ABSTRACT
Updates in whole genome sequencing technologies have revealed various RNA modifications in cancer, among which RNA methylation is a frequent posttranscriptional modification. RNA methylation is essential for regulating biological processes such as RNA transcription, splicing, structure, stability, and translation. Its dysfunction is strongly associated with the development of human malignancies. Research advances with respect to the regulatory role of RNA modifications in ovarian cancer include N6-methyladenosine (m6A), 5-methylcytosine (m5C), N1-methyladenosine (m1A), and N7-methylguanosine (m7G). Numerous studies have demonstrated that epigenetic modifications of RNA can influence the progression and metastasis of ovarian cancer and may provide excellent targets for cancer therapy. This review highlights advances in research on RNA methylation modifications and ovarian cancer prognosis, carcinogenesis, and resistance, which could provide a theoretical foundation for designing therapeutic strategies for ovarian cancer based on RNA methylation modifications.
Introduction
One of the three main gynaecologic malignancies, ovarian cancer (OC), is also the most common cause of death from gynaecologic tumours [Citation1]. According to statistics, there will be approximately 53,342 new cases and approximately 37,519 deaths from OC in China in 2020, accounting for 17.63% and 18.10% of the global cases respectively, and the cancer burden will increase annually [Citation2]. However, prognosis is dismal because ovarian cancer is usually advanced when diagnosed due to the lack of early diagnostic methods. Despite improvements in surgery, chemotherapy, novel immunotherapies, and overall survival at all stages, various molecular processes in ovarian cancer that contribute to tumour growth and therapeutic targets are still unknown [Citation3,Citation4].
DNA, RNA and protein postsynthetic chemical modifications are involved in alterations at three levels: transcriptional, posttranscriptional and posttranslational, respectively. Of these, posttranscriptional regulation involves RNA modifications and ncRNA, and this mechanism of translational control is known as epitranscriptomics. Over the past five decades, more than 100 RNA postsynthetic modifications have been identified in different RNA classes [Citation5]. All kinds of known RNA have been discovered to be modified, with the vast majority of modifications occurring only in ribosomal RNA (rRNA) and/or transfer RNA (tRNA). Recent studies suggest that RNA modifications may affect molecular processes such as RNA metabolism, splicing, stability, and translation [Citation6,Citation7]. Methylation modifications are among the most abundant RNA modifications. The most common internal modifications in eukaryotic RNAs include N6-methyladenosine (m6A), 5-methylcytosine (m5C), 7-methylguanosine (m7G), N1-methyladenosine (m1A) and others [Citation8]. mRNA modifications, especially m6A modifications, are the most prevalent internal modifications in mRNAs. The primary roles of m6A are to regulate DNA damage, proliferation, cell survival, migration and invasion, progression, and chemoresistance in cancer [Citation9].
The dynamic modification process of RNA necessitates the involvement of numerous particular proteins known as RNA modifying proteins (RMPs). Among these, methyltransferases (also known as writers), demethylases (commonly known as erasers), and binding proteins (usually known as readers) are involved in the insertion, deletion, and recognition of RNA modifications, respectively [Citation7,Citation10]. The advent of analytical methods such as MeRIP-seq, miCLIP, and other specific sequencing allows for the study of the location and quality of methylation modifications on RNA, as well as the identification of writers, erasers, and readers that modulate the transcriptomic mark [Citation11,Citation12]. RNA methylation modifications and their associated regulatory proteins are aberrantly expressed in cancer and are crucial to the development of cancer [Citation13,Citation14]. According to the RNA-seq data used by GEPIA (https://gepia.cancer-pku.cn), the RNA modification proteins YTHDC1/2, HNRNPCA2B1, IGF2BP2/3 and YBX1 were significantly highly expressed and only METTL3/16 expressed at low levels in ovarian cancer tissues; other regulatory enzymes were not significantly differentially expressed. Interestingly, these regulators show inconsistent expression trends and RNA metabolic effects in other tumours. For instance, catalytic m6A-modified methyltransferase-like 3 (METTL3) is highly expressed in malignancies such as cholangiocarcinoma, thymoma, and diffuse large B-cell tumours according to the GEPIA website (https://gepia.cancer-pku.cn). Previous evidence suggests that METTL3 mediates IFIT2 mRNA decay in an m6A-dependent manner to inhibit the progression of intrahepatic cholangiocarcinoma [Citation15]. Aly/REF Export Factor (ALYREF) promotes mRNA export by acting as a reader of m5C and is associated with prognosis in hepatocellular and glial carcinomas [Citation16–18]. Similarly, promoter hypermethylation of the m1A demethylase ALKBH3 is an independent factor associated with poor prognosis in Hodgkin’s lymphoma [Citation19]. METTL1 and WDR4 are complex catalyst of m1A modification, and METTL1 overexpression in bladder cancer is linked to a poor prognosis and mediates m7G tRNA modification [Citation20].
Numerous investigations have confirmed that RNA methylation modifications, such as m6A, m5C, m1A, and m7G, are related to various cancers and are involved in their biological processes [Citation13]. However, research on the role of RNA methylation in the progression of ovarian carcinogenesis remains incomplete. In this review, we will discuss the cellular functions and molecular mechanisms of RNA modifications in regulating gene expression in ovarian cancer. Our review will focus on m6A, m5C, m1A, and m7G modified coding and noncoding RNAs, with emphasis on the molecular processes of these RMPs in cell proliferation, migration and invasion, stem cell differentiation, immunity, and drug resistance of OC. In addition, RNA-modified regulators will also be of interest as potential molecular targets and therapeutic targets for ovarian cancer will also be of interest.
RNA methylation in ovarian cancer
N6-methyladenosine methyltransferase
The methyltransferase complex (MTC), which has METTL3 as its core methyltransferase subunit, is responsible for installation of m6A on mRNA. Regulators such as METTL14/16, Wilms tumor1 associated protein (WTAP), VIRMA(KIAA1429), RNA binding motif protein 15(RBM15), and zinc finger CCCHType Containing 13 (ZC3H13) perform different biological functions during m6A modification. The regulator METTL3 is responsible for catalysing m6A modification [Citation21]. METTL3 demonstrates hypomethylation and elevated expression in the tissues and cells of ovarian cancer [Citation22]. METTL3 has been shown in various studies to contribute to the tumorigenesis of ovarian cancer. In addition, METTL3 mediates m6A modification in endometrioid epithelial ovarian cancer(EEOC) without the assistance of WTAP or METTL14 [Citation23]. METTL14 can assist METTL3 in substrate recognition and expressed at low levels in ovarian cancer [Citation24,Citation25]. New studies have shown that METTL16 catalyzes m6A on mRNAs in the nucleus and encourages mRNA translation in the cytoplasm [Citation26]. The METTL3-METTL14 heterodimer is promoted by WTAP to the nuclear speckle [Citation27]. VIRMA directs methyltransferase components to specific RNA regions [Citation24]. WTAP and VIRMA are substantially expressed in ovarian cancer and correlate with prognosis [Citation28,Citation29]. RBM15 recruits the complex to specific RNA locations by binding the m6A complex, and its increased expression is associated with ovarian cancer metastasis [Citation30,Citation31]. ZC3H13 anchors the complex containing WTAP in the nucleus to promote m6A modification [Citation32]. In ovarian cancer, the expression of ZC3H13 was found to correspond with poor overall survival of patients and the degree of immune infiltration [Citation31,Citation33].
N6-methyladenosine demethylase
Fat mass and obesity-associated protein (FTO), as the first discovered ‘eraser’, was found to delete the m6A modification by using the nuclear RNA form of m6A as a substrate [Citation34]. In addition, ALKBH5, another demethylase in the ALKB family, was found to significantly affect mRNA export and RNA metabolism in mammals [Citation35]. Patients with ovarian cancer had low levels of FTO and ALKBH5, whereas those with higher levels of both presented shorter overall survival and progression-free survival (PFS) [Citation36]. In various ovarian cancer studies, the erasers FTO and ALKBH5 regulate various biological processes.
N6-methyladenosine reader
Identified ‘readers’ that recognize m6A modification sites include the YTHDF1/2/3 family of YT521-B homologous (YTH) structural domain proteins, the YTH structural domain protein YTHDC1/2, the insulin-like growth factor 2 mRNA binding protein IGF2BP1/2/3 and the heterogeneous nuclear ribonucleoprotein (HNRNP) family. While YTHDF1 enhances mRNA translation and YTHDF2 reduces mRNA stability, YTHDF3 synergizes with YTHDF1 to facilitate protein synthesis and affects YTHDF2-mediated mRNA decay [Citation37,Citation38]. YTHDF1 and YTHDF2 are significantly expressed in ovarian cancer and may be associated with prognosis, while YTHDF3 may increase the pathological staging of ovaries [Citation39,Citation40]. YTHDC1 promotes RNA splicing and translocation, and YTHDC2 enhances the translation of target RNAs [Citation41,Citation42]. YTHDC1 promotes pre-RNA splicing and export; YTHDC2 enhances context-dependent mRNA translation or recognizes partial mRNAs to accelerate their degradation [Citation41,Citation42]. IGF2BP1/2/3 recognizes m6A sites through specific KH structural domains and enhances mRNA stability and translation [Citation43]. IGF2BP1 enhances OC cell invasion by antagonizing miRNA damage gene expression [Citation44]. The HNRNP family contains HNRNPC, HNRNPG and HNRNPA2B1, which modulate pre-mRNA processing through the ‘m6A switch mechanism’ [Citation45,Citation46]. The ability of HNRNPA2B1 to bind to the vicinity of m6A sites is enhanced to facilitate nuclear events such as primary microRNA processing [Citation47]. HNRNPA2B1 promotes the OC malignant phenotype through upregulation of Lin28B expression [Citation48]. In addition, HNRNPC is considered a predictor of paclitaxel resistance [Citation31].
5-methylcytosine
5-Methylcytosine (m5C), the methylation of cytidine at position 5, is a posttranscriptional modification. The NOP/SUN RNA methyltransferase (NSUN) family, which includes members NSUN1 through NSUN7, DNA methyltransferase 2 (DNMT2), and tRNA aspartic acid methyltransferase 1, is responsible for catalysing the m5C modification [Citation49]. NSUN2, a major mRNA methyltransferase, is associated with stress response pathways and stem cell differentiation, and it may impact the growth, maintenance, and progression of tumours [Citation50]. DNMT3A/3B interacts with microRNA-29b in a double negative feedback manner, leading to OC progression [Citation51]. m5C-modified binding proteins (YTHDF2, ALYREF, YBX1) participate in m5C modification in a methylase-dependent or independent manner. According to reports, ALYREF is a specific mRNA m5C binding protein [Citation16]. Y-box binding protein 1(YBX1) maintains RNA stability by recruiting the ELAVL1 protein to recognize m5C modifications. YBX1 nuclear translocation is regulated by Akt activation and affects the expression of drug resistance genes in OC cells [Citation52]. In ovarian cancer, the potential role of m5C regulators may influence metabolic heterogeneity and drug chemotherapy sensitivity and correlate with prognosis in OC patients [Citation53].
N1-methyladenosine
N1-methyladenosine (m1A) is the methylation of the nitrogen atom at position 1 of adenosine on tRNA. Transfer RNA methyltransferase 10 (TRMT10) and the transfer RNA methyltransferase 6/61 (TRM6/61) complex are currently known methyltransferases. Different studies have shown that m1A is mainly present in the 5’-UTR structural domain associated with high levels of translation, while other m1A sites are mostly concentrated in the CDS and 3’-UTR regions [Citation54,Citation55]. m1A modifications on tRNAs can be deleted by ALKBH1/3 [Citation56,Citation57]. In ovarian cancer, ALKBH3-mediated m1A demethylation enhances CSF-1 mRNA stability [Citation58]. In addition, studies have confirmed that YTHDF2 can recognize specific methylation by m1A and affect transcript stability [Citation59].
7-methylguanosine
The majority of 7-methylguanosine (m7G) is located at the 5’ terminal structure (also called the “cap”) of mRNA, and the “cap” reduces exonuclease degradation to improve mRNA stability [Citation60–62]. WDR4, RNMT, RAM, WBSCR22, and TMRT112 have been identified as m7G methyltransferases [Citation63,Citation64]. Global translation is ultimately controlled by the METTL1/WDR4 complex, which adds m7G modifications to mRNA (internal site), tRNA (G46 site), and miRNA (G-quadruplex structure). The RNMT/RAM complex regulates m7G modifications of the 5’ cap of mRNA, which mediates the process of mRNA nuclear export and translation. The WBSCR22/TMRT112 complex adds m7G modifications at the G1638 site of 18S rRNA to promote 18S rRNA maturation [Citation65]. These m7G regulators play different roles in various other cancers. show the roles of m6A, m5C, m1A and m7G modified proteins in RNA metabolism.
Relationship between RNA methylation modifications and the prognosis of ovarian cancer
To explore the clinicopathological characteristics and prognostic values of methylation regulators in ovarian cancer, the cBioPortal tool was used to evaluate RNA-seq and clinicopathological data from TCGA, consensus clustering to distinguish expression, regression models to determine prognostic relevance, ROC curves, and risk scores to predict survival, and the TIMER online database and nomogram were used to integrate data. These analyses suggest that RNA m6A methylation regulators are associated with clinicopathology and prognosis in ovarian cancer [Citation29]. A meta-analysis showed that aberrant METTL3 expression in different cancers, including ovarian cancer, tended to predict poor prognosis [Citation66]. Comprehensive biological information analysis and gene enrichment analysis (GSEA) showed that high WTAP expression was significantly associated with poor outcomes in ovarian cancer and cell cycle regulation [Citation67]. According to the study, IGF2BP2, KIAA1429, and YTHDF1 regulators are highly amplified in OC, and the majority of m6A genes, including METTL3, KIAA1429, HNRNPC, ZC3H13, and IGF2BP2, are upregulated in ovarian cancer tissues. These m6A-modified proteins could be clinical prognostic factors in ovarian cancer [Citation68]. In OC, the m6A regulators IGF2BP1, VIRMA, and ZC3H13 were connected to prognosis and the tumour-associated WNT pathway [Citation69]. More studies have shown that high expression levels of WTAP, FTO, ALKBH5, and YTHDF3 are related to shorter OS and PFS, and the m6A action of RBM15B, ZC3H13, YTHDF1, and IGF2BP1 may mediate immune infiltration [Citation31]. METTL14 was highly expressed in samples from individuals with EOC and was associated with a poorer prognosis [Citation70]. Microarray data analysis revealed that METTL14 was less enriched in OC tissues than in normal tissues, and its low expression reduced overall patient survival [Citation25]. According to risk score models, patients with ovarian cancer who had low VIRMA or high HNRNPA2B1 expression had prolonged half survival, and the constructed miRNA-m6A regulator (VIRMA, IGF2BP1, HNRNPA2B1)-target gene regulatory network could predict the prognosis of OC patients [Citation71]. In addition, noncoding RNA lncRNAs play an essential role in the development of various cancers, and m6A is the most widely modified modality. Yang et al. investigated the potential mechanisms involved in m6A modification of lncRNAs in OC and identified seven m6A-associated lncRNA gene signatures that could predict the prognosis of OC patients [Citation72,Citation73]. In the most recent study, an RNA modification pattern scoring system (called the RMW score) based on 26 RNA modification ‘writers’ of m6A, m1A, APA, and A-I was created to measure individual OC patients, which correlated with the tumour immune microenvironment (TME), predicted drug sensitivity in OC patients, and predicted survival in combination with prognostic clinical features, and as an individualized treatment strategy [Citation74]. Compared to normal tissues, epithelial ovarian cancer exhibits a high level of m5C expression, and its expression is correlated with pathological stage, tumour grade, and lymph node metastasis [Citation75]. M5C is involved in ovarian cancer progression and correlates with tumour prognosis; among the four m5C regulators, the FC fragment of IgG-binding protein FCGBP and HOXB3 are risk factors for the prognostic risk score, while TYMSOS and CLDN19 are protective factors for the prognostic risk score [Citation76]. Nuclear YBX1 expression level may be an independent factor for poor prognosis of OC patients [Citation77]. The lncRNA COL4A3, which is regulated by m5C modification and enriched in multiple pathways, has a clinically significant relationship with the prognosis of HGSOC [Citation78]. Analyses in public databases and cellular functional characterization verified that overexpression of the m1A-modified reader TRMT10C predicted poorer survival in gynaecologic cancers [Citation79]. The m1A regulators ALKBH1/3, TRMT6, TRMT10C, and YTHDF1/2 are pro-oncogenes, and OC patients with high expression of these regulators have a poor prognosis [Citation80]. In ovarian plasmacytic cystic adenocarcinoma, a substantial correlation was observed between the expression of the m7G modifier METTL1 and OS and disease-specific survival (DSS) [Citation81]. show the main role of regulators of RNA modification related with prognosis of ovarian cancer. In summary, various RNA methylation regulators can predict survival in ovarian cancer patients, which provides a basis for understanding tumour progression, aiding diagnosis, and developing treatment plans.
Table 1. The main role of regulators of RNA modification related with prognosis of ovarian cancer.
Role of RNA methylation modifications in ovarian cancer carcinogenesis
It is generally known that m6A plays a crucial role in tumours and display different characteristics in different cancers. In the last decade, m5C modifications have been linked to a variety of cancer types, but the precise mechanisms are still unclear. Although the study of m7G and m1A in cancer is not deep and comprehensive, the critical role of m1A and m7G in the biological processes of tumour cannot be ignored [Citation13,Citation65]. A growing number of studies have shown correlations between RNA methylation and cancer development and progression. The following sections will describe in detail the roles of various RNA methylation regulators in OCs and their effects.
Role of RNA methylation modifications in ovarian cancer proliferation, invasion and migration, and apoptosis
Methyltransferase-like 3 (METTL3) mediates the maturation and upregulation of miR-126-2p to activate the PI3K/Akt/mTOR pathway by directly binding to phosphatase and tensin homologues (PTEN) to promote ovarian cancer progression and tumorigenesis; moreover, knockdown of METTL3 inhibits ovarian tumour formation in vivo [Citation22]. Downregulation of METTL3 inhibited cell proliferation, colony formation, and invasion, induced apoptosis, and suppressed AKT pathway activation in ovarian cancer [Citation85]. In the EOC cell lines A2780 and SKOV3, high expression of METTL14 promoted cell proliferation invasion and migration [Citation70]. METTL14 targeted TROAP mRNA through m6A modification to reduce stability, thus inhibiting cell proliferation and suppressing the proliferative capacity of ovarian cancer cells in vitro and in vivo [Citation25]. Interestingly, METTL14 and WTAP expression did not differ significantly in EEOC and adjacent tissues [Citation23]. Hypoxia-inducible factor (HIF)-1α activates WTAP transcription causing enhanced proliferation and migration of ovarian cancer in vitro, and WTAP targets DGCR8 in an m6A-modified manner to promote maturation of precursor miRNAs [Citation86]. LncRNA ubiquitination modifier activator 6 antisense RNA1 (UBA6-AS1) recruits RBM15 to mediate UBA6 m6A methylation, and IGF2BP1 enhances UBA6 stability by recognizing its m6A modification, ultimately encouraging ovarian cancer cell proliferation, invasion, and migration [Citation87]. Coculture of OC cells with M2 macrophages enhanced the proproliferative and antiapoptotic ability of ALKBH5 and promoted tumour growth in vivo. Increased NANOG RNA expression by ALKBH5 demethylation leads to enhanced aggressiveness of OC cells [Citation82]. ALKBH5 enhances BCL-2 mRNA stability in an m6A-demethylation manner and promotes autophagy-inhibiting complex Beclin1-BCL-2 interactions. High ALKBH5 level expression in OC tissues mediates BCL-2 upregulation, which regulates OC proliferation, invasion and autophagy, and controls the mTOR pathway involved in autophagy [Citation88]. Knockdown of YTHDF1 reduced OC cell, invasive and migratory abilities in vitro, and inhibited tumorigenesis and metastasis in vivo. YTHDF1 recognizes the target mRNA EIF3C in an m6A-dependent manner to facilitate its protein synthesis and plays an essential role in ovarian cancer growth and migration [Citation39]. YTHDF2 significantly downregulated total mRNA m6A levels to enhance EOC cell proliferation and migration, and its overexpression rescued the tumour suppressive effect induced by the target gene miR-145 [Citation89]. Recent research reports that the tumour suppressor FBW7 mediates YTHDF2 protein hydrolysis via ubiquitination to inhibit ovarian cancer proliferation and growth; In contrast, FBW7 inhibits BMF mRNA degradation in an m6A-YTHDF2 manner to support ovarian cancer apoptosis, which is a downstream target of YTHDF2 [Citation40]. Codifferentially expressed genes with overlapping m5C peaks and RNA-seq in plasmacytoid ovarian cancer correlated with cell growth, maintenance, cytoplasmic membrane, and cell adhesion molecule activity [Citation83]. The results in the GEO and TCGA databases showed that NSUN2 was significantly upregulated in ovarian cancer, and its protein levels were positively correlated with cancer stage. At the same time, there was no effective regulation of cell proliferation and migration in ovarian cancer [Citation90]. Knockdown of the m1A writer TRMT10C inhibited proliferation, colony formation, and migration of ovarian and cervical cancer cells [Citation79]. Moreover, the m1A ‘eraser’ ALKBH3 enhanced the stability of cytokine CSF-1 and the invasive ability of cancer cells but it had no effect on cell proliferation and migration [Citation58] (). Obviously, these regulators have different or even opposite effects on the biological ability of ovarian cancer including proliferation, invasion, migration, and apoptosis under different mechanisms of action. This dynamic modification of RNA methylation reminds us of the importance of maintaining proper balance and avoiding excessive dysregulation of one regulator.
Figure 2. Diagram of RNA modification regulators important for ovarian cancer growth, invasion, migration, and apoptosis. By hindering the miR-126-5p-targeted suppression of PTEN and hence obstructing the PI3K/Akt/mTOR pathway, METTL3 aids in the development and carcinogenesis of ovarian cancer. The AKT pathway was activated by METTL3, which also encouraged proliferation, invasion, and migration. By focusing on TROAP, METTL14 encouraged procreation, invasion, and migration while hindering procreation capacity. HIF-1‘s upregulation of WTAP expression interferes with miRNA processing and speeds up tumour growth, invasion, and migration. By enlisting RBM15, UBA6-AS1 boosted the m6A methylation of UBA6 mRNA. IGF2BP1 was discovered to be the m6A reader protein of the UBA6-AS1-RBM15-mediated m6A modification of UBA6 mRNA, which improved UBA6 mRNA stability and inhibited the proliferation, migration, and invasion of OC cells functionally. YTHDF1 aided in the carcinogenesis of ovarian cancer by promoting the proliferation, invasion, and migration of cells, as well as increasing the translation of EIF3C in an m6A-dependent manner. MiR-145 inhibited YTHDF2‘s ability to increase the proliferation and migration of EOC cell lines, and FBW7 inhibits YTHDF2-mediated BMF mRNA degradation in ovarian cancer to slow tumour growth and development. Through raising NANOG mRNA expression, ALKBH5 encouraged OC cells to be aggressive and anti-apoptosis. The expression of ALKBH5 was increased, which facilitated the growth and invasion of epithelial ovarian cancer cells. ALKBH5 also activates the mTOR pathway to control autophagy and facilitates the connection between Bcl-2 and Beclin1, which is dependent on m6A. In ovarian cancer, NSUN2 did not significantly control cell migration and proliferation. TRMT10C encouraged OC migration, invasion, and proliferation. In order to encourage the invasiveness of ovarian cancer cells, ALKBH3 improved the stability of CSF-1.
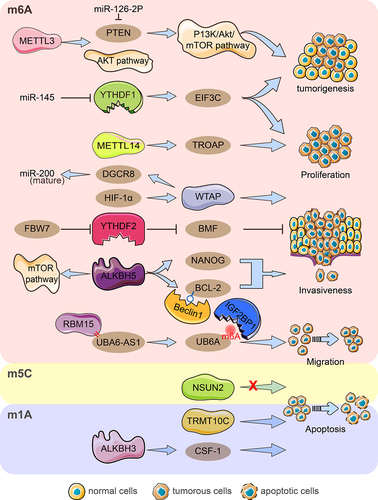
Role of RNA methylation modifications in cancer stem cells, metastasis, tumour microenvironment, and immune infiltration
Using various biological investigations, 21 m6A regulators were divided into two groups: those with low m6A scores and those with high m6A scores. Low m6A scores were associated with immune infiltration and responses to immune detection site inhibitors (ICIs) [Citation91]. RNA modification patterns in ovarian cancer characterized by high immune infiltration and immune activation showed higher RNW scores, which were able to predict patient response to immunotherapy [Citation74]. It has been suggested that m6A modification is linked to cellular immune infiltration and immune gene labelling. The m6A regulators RBM15, ZC3H13, YTHDF1, and IGF2BP1 may cause ovarian cancer immune infiltration [Citation31]. In another study, phospholipase A2 activating protein (PLAA) degraded METTL3 via the ubiquitination pathway, which resulted in the destabilization of the m6A-modified mRNA oncogene TRPC3 and prevented the metastasis of ovarian cancer cells [Citation92]. FTO is expressed at low levels in ovarian cancer stem cells (OCSC), and its overexpression inhibits OCSC proliferation, self-renewal and spherogenesis, and blocks cAMP signalling dependent on m6A demethylation [Citation93]. TRIM29 expression levels correlate with prognosis in OC and enhance CSC-like features in cisplatin-resistant OC cells; YTHDF1 promotes TRIM29 translation in an m6A-dependent manner which contributes to the stem cell-like phenotype in cisplatin-resistant ovarian cancer [Citation94]. Ovarian cancer cells cocultured with M2 macrophages showed the increased expression of the molecular toleration-like receptor (TLR4) in the tumour microenvironment (TME), which upregulated ALKBH5 by activating the NF-κB pathway, and eventually promoted ovarian carcinogenesis in the mock TME [Citation82]. HIF-1α upregulation of WTAP expression interferes with miRNA processing and accelerates the Warburg effect (glucose metabolism by regulating glycolytic enzymes) in ovarian cancer in an m6A-dependent manner [Citation86]. In addition, one study found that RBM15 upregulation and YTHDC1 downregulation were associated with OC cell metastasis [Citation31]. In a retrospective study, GSEA found that high WTAP expression was enriched in MYC targets and cell cycle regulation-related pathways [Citation67]. Different m1A modification patterns had various immune infiltration profiles, with m1A cluster B having the most abundant cellular immune infiltration and the greatest sensitivity to immunotherapy [Citation80] (). According to other studies, m6A methylation promotes immunosuppressive TME properties and supports tumour proliferation through pathways involving hypoxia, metabolic dysregulation, immune cells, and stem cell function. HIF affects tumour cells through m6A methylation modifications under hypoxic conditions. Tumour cells under hypoxia conditions promote the CSC phenotype and contribute to immunosuppressive TME formation. m6A-mediated metabolic dysregulation produces an acidic environment that further supports tumour growth and exacerbates tumour hypoxia. In addition, m6A methylation directly regulates immune cells and promotes the progressive establishment of an immunosuppressive TME.
Figure 3. Diagram illustrating the role of RNA modification regulators in ovarian cancer spread, OCSC pathogenesis, TME, and immune infiltration. Metastasis: Through METTL3-mediated m6A alteration of TRPC3 mRNA, PLAA inhibits ovarian cancer metastasis. RBM15 encouraged metastasis of OC, while YTHDF1 inhibited it. CSC: In ovarian cancers and CSC, FTO expression is reduced, and the cAMP pathway which is important in stemness and tumour initiation is controlled. The cisplatin-resistant ovarian cancer cells’ stem cell-like phenotype is made possible by TRIM29 overexpression caused by m6A-YTHDF1. TME: TLR4 upregulates ALKBH5 expression in ovarian cancer cells co-cultured with M2 macrophages via activating the NF-B pathway, eventually driving ovarian carcinogenesis in the TME. Upregulation of WTAP expression by HIF-1α intercedes with miRNA processing, accelerates the Warburg impact. Immune infiltration: In ovarian cancer, YTHDF1, RBM15, ZC3H13, IGF2BP2, and the m1A-B cluster cloud mediate various degrees of immune infiltration.
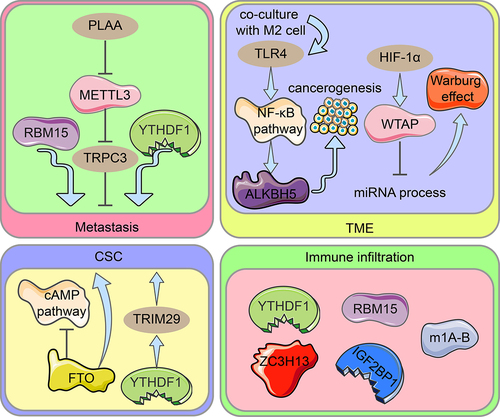
RNA methylation modifications as potential drug targets in ovarian cancer
Various studies have shown that RNA methylation is linked to tumour growth and may be used as a prognostic indicator for various cancers. FTO inhibitors have been most abundantly studied. For instance, Rhein was the first identified competitive inhibitor for FTO that can increase the cellular m6A on mRNA. It reversibly binds to the FTO or ALKB to form a complex and prevent the recognition of m6A substrates inside cells [Citation95]. MA, a nonsteroidal anti-inflammatory drug, inhibits FTO demethylation of an m6A-containing ssDNA or ssRNA by inhibiting FTO rather than ALKBH5 [Citation96]. Two FTO inhibitors discovered by using structure-based rational design, FB23 and FB23–2, can directly bind to FTO protein and selectively inhibit the m6A demethylase activity of FTO [Citation97]. Similarly, two FTO inhibitors developed after screening, structural optimization and bioassays, 18077 and 18,097, selectively inhibited FTO demethylase activity and showed anticancer activity [Citation98]. R-2-hydroxyglutaric acid (R-2HG) targets binding to FTO to inhibit demethylase activity and anti-tumour effects of 2HG in inhibiting the proliferation/survival of FTO-high cancer cells by targeting FTO/m6A/MYC/CEBPA signalling [Citation99]. The nucleotide mimic with the tethered 2OG-binding component exhibited good potential in inhibiting FTO activity [Citation100]. In addition, small molecule activators of METTL3 and inhibitors of METTL3, ALKBH5, and IGF2BP1 have been validated. tANK-binding kinase 1 (TBK1) interacts with METTL3 at serine 67 and phosphorylates METTL3 to promote its translational function [Citation101]. S-adenosyl homocysteine (SAH) is an S-adenosyl methionine (SAM) dependent methyltransferase inhibitor. Eltrombopag noncompetitively binds to the metastable binding site on the METTL3-METTL14 complex to inhibit its m6A modification activity [Citation102]. ALKH5 (ALK-04), a specific inhibitor of ALKBH5, enhances immunotherapeutic efficacy [Citation103]. The small molecule compound ‘7773’ can directly bind to the RRM12 and KH34 structural domains on IGF2BP1 to affect the binding of other RNA targets [Citation104]. Thus, various regulators of RNA methylation may serve as biomarkers for ovarian cancer diagnosis and potential drug targets for individualized therapy. Although inhibitors or activators of these RNA methylation regulators are expected to improve clinical outcomes for patients with tumours, insufficient experimental data and the lack of clinical trials will make it difficult for these therapeutic strategies to work.
In EOC, the HOXA10-ALKBH5 positive feedback loop promotes cellular cisplatin resistance in vivo and in vitro. ALKBH5 removes the m6A modification of JAK2 mRNA and inhibits YTHDF2-mediated JAK2 degradation to maintain JAK2 mRNA expression, which further activates the JAK2/STAT3 signalling pathway to promote EOC growth and cisplatin drug resistance [Citation105]. m6A-modified FZD10 mRNA was upregulated in PARPi-resistant cells and knockdown of FZD10 inhibited Wnt/β-catenin signalling to sensitize drug-resistant cells to PARPi drugs. In addition, inhibition of FTO and ALKBH5 reduced m6A modification which contributed to the upregulation of m6A-FZD10 mRNA [Citation106]. METTL3-mediated m6A modification of lncRNA RHPN6-AS1 enhances cisplatin resistance in ovarian cancer through activation of the PI3K/AKT pathway [Citation84]. The METTL3/YTHDF2 axis promotes degradation of tumour suppressor IFFO1 mRNA in an m6A-dependent manner to promote nucleation of β-catenin and enhance cisplatin resistance in ovarian cancer [Citation107]. HNPNPC was considered a predictor of paclitaxel resistance based on the 6-month relapse-free survival (RFS) of ovarian cancer chemotherapy [Citation31]. In addition, the ubiquitination of the m5C ‘reader’ YBX1 resulted in the destabilization of its modified mRNA and reduced EOC resistance to cisplatin [Citation108] (). MiR-152 and miR-185 jointly promote cisplatin sensitivity in ovarian cancer cells by directly targeting DNMT1 [Citation109]. Negative feedback between miR-143 and DNMT3A regulates cisplatin resistance in ovarian cancer [Citation110]. M7G methylation has also been shown to be involved in tumour chemoresistance. For instance, low METTL1 expression alleviated nasopharyngeal carcinoma resistance to cisplatin and doxorubicin and cervical cancer resistance to 5-fluorouracil, and WRD4 enhanced sorafenib resistance in hepatocellular carcinoma by promoting the EMT process [Citation111–113]. In short, these modulators of RNA methylation as oncogenes (cancer suppressor genes) or targeted proteins to regulate chemotherapy resistance in ovarian cancer, which are expected to become new biomarkers and drug targets for cancer therapy.
Figure 4. An illustration of the regulators of RNA alteration responsible for ovarian cancer medication resistance. The m6A regulator HNRNPC may be related to and be able to foretell OC resistance to paclitaxel. By facilitating JAK2 m6A demethylation, the ALKBH5-HOXA10 loop promotes EOC resistance to cisplatin by activating the JAK2/STAT3 signalling pathway simultaneously. METTL3-mediated m6A modification of RHPN1-AS1 accelerates cisplatin resistance by activating PI3K/AKT pathway. The METTL3/YTHDF2 axis regulated the mRNA stability of IFFO1 in an m6A-dependent manner and enhances cisplatin resistance. The ubiquitination of YBX-1 caused the target m5C-modified mRnas to become unstable, which made EOC cells more sensitive to the chemotherapy drug cisplatin. To increase FZD10 mRNA m6A modification, activate the Wnt/-catenin pathway, and improve PARPi resistance, it was necessary to downregulate the m6A demethylases FTO and ALKBH5.
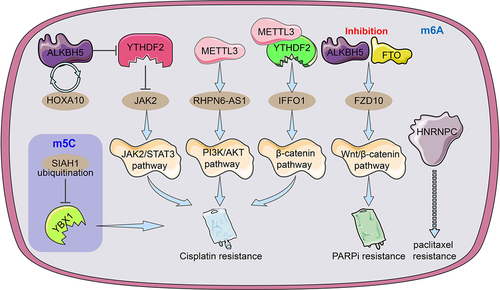
Conclusion and future directions
Overall, the following variables determine how RNA modification of target genes affects the development of ovarian cancer: 1) whether the regulator is an oncogene-promoting or oncogene-suppressing gene; 2) the abnormal level of RNA modification in cancer; and 3) the regulatory mechanism of the target mRNA modification. On account of various methylation modification regulators have different biological properties, some small molecule inhibitors or activators targeting RMPs have emerged that can be involved in regulating the level of RNA modification. It is predicted that RNA methylation might serve as a prospective molecular marker for early diagnosis and individualized treatment of cancer. However, RNA modifications show differences in different cancers or cell lines. Although m6A modifications have been extensively studied in various other cancers, research on RNA methylation in ovarian cancer is still in the preliminary stage.
Epigenetic transcriptional regulation mainly involves DNA methylation, histone modifications and chromatin remodelling. Dysregulation of DNA methylation and histone deacetylation or methylation are the most intensively studied epigenetic changes in cancer and their dysregulation contributes to cancer progression. DNMTs are the enzymes responsible for DNA methylation, of which DNMT1, DNMT3A and DNMT3B have methyltransferase activity [Citation114]. Currently, two DNA methylation reagents based on nucleoside analogues are azacytidine (AZA, 5-azacytidine) and decitabine (DAC, 5-AZA-dC). These two DNA methylation reagents have been approved by the FDA for myelodysplastic syndromes (MDS) and chronic myelomonocytic leukaemial. Several other novel DNMT inhibitors (DNMTi) are also emerging in clinical therapy. Guadecitabine (SGI-110, Astex), hydrazine, and 5-fluoro-20 -deoxycytidine (FdCyd) are all being used in clinical trials [Citation115–117]. Some studies have reported that azacytidine can affect RNA metabolism by interfering with the synthesis, stability and function of rRNA, mRNA and tRNA. There are several clinical trials using DNMTi in the treatment of OC patients.
Histone lysine methyltransferases (KMTs) label histone lysines and create substrates for histone demethylases. KMTs are similar to DNMTs and catalyse methylation labelling with SUV39H1, DOT1L, and EZH2, a member of the multicomponent protein family. EPZ-5676, a DOT1L inhibitor, targets S-adenosine homocysteine analogues and has been used in clinical trials in AML. Two inhibitors of EZH2, EPZ-6438 and CPI-1205, have both been applied in clinical trials for different tumours. Lysine demethylases (KDM) contain the KDM1 subfamily of lysine-specific demethylases (LSD) and the KDM2–7 subfamily containing the Jumonji C (JmjC) structural domain [Citation118,Citation119]. Studies showing that monoamine oxidase (MAO) inhibitors inhibit LSD1 have been widely used to support clinical trials [Citation120]. The LSD1 inhibitor GSK2879552 inhibits small cell lung cancer (SCLC) and AML cell proliferation and predicts the sensitivity of SCLC-derived xenografts to small molecule inhibitors [Citation121]. In addition, the non-competitive inhibitor 2d (verlindamycin) and the competitive inhibitor oligomeric amine PG-11144 showed antitumour effects [Citation122–124]. Impressively, the combined pairing of these key enzyme inhibitors resulted in better anticancer effects. In conclusion, dysregulation of DNA methylation and posttranslational histone modification processes is an important target for therapeutic intervention in cancer.
RNA modifications interact inextricably with epigenetic modifications. DNA methylation directly controls the genetic expression of m6A regulators. In pancreatic duct epithelial cells, cigarette smoke condensate causes hypomethylation of the METTL3 promoter, induces METTL3 expression and promotes PC development and progression [Citation125]. In addition, RNA methylation and DNA methylation share the same methyl donors and epigenetic marks. RNA methylation selectively acts on transcripts encoding epigenetic modifiers of histone modifications to regulate histone modifications. YTHDC1 recruits the H3K9me2 demethylase KDM3B to m6A-associated chromatin regions to regulate local H3K9me2 levels and gene expression [Citation126]. Histone modifications mediate RNA m6A methylation at the posttranscriptional and transcriptional levels. Suppression of METTL14 is triggered by H3K2me4 loss induced by methyltransferase SETD3 knockdown or demethylase KDM36A overexpression, leading to overall m6A demethylation [Citation127].
Unlike DNA methylation and histone modifications, RNA methyltransferases and their inhibitors have not been studied thoroughly enough. Some inhibitors of FTO and several inhibitors of METTL3 and ALKBH5 have been found to exert regulatory effects on RNA methylation modifications. The lack of in vivo and in vitro cellular experiments and clinical trials in cancer implies the great potential of RNA methylation in cancer therapy. Perhaps, the development of effective RNA methylation inhibitors or demethylating agents may provide prospective therapeutic strategies for OC and recurrent drug resistance.
Author contributions
XZ designed this study. YX and XZ obtained the funding. SZ wrote the manuscript. SZ, MZ, JX and JZ draft the figures. XZ and XY revised the manuscript. All authors read, revised, and approved the final manuscript.
Acknowledgments
This research was supported by the Natural Science Foundation of China (Grant Nos. 82172838 and 81871343), the Natural Science Foundation of Jiangsu Province (Grant No. BK20201223) and the Science and Technology Innovation Fund of Zhenjiang city(Grant No.SH2022048).
Disclosure statement
No potential conflict of interest was reported by the authors.
Data availability statement
Not available.
Additional information
Funding
References
- Siegel RL, Miller KD, Jemal A. Cancer statistics, 2019. CA Cancer J Clin. 2019;69:7–34.
- Cao W, Chen H-D, Yu Y-W, et al. Changing profiles of cancer burden worldwide and in China: a secondary analysis of the global cancer statistics 2020. Chin Med J (Engl). 2021;134:783–791.
- Bitler BG, Watson ZL, Wheeler LJ, et al. PARP inhibitors: clinical utility and possibilities of overcoming resistance. Gynecol Oncol. 2017;147:695–704.
- Morand S, Devanaboyina M, Staats H, et al. Ovarian cancer immunotherapy and personalized medicine. Int J Mol Sci. 2021;22:6532.
- He L, Li H, Wu A, et al. Functions of N6-methyladenosine and its role in cancer. Mol Cancer. 2019;18:176.
- Xue C, Zhao Y, Li L. Advances in RNA cytosine-5 methylation: detection, regulatory mechanisms, biological functions and links to cancer. Biomark Res. 2020;8:43.
- Nombela P, Miguel-López B, Blanco S. The role of m6A, m5C and Ψ RNA modifications in cancer: novel therapeutic opportunities. Mol Cancer. 2021;20:18.
- Zhao L-Y, Song J, Liu Y, et al. Mapping the epigenetic modifications of DNA and RNA. Protein Cell. 2020;11:792–808.
- Xiang Y, Laurent B, Hsu C-H, et al. RNA m6A methylation regulates the ultraviolet-induced DNA damage response. Nature. 2017;543:573–576.
- Frye M, Harada BT, Behm M, et al. RNA modifications modulate gene expression during development. Science. 2018;361:1346–1349.
- Li X, Xiong X, Yi C. Epitranscriptome sequencing technologies: decoding RNA modifications. Nat Methods. 2016;14:23–31.
- Li X, Liang Q-X, Lin J-R, et al. Epitranscriptomic technologies and analyses. Sci China Life Sci. 2020;63:501–515.
- Barbieri I, Kouzarides T. Role of RNA modifications in cancer. Nat Rev Cancer. 2020;20:303–322.
- Sun T, Wu R, Ming L. The role of m6A RNA methylation in cancer. Biomed Pharmacother. 2019;112:108613.
- Xu Q-C, Tien Y-C, Shi Y-H, et al. METTL3 promotes intrahepatic cholangiocarcinoma progression by regulating IFIT2 expression in an m6A-YTHDF2-dependent manner. Oncogene. 2022;41:1622–1633.
- Yang X, Yang Y, Sun B-F, et al. 5-methylcytosine promotes mRNA export - NSUN2 as the methyltransferase and ALYREF as an m5C reader. Cell Res. 2017;27:606–625.
- He Y, Yu X, Li J, et al. Role of m5C-related regulatory genes in the diagnosis and prognosis of hepatocellular carcinoma. Am J Transl Res. 2020;12:912–922.
- L X, M Y. Expression and prognostic characteristics of m5 C regulators in low-grade glioma. J Cell Mol Med. 2021;25:1383–1393.
- Esteve-Puig R, Climent F, Piñeyro D, et al. Epigenetic loss of m1A RNA demethylase ALKBH3 in Hodgkin lymphoma targets collagen, conferring poor clinical outcome. Blood. 2021;137:994–999.
- Ying X, Liu B, Yuan Z, et al. METTL1-m7 G-EGFR/EFEMP1 axis promotes the bladder cancer development. Clin Transl Med. 2021;11:e675.
- Bokar JA, Shambaugh ME, Polayes D, et al. Purification and cDNA cloning of the AdoMet-binding subunit of the human mRNA (N6-adenosine)-methyltransferase. RNA. 1997;3:1233–1247.
- Bi X, Lv X, Liu D, et al. METTL3-mediated maturation of miR-126-5p promotes ovarian cancer progression via PTEN-mediated PI3K/Akt/mTOR pathway. Cancer Gene Ther. 2021;28:335–349.
- Ma Z, Li Q, Liu P, et al. METTL3 regulates m6A in endometrioid epithelial ovarian cancer independently of METTl14 and WTAP. Cell Biol Int. 2020;44:2524–2531.
- Schwartz S, Mumbach MR, Jovanovic M, et al. Perturbation of m6A writers reveals two distinct classes of mRNA methylation at internal and 5’ sites. Cell Rep. 2014;8:284–296.
- Li Y, Peng H, Jiang P, et al. Downregulation of methyltransferase-like 14 promotes ovarian cancer cell proliferation through stabilizing TROAP mRNA. Front Oncol. 2022;12:824258.
- Su R, Dong L, Li Y, et al. METTL16 exerts an m6A-independent function to facilitate translation and tumorigenesis. Nat Cell Biol. 2022;24:205–216.
- Agarwala SD, Blitzblau HG, Hochwagen A, et al. RNA methylation by the MIS complex regulates a cell fate decision in yeast. PLoS Genet. 2012;8:e1002732.
- Yu H-L, Ma X-D, Tong J-F, et al. WTAP is a prognostic marker of high-grade serous ovarian cancer and regulates the progression of ovarian cancer cells. Onco Targets Ther. 2019;12:6191–6201.
- Zhang C, Liu J, Guo H, et al. M6a RNA methylation regulators were associated with the malignancy and prognosis of ovarian cancer. Bioengineered. 2021;12:3159–3176.
- M(6)a RNA methylation promotes XIST-mediated transcriptional repression - PubMed. [update 2022 Nov 1]. Available from: https://pubmed.ncbi.nlm.nih.gov/27602518/
- Wang Q, Zhang Q, Li Q, et al. Clinicopathological and immunological characterization of RNA m6 a methylation regulators in ovarian cancer. Mol Genet Genomic Med. 2021;9:e1547.
- Wen J, Lv R, Ma H, et al. Zc3h13 regulates nuclear RNA m6A methylation and mouse embryonic stem cell self-renewal. Mol Cell. 2018;69:1028–1038.e6.
- Tan W, Liu S, Deng Z, et al. Gene signature of m6A-related targets to predict prognosis and immunotherapy response in ovarian cancer. J Cancer Res Clin Oncol. 2022; DOI:10.1007/s00432-022-04162-3
- J G, F Y, Z X, et al. N6-methyladenosine in nuclear RNA is a major substrate of the obesity-associated FTO. Nat Chem Biol. 2011;7:885–887.
- Zheng G, Dahl JA, Niu Y, et al. ALKBH5 is a mammalian RNA demethylase that impacts RNA metabolism and mouse fertility. Mol Cell. 2013;49:18–29.
- Cai Y, Wu G, Peng B, et al. Expression and molecular profiles of the AlkB family in ovarian serous carcinoma. Aging. 2021;13:9679–9692.
- Wang X, Zhao BS, Roundtree IA, et al. N(6)-methyladenosine modulates messenger RNA translation efficiency. Cell. 2015;161:1388–1399.
- Shi H, Wang X, Lu Z, et al. YTHDF3 facilitates translation and decay of N6-methyladenosine-modified RNA. Cell Res. 2017;27:315–328.
- Liu T, Wei Q, Jin J, et al. The m6A reader YTHDF1 promotes ovarian cancer progression via augmenting EIF3C translation. Nucleic Acids Res. 2020;48:3816–3831.
- Xu F, Li J, Ni M, et al. FBW7 suppresses ovarian cancer development by targeting the N6-methyladenosine binding protein YTHDF2. Mol Cancer. 2021;20:45.
- Xiao W, Adhikari S, Dahal U, et al. Nuclear m(6)A reader YTHDC1 regulates mRNA splicing. Mol Cell. 2016;61:507–519.
- Hsu PJ, Zhu Y, Ma H, et al. Ythdc2 is an N6-methyladenosine binding protein that regulates mammalian spermatogenesis. Cell Res. 2017;27:1115–1127.
- Huang H, Weng H, Sun W, et al. Recognition of RNA N6-methyladenosine by IGF2BP proteins enhances mRNA stability and translation. Nat Cell Biol. 2018;20:285–295.
- Müller S, Bley N, Glaß M, et al. IGF2BP1 enhances an aggressive tumor cell phenotype by impairing miRNA-directed downregulation of oncogenic factors. Nucleic Acids Res. 2018;46:6285–6303.
- Zhou KI, Shi H, Lyu R, et al. Regulation of co-transcriptional pre-mRNA splicing by m6A through the low-complexity protein hnRNPG. Mol Cell. 2019;76:70–81.e9.
- Liu N, Dai Q, Zheng G, et al. N(6)-methyladenosine-dependent RNA structural switches regulate RNA-protein interactions. Nature. 2015;518:560–564.
- Wu B, Su S, Patil DP, et al. Molecular basis for the specific and multivariant recognitions of RNA substrates by human hnRNP A2/B1. Nat Commun. 2018;9:420.
- Yang Y, Wei Q, Tang Y, et al. Loss of hnRNPA2B1 inhibits malignant capability and promotes apoptosis via down-regulating Lin28B expression in ovarian cancer. Cancer Lett. 2020;475:43–52.
- Motorin Y, Lyko F, Helm M. 5-methylcytosine in RNA: detection, enzymatic formation and biological functions. Nucleic Acids Res. 2010;38:1415–1430.
- Popis MC, Blanco S, Frye M. Posttranscriptional methylation of transfer and ribosomal RNA in stress response pathways, cell differentiation, and cancer. Curr Opin Oncol. 2016;28:65–71.
- Teng Y, Zuo X, Hou M, et al. A double-negative feedback interaction between MicroRNA-29b and DNMT3A/3B contributes to ovarian cancer progression. Cell Physiol Biochem. 2016;39:2341–2352.
- Basaki Y, Hosoi F, Oda Y, et al. Akt-dependent nuclear localization of Y-box-binding protein 1 in acquisition of malignant characteristics by human ovarian cancer cells. Oncogene. 2007;26:2736–2746.
- Xu J, Liu X, Chen Y, et al. RNA 5-methylcytosine regulators contribute to metabolism heterogeneity and predict prognosis in ovarian cancer. Front Cell Dev Biol. 2022;10:807786.
- Dominissini D, Nachtergaele S, Moshitch-Moshkovitz S, et al. The dynamic N(1)-methyladenosine methylome in eukaryotic messenger RNA. Nature. 2016;530:441–446.
- Zhou H, Rauch S, Dai Q, et al. Evolution of a reverse transcriptase to map N1-methyladenosine in human messenger RNA. Nat Methods. 2019;16:1281–1288.
- Chen Z, Qi M, Shen B, et al. Transfer RNA demethylase ALKBH3 promotes cancer progression via induction of tRNA-derived small RNAs. Nucleic Acids Res. 2019;47:2533–2545.
- Liu F, Clark W, Luo G, et al. ALKBH1-mediated tRNA demethylation regulates translation. Cell. 2016;167:816–828.e16.
- Woo H-H, Chambers SK. Human ALKBH3-induced m1A demethylation increases the CSF-1 mRNA stability in breast and ovarian cancer cells. Biochim Biophys Acta, Gene Regul Mech. 2019;1862:35–46.
- Seo KW, Kleiner RE. YTHDF2 recognition of N1-methyladenosine (m1A)-modified RNA is associated with transcript destabilization. ACS Chem Biol. 2020;15:132–139.
- Furuichi Y. Discovery of m(7)G-cap in eukaryotic mRnas. Proc Jpn Acad Ser B Phys Biol Sci. 2015;91:394–409.
- Furuichi Y, LaFiandra A, Shatkin AJ. 5’-Terminal structure and mRNA stability. Nature. 1977;266:235–239.
- Shimotohno K, Kodama Y, Hashimoto J, et al. Importance of 5’-terminal blocking structure to stabilize mRNA in eukaryotic protein synthesis. Proc Natl Acad Sci U S A. 1977;74:2734–2738.
- Alexandrov A, Martzen MR, Phizicky EM. Two proteins that form a complex are required for 7-methylguanosine modification of yeast tRNA. RNA. 2002;8:1253–1266.
- Trotman JB, Giltmier AJ, Mukherjee C, et al. RNA guanine-7 methyltransferase catalyzes the methylation of cytoplasmically recapped RNAs. Nucleic Acids Res. 2017;45:10726–10739.
- Luo Y, Yao Y, Wu P, et al. The potential role of N7-methylguanosine (m7G) in cancer. J Hematol Oncol. 2022;15:63.
- Liu K, Gao Y, Gan K, et al. Prognostic roles of N6-methyladenosine METTL3 in different cancers: a system review and meta-analysis. Cancer Control. 2021;28:1073274821997455.
- Han X, Liu J, Cheng G, et al. Gene signatures and prognostic values of m6A RNA methylation regulators in ovarian cancer. Cancer Control. 2020;27:1073274820960460.
- Wei Q, Yang D, Liu X, et al. Exploration of the role of m6 a RNA methylation regulators in malignant progression and clinical prognosis of ovarian cancer. Front Genet. 2021;12:650554.
- Fan L, Lin Y, Lei H, et al. A newly defined risk signature, consisting of three m6A RNA methylation regulators, predicts the prognosis of ovarian cancer. Aging. 2020;12:18453–18475.
- Wei YS, Yao DS, Li L, et al. Expression of METTL14 in epithelial ovarian cancer and the effect on cell proliferation, invasion and migration of A2780 and SKOV3 cells. Zhonghua Fu Chan Ke Za Zhi. 2022;57:46–56.
- Li Q, Ren C-C, Chen Y-N, et al. A risk score model incorporating three m6A RNA methylation regulators and a related network of miRnas-m6A regulators-m6A Target genes to predict the prognosis of patients with ovarian cancer. Front Cell Dev Biol. 2021;9:703969.
- Mercer TR, Dinger ME, Mattick JS. Long non-coding RNAs: insights into functions. Nat Rev Genet. 2009;10:155–159.
- Song Y, Qu H. Identification and validation of a seven m6A-related lncRnas signature predicting prognosis of ovarian cancer. BMC Cancer. 2022;22:633.
- Ni X, Chen C, Cui G, et al. Crosstalk of RNA adenosine modification-related subtypes, establishment of a prognostic model, and immune infiltration characteristics in ovarian cancer. Front Immunol. 2022;13:932876.
- Zhang L-Y, Li P-L, Wang T-Z, et al. Prognostic values of 5-hmC, 5-Mc and TET2 in epithelial ovarian cancer. Arch Gynecol Obstet. 2015;292:891–897.
- Wang L, Gao S. Identification of 5-methylcytosine-related signature for predicting prognosis in ovarian cancer. Biol Res. 2021;54:18.
- Oda Y, Ohishi Y, Basaki Y, et al. Prognostic implications of the nuclear localization of Y-box-binding protein-1 and CXCR4 expression in ovarian cancer: their correlation with activated Akt, LRP/MVP and P-glycoprotein expression. Cancer Sci. 2007;98:1020–1026.
- M L, Z Q, H X. Abnormal 5-methylcytosine lncRNA methylome is involved in human high-grade serous ovarian cancer. Am J Transl Res. 2021;13:13625–13639.
- Wang Q, Zhang Q, Huang Y, et al. M1a regulator TRMT10C predicts poorer survival and contributes to malignant behavior in gynecological cancers. DNA Cell Biol. 2020;39:1767–1778.
- Liu J, Chen C, Wang Y, et al. Comprehensive of N1-methyladenosine modifications patterns and immunological characteristics in ovarian cancer. Front Immunol. 2021;12:746647.
- Li L, Yang Y, Wang Z, et al. Prognostic role of METTL1 in glioma. Cancer Cell Int. 2021;21:633.
- Jiang Y, Wan Y, Gong M, et al. RNA demethylase ALKBH5 promotes ovarian carcinogenesis in a simulated tumour microenvironment through stimulating NF-κB pathway. J Cell Mol Med. 2020;24:6137–6148.
- Meng L, Zhang Q, Huang X. Comprehensive analysis of 5-methylcytosine profiles of messenger RNA in human high-grade serous ovarian cancer by MeRIP sequencing. Cancer Manag Res. 2021;13:6005–6018.
- Cui S. METTL3-mediated m6A modification of lnc RNA RHPN1-AS1 enhances cisplatin resistance in ovarian cancer by activating PI3K/AKT pathway. J Clin Lab Anal. 2022;36:e24761.
- Liang S, Guan H, Lin X, et al. METTL3 serves an oncogenic role in human ovarian cancer cells partially via the AKT signaling pathway. Oncol Lett. 2020;19:3197–3204.
- Lyu Y, Zhang Y, Wang Y, et al. HIF-1α regulated WTAP overexpression promoting the Warburg effect of ovarian cancer by m6A-Dependent manner. J Immunol Res. 2022;2022:6130806.
- Wang Y, Chen Z. Long noncoding RNA UBA6-AS1 inhibits the malignancy of ovarian cancer cells via suppressing the decay of UBA6 mRNA. Bioengineered. 2022;13:178–189.
- Zhu H, Gan X, Jiang X, et al. ALKBH5 inhibited autophagy of epithelial ovarian cancer through miR-7 and BCL-2. J Exp Clin Cancer Res. 2019;38:163.
- Li J, Wu L, Pei M, et al. YTHDF2, a protein repressed by miR-145, regulates proliferation, apoptosis, and migration in ovarian cancer cells. J Ovarian Res. 2020;13:111.
- Wang L, Zhang J, Su Y, et al. Distinct roles of m5C RNA methyltransferase NSUN2 in major gynecologic cancers. Front Oncol. 2022;12:786266.
- Gu J, Bi F. Significance of N6-methyladenosine RNA methylation regulators in immune infiltrates of ovarian cancer. Front Genet. 2021;12:671179.
- Shen Z, Gu L, Liu Y, et al. PLAA suppresses ovarian cancer metastasis via METTL3-mediated m6A modification of TRPC3 mRNA. Oncogene. 2022;41:4145–4158.
- Huang H, Wang Y, Kandpal M, et al. FTO-Dependent N 6-methyladenosine modifications inhibit ovarian cancer stem cell self-renewal by blocking cAMP signaling. Cancer Res. 2020;80:3200–3214.
- Hao L, Wang J-M, Liu B-Q, et al. M6A-YTHDF1-mediated TRIM29 upregulation facilitates the stem cell-like phenotype of cisplatin-resistant ovarian cancer cells. Biochim Biophys Acta, Mol Cell Res. 2021;1868:118878.
- Chen B, Ye F, Yu L, et al. Development of cell-active N6-methyladenosine RNA demethylase FTO inhibitor. J Am Chem Soc. 2012;134:17963–17971.
- Huang Y, Yan J, Li Q, et al. Meclofenamic acid selectively inhibits FTO demethylation of m6A over ALKBH5. Nucleic Acids Res. 2015;43:373–384.
- Huang Y, Su R, Sheng Y, et al. Small-molecule targeting of oncogenic FTO demethylase in acute myeloid leukemia. Cancer Cell. 2019;35:677–691.e10.
- Xie G, Wu X-N, Ling Y, et al. A novel inhibitor of N 6-methyladenosine demethylase FTO induces mRNA methylation and shows anti-cancer activities. Acta Pharm Sin B. 2022;12:853–866.
- Sugihara H, Miyaji K, Yamanouchi K, et al. Progranulin deficiency leads to prolonged persistence of macrophages, accompanied with myofiber hypertrophy in regenerating muscle. J Vet Med Sci. 2018;80:346–353.
- Zheng G, Cox T, Tribbey L, et al. Synthesis of a FTO inhibitor with anticonvulsant activity. ACS Chem Neurosci. 2014;5:658–665.
- Chen J, Wei X, Wang X, et al. TBK1-METTL3 axis facilitates antiviral immunity. Cell Rep. 2022;38:110373.
- Lee J-H, Choi N, Kim S, et al. Eltrombopag as an allosteric inhibitor of the METTL3-14 complex affecting the m6A methylation of RNA in acute myeloid leukemia cells. Pharmaceuticals (Basel). 2022;15:440.
- Li N, Kang Y, Wang L, et al. ALKBH5 regulates anti-PD-1 therapy response by modulating lactate and suppressive immune cell accumulation in tumor microenvironment. Proc Natl Acad Sci U S A. 2020;117:20159–20170.
- Wallis N, Oberman F, Shurrush K, et al. Small molecule inhibitor of Igf2bp1 represses Kras and a pro-oncogenic phenotype in cancer cells. RNA Biol. 2022;19:26–43.
- Nie S, Zhang L, Liu J, et al. ALKBH5-HOXA10 loop-mediated JAK2 m6A demethylation and cisplatin resistance in epithelial ovarian cancer. J Exp Clin Cancer Res. 2021;40:284.
- Fukumoto T, Zhu H, Nacarelli T, et al. N6-methylation of adenosine of FZD10 mRNA contributes to PARP inhibitor resistance. Cancer Res. 2019;79:2812–2820.
- Zhang Y, Qiu J-G, Jia X-Y, et al. METTL3-mediated N6-methyladenosine modification and HDAC5/YY1 promote IFFO1 downregulation in tumor development and chemo-resistance. Cancer Lett. 2023;553:215971.
- Gao W, Chen L, Lin L, et al. SIAH1 reverses chemoresistance in epithelial ovarian cancer via ubiquitination of YBX-1. Oncogenesis. 2022;11:13.
- Xiang Y, Ma N, Wang D, et al. MiR-152 and miR-185 co-contribute to ovarian cancer cells cisplatin sensitivity by targeting DNMT1 directly: a novel epigenetic therapy independent of decitabine. Oncogene. 2014;33:378–386.
- Han X, Liu D, Zhou Y, et al. The negative feedback between miR-143 and DNMT3A regulates cisplatin resistance in ovarian cancer. Cell Biol Int. 2021;45:227–237.
- Chen B, Jiang W, Huang Y, et al. N7-methylguanosine tRNA modification promotes tumorigenesis and chemoresistance through WNT/β-catenin pathway in nasopharyngeal carcinoma. Oncogene. 2022;41:2239–2253.
- Okamoto M, Fujiwara M, Hori M, et al. tRNA modifying enzymes, NSUN2 and METTL1, determine sensitivity to 5-fluorouracil in HeLa cells. PLoS Genet. 2014;10:e1004639.
- Xia P, Zhang H, Xu K, et al. MYC-targeted WDR4 promotes proliferation, metastasis, and sorafenib resistance by inducing CCNB1 translation in hepatocellular carcinoma. Cell Death Dis. 2021;12:691.
- Denis H, Ndlovu MN, Fuks F. Regulation of mammalian DNA methyltransferases: a route to new mechanisms. EMBO Rep. 2011;12:647–656.
- Issa JP, Baylin SB, Herman JG. DNA methylation changes in hematologic malignancies: biologic and clinical implications. Leukemia. 1997;11(Suppl 1):S7–11.
- Candelaria M, Gallardo-Rincón D, Arce C, et al. A phase II study of epigenetic therapy with hydralazine and magnesium valproate to overcome chemotherapy resistance in refractory solid tumors. Ann Oncol. 2007;18:1529–1538.
- Newman EM, Morgan RJ, Kummar S, et al. A phase I, pharmacokinetic, and pharmacodynamic evaluation of the DNA methyltransferase inhibitor 5-fluoro-2’-deoxycytidine, administered with tetrahydrouridine. Cancer Chemother Pharmacol. 2015;75:537–546.
- Dimitrova E, Turberfield AH, Klose RJ. Histone demethylases in chromatin biology and beyond. EMBO Rep. 2015;16:1620–1639.
- Klose RJ, Kallin EM, Zhang Y. JmjC-domain-containing proteins and histone demethylation. Nat Rev Genet. 2006;7:715–727.
- Schmidt DMZ, McCafferty DG. Trans-2-Phenylcyclopropylamine is a mechanism-based inactivator of the histone demethylase LSD1. Biochemistry. 2007;46:4408–4416.
- Mohammad HP, Smitheman KN, Kamat CD, et al. A DNA hypomethylation signature predicts antitumor activity of LSD1 inhibitors in SCLC. Cancer Cell. 2015;28:57–69.
- Huang Y, Stewart TM, Wu Y, et al. Novel oligoamine analogues inhibit lysine-specific demethylase 1 and induce reexpression of epigenetically silenced genes. Clin Cancer Res. 2009;15:7217–7228.
- Murray-Stewart T, Woster PM, Casero RA. The re-expression of the epigenetically silenced e-cadherin gene by a polyamine analogue lysine-specific demethylase-1 (LSD1) inhibitor in human acute myeloid leukemia cell lines. Amino Acids. 2014;46:585–594.
- Zhu Q, Huang Y, Marton LJ, et al. Polyamine analogs modulate gene expression by inhibiting lysine-specific demethylase 1 (LSD1) and altering chromatin structure in human breast cancer cells. Amino Acids. 2012;42:887–898.
- Zhang J, Bai R, Li M, et al. Excessive miR-25-3p maturation via N6-methyladenosine stimulated by cigarette smoke promotes pancreatic cancer progression. Nat Commun. 2019;10:1858.
- Li Y, Xia L, Tan K, et al. N6-methyladenosine co-transcriptionally directs the demethylation of histone H3K9me2. Nat Genet. 2020;52:870–877.
- Huang H, Weng H, Zhou K, et al. Histone H3 trimethylation at lysine 36 guides m6A RNA modification co-transcriptionally. Nature. 2019;567:414–419.