysis [Citation9–11]. Currently there is no way to precisely examine EV separately, but there are emerging appropriate techniques; including flow cytometry [Citation12–16].
Thus, when one says the term exosomes, one is in fact speaking of a very diverse mixture of very great numbers; millions and billions of nanovesicles. Thus, current descriptive and phenotypic methods certainly cannot adequately describe their great individual diversity into undoubtedly many many subsets [Citation3,Citation6,Citation7]. Using current descriptive aspects of the vesicles, the exosome group account for most documented transfers of functional macromolecules like miRNAs [Citation17]; whereas transfers by MV are less common [Citation18–20], as are transferred by related microparticles [Citation21,Citation22]; and possibly even DNA transfers by the non-canonical autophagy-related amphosomes [Citation3], as well as the very small exomeres [Citation8,Citation23].
A very early evolutionary history of exosome origins in ‘the primordial soup’ may account for the carrier effects of their resistance to harsh conditions
Of great importance, exosomes likely are descendants of very ancient conserved nanoparticles that seemingly are fundamental to life [Citation24,Citation25]. Their carriage and transfers of miRNAs are hypothesized to likely have arisen amid the early events in the evolution of life [Citation26]. Thus, we propose that exosome antecedents preceded cells in evolution, as others have postulated [Citation25]. These ancient vesicles may have arisen among the ‘RNA world’ in the harsh conditions of the ‘primordial soup’. They resemble postulated early developing protocells that evolved to have bi-lamellar surface membranes containing RNA polynucleotides with enzymatic and self-replenishing properties [Citation26–28]. As a consequence of such postulated very early origin amid very noxious conditions, descendent current day exosomes have outstanding abilities to withstand very harsh conditions, that are not resisted by prokaryote and eukaryote cells, and can provide carrier effects for miRNA transfers. In this regard, it is important to note that some types of EV carrying proteins and RNAs exist in all species; completely across all phyla, from prokaryote bacterial outer membrane vesicles (OMV), to exosomes in archaea, fungi, worms, insects, plants and all vertebrates including mammals [Citation29].
Conservation of such resistance properties of EV protocells developed early in evolution are likely in part due to their unusual protective membranes, that consists of a unique phospholipid lipid composition [Citation30–35]. The specific lipid composition and tight lipid packing can vary by pH, featuring asymmetrical distribution of phosphatidylethanolamine, with a rapid exchange of lipids between the two membrane leaflets; distinguishing exosomes from cells and other types of vesicles [Citation32]. These unique membrane characteristics may be responsible for the outstanding ability of exosomes from activated cells and those present in mothers’ milk to resist very low pH, together in a strong mixture of digestive enzymes in the stomach, and similarly in the hypermetabolic hypoxic microenvironment of cancer tissues [Citation36].
Resistance of some exosomes to harsh conditions like hypoxia and acid/enzyme mixtures are important likely ancient carrier properties
Exosome aspects that likely contribute to carrier effects are those enabling their unusual abilities to withstand degeneration in toxic conditions without loss of contained RNAs. One example of the need for carrier effects on delivery of miRNAs is that there are many studies cells stimulated under hypoxic conditions that release exosomes resistant to hypoxia. This is pertinent to their ability to operate in the noxious microenvironment of cancers. Thus, local cancer hypoxia increase exosome release [Citation37], and these hypoxia resistant exosomes Darwinian select for enhanced tumour survival [Citation38,Citation39], aggressiveness, chemoresistance [Citation40] and invasiveness [Citation41]. Besides general tumour promotion [Citation42,Citation43], there is enhanced angiogenesis [Citation44–46], promotion of tumour favouring local M2-type macrophages [Citation47,Citation48] and even transfer of particular oncogenic miRNAs to convert normoxic cells towards malignancy [Citation49] and other sites of ischaemia and injury [Citation50]. In fact, because hypoxia promotes production of exosomes with superior activities [Citation39,Citation43], it is generally advised to use hypoxic conditions of culture when producing exosomes for study or for therapies. Further, there also is exosome resistance to hyperoxia [Citation51,Citation52].
Carrier effects of exosomes in dietary milk permit resistance to the profound acidic and digestive enzyme-rich environment of the stomach
Thus, we postulate that remarkable current day exosome hardiness is due to their evolutionarily descent from ancient resistant nanovesicles that evolved in the primordial conditions near to the origin of life. These properties can allow for resistance to unusual noxious conditions in the environment of exosome-producing or the receiving cells, and can serve for survival and function of exosomes in ingested foods, such as those in mothers’ milk. This constitutes both carrier-delivery and likely carrier-acting functions of exosomes contained in maternal milk, to likely promote eventual function of their contained miRNAs after transfers to developing targeted cells in neonate recipients.
Accordingly, it is well documented that maternal breast milk colostrum contains great numbers of exosomes that survive the acid digestion of the neonatal stomach to then act in development of various systems in the neonates. These can include: participation of the ingested exosomes and their contents in generation of the neonatal intestine [Citation53,Citation54], and development of the neural [Citation55,Citation56] and endocrine systems [Citation57–60], and most importantly the neonatal immune system; especially in relation to subsequent development of allergies. This can involve immune regulation [Citation61,Citation62], thymic regulatory T cell maturation preventing the development of atopy [Citation63,Citation64], and differences in human breast milk exosome populations in relation to allergic sensitization [Citation65], as well as content of immune-related microRNAs [Citation66], some identified as GI absorbed in from cows milk and able to affect gene expression in recipient peripheral blood mononuclear cells [Citation67]. Therefore, effects of maternal milk exosomes on generation of a mature immune system in neonates include development of immune tolerance to diverse foreign antigens (Ag) in the diet [Citation60,Citation65,Citation66,Citation68], and especially to Ag of the newly establishing neonatal microbiome [Citation69–71]. Interestingly this has recently been shown to include direct effects of the dietary milk exosomes on the microbiome itself [Citation72].
Therefore, milk exosomes are enriched in contents that are involved in neonatal development; including mothers milk from humans, and other species like cows and pigs [Citation57–60,Citation73,Citation74,Citation75,Citation76]. Thus, exosomes in milk [Citation59,Citation73,Citation77], and consequently their contained miRNAs [Citation77–79], are able to resist the very harsh conditions when passing the human neonatal stomach. There are many detailed studies confirming and extending these concepts by Hirschi [Citation80–84], Vance [Citation81], and Zempleni [Citation66,Citation78,Citation79,Citation85–88] on bovine milk exosomes or miRNAs. However, there are limits. Even though these milk exosomes can resist harsh stomach conditions as well as even boiling and multiple freeze thawing, they do not survive greater harsh treatments of milk like Pasteurization and homogenization [Citation88], as well as microwave cooking [Citation89] and fermentation [Citation90]. As a consequence, there are continually increasing studies elegantly confirming the presence of allogeneic milk miRNAs in the blood of humans ingesting milk. Such data now extend to additionally showing functional significance of these miRNAs [Citation63,Citation65–68,Citation78,Citation91–94]. Therefore, these new data confirm that development of various neonatal organ systems depends in part on consumption of exosome-rich mothers breast milk and thus on miRNA transfers that depend on the carrier functions of these exosomes [Citation60,Citation65,Citation75,Citation81,Citation95,Citation96].
There even are recent suggestions of possible therapeutic applications of these milk exosomes [Citation81,Citation84,Citation97–99], to make use of their carrier effects for delivery of drugs. This concept is increasingly being shown for xenogeneic exosomes derived from plants in the diet. These new data extend to demonstrating peripheral blood or tissue presence of miRNAs derived from ingested xenogeneic plants in humans and mice [Citation82,Citation84,Citation97,Citation100–103], and even in human breast milk after maternal plant digestion [Citation100,Citation104]. In some cases, the data confirm the concept of cross-kingdom and cross-species exchanges by additionally showing functional significance of the miRNAs from the ingested plants in the human recipients [Citation94,Citation98,Citation105–107], or orally ingested exosome carrier miRNAs [Citation108,Citation109]. These new, diverse and upgraded studies, favour the concept of allogeneic milk and even xenogeneic plant exosome cross kingdom mediated informational transfer across species via carrier-acting exosomes. As a result, the carrier effects of the involved exosomes that we postulate are a crucial contributor to enable the final transfer of exosomal miRNAs to achieve functional alterations in the targeted cells of neonatal recipients.
Our related immunology experiments on oral transfer of suppressive exosomes that can pass the stomach, to inhibit recipient T cell mediated skin responses in both an Ag and miRNA-specific manner
There are questions and controversies concerning the postulates above about systemic effects of maternal milk exosome RNAs transferred to neonates, that are thought to act on the development of various functional neonatal systems. Related to this area are our studies of exosome mediated immunoregulation of classical effector T cell mediated delayed-type hypersensitivity (DTH). Here, is a clear example of oral systemic transfer across the acid/enzyme stomach environment of exosome carrier-delivering and functional carrier-acting transfer of a specific miRNA type. The miRNA transferred is in remarkably small amounts systemically far from the intestinal site of absorption to mediate suppressive functions in the ear skin [Citation109] () [Citation108,Citation110] ().
Figure 2. A. Oral and other systemic routes of administration of CD8pos suppressor T cell-derived exosomes strongly inhibit cutaneous OVA protein-induced DTH in actively immunized hosts. The Figure shows that oral (PO) administration of a single physiological dose of suppressive exosomes (1010 vesicles) from CD8+ suppressor T cells from ovalbumin (OVA) protein antigen (Ag) immune high Ag dose tolerized mice are inhibitory of active T cell mediated delayed-type hypersensitivity (DTH) elicited by on day 4 after optimal immunization by intradermal OVA Ag injection of only five micrograms into the ears of murine hosts immunized with an optimal 100 µg dose of OVA intradermally. Further, this single systemic transferred physiological dose of Ag tolerance induced inhibitory exosomes, can last very significantly for at least 120 h (4 days), when such systemic treatment is begun just after the peak of the maximal elicited 24 h active ear swelling response of the recipients. The Figure shows that the strong and prolonged suppressive results of administered suppressive exosomes are similar among various conventional systemic treatment routes (IP, intraperitoneal, green line; IV, intravenous, blue line; and even ID, intradermal, black dashed line), that all very strongly inhibit elicited existing active DTH by 50–90% over the subsequent 4 days (120 h); with oral administration the most profoundly suppressive (red line). B. CASEIN milk protein-induced active in vivo elicited DTH, is suppressed by Casein-specific B1a B cell, miRNA-150-associated suppressor exosomes, given systemically by various routes, in a single physiological dose at the 24 h peak of response; lasts at least for 4 days; especially the most powerful inhibitory response to oral exosome administration. Suppression of delayed-type hypersensitivity (DTH) to soluble casein (Cas) Ag by miRNA-150- associated B1 B cell-derived small exosome EVs of Cas-Ag-immunized mice. For supernatant exosomes, mice had been intradermally (ID) immunized with saline solution of soluble Cas antigen 3 days before harvest of lymph nodes and spleens containing Cas-immune B1 B cells, that were cultured for a subsequent 48 h. The resulting supernatant was ultra-filtered and then ultracentrifuged to concentrate Cas-specific B1a B cell-derived exosome extracellular vesicles, that then were supplemented with miRNA-150 by association in vitro at 37° C with miRNA-150 and then washed free of unattached miRNA-150 by another round of 100,000 g ultracentrifugation. Mice that had been optimally ID immunized with saline solution of soluble Cas antigen (100 μg per mouse) 5 days before challenging by ID ear administration of the same Cas solution (at only 5 μg per ear lobe). Then, after measurement of the 24-h ear swelling responses, miRNA-150-supplemented, Cas-Ag specific B1a B cell-derived exosome EVs were administered either intraperitoneally (IP, purple line), ID (green line), intravenously (IV, orange line) or orally (PO, redline), and subsequent ear swelling responses were measured daily up to 120 h post ear challenge, and expressed as delta ± SEM [units (U) × 10−2 mm]; all compared to the control treated group (black line). All routes of administration were suppressive, but the oral route clearly was the most powerful
![Figure 2. A. Oral and other systemic routes of administration of CD8pos suppressor T cell-derived exosomes strongly inhibit cutaneous OVA protein-induced DTH in actively immunized hosts. The Figure shows that oral (PO) administration of a single physiological dose of suppressive exosomes (1010 vesicles) from CD8+ suppressor T cells from ovalbumin (OVA) protein antigen (Ag) immune high Ag dose tolerized mice are inhibitory of active T cell mediated delayed-type hypersensitivity (DTH) elicited by on day 4 after optimal immunization by intradermal OVA Ag injection of only five micrograms into the ears of murine hosts immunized with an optimal 100 µg dose of OVA intradermally. Further, this single systemic transferred physiological dose of Ag tolerance induced inhibitory exosomes, can last very significantly for at least 120 h (4 days), when such systemic treatment is begun just after the peak of the maximal elicited 24 h active ear swelling response of the recipients. The Figure shows that the strong and prolonged suppressive results of administered suppressive exosomes are similar among various conventional systemic treatment routes (IP, intraperitoneal, green line; IV, intravenous, blue line; and even ID, intradermal, black dashed line), that all very strongly inhibit elicited existing active DTH by 50–90% over the subsequent 4 days (120 h); with oral administration the most profoundly suppressive (red line). B. CASEIN milk protein-induced active in vivo elicited DTH, is suppressed by Casein-specific B1a B cell, miRNA-150-associated suppressor exosomes, given systemically by various routes, in a single physiological dose at the 24 h peak of response; lasts at least for 4 days; especially the most powerful inhibitory response to oral exosome administration. Suppression of delayed-type hypersensitivity (DTH) to soluble casein (Cas) Ag by miRNA-150- associated B1 B cell-derived small exosome EVs of Cas-Ag-immunized mice. For supernatant exosomes, mice had been intradermally (ID) immunized with saline solution of soluble Cas antigen 3 days before harvest of lymph nodes and spleens containing Cas-immune B1 B cells, that were cultured for a subsequent 48 h. The resulting supernatant was ultra-filtered and then ultracentrifuged to concentrate Cas-specific B1a B cell-derived exosome extracellular vesicles, that then were supplemented with miRNA-150 by association in vitro at 37° C with miRNA-150 and then washed free of unattached miRNA-150 by another round of 100,000 g ultracentrifugation. Mice that had been optimally ID immunized with saline solution of soluble Cas antigen (100 μg per mouse) 5 days before challenging by ID ear administration of the same Cas solution (at only 5 μg per ear lobe). Then, after measurement of the 24-h ear swelling responses, miRNA-150-supplemented, Cas-Ag specific B1a B cell-derived exosome EVs were administered either intraperitoneally (IP, purple line), ID (green line), intravenously (IV, orange line) or orally (PO, redline), and subsequent ear swelling responses were measured daily up to 120 h post ear challenge, and expressed as delta ± SEM [units (U) × 10−2 mm]; all compared to the control treated group (black line). All routes of administration were suppressive, but the oral route clearly was the most powerful](/cms/asset/0ccc3c98-7c8b-4729-ac0f-95e05bb3a0c1/krnb_a_1885189_f0002_c.jpg)
In these studies, we orally administered antibody (Ab) light chain-coated, and thus Ag-specific, CD8pos suppressor T cell-derived exosomes, that we show deliver inhibitory miRNA-150 to inhibit cutaneous elicited contact sensitivity and DTH in the ear skin of recipients [Citation108,Citation109]. In experiments that are notably relevant to this discussion, oral administration of these inhibitory exosomes was compared to conventional systemic intravenous (IV) and intraperitoneal (IP) treatment routes. The suppressor exosomes given by all three routes acted strongly to inhibit immune inflammatory DTH responses in the ear skin by 90–95%, over the succeeding 4 days, following a single physiological dose, that was given only at the peak of the in vivo response [Citation109] () [Citation108] (). Very importantly, in all instances oral exosome treatment was even more effective than the widely experimentally used, but less clinically applicable, IV or IP routes [Citation108,Citation109].
We later discuss our concept that such unusual biologic effects of exosomes, that allow them to withstand such harsh conditions, in order to mediate carrier effects, are a subpopulation that we call ‘activated’. They seem to be produced by activated cells and are thereby induced to have unusual hardiness properties. The resistance of such subpopulations of exosomes to harsh conditions are postulated to be due to special exosome membrane lipid changes [Citation30–35].
Thus, as in the neonate consuming exosomes in milk, the oral route for administration of a particularly resistant activated subset suppressor T cell-derived exosomes allows them to strongly inhibit cutaneous DTH. There is systemic distribution of these carrier-delivering and carrier-acting functional immunosuppressive exosomes. In this case, they are able to transmit miRNA-150 into distant targeted antigen presenting cells regulating effector T cells [Citation111] at the skin site of these immune responses. Accordingly, activation properties of an orally administered exosome subset enable carrier-delivery for stomach transit, and then ability to cross postulated specialized absorptive cells of the small intestine. This is followed by systemic distribution in the recipients, for exosome carrier-acting promotion of quite low-dose miRNA-150 transfers to targeted cells to functionally suppress cutaneous DTH responses [Citation108,Citation109,Citation111–113].
There is doubt of successful transfers by low levels of exosome miRNAs, that may be due to the accompanying exosome carrier-acting effects
There are important judgements by authoritative leaders in the RNA field, who conclude that exosome transfers of miRNAs are ineffective; especially the low doses. These critiques from the miRNA cognoscente are correct according to the established point of view generated by conventional experiments of just adding miRNA alone to cells or artificial genetic expression of a particular RNA. However, both of these conventional approaches of are non-physiological. Further, genetic expression via vector treatments can induce intracellular background alterations; often involving changes of endogenous miRNAs [Citation114–117]. Therefore, these current paradigms, that are relevant to miRNA levels and their function when transferred via exosomes, ignore the more physiological participation of exosomes in the delivery and subsequent intracellular carrier-mediated actions of the exosomes after uptake.
Current conventional canonical concepts, that these expert opinions are based on, are independent of any exosome carrier effects on miRNA transfers. They are based on high dose non-physiological experimental transfers of miRNA acting alone, or artificial gene expression to modulate expression of targeted cell genes via their seed sequence complementarity with the 3ʹ untranslated sequences of silenced intracellular cytoplasmic mRNAs. This inhibits gene translation, leading to functional consequences of decreased expression of specific DNA encoded proteins. However, these conclusions based on current canonical concepts do not consider the likely numerous physiological carrier-delivering effects on small amounts of transferred miRNAs, and also subsequent exosome carrier-acting effects on target cell activation via likely promotion of intracellular miRNA functional mechanisms of the delivered miRNA; possibly involving effects beyond mere binding to mRNAs.
Ingested small amounts of xenogeneic plant miRNAs exemplify potential carrier effects of exosome transfers in humans
As example, it is said to be impossible that the very low copy numbers of miRNA transferred across species, say by plants in the diet [Citation118], are able to mediate function. This view is correct according to established data analysis and canonical concepts, but as noted above do not consider the exosome carrier aspects. A prescient initial study in this cross-kingdom transfer area, dealt with xenogeneic dietary plant miRNAs in humans. It was reported that plant (rice) miRNA in the high rice diet of Chinese subjects transferred biologic function in humans [Citation118]. Confounding to some investigators was a very large range of plant-derived miRNAs found in the blood of subjects by an assay that could detect as little as femtomoles (10−15 M) of miRNA [Citation118].
Crucial here was RNA measurements of the total small RNA isolated from human serum after treatment with the oxidizing agent sodium periodate. This is because plant miRNAs are 2′-O-methyl modified on their terminal nucleotide, that renders them resistant to periodate compared to mammalian miRNAs that have free terminal nucleotide sites sensitive to periodate. Further, the data were interpreted by the authors as showing that on dietary ingestion, these particular cross kingdom plant miRNAs entered the human GI tract that produced miRNA-carrying exosomes that then pass into the host systemically, to then be transmitted via the blood to subsequently target liver hepatocytes to favourably influence lipid metabolism [Citation118]. Specifically, a host mRNA transcript encoding a lipid-associated receptor protein in the liver, had an 3ʹ untranslated sequence targeted by the exosome transferred plant miRNA.
Quantitation of the involved exosome transferred plant miRNA was at only femtomole amounts (10−15). This very small, transferred amount of miRNA was found sufficient to act on the calculated copy number of target mRNA in the liver [Citation118]. Overall, this was said to be the first demonstration of potential cross animal kingdom transfer of miRNA functions; here from plants to people involving carrier function of exosomes. Since then there have been many other examples of such cross-kingdom-acting exosomes and their miRNAs mediating such carrier-aided transfers in variety of instances. These have been interactions between nematode [Citation119] and protozoan [Citation104,Citation120] parasites and their mammalian hosts, fungi parasitizing plants [Citation121–123], parasitic plants and hosts [Citation124], as well as between ticks and arthropod hosts [Citation125]. Improved detection of plant miRNAs in mammalian hosts [Citation110,Citation126,Citation127] has allowed demonstration of several more instances of plant exosome carried cross-kingdom transferred regulatory miRNAs [Citation103,Citation128–130].
These recent additional studies confirmed this concept; showing exosome and miRNA function in such interspecies transfers in a variety of systems [Citation103,Citation123,Citation124,Citation129–134]. One such study showed that miRNAs mediate plant–plant interactions; silencing host genes to facilitate parasitic plant growth [Citation124], but mediation via exosomes was not evaluated. However, another study of plant–fungal microRNA interactions suggested that plant exosome-like EV transport small RNAs into a fungal pathogen that suppresses its pathogenicity by silencing fungal virulence genes [Citation124]. In yet another such instance in mice, plant exosomes shaped the GI microbiome with preferential uptake by Lactobacillus rhamnosus, leading to host IL-22 production that improved barrier function in a model of colitis [Citation123].
Low quantitative miRNA copy number issues are thought to make exosome functional transfers doubtful
Relevant here is a widely quoted quantitative analysis of miRNA abundance in exosomes, mentioned previously [Citation135]. Human body fluids and various cell supernatants were studied by excellent quantitative assays and found on average to have only one copy of miRNA per exosome [Citation135]. Therefore, a particular given miRNA, on average is in a distinct minority of exosomes. Even for the most abundant of miRNAs, across six different cell sources of exosomes; showed as few as 1 molecule per 10,000 exosomes. Considering functional transfers, this is quite daunting, since if miRNAs were distributed homogenously across an exosome population, then on average, more than 10,000 (104) exosomes would be needed for delivery of one copy of a given miRNA to an acceptor cell!
This suggests that most individual exosomes do not have biologically significant numbers of given miRNAs and thus are unlikely to mediate particular functional transfers, leading to consideration of special exosome subsets. Current concepts hold that 100–1000 miRNA copies of a particular miRNA are needed for miRNA to be transferred per targeted cell to mediate function [Citation136–144]. Accordingly, for the minimum of the 100 copies needed, on average a total of one million exosomes would be needed (100 x 10,000 = 106). At a physiologic transfer dose of a billion exosomes (1010), then one ten-thousandth (0.001%) of the total exosomes would be needed per target cell, to achieve a functional effect; that is extreme but seems possible.
However, since the 1 miRNA copy of a particular sequence in 106 exosomes is a calculated number [Citation145], it could instead be 10 copies per exosome or even 100, that would progressively be a rarer and rarer exosome subset mediating transfers to satisfy the rules at 100 copies required per exosome (respectively, 0.0001%) or 1 per hundred thousand exosomes of the total for 10 copies, or 1 copy per million exosomes of the total for the high 100 copy special exosomes. Therefore, a rare heavily miRNA loaded subset would, as a single exosome, be able to transfer miRNA function to one target cell. These do seem to be unlikely frequencies to target a given cell, but as noted are possible. Thus, either the low occupancy (1 per 104 of a physiological transfer dose of 1010 exosomes), or very much more likely the high occupancy model of very rare, specialized exosome subsets of 100 miRNA copies each, would achieve the needed 100 copies per targeted cell, as proposed previously [Citation135].This would be an aspect of exosome-carrier-effects, as it is a very special exosome subset of very low frequency, likely with a specific surface array signature of ligands for semi-specific binding to the surface of targeted cells to engender carrier-delivery of an unusually high miRNA copy number for uptake by particularly receptive target cells.
Target acceptor cell receptive properties can select particular carrier-delivering aspects of exosomes
Such transfers of function by very rare exosome nanovesicles with large numbers of copies of a given miRNA raises the concept of target cell receptive recognition of a specific signature of particular adhesin and receptor molecules on the surface of particular exosome subsets. Thus, an aspect of special carrier properties for exosome-delivery, is that an extremely rare special high miRNA carrying exosome subset would be need to also have a rather infrequent array of semi-specific exosome surface membrane components able to strongly bind to an unusual combination of surface entities on particularly receptive target cells. One example might be specific Ab surface coating of exosomes that we discovered on immunosuppressive exosomes that can bind specific Ag determinants of peptides complexed in MHC on the surface of targeted APC [Citation109,Citation112,Citation113], consisting therefore of rare exosome subsets binding to rare target cells.
Other analogous situations that are like this could include exosomes surface expression of various special ligands such as: heat shock proteins [Citation146,Citation147], heparan sulphate [Citation148], kit ligand [Citation149], fibronectin [Citation150], fas ligand [Citation151], epidermal growth factor receptor (EGFR) [Citation14], amphiregulin [Citation152], signalling molecules like wnt [Citation45,Citation153,Citation154], and Ephrin [Citation155]; cytokines like TGF-β [Citation156–158], and IFN-γ and its receptor [Citation159]. Thus, instances of such special binding exosomes could satisfy the copy number rules met by rare exosome subsets binding rare target cells, that might then stimulate augmented transfers via an exosome carrier-acting intracellular miRNA molecular cascade to possibly achieve a functional effect. This would be analogous to the surprising previous immunological finding that as few as one Ag-specific T cell is sufficient to mediate in vivo elicitation of classical Ag-specific DTH in the footpad of mice [Citation162]. Indeed, these special molecular binding and receiving properties would seem to be the raison d’être of such unique exosome coatings. Thus, perhaps these uncommon surface expressed entities are evolutionarily on the surface to serve the function of rare exosomes carrying unusual contents to specifically receptive targeted cells, as postulated here.
As little as femtomoles of a given miRNA associated with exosomes can be sufficient to transfer functional activity
As mentioned above, and unbelievable to many, are our findings showing that the carrier properties allow exosome transfer of a very low femtomole level of a particular miRNA, to function to mediate immune suppressive activity measured in vivo and in vitro [Citation160] (). In this system, inhibitory miRNA-150, that is a minor portion if the total RNA cargo of the pertinent exosomes, mediates transferred carrier functional effects of these nano EV. Transfer is via antigen (Ag)-specific targeting due to the Ab coating of the exosomes, rendering them functionally Ag-specific. Here, a physiological dose of 2 × 1010 genetically miRNA-150neg and thus non-suppressive exosomes are specific surface Ab positive as derived from B1a cells activated by light immunization of donor animals. These surface Ag-specific B cell-derived exosomes could be associated ex vivo with just 50 femtomoles of miRNA-150, that was the limit of transferred suppressive function in an miRNA dose-response experiment [Citation160].
Figure 3. A. Added miRNA-150 reconstitutes suppression mediated by exosomes from Ag-tolerized mRNA-150−/- mice. miRNA-150−/- mice show definitively that miR-150 is the suppressive entity in the exosome-like nanovesicles derived from CD8+ suppressor T cells of high dose Ag-tolerized mice. Nanovesicle small exosomes from OX-hapten-Ag tolerized miRNA-150−/- mice associated by in vitro incubation at 37° C with added miR-150, mediated suppression of immune ear swelling responses (Group D, 82% suppression) like exosomes from wild type Ag-tolerized mice (Group B, 78% suppression), both vs. Group A positive wild type controls, 0% suppression), whereas exosomes associated instead with control miRNA-150* (Group E) or with miRNA-mimic control (Group F) did not result in suppression; respectively 3% and 1% suppression.
B. Added miRNA-150 for dose response suppression by 1010 Ag-Specific B1a B cell-derived exosomes; femtomole associated exosomes suffice in vivo. Suppression of CS immune ear swelling responses by extracellular miRNA from TNP Ag-specific Ts cell-derived supernatant that was freed from lysed exosomes, or a dose response of miR-150 added alone, that were associated with B1a B-cell derived Ag-specific exosomes. Treatment of the TNP Ag- specific-CS-effector mixed cells (shown alone in Group A) with 2 day immune B1a B cell-derived TNP-Ag-specific exosomes that were associated by in vitro incubation at 37° C with the soluble Qiagen RNA fraction from TNP CD8 + T suppressor cell-derived exosomes (Group D) vs. control normal cell derived exosomes (Group B), or similar extract from normal cell exosomes (Group C), results in significant suppression of the adoptive immune cell transfer of an OX Ag-specific CS effector cell mixture (49% suppression vs 0–6% suppression of the control groups A, B and C). Similarly, 2 day immune Ag-specific B-1a B cell-derived exosomes, supplemented with serial decreasing doses of miR-150 alone, mediated suppression of TNP-CS-effector cell adoptive transfer of CS ear swelling responses (Groups E, F and G), down to a dose of 750pg miRNA-150 added per eventual recipient, which is 50 femtomoles per eventual recipient (Group G). Similar results were obtained in two other experiments in the CS system
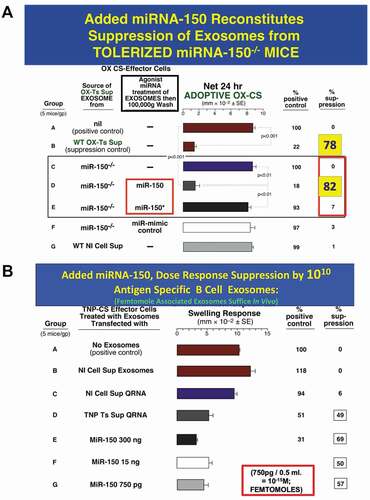
This carrier delivered and carrier functioning transfer of a very low amount of miRNA-150 enabled strong exosome suppression of a mixture of immune effector cells containing rare actually targeted APC and yet rarer particularly Ag-specific effector T cells when combined in vitro. When administered in vivo, what was inhibited was the effector T cell mediation of immune inflammatory ear skin responses of contact sensitivity (CS) in adoptive cell recipients [Citation160,Citation161]. In newer work in another related system of classical DTH immunity to the protein Ag ovalbumin, we found instead that nanomoles of miRNA-150 were the limiting effective number of copies in a similar dose-response experiment [Citation109]. This large quantitative difference may be due to the target Ag being a hapten conjugated to MHC in CS and a peptide complexed in MHC in the DTH responses [discussed in (111)].
These minute femtomole amounts of miRNA could not possibly survive in vivo nor mediate effects alone (i.e. exosome carrier acting) without their carrier-delivery by exosomes. Further, the exosome Ab coating, able to bind Ag peptides complexed to MHC on the targeted APC surface, is required for this exosome mediated transfer [Citation109,Citation161]. Unusually, here the Ab exosome coating is free immunoglobulin light chains (FLC). Note that these individual chains are monovalent in binding, but when displayed as multiples on the exosome surface, would gain significant avidity for effective high-affinity binding. This surface display induces activating function via cross-linking Ag at the target cell membrane, for the distinct exosome carrier-acting effect. This likely leads to epigenetic functional alterations due to the exosome transferred miRNA acting on endogenous mRNA encoding proteins involved in the expression of the appropriate DNA genes. These combined exosome carrier-delivery and carrier-acting mechanisms likely explain the exosome mediated quantitative in vivo and in vitro functional response at this very low dose of miRNA that were demonstrated definitively.
However, the experimental data here is incomplete, as it is not yet possible to determine the percent association of the added femtomoles or nanomoles of the associated miRNA-150 with the total 2 × 1010 exosomes of the exosome transferring dose. For calculations, we assume miRNA association to the exosomes at 100%, but it certainly is likely less for the postulated rare acting exosome subset that actually is involved, at least by a factor of ten. Further, it is not yet determined whether the low dose of miRNA-150 applied is associated with the exosome surface, or more conventionally became internal. Note the very interesting recent findings that there can be abundant polynucleotides on the exosome surface; most being RNAs compared to DNAs [Citation163,Citation164]. As background, the total miRNA concentration in serum/plasma is about 2–6 femtomoles per ml [Citation165]. Accordingly, although the low levels of miRNA we have found are functional when delivered by exosomes, that according to canonical non-exosome concepts are thought non-functional, in this instance given the Ab FLC activating effects of the delivering exosomes at the surface of the targeted cells, enables mediation of function.
There are three related quantitative aspects of exosome carrier aspects that greatly enhance effects of transferred miRNAs
These are:
1). The minute attoliter volume inside these small exosome nanovesicles causes a high concentration of contained miRNA
2). The crucial transferred miRNA is highly likely present in a minority of exosomes,
3). These few minute volume exosomes transfer their contained specific miRNA to a minority cell subset among the targeted population.
1). The minute attoliter volume inside these exosome nanovesicles causing a high concentration of contained miRNA
The particular miRNA is significantly molecularly concentrated in the relevant exosomes for potential activity in the targeted cells because it is in a minute intra exosome volume. We have calculated this to be 150 attoliters (10−18 Litres) per average 100 nanometres diameter exosome sphere, taking into account the space occupied by membrane components. This produces an unexpectedly high Molar concentration of the involved miRNA for transfer of biologic activity by the exosomes.
As an illustration, note that if one adds one femtomole of an miRNA to just one millilitre of medium the concentration is one femtomole per ml (10−3 litres). In contrast, if the added one femtomole is present in 1010 exosomes at a volume of 150 attoliters per exosome, then the concentration in the exosomes with rounding off is one femtomole per 10−8 litres (1010 exosomes x 10−18 litres each = 10−8 Litres). Therefore, exosome carriage brings a 100,000-fold (105) increased concentration, i.e. the miRNA in 10−3 litres is now in 10−8 litres to thus a 100 nanomolar concentration (10−15 moles or femtomoles to 10−7 Molar) thereby constituting an obvious highly increased carrier-acting effect due to a hundred-thousand-fold concentration of the contained miRNA, with thus great potential augmentation the exosome transfer of biologic function.
2). The crucial transferred miRNA is proposed to be present in a minority of exosomes
Another important ‘carrier effect’ is that only a small subset of the applied exosomes actually participate in the miRNA transfers. In the analysis above concerning miRNA copy numbers transferred, we noted that a small subset of exosomes with high specific miRNA content would be needed to have sufficient miRNA copies to meet the intracellular quantitative requirements for miRNA mediated molecular processes. Here, we consider this from a transfer perspective.
In our system of T cell mediated immune suppression of the CS type of DTH mediated by TNP-hapten-Ag-specific exosomes, their anti-hapten Ab-FLC surface coating is required in order for miRNA-150 to be delivered to then act epigenetically. As noted, 50 femtomoles of miRNA-150 were found to be the quantitative limit for 1010 delivering exosomes when employing functional dose-response testing [Citation160] (). An important related finding was that because of the Ab coating specific Ag-linked affinity columns clearly separated the active vs. the non-functional exosomes [Citation161], we found that only about 10% of the miRNA-150-associated exosomes that bound and then were eluted from the Ag-affinity column were biologically inhibitory [Citation108,Citation161]. Similar findings emerged from use of anti-CD9 tetraspanin affinity column chromatography [Citation108,Citation109] and flow cytometry showed that the Ag-specific Ab FLC and CD9 were on the surface of the same nanovesicles [Citation109].
Further note that control exosomes from normal, non-immune unmanipulated animals were inactive, had little surface Ab FLC and significantly less expressed CD9, as were the non-active exosomes from immune animals that passed the Ag- and Ab-affinity columns [Citation108,Citation109]. These biologically inactive exosomes are a distinct and differing subset not able to associate with miRNA-150 nor able to bind Ab FLC to their surface. Thus, exosomes eluted from binding to the Ag-affinity or Ab-affinity columns, were uniquely able to surface bind Ab-FLC and therefore Ag-specifically transfer miRNA-150 to mediate targeted cell suppression. Therefore, these are considered an ‘activated’ subpopulation of carrier exosomes. It seemed that the activation properties were conferred by exosome-producing donor cells that were activated by stimulating processes association with immunization of these cell donors per se. This has been noted in other systems [Citation166–168].
At 10% of the total, these ‘activated’ exosomes are 109 of the total 1010 exosomes we used as a treatment dose. This dose was chosen because it is the number of exosomes per ml of mouse blood. This 10% activated subpopulation was thus actually the fraction of the total exosomes that associated with the 50 femtomoles of miRNA-150 for mediation of the functional endpoint of the dose-response experiment [Citation160] (). Specifically, when this minor 10% of ‘activated’ Ab FLCpos and miRNA-150pos exosome subpopulation, was used to pulse the total targeted CS effector cell mixture, they mediated all of the suppression [Citation108,Citation109,Citation161]. Thus, for calculations, the amount of actually transferred miRNA per exosome, now at 105 augmented concentration, can therefore be increased by a factor of 10, since only about 10% of the total have these activated transferring properties.
3). These few minute volume exosomes transfer their contained specific miRNA to a minority cell subset among the targeted population
Yet another important quantitative ‘carrier effect’ is that only a small subset of the total recipient cell population may actually be targeted for the miRNA transfers. In our suppressor immune system, we determined that the inhibitory miRNA-150 carrying exosomes actually target rare antigen presenting cell (APC) macrophages and dendritic cells (DC), and not the effector T cells of the effector cell mixture [Citation113]. These T cells are subsequently suppressed by these APC via their production of secondary suppressive exosomes with specific peptide Ag/MHC surface complexes that finally act on the effector T cells at the immune synapse formed between their ab-TCR and the specific peptide Ag/MHC surface complexes on the APC or their secreted secondary suppressive exosomes [Citation112].
For experiments on inhibition of the adoptive cell transfer of protein Ag-induced DTH, these actually targeted APC recipients of miRNA-150, are a minor subpopulation, along with the actual Ag/MHC-specific effector T cells they eventually suppress. These APC and the particular OVA-peptide-Ag/MHC-specific effector T cells are among the treated immune effector lymphoid cell mixture pulsed in vitro with the 10% of ‘activated’ Ab FLCpos, miRNA-150pos suppressive exosome subpopulation among the 1010 total exosomes applied [Citation113]. These APC are a distinct minority population of about only 0.1% of the total employed immune spleen and lymph node effector cell mixture of 107 pooled DTH-effector spleen and lymph node cells.
Summarizing these quantitative considerations
The 150 × 10−15 femtomoles limiting effective dose is augmented overall by 109 -fold by consideration of exosome carrier effects. Thus, there is 105 augmentation per volume considerations x 101 per fractional proportion of involved exosomes x 103 per the fractional proportion of targeted APC = overall: 105 × 101 x 103 = 109 -fold augmentation. Thus, this 109 -fold augmentation of the 10−15 femtomoles of miRNA-150 applied via the activated exosome subset results in 10−8 equivalent moles, or potentially a concentration to hundreds of micromolar by these exosome carrier considerations.
Exosome subsets like those surviving harsh conditions, or those involved in the immune tolerance system are considered to be ‘activated’
We noted that this exosome subpopulation that was generated in high Ag dose-induced immune tolerance was different from exosomes produced by normal cells; thus, we called them immune ‘activated’. In addition, we found that in vitro culture of exosome producing cells, that were harvested from animals immunized in various ways had acquired the ability to produce such a subpopulation of exosomes with these unusual properties. They are thus called ‘activated’ because they are from seemingly activated donor cells derived from immunized hosts. The exosome properties induced could therefore mediate unprecedented interactions of the exosome surface influencing interactions with also rare particularly receptive targeted acceptor cells that were not abilities of exosomes derived from cells of unmanipulated normal donors.
These ‘activation’ properties contribute significantly to the exosome carrier effects
They include ability to surface bind either in vivo available or investigator selected Ab free light chains (FLC) to render the exosomes desired Ag-specific [Citation108,Citation109,Citation111,Citation161], and further ability to associate with added miRNA of choice [Citation108,Citation111,Citation160,Citation161]. These abilities are postulated due to an altered composition of their surface membrane lipids [Citation34–39], that can be significantly changed rapidly by particular activating conditions of mice immunized or likely treated in various ways; including Ag-tolerization. The unusual lipid composition of activated exosomes may result in relative increased size and most importantly distinctly greater viscosity of activated exosome membranes [Citation36,Citation172]. This increased membrane viscosity and resulting stiffness [Citation36] may account for the unusual and specific Ab FLC binding and selected mRNA association, as well as resistance to harsh conditions like hypoxia and survival in the acid/enzyme mixture of the stomach; compared to exosomes derived from non-stimulated cells. There are other modes of activation besides immunization, such as the donor cell stage of maturation [Citation168]. It is noteworthy that prior studies by others have shown that human lymphocytes and leukocytes also can demonstrate surface binding with Ab FLC [Citation169,Citation170,Citation171].
To summarize findings in our system, this Ab FLC coating allowed Ag-specific binding of a 10% functional subpopulation of exosomes to Ag-linked affinity columns. Compared to the remaining non- Ag-binding, non-functional exosomes the Ag-column, these eluted exosomes had shared activation properties. These were molecular expression of the particular miRNA-150 mediating suppressive function when evaluated both by in vivo and in vitro assays and the ability to resist the strong acid/enzyme environment of the stomach.
Ability of subpopulations of activated exosomes to associate with added miRNA is an important carrier effect
When we showed that T cell suppression of CS and DTH in Ag hi dose tolerized mice was due to Ag-specific exosomes delivering miRNA-150, we then showed this more definitively by demonstrating that miRNA-150 deficient, but Ag-specific exosomes could be restored to suppression by merely adding miRNA-150. Thus, for eventual T cell suppression, we harvested exosomes from CD8pos suppressor T cells of tolerized mice that were miRN-150 genetically deficient and added miRNA-150 by association during incubation in vitro in a 37°C water. We then washed away free miRNA-150 and showed restoration of suppressive activity [Citation45] (). Further, and very importantly, having shown that the essential properties of the suppressive T cell-derived exosomes were Ag-specificity due to surface Ag-specific Ab FLC and carriage of miRNA-150, we induced production of solely Ag-specific exosomes by B cells of lightly Ag-immunized hosts that were not suppressive, but were rendered so by association with miRNA-150 during similar associating in vitro incubation [Citation20]. In this case a dose-response of the added miRNA-150 was done. As noted above, the limiting dose of associating miRN-150 was as little 50 nanomoles for this hapten-Ag-specific CS system [Citation20] ().
These results were not confined to such artificial experiments of adding a single miRNA but were confirmed with the mixture of Qiagen column enriched RNA extracted from the exosomes of which miRNA-150 was a rare constituent. When this mixture of RNAs was used in vitro or in injected in vivo, there also was mediation of Ag-specific and miRNA-150-dependent suppression of CS by the contained miRNA-150 acting via host B1a cell derived Ag-specific exosomes [Citation160] .
All together the ability of subpopulations of activated exosomes to associate with added chosen or mixed miRNA is a clear demonstration of the essential carrier property of exosomes for delivery and then functional action of transferred miRNA; in this case very small miRNA doses way beyond conventional concepts based on mere addition or genetic expression of miRNA.
Overall summary
This review focused on the carrier roles of exosomes participating in their mediation of miRNAs transfers to targeted acceptor cells. It is posited that these inherent aspects of such physiologic exosome transfers likely provide underappreciated assistance for resulting biological functions of the transferred miRNAs. We have considered the doubts of miRNA experts that exosomes cannot mediate such transfers because the amounts involved are below conventions of the field that were established without consideration of the role of exosomes. Especially doubtful to these experts are the reported successful low-dose miRNA transfers. However, these conventional views ignore exosome carrier effects that thus are ‘The Elephant in the Room’. The conventional negative opinions are based on current canonical rules derived from mostly non-physiologic prior studies, simply adding naked miRNAs or also non-physiologic genetic expression that additionally is non-quantitative.
Thus, carrier effects of exosomes on their miRNA transferred biologic activities is the central point of this review. These carrier-delivering and carrier-acting exosome aspects are predicted to promote the transfer and subsequent mediated molecular functions, often crucial for epigenetic alterations on DNA gene expression in the targeted cells, induced by their transferred miRNAs. Thus, exosome miRNA transfers acting via both ‘carrier-delivery’ to the acceptor cells and then ‘carrier-acting’ intracellular functions are the “Elephant in the Room; i.e. over looked by both the doubting scientists of the miRNA cognoscente and generally by anti- exosome scientific luddites who perhaps cannot bear contemplating new exosome mediated processes that might be involved in their own systems.
Contributing to advantageous carrier effects is the great heterogeneity of EV, such as exosomes resulting in the possibility that no two of these natural nanoparticles may be exactly alike. These many different subsets are relevant to their varied functional carrier properties in their mediation of transfers of miRNAs, since rare subpopulations may have special properties, like carriage of large amounts of a given miRNA. Extracellular carrier effects of the exosomes likely are contributed to by the myriad of binding and agonist surface signature entities that can molecularly target especially receptive particular acceptor cells via their corresponding surface ligands and receptors. These then activate the target cells to express intracellular processes allowing exosome carrier stimulated functions of the low doses of the transferred miRNAs.
Exosome survival amidst harsh conditions is another obvious extracellular carrier effect since no survival means no transfer. A very early evolutionary history of exosome origins in the ancient pre-cell noxious environment of ‘the primordial soup’ near to the origin of life, more than three billion year ago may account for their unusual resistance as carriers in harsh microenvironmental conditions. This includes resistance to exposure to strong hypoxia, even hyperoxia and to acid/enzyme mixtures, say at hypermetabolic sites of growing cancers. The latter has been demonstrated repeatedly in the ability of exosomes in milk to pass the recipient neonatal stomach to then enable subsequent intestinal uptake and then participation in the development of various biologic systems of the newborn baby.
The involved exosomes are considered to be ‘activated’ as they are derived from especially activated cell donors. They are suggested to be descendants of pro cells with resistant bilamellar membranes that arose in the noxious primordial soup environment of early evolutionary history, that may have brought to modern times subpopulations of exosomes with membranes of unusual lipid composition. This likely has resulted in high surface viscosity, allowing mediation of some carrier effects. One example is demonstrated in current experiments showing oral transfer of activated inhibitory exosomes from immune activated suppressor T cells, that can pass the adult stomach to transfer systemically inhibitory miRNA-150 to suppress recipient effector T cell mediated skin immune inflammatory responses in an Ag-specific and miRNA gene-specific manner for several days after a single physiological dose.
Some experts doubt successful transfers by low levels of exosome miRNAs that may in fact be due to the accompanying carrier-acting effects; previously not accounted for. Our work demonstrates that subpopulations of activated exosomes are able associate in vitro or in vivo with added chosen miRNA to represent a clear carrier property. Quantitative miRNA copy number issues are thought to make exosome functional transfers doubtful. In fact, we have shown that as little as femtomoles of a given miRNA associated with exosomes can be sufficient to transfer functional activity. To account for this, a very important and obvious exosome-carrier quantitative effect is the minute attoliter volume inside these nanovesicles causing a high concentration of contained low-dose miRNAs. Another is that only a small subset of the applied exosomes actually participates in the miRNA transfers. An additional related ‘carrier effect’ is that only a small subset of the total recipient cell population is actually targeted for the miRNA transfers. All together, these various recognized and postulated carrier properties of exosomes are proposed to enable miRNA transfers not contemplated as possible by classical canonical thinking that does not consider the biologic roles of the transferring exosomes.
Disclosure of Potential Conflicts of Interest
No potential conflict of interest was reported by the author.
Additional information
Funding
References
- Turchinovich A, Tonevitsky AG, Cho WC, et al. Check and mate to exosomal extracellular miRNA: new lesson from a new approach. Front Mol Biosci. 2015;2:11.
- Turchinovich A, Tonevitsky AG, Burwinkel B. Extracellular miRNA: a collision of two paradigms. Trends Biochem Sci. 2016 Oct;41(10):883–892.
- Jeppesen DK, Fenix IM, Franklin JL, et al. Reassessment of exosome composition. CELL. 2019 APRIL 04;177(2): P428-445.E18.
- Li M, Zeringer E, Barta T, et al. Analysis of the RNA content of the exosomes derived from blood serum and urine and its potential as biomarkers. Philos Trans R Soc Lond B Biol Sci. 2014;369(1652):20130502.
- Statello L, Maugeri M, Garre E, et al. Identification of RNA-binding proteins in exosomes capable of interacting with different types of RNA: RBP- facilitated transport of RNAs into exosomes. PLoS One. 2018 Apr 24;13(4):e0195969.
- Askenase PW. Current exosome research is too descriptive and phenomenological: vast numbers of exosome subsets suggest first performing a functional top down determination of the involved genes. International Journal of Molecular Sciences. 2021;22. Submitted. DOI:10.3390/ijms22031429.
- Tkach M, Kowal J, Thery C. Why the need and how to approach the functional diversity of extracellular vesicles. Philos Trans R Soc Lond B Biol Sci. 2018;373:20160479.
- Zhang H, Freitas D, Kim HS, et al. Identification of distinct nanoparticles and subsets of extracellular vesicles by asymmetric flow field-flow fractionation. Nat Cell Biol. 2018;20:332–343.
- Horvathova I, Voigt F, Kotrys AV, et al. The dynamics of mRNA turnover revealed by single-molecule imaging in single cells. Mol Cell. 2017;68:615–625 e619.
- Regev A, Teichmann SA, Lander ES, et al. The human cell atlas. Elife. 2017;6:e27041.
- Adey AC. Haplotype resolution at the single-cell level. Proc Natl Acad Sci USA. 2017;114:12362–12364.
- Smith ZJ, Lee C, Rojalin T, et al. Single exosome study reveals subpopulations distributed among cell lines with variability related to membrane content. J Extracell Vesicles. 2015;4:28533.
- Kibria G, Ramos E, Lee K, et al. A rapid, automated surface protein profiling of single circulating exosomes in human blood. Sci Rep. 2016;6:36502.
- Higginbotham JN, Zhang Q, Jeppesen DK, et al. Identification and characterization of EGF receptor in individual exosomes by fluorescence-activated vesicle sorting. J Extracell Vesicles. 2016;5:29254.
- Wu D, Yan J, Shen X, et al. Profiling surface proteins on individual exosomes using a proximity barcoding assay. Nat Commun. 2019;10:3854.
- Pospichalova V, Svoboda J, Dave Z, et al. Simplified protocol for flow cytometry analysis of fluorescently labeled exosomes and microvesicles using dedicated flow cytometer. J Extracell Vesicles. 2015;4:1.
- Skog J, Würdinger T, Van Rijn S, et al. Glioblastoma microvesicles transport RNA and proteins that promote tumour growth and provide diagnostic biomarkers. Nat Cell Biol. 2008;10(12):1470–1476.
- Zhang L, Valencia CA, Dong B, et al. Transfer of microRNAs by extracellular membrane microvesicles: a nascent crosstalk model in tumor pathogenesis, especially tumor cell-microenvironment interactions. J Hem Oncol. 2015;8:14.
- Li J, Zhang Y, Liu Y, et al. Microvesicle- mediated transfer of microRNA-150 from monocytes to endothelial cells promotes angiogenesis. J Biol Chem. 2013;288:23586–23596.
- Hulsmans M, Holvoet P. MicroRNA-containing microvesicles regulating inflammation in association with atherosclerotic disease. Cardiovasc Res. 2013 Oct 1;100(1):7–18.
- Jaiswal R, Luk F, Gong J, et al. Microparticle conferred microRNA profiles-implications in the transfer and dominance of cancer traits. Mol Cancer. 2012;11:37.
- Gong J, Luk F, Jaiswal R, et al. Microparticles mediate the intercellular regulation of microRNA-503 and proline-rich tyrosine kinase 2 to alter the migration and invasion capacity of breast cancer cells. Front Oncol. 2014;4:220.
- Zhang Q, Higginbotham JN, Jeppesen DK, et al. Transfer of functional cargo in exomeres. Cell Rep. 2019 Apr 16;27(3):940–954.e6.
- Chahar HS, Bao X, Casola A. Exosomes and their role in the life cycle and pathogenesis of RNA viruses. Viruses. 2015;7:3204–3225.
- Gill S, Forterre P. Origin of life: LUCA and extracellular membrane vesicles (EMVs). Int J Astrobiol. 2016;15:7–15.
- Schrum JP, Zhu TF, Szostak JW. The origins of cellular life. Cold Spring Harb Perspect Biol. 2010 Sep;2(9):a002212.
- Szostak JW. The eightfold path to non-enzymatic RNA replication. J Syst Chem. 2012;3:2.
- Adamala K, Szostak JW. Nonenzymatic template-directed RNA synthesis inside model protocells. Science. 2013;342:1098–1100.
- Deatherage BL 1, Cookson BT. Membrane vesicle release in bacteria, eukaryotes, and archaea: a conserved yet underappreciated aspect of microbial life. Infect Immun. 2012 Jun;80(6):1948–1957.
- Record M, Carayon K, Poirot M, et al. Exosomes as new vesicular lipid transporters involved in cell–cell communication and various pathophysiologies. Biochim Biophys Acta (BBA)-Mol Cell Biol Lipids. 2014;1841:108–120.
- Skotland T, Sandvig K, Llorente A. Lipids in exosomes: current knowledge and the way forward. Prog Lipid Res. 2017 Apr;66:30–41.
- Laulagnier K, Motta C, Hamdi S, et al. Mast cell- and dendritic cell-derived exosomes display a specific lipid composition and an unusual membrane organization. Biochem J. 2004 May 15;380(Pt 1):161–171.
- Record M 1, Silvente-Poirot S 2, Poirot M 3, et al. Thematic review series: exosomes and microvesicles: lipids as key components of their biogenesis and functions. J Lipid Res. 2019 Jan;60(1):9–18.
- Skotland T, Hessvik NP, Sandvig K, et al. Exosomal lipid composition and the role of ether lipids and phosphoinositides in exosome biology. J Lipid Res. 2019 Jan;60(1):9–18.
- Hough KP, Wilson LS, Trevor JL, et al. Deshane JS. Unique lipid signatures of extracellular vesicles from the airways of asthmatics. Sci Rep. 2018 Jul 9;8(1):10340.
- Federici C, Petrucci F, Caimi S, et al. Exosome release and low pH belong to a framework of resistance of human melanoma cells to cisplatin. PloS One. 2014;9:e8819.
- King HW, Michael MZ, Gleadle JM. Hypoxic enhancement of exosome release by breast cancer cells. BMC Cancer. 2012;12:421.
- Panigrahi GK, Praharaj PP. Hypoxia-induced exosome secretion survival of African-American and Caucasian prostate cancer cells. Sci Rep. 2018;8:Article number: 3853.
- Panigrahi GK, Praharaj PP, Peak TC, et al. Hypoxia-induced exosome secretion promotes survival of African-American and Caucasian prostate cancer cells. Sci Rep. 2018;8:3853.
- Dorayappan KDP, Wanner R, Wallbillich JJ, et al. Hypoxia-induced exosomes contribute to a more aggressive and chemoresistant ovarian cancer phenotype: a novel mechanism linking STAT3/Rab proteins. Oncogene. 2018;37(28):3806–3821.
- Ramteke A, Ting H, Agarwal C, et al. Exosomes secreted under hypoxia enhance invasiveness and stemness of prostate cancer cells by targeting adherens junction molecules. Mol Carcinog. 2015;54(7):554–565.
- Deep G, Panigrahi GK. Hypoxia-induced signaling promotes prostate cancer progression: exosomes role as messenger of hypoxic response in tumor microenvironment. Crit Rev Oncog. 2015;20(5–6):419–434.
- Shao C, Yang F, Miao S, et al. Role of hypoxia-induced exosomes in tumor biology. Mol Cancer. 2018;17(1):120.
- Kucharzewska P, Christianson HC. Exosomes reflect the hypoxic status of glioma cells and mediate hypoxia-dependent activation of vascular cells during tumor development. Proc Natl Acad Sci USA. 2013;110(18):7312–7317.
- Huang Z, Feng Y. Exosomes derived from hypoxic colorectal cancer cells promote angiogenesis through Wnt4-induced -catenin signaling in endothelial cells. Oncol Res. 2017;25:651–661.
- Almeria C, Weiss R, Roy M, et al. Hypoxia conditioned mesenchymal stem cell-derived extracellular vesicles induce increased vascular tube formation in vitro. Front Bioeng Biotechnol. 2019;7:292.
- Wang X, Luo G, Zhang K, et al. Hypoxic tumor-derived exosomal miR-301a mediates M2 macrophage polarization viaPTEN/PI3Kgamma to promote pancreatic cancer metastasis. Cancer Res. 2018;80(4):922.
- Chen X, Zhou J, Li X, et al. Exosomes derived from hypoxic epithelial ovarian cancer cells deliver microRNAs to macrophages and elicit a tumor- promoted phenotype. Cancer Lett. 2018 Oct 28;435:80–91.
- Li L, Li C, Wang S, et al. Exosomes derived from hypoxic oral squamous cell carcinoma cells deliver miR-21 to normoxic cells to elicit a prometastatic phenotype. Cancer Res. 2016;76(7):1770–1780.
- Han KH, Kim AK, Kim MH, et al. Enhancement of angiogenic effects by hypoxia-preconditioned human umbilical cord-derived mesenchymal stem cells in a mouse model of hindlimb ischemia. Cell Biol Int. 2016 Jan;40(1):27–35.
- Veerappan A, Thompson M, Savage AR, et al. Mast cells and exosomes in hyperoxia-induced neonatal lung disease. Am J Physiol Lung Cell Mol Physiol. 2016 Jun 1;310(11):L1218–32.
- Zhang X, Lu A, Li Z, et al. Exosomes secreted by endothelial progenitor cells improve the bioactivity of pulmonary microvascular endothelial cells exposed to hyperoxia in vitro. Ann Transl Med. 2019;7(12):254.
- Benmoussa A, Lee CHC, Laffont B, et al. Commercial dairy cow milk microRNAs resist digestion under simulated gastrointestinal tract conditions. J Nutr. 2016;146:2206–2215.
- Parigi SM, Eldh M, Larssen P, et al. Breast milk and solid food shaping intestinal immunity. Front Immunol. 2015;6:415.
- Liu C, Teng ZQ, Santistevan NJ, et al. Epigenetic regulation of miR-184 by MBD1 governs neural stem cell proliferation and differentiation. Cell Stem Cell. 2010;6(5):433–444.
- McKiernan RC, Jimenez-Mateos EM, Sano T, et al. Expression profiling the microRNA response to epileptic preconditioning identifies miR-184 as a modulator of seizure-induced neuronal death. Exp Neurol. 2012;237(2):346–354.
- Oliveira MC, Di Ceglie I, Arntz OJ, et al. Milk-derived nanoparticle fraction promotes the formation of small osteoclasts but reduces bone resorption. J Cell Physiol. 2017;232:225–233.
- Hoeflich A, Meyer Z. Functional analysis of the IGF-system in milk. Best Pract Res Clin Endocrinol Metab. 2017 Aug;31(4):409–418.
- Bartol FF, Wiley AA, George AF, et al. Postnatal reproductive development and the lactocrine hypothesis. J Animal Sci. 2017 May;95(5):2200–2210.
- Melnik BC. Milk exosomes and microRNAs: potential epigenetic regulators. Handbook of nutrition, diet, and epigenetics. In: Patel VB, Preedy VR, editors. Handbook of nutrition, diet, and epigenetics. Springer International Publishing AG; 2019. p. 1467–1494. doi:10.1007/978-3-319-31143-2_86-1
- Sun Q, Chen X, Yu J, et al. Immune modulatory function of abundant immune-related microRNAs in microvesicles from bovine colostrum. Protein Cell. 2013;4:197–210.
- Admyre C, Johansson SM, Qazi KR, et al. Exosomes with immune modulatory features are present in human breast milk. J Immunol. 2007;179:1969–1978.
- Melnik BC, John SM, Schmitz G. Milk: an exosomal microRNA transmitter promoting thymic regulatory T cell maturation preventing the development of atopy? J Transl Med. 2014;12:43.
- Zhou Q, Li M, Wang X, et al. thymic regulatory T cell maturation preventing the development of atopy are abundant in breast milk exosomes. Int J Biol Sci. 2012;8:118–123.
- Torregrosa Paredes P, Gutzeit C, Johansson S, et al. Differences in exosome populations in human breast milk in relation to allergic sensitization and lifestyle. Allergy. 2014;69:463–471.
- Kosaka N, Izumi H, Sekine K, et al. microRNA as a new immune-regulatory agent in breast milk. Silence. 2010;1:7.
- Baier SR, Nguyen C, Xie F, et al. MicroRNAs are absorbed in biologically meaningful amounts from nutritionally relevant doses of cow milk and affect gene expression in peripheral blood mononuclear cells, HEK-293 kidney cell cultures, and mouse livers. J Nutr. 2014;144(10):1495–1500.
- de la Torre Gomez C, Goreham RV, Bech Serra JJ, et al. Exosomics”—A Review of biophysics, biology and biochemistry of exosomes with a focus on human breast milk. Front Genet. 2018 Mar 27;9:92.
- Cong X, Henderson WA, Graf J, et al. Early life experience and gut microbiome: the brain-gut-microbiota signaling system. Adv Neonatal Care. 2015;15:314–323.
- Gritz EC, Bhandari V. The human neonatal gut microbiome: a brief review. Front Pediatr. 2015;3:17.
- Fujimura KE, Sitarik AR, Havstad S, et al. Neonatal gut microbiota associates with childhood multisensitized atopy and T cell differentiation. Nat Med. 2016 Oct;22(10):1187–1191.
- Laffitte L. Ed Yong. I contain multitudes: the microbes within us and a grander view of life. J Big History. 2017;1:63–65.
- Izumi H, Kosaka N, Shimizu T, et al. Bovine milk contains microRNA and messenger RNA that are stable under degradative conditions. J Dairy Sci. 2012;95:4831–4841.
- Aqila F, Munagalaa R, Jeyabalana J, et al. Milk exosomes-natural nanoparticles for siRNA delivery. Cancer Lett. 2019 May 1;449:186–195.
- Chen T, Xi Q-Y, Ye R-S, et al. Exploration of microRNAs in porcine milk exosomes. BMC Genomics. 2014;15(1):100.
- Kahn S, Liao Y, Du X, et al. Exosomal microRNAs in milk from mothers delivering preterm infants survive in vitro digestion and are taken up by human intestinal cells. Mol Nutr Food Res. 2018;62:e1701050.
- Pieters BC, Arntz OJ, Bennink MB, et al. Commercial cow milk contains physically stable extracellular vesicles expressing immunoregulatory TGF-β. PLoS One. 2015;10:e0121123.
- Zempleni J, Baier SR, Howard KM, et al. Gene regulation by dietary microRNAs 1. Can J Physiol Pharmacol. 2015;93:1097–1102.
- Zempleni J, Baier SR, Hirschi K. Diet-responsive microRNAs are likely exogenous. J Biol Chem. 2015;290:25197–25197.
- Manca S, Upadhyaya B, Mutai E, et al. Milk exosomes are bioavailable and distinct microRNA cargos have unique tissue distribution patterns. Sci Rep. 2018;8:11321.
- Hirschi KD, Pruss GJ, Vance V. Dietary delivery: a new avenue for microRNA therapeutics? Trends Biotechnol. 2015;33:431–432.
- Yang J, Farmer LM, Agyekum AA, et al. Detection of dietary plant-based small RNAs in animals. Cell Res. 2015;25:517.
- Yang J, Farmer LM, Agyekum AA, et al. Detection of an abundant plant-based small RNA in healthy consumers. PloS One. 2015;10:e0137516.
- Yarmarkovich M, Hirschi KD. Digesting dietary miRNA therapeutics. Oncotarget. 2015;6:13848.
- Kusuma RJ, Manca S, Friemel T, et al. Human vascular endothelial cells transport foreign exosomes from cow’s milk by endocytosis. Am J Physiol Cell Physiol. 2016;310:C800–C807.
- Wolf T, Baier SR, Zempleni J. The intestinal transport of bovine milk exosomes is mediated by endocytosis in human colon carcinoma Caco-2 cells and rat small intestinal IEC-6 cells. J Nutr. 2015;145:2201–2206.
- Shu J, Chiang K, Zempleni J, et al. Computational characterization of exogenous microRNAs that can be transferred into human circulation. PloS One. 2015;10:e0140587.
- Howard KM 1, Jati Kusuma R, Baier SR, et al. Loss of miRNAs during processing and storage pasturization of cow’s (Bos taurus) milk. J Agric Food Chem. 2015 Jan 21;63(2):588–592.
- Zhao Z, Yu S, Xu M, et al. Effects of microwave on extracellular vesicles and microRNA in milk. J Dairy Sci. 2018;101(4):2932–2940.
- Yu S, Zhao Z, Sun L, et al. Fermentation results in quantitative changes in milk-derived exosomes and different effects on cell growth and survival. J Agric Food Chem. 2017;65(6):1220–1228.
- Title AC, Denzler R ‡, Stoffel M. Uptake and function studies of maternal milk-derived microRNAs. J Biol Chem. 2015 Sep 25;290(39):23680–23691.
- Hock A, Miyake H, Li B, et al. Breast milk-derived exosomes promote intestinal epithelial cell growth. J Pediatr Surg. 2017;52:755–759.
- Melnik BC. Milk: an epigenetic amplifier of FTO-mediated transcription? Implications for Western diseases. J Transl Med. 2015;13:385.
- Zhang L 1, Chen T 1, Yin Y 2,3, et al. Dietary microRNA—a novel functional component of food. Adv Nutr. 2019;10:711–721.
- Arntz OJ, Pieters BCH, Oliveira MC, et al. Oral administration of bovine-derived extracellular vesicles attenuates arthritis in two mouse models. Mol Nutr Food Res. 2015;59(9):1701–1712.
- Ma J, Wang C, Long K, et al. Exosomal microRNAs in giant panda (Ailuropoda melanoleuca) breast milk: potential maternal regulators for the development of newborn cubs. Sci Rep. 2017;7(1):3507.
- Yang J, Hirschi KD, Farmer LM. Dietary RNAs: new stories regarding oral delivery. Nutrients. 2015;7:3184–3199.
- Zhanga S 1, Sanga X 1, Houa D 1, et al. Plant-derived RNAi therapeutics: a strategic inhibitor of HBsAg. Biomaterials. 2019 July;210:83–93.
- Mlotshwa S, Pruss GJ, MacArthur JL, et al. A novel chemopreventive strategy based on therapeutic microRNAs produced in plants. Cell Res. 2015;25:521.
- Lukasik A, Brzozowska I, Zielenkiewicz U, et al. Detection of plant miRNAs abundance in human breast milk. Int J Mol Sci. 2017;19(1):37.
- Liang G, Zhu Y, Sun B, et al. Assessing the survival of exogenous plant microRNA in mice. Food Sci Nutr. 2014;2(4):380–388.
- Liu YC, Chen WL, Kung WH, et al. Plant miRNAs found in human circulating system provide evidences of cross kingdom RNAi. BMC Genomics. 2017;18(Suppl2):112.
- Beatty M, Guduric-Fuchs J, Brown E, et al. Small RNAs from plants, bacteria and fungi within the order Hypocreales are ubiquitous in human plasma. BMC Genomics. 2014;15:933.
- Eichenberger RM, Talukder MH, Field MA, et al. Characterization of Trichuris muris secreted proteins and extracellular vesicles provides new insights into host–parasite communication. J Extracell Vesicles. 2018;7(1):1428004.
- Mu J, Zhuang X, Wang Q, et al. Interspecies communication between plant and mouse gut host cells through edible plant derived exosome-like nanoparticles. Mol Nutr Food Res. 2014;58:1561–1573.
- Pastrello C, Tsay M, McQuaid R, et al. Circulating plant miRNAs can regulate human gene expression in vitro. Sci Rep. 2016;6:32773.
- Chin AR, Fong MY, Somlo G, et al. Cross-kingdom inhibition of breast cancer growth by plant miR159. Cell Res. 2016;26:217–228.
- Wąsik M, Nazimek K, Nowak B, et al. Delayed-type hypersensitivity underlying casein allergy is suppressed by extracellular vesicles carrying miRNA-150. Nutrients. 2019 Apr 23;11(4): pii: E907.
- Nazimek K, Bryniarski K, Ptak W, et al. Orally administered, antigen-specific, T and B cell-derived suppressor exosomes deliver miRNA-150 to strongly inhibit DTH by binding to peptides in MHC of APC due to antibody light chain coating. Int J Mol Sci. 2020;21:5540.
- Liang H, Zhang S, Fu Z, et al. Effective detection and quantification of dietetically absorbed plant microRNAs in human plasma. J Nutr Biochem. 2015;26(5):505–512.
- Nazimek K, Bryniarski K, Askenase PW. Functions of exosomes and microbial. Extracellular vesicles in allergy and contact and delayed-type hypersensitivity. Int Arch Allergy Immunol. 2016;171:1–26.
- Nazimek K, Bustos-Morán E, Blas-Rus N, et al. Regulation at the immune synapse by a multi-exosome-miRNA circuit of APC-connected T cells. 2021. submitted.
- Nazimek K, Ptak W, Nowak B, et al. Macrophages play an essential role in antigen-specific immune suppression mediated by T CD8+ cell-derived exosomes. Immunology. 2015 Sep;146(1):23–32.
- Voutila J, Sætrom P, Mintz P, et al. Gene expression profile changes after short-activating RNA- mediated induction of endogenous pluripotency factors in human mesenchymal stem cells. Mol Ther Nucleic Acids. 2012 Aug;1(8):e35.
- Lin C-W, Jan M-S, StevenKuo J-H. The microRNA expression profiles in extracellular vesicles from HeLa cancer cells in response to cationic lipid- or polyethylenimine-mediated gene delivery. J Drug Target. 2019 Jan;27(1):94–102.
- Lin CW, Jan MS, Kuo JH. The vector-related influences of autophagic microRNA delivery by Lipofectamine 2000 and polyethylenimine 25K on mouse embryonic fibroblast cells. Eur J Pharm Sci. 2017;101:11–21.
- Huerfano S, Ryabchenko B, Forstová J. Nucleofection of expression vectors induces a robust interferon response and inhibition of cell proliferation. DNA Cell Biol. 2013 Aug;32(8):467–479.
- Zhang L, Hou D, Chen X, et al. Exogenous plant MIR168a specifically targets mammalian LDLRAP1: evidence of cross-kingdom regulation by microRNA. Cell Res. 2012;22:107–126.
- Buck AH, Coakley G, Simbari F, et al. Exosomes secreted by nematode parasites transfer small RNAs to mammalian cells and modulate innate immunity. Nat Commun. 2014;5. DOI:10.1038/ncomms6488
- Sisquella X, Ofir-Birin Y, Pimentel MA, et al. Malaria parasite DNA-harbouring vesicles activate cytosolic immune sensors. Nat Commun. 1985 (2017);8:1–15.
- Samuel M, Bleackley M, Anderson M, et al. Extracellular vesicles including exosomes in cross kingdom regulation: a viewpoint from plant-fungal interactions. Front Plant Sci. 2015 Sep 23;6:766.
- Regente M, Pinedo M, San Clemente H, et al. Plant extracellular vesicles are incorporated by a fungal pathogen and inhibit its growth. J Exp Bot. 2017 Nov 28;68(20):5485–5495.
- Cai Q, Qiao L, Wang M, et al. Plants send small RNAs in extracellular vesicles to fungal pathogen to silence virulence genes. Science. 2018 Jun 08;360(6393):1126–1129.
- Shahid S, Kim G, Johnson NR, et al. MicroRNAs from the parasitic plant Cuscuta campestris target host messenger RNAs. Nature. 2018 Jan 04;553:82–85.
- Zhou W, Woodson M, Neupane B, et al. Exosomes serve as novel modes of tick-borne flavivirus transmission from arthropod to human cells and facilitates dissemination of viral RNA and proteins to the vertebrate neuronal cells. PLoS Pathog. 2018;14(1):e1006764.
- Philip A, Ferro VA, Tate RJ. Determination of the potential bioavailability of plant microRNAs using a simulated human digestion process. Mol Nutr Food Res. 2015;59(10):1962–1972.
- Chen X, Dai GH, Ren ZM, et al. Identification of dietetically absorbed rapeseed (Brassica campestris L.) bee pollen microRNAs in serum of mice. Biomed Res Int. 2016;1–6. Article ID 5413849.
- Teng Y, Ren Y, Sayed M, et al. Plant-derived exosomal microRNAs shape the gut microbiota. Cell Host Microbe. 2018 Nov 14;24(5):637–652.e8.
- Chen X, Wu RZ, Zhu YQ, et al. Study on the inhibition of Mfn1 by plant-derived miR5338 mediating the treatment of BPH with rape bee pollen. BMC Complement AlternMed. 2018;18(1):38.
- Ju S, Mu J, Dokland T, et al. Grape exosome-like nanoparticles induce intestinal stem cells and protect mice from DSS- induced colitis. Mol Ther. 2013;21(7):1345–1357.
- Wang W, Hang C, Zhang Y, et al. Dietary miR-451 protects erythroid cells from oxidative stress via increasing the activity of Foxo3 pathway. Oncotarget. 2017;8(63):107109–107124.
- Hou D, He F, Ma L, et al. The potential atheroprotective role of plant MIR156a as a repressor of monocyte recruitment on inflamed human endothelial cells. J Nutr Biochem. 2018;57:197–205.
- Zhang H, Li Y, Liu Y, et al. Role of plant microRNA in cross-species regulatory networks of humans. BMC Syst Biol. 2016;10:60.
- Cavalieri D, Rizzetto L, Tocci N, et al. Plant microRNAs as novel immunomodulatory agents. Sci Rep. 2016;6:25761.
- Chevillet JR, Kang Q, Ruf IK, et al. Quantitative and stoichiometric analysis of the microRNA content of exosomes. Proc Natl Acad Sci USA. 2014;111:14888–14893.
- Landgraf P, Rusu M, Sheridan R, et al. A mammalian microRNA expression atlas based on small RNA library sequencing. Cell. 2007;129:1401–1414.
- Williams Z, Ben-Dov IZ, Elias R, et al. Comprehensive profiling of circulating microRNA via small RNA sequencing of cDNA libraries reveals biomarker potential and limitations. Proc Nat Acad Sci. 2013;110:4255–4260.
- Brown BD, Gentner B, Cantore A, et al. Endogenous microRNA can be broadly exploited to regulate transgene expression according to tissue, lineage and differentiation state. Nat Biotechnol. 2007;25:1457–1467.
- Mullokandov G, Baccarini A, Ruzo A, et al. High-throughput assessment of microRNA activity and function using microRNA sensor and decoy libraries. Nat Methods. 2012;9:840–846.
- Landthaler M, Gaidatzis D, Rothballer A, et al. Molecular characterization of human Argonaute-containing ribonucleoprotein complexes and their bound target mRNAs. RNA. 2008;14:2580–2596.
- Baccarini A, Chauhan H, Gardner TJ, et al. Kinetic analysis reveals the fate of a microRNA following target regulation in mammalian cells. Curr Biol. 2011;21:369–376.
- Chen C, Ridzon DA, Broomer AJ, et al. Real-time quantification of microRNAs by stem–loop RT–PCR. Nucleic Acids Res. 2005 Nov 1;33(20):e179.
- Kim D, Sung YM, Park J, et al. General rules for functional microRNA targeting. Nat Genet. 2016 Dec;48(12):1517–1526.
- Brown BD, Naldini L. Exploiting and antagonizing microRNA regulation for therapeutic and experimental applications. Nat Rev Genet. 2009;10:578–5.
- Bissels U, Wild S, Tomiuk S, et al. Absolute quantification of microRNAs by using a universal reference. RNA. 2009 Dec;15(12):2375–2384.
- Anand PK, Anand E, Bleck CK, et al. Exosomal Hsp70 induces a pro- inflammatory response to foreign particles including mycobacteria. PloS One. 2010;5:e10136.
- Gastpar R, Gehrmann M, Bausero MA, et al. Heat shock protein 70 surface-positive tumor exosomes stimulate migratory and cytolytic activity of natural killer cells. Cancer Res. 2005;65:5238–5247.
- Christianson HC, Svensson KJ, Van Kuppevelt TH, et al. Cancer cell exosomes depend on cell-surface heparan sulfate proteoglycans for their internalization and functional activity. Proc Natl Acad Sci USA. 2013;110:17380–17385.
- Atay S, Banskota S, Crow J, et al. Oncogenic KIT-containing exosomes increase gastrointestinal stro- mal tumor cell invasion. Proc Natl Acad Sci USA. 2014;111(2):711–716.
- Purushothaman A, Bandari SK, Liu J, et al. Fibronectin on the surface of myeloma cell-derived exosomes mediates exosome-cell interactions. J Biol Chem. 2016;291:1652–1663.
- Lundy SK, Taitano SH, Van der Vlugt LEPM. Exosomes from B lymphocytes are a major source of Fas ligand. J Immunol. 2017 May 1;198(1 Supplement):80.6.
- Higginbotham JN, Beckler DM, Gephart JD, et al. Amphiregulin exosomes increase cancer cell invasion. Curr Biol. 2011;21:779–786.
- Gross JC, Chaudhary V, Bartscherer K, et al. Active Wnt proteins are secreted on exosomes. Nat Cell Biol. 2012;14:1036–1045.
- McBride JD, Rodriguez-Menocal L, Guzman W, et al. Bone marrow mesenchymal stem cell-derived cd63(+) exosomes transport wnt3a exteriorly and Enhance Dermal Fibroblast Proliferation, Migration, and angiogenesis in vitro. Stem Cells Dev. 2017;26:1384–1398.
- Pasquale EB. Exosomes expand the sphere of influence of Eph receptors and ephrins. J Cell Biol. 2016;214:jcb. 201606074.
- Webber J, Steadman R, Mason MD, et al. Cancer exosomes trigger fibroblast to myofibroblast differentiation. Cancer Res. 2010;70:9621–9630.
- Wada J, Onishi H, Suzuki H, et al. Surface-bound TGF- beta-1 on effusion-derived exosomes participates in maintenance of number and suppressive function of regulatory T-cells in malignant effusions. Anticancer Res. 2010;30:3747–3757.
- Shelke GV, Yin Y, Jang SC, et al. Endosomal signaling via exosome surface TGF-b. J Extracell Vesicles. 2019;8(1):1650458.
- Cossetti C, Iraci N, Mercer TR, et al. Extracellular vesicles from neural stem cells transfer IFN-γ via Ifngr1 to activate Stat1 signaling in target cells. Mol Cell. 2014;56:193–204.
- Bryniarski K, Ptak W, Sikora E, et al. Free extracellular miRNA functionally targets cells by transfecting exosomes from their companion cells. PLoS One. 2015;10(4):e0122991.
- Bryniarski K, Ptak W, Jayakumar A, et al. Antigen-specific, antibody-coated, exosome-like nanovesicles deliver suppressor T-cell microRNA-150 to effector T cells to inhibit contact sensitivity. J Allergy Clin Immunol. 2013;132:170–181. e179.
- Marchal G, Seman M, Milon G, et al. Local adoptive transfer of skin delayed-type hypersensitivity initiated by a single T lymphocyte. J Immunol. 1982;129:954–958.
- Buzás EI, Tóth EÁ, Sódar BW, et al. Molecular interactions at the surface of extracellular vesicles. Semin Immunopathol. 2018;40(5):453–464.
- Lázaro-Ibáñez E, Lässer C, Shelke GV, et al. DNA analysis of low- and high-density fractions defines heterogeneous subpopulations of small extracellular vesicles based on their DNA cargo and topology. J Extracell Vesicles. 2019 Aug 27;8(1):1656993.
- Wang K, Yuan Y, Cho JH, et al. Comparing the microRNA spectrum between serum and plasma. PLoS One. 2012;7:e41561.
- Pulliam L, Gupta A. Modulation of cellular function through immune-activated exosomes. DNA Cell Biol. 2015;34(7):459–463.
- De Silva N, Samblas M, Martínez JA, et al. Effects of exosomes from LPS-activated macrophages on adipocyte gene expression, differentiation, and insulin-dependent glucose uptake. J Physiol Biochem. 2018 Nov;74(4):559–568.
- Genschmer KR, Russell DW, Lal C, et al. Activated PMN exosomes: pathogenic entities causing matrix destruction and disease in the Lung. Cell. 2019;176(1–2):113–126.e15.
- Hutchinson AT, Jones DR, Raison RL. The ability to interact with cell membranes suggests possible biological roles for free light chain. Immunol Lett. 2012;142:75–77.
- Hutchinson AT, Ramsland PA, Jones DR, et al. Free Ig light chains interact with sphingomyelin and are found on the surface of myeloma plasma cells in an aggregated form. J Immunol. 2010;185:4179–4188.
- Hutchinson AT, Malik A, Berkahn MB, et al. Formation of assemblies on cell membranes by secreted proteins: molecular studies of free λ light chain aggregates found on the surface of myeloma cells. Biochem J. 2013;454:479–489.
- Li N, Huang Z, Zhang X, et al. Reflecting size differences of exosomes by using the combination of membrane-targeting viscosity probe and fluorescence lifetime imaging microscopy. Anal Chem. 2019;91(23):15308–15316.