ABSTRACT
Human bone marrow mesenchymal stem cells (HBMSCs) can promote new bone formation. Previous studies have proven the ability of long non-coding RNAs (lncRNAs) to modulate the osteogenic differentiation of mesenchymal stem cells. However, the molecular mechanism modulated by lncRNAs in affecting the osteogenic differentiation of HBMSCs remains largely unknown. Thus, this study aims to reveal the role of lncRNA ubiquitin-specific peptidase 2 antisense RNA 1 (USP2-AS1) in regulating the osteogenic differentiation of HBMSCs and investigate its regulatory mechanism. Through bioinformatics analysis and RT-qPCR, we confirmed that USP2-AS1 expression was increased in HBMSCs after culturing in osteogenic differentiation medium (OM-HBMSCs). Moreover, we uncovered that knockdown of USP2-AS1 inhibited the osteogenic differentiation of HBMSCs. Further exploration indicated that USP2-AS1 positively regulated the expression of its nearby gene USP2. Mechanistically, USP2-AS1 recruited lysine demethylase 3A (KDM3A) to stabilize ETS proto-oncogene 1 (ETS1), transcription factor that transcriptionally activated USP2. Additionally, USP2-induced Wnt/β-catenin signalling pathway activation via deubiquitination of β-catenin protein. In summary, our study proved that lncRNA USP2-AS1 facilitates the osteogenic differentiation of HBMSCs by targeting KDM3A/ETS1/USP2 axis to activate the Wnt/β-catenin signalling pathway.
Introduction
Human bone marrow mesenchymal stem cells (HBMSCs) play an important role in new bone formation. According to previous studies, the imbalance of osteoblast and adipocyte differentiation of HBMSCs can lead to osteoporosis [Citation1,Citation2]. Nonetheless, the molecular regulatory mechanisms of osteogenic differentiation remain unclear. Hence, this study aims at investigating the novel mechanism that is essential to the osteogenic differentiation of HBMSCs.
Long non-coding RNAs (lncRNAs) are a category of RNAs with limited protein-coding ability, which have over 200 nucleotides in length [Citation3]. According to previous researches, lncRNAs serve as key regulators during osteogenic differentiation [Citation4]. For instance, lncRNA MALAT1 targets the MAPK pathway to hamper osteogenic differentiation of mesenchymal stem cell in osteoporosis rats [Citation5]. LINC00314 activates the Wnt/β-catenin signalling pathway via targeting miR-129-5p/GRM5, thus facilitating osteogenic differentiation of stem cells derived from adipose [Citation6]. Additionally, FAM83H-AS1 serves as a crucial participant in regulating SpA-inhibited osteogenic differentiation of HBMSCs [Citation7]. Previous study has unclosed that lncRNA ubiquitin-specific peptidase 2 antisense RNA 1 (USP2-AS1) could exacerbate cell malignant behaviours in multiple cancers, including colon cancer and ovarian cancer [Citation8,Citation9]. However, its functions in the osteogenic differentiation of HBMSCs remain elusive.
The former study has revealed that ubiquitin-specific protease 53 can regulate the osteogenic differentiation of mesenchymal stem cells [Citation10]. Ubiquitin-specific peptidase 2 (USP2) belongs to deubiquitinating enzymes. To date, no study has revealed its role in the osteogenic differentiation of mesenchymal stem cells. In addition, some published studies have demonstrated that lncRNAs can exert their functions through regulating the adjoining genes [Citation11–13]. For instance, MAK5-AS1 cis-regulates the nearby gene MK5 and functions as a let-7f-1-3p sponge in colorectal cancer [Citation14]. TRPM2-AS contributes to enhanced mRNA stability of its nearby gene TRPM2 via interaction with TAF15 [Citation13]. However, whether lncRNA USP2-AS1 influences the osteogenic differentiation of HBMSCs by regulating USP2 expression remain to be further elucidated.
To sum up, the current study is aimed at unveiling the role of USP2-AS1 in modulating the osteogenic differentiation of HBMSCs and its correlation with its nearby gene USP2.
Materials and methods
Cell lines and culture
HBMSCs were commercially attained from ATCC (Manassas, VA, USA). HBMSCs cultured in Dulbecco’s modified Eagle’s proliferation medium (PM) added with 10% FBS were defined as PM-HBMSCs, and these cells were maintained at 37°C with 5% CO2. As for osteogenic differentiation, HBMSCs were kept in osteogenic differentiation medium (OM) supplemented with 0.05 mmol/L ascorbic acid, 100 mmol/L dexamethasone and 10 mmol/L β-glycerophosphate, and this kind of cells was called OM-HBMSCs.
Plasmid transfection
Small interfering RNAs (siRNAs) specifically targeting USP2-AS1 (si1/2/3-USP2-AS1), EST1 (si1/2/3-ETS1), USP2 (si1/2/3-USP2), KDM3A (si1/2/3-KDM3A), RUNX1 (si1/2/3-RUNX1), CTCF (si1/2/3-CTCF), E2F4 (si1/2/3-E2F4) and MYC (si1/2/3-MYC) were synthesized by GenePharma (Shanghai, China). The whole sequence of USP2, USP2-AS1 or ETS1 was separately cloned into pcDNA3.1 vector to generate their respective overexpression vector (pcDNA3.1-USP2, pcDNA3.1-USP2-AS1 and pcDNA3.1-ETS1). All cells were placed in 24-well culture plates (2 × 10 [Citation5]/well) for transfection of the above-mentioned plasmids (0.8 μg/50 μl) for 48 h with utilization of Lipofectamine 3000 (2.0 μl/50 μl; L3000075, Invitrogen, Carlsbad, CA, USA).
Alkaline phosphatase (ALP) staining and activity
BCIP/NBT Alkaline Phosphatase Color Development Kit (C3206, Beyotime, Shanghai, China) was used for ALP staining. ALP activity of OM-HBMSC (after 14-day osteogenic differentiation) was measured by quantifying the absorbance at 405 nm using a spectrophotometer (840–210600, Thermo Fisher Scientific, Rockford, IL, USA).
Alizarin red S (ARS) staining and quantification
For ARS staining and quantification, OM-HBMSCs (after 14-day osteogenic differentiation) in each group were washed twice with pre-cooled PBS and fixed in 4% paraformaldehyde for 10 min, followed by 10 min of incubation with 30 mM ARS (pH = 4.2, Sigma, St. Louis, MO, USA) at room temperature. After washing, results were obtained using a microscope (DMI1, Leica, Wetzlar, Germany).
RNA extraction and reverse transcription quantitative polymerase chain reaction (RT-qPCR)
Total RNAs were isolated from cultured cells (2 × 10) [Citation5] utilizing TRIzol reagent. A total of 20 μl RNA was applied to carry out reverse transcription reaction. An equal amount of 1 μl complementary DNA (cDNA) was synthesized for gene expression detection. SYBR Green PCR Master Mix (4309155, Applied Biosystems, Foster city, CA, USA) was utilized to implement qPCR. Ultimately, 2−ΔΔCt approach was adopted to calculate relative gene expression. β-actin and U6 were used as internal controls for normalization. PrimeScript RT reagent Kit with gDNA Eraser (RR047A, Takara, Tokyo, Japan) was adopted to eliminate genomic DNA contamination. The sequence containing the target fragment of quantitative PCR was amplified by PCR, and the amplified products were, respectively, inserted into pGEM-T Easy vector to construct the TA3 recombinant vector. The amplification efficiency of primer pairs was analysed by fluorescence quantitative PCR method using TA3 vector serving as template.
RNA pull-down assay
Biotinylated KDM3A (1 μg) synthesized by Ribobio (Guangzhou, China) was treated with structure buffer for secondary structures formation. The structure buffer consisted of 200 mM NaCl, 20 mM Tris, pH 7.5, 2.5 mM MgCl2, 60 U/mL Superase-In (Ambion), 0.05% Igepal, 1 mM DTT, protease inhibitors (Roche). The secondary structures were then heated at 95°C for 2 min. Subsequently, the samples were ice bathed for 3 min. After that, biotin-labelled and denatured RNAs were mixed with M-280 streptavidin magnetic beads (15 μL) in order to obtain the bead-coated RNAs. Cell lysates were obtained after application of lysis buffer containing 0.1% SDS, 1% Trition X-100, 20 mM Tris-HCl pH8.0, 2 mM EDTA, 150 mM NaCl. Then, cell lysate and the bead-coated RNAs were co-cultured for 1 h at room temperature. Subsequent to centrifugation, the proteins were eluted and then subjected to western blot analysis.
Glutathione-S-transferase (GST) pull-down assay
GST-conjugated USP2 protein was expressed in OM-HBMSCs and was purified in accordance with protocols. Glutathione-Sepharose beads commercially attained from GE Healthcare (Waukesha, WI, USA) were coupled with either GST or the GST-USP2 purified protein, followed by incubation with the solubilized membrane proteins at 4°C for 1 h. According to the manufacturer’s instructions, the membrane proteins of OM-HBMSCs were extracted utilizing a ProteoExtract Native Membrane Protein Extraction kit (Calbiochem, San Diego, CA, USA). Subsequent to the rinse of the beads three times utilizing washing buffer (50 mM HEPES-KOH, 150 mM NaCl, 1 mM MgCl2, 0.2% Triton-X-100, pH 7.2), the proteins were separated from the beads with 10% SDS-PAGE and were then visualized utilizing Coomassie Brilliant Blue R-250 staining. The differentially apparent proteins were isolated from the gel, followed by identification with mass spectrometry.
Western blot
Total protein obtained from indicated HBMSCs was extracted using RIPA buffer. Subsequent to separation on SDS-PAGE, proteins were transferred to polyvinylidene fluoride membranes and then blocked in 5% skim milk. The membranes were subsequently incubated with primary antibodies at 4°C overnight, followed by incubation with secondary antibodies (ab131368, Abcam, Cambridge, MA, UK) for 1 h. After washing with Tris-buffered saline with Tween, the proteins were measured via enhanced chemiluminescence detection system. Primary antibodies used in this experiment were as follows: Anti-ETS1 (ab220361, Abcam), Anti-H3K9me2 (720092, Invitrogen), Anti-β-actin (K200058M, Solarbio, Beijing, China), Anti-KDM3A (ab243641, Abcam), Anti-β-catenin (ab32572, Abcam), Anti-RUNX2 (ab236639, Abcam), Anti-ALP (ab229126, Abcam), Anti-OSX (ab209484, Abcam), Anti-USP2 (ab66556, Abcam), Anti-GAPDH (ab8245, Abcam) and Anti-OCN (ab93876, Abcam).
Luciferase reporter assay
To determine the regulatory pattern of USP2-AS1 on USP2 expression, sequence of USP2 promoter or USP2 3’UTR was, respectively, sub-cloned into pGL3 or pmirGLO vectors, which was co-transfected with pcDNA3.1 or pcDNA3.1-USP2-AS1 into OM-HBMSCs. After PCR amplification, wild-type ETS1-targeted sequences within USP2 promoter and the corresponding mutated sequences (site 1 Mut: GAAGGA; site 2 Mut: AAAGGA; site 3 Mut: GAAGGA; site 4 Mut: AAAGGA; site 5 Mut: AAAGGA; site 6 Mut: GAAGGA) synthesized with Muta-directTM were inserted into the downstream of firefly gene in pGL3 luciferase reporter vector to construct pGL3-USP2 promoter WT, pGL3-USP2 promoter site 1 Mut, pGL3-USP2 promoter site 2 Mut, pGL3-USP2 promoter site 3 Mut, pGL3-USP2 promoter site 4 Mut, pGL3-USP2 promoter site 5 Mut and pGL3-USP2 promoter site 6 Mut. Next, the above constructs were co-transfected with pcDNA3.1-ETS1 or pcDNA3.1 into OM-HBMSCs via Lipofectamine 2000 kit (11668019, Invitrogen). All luciferase activities were measured utilizing Dual-Luciferase Reporter Assay System (Promega) and normalized to Renilla luciferase (internal control).
RNA immunoprecipitation (RIP) assay
HBMSCs were firstly lysed by RIP lysis buffer comprising RNase inhibitor and protease inhibitor cocktail. Lysates were cultured with indicated antibodies including Anti-Ago2, Anti-ETS1, Anti-KDM3A and immunoglobulin G (IgG) antibody overnight at 4°C with gentle rotation, followed by incubation with protein A/G beads for 1 h. RNA was eluted from the beads using 400 μl elution buffer. And then, the eluted RNA was precipitated in ethanol, followed by dissolution utilizing RNase-free water. Finally, RT-qPCR was carried out to analyse RNA enrichment.
Chromatin immunoprecipitation (ChIP) assay
ChIP assay was implemented with HBMSCs and the cells were firstly cross-linked in 4% paraformaldehyde. Subsequently, the samples were sonicated into chromatin fragments (200–1000 bp) which were then subjected to overnight incubation with the antibodies against ETS1, H3K9me2 or negative control IgG at 4°C. After that, the fragments were subjected to incubation with protein A/G magnetic beads for 2 h. DNA samples were extracted utilizing chloroform, phenol and isoamyl alcohol as instructed by the supplier’s protocols. RT-qPCR analysis was applied to detect the immunoprecipitated DNAs.
Co-immunoprecipitation (co-IP) assay
The prepared cell lysates were obtained from the treated HBMSCs in IP lysis buffer, followed by overnight culture with indicated antibodies and control IgG antibody at 4°C. After being mixed with magnetic beads, samples were washed by IP lysis buffer, followed by quantification utilizing western blot.
Fluorescence in situ hybridization (FISH) assay
4% Formaldehyde was firstly applied to fix HBMSCs which were later rinsed by PBS. Subsequently, the fixed samples were placed on the slides and dried in the oven at 46°C for 10 min. After being immersed in 50%, 80% and 95% ethanol solution for 3 min, respectively, the samples were dried in air. Subsequent to dehydration, cells were treated with FISH probes specifically designed for USP2-AS1. Three hours subsequent to hybridization, cell nuclei were dyed utilizing DAPI solution. Lastly, a fluorescence microscope was employed to observe the dyed cells.
Immunofluorescence (IF) assay
HBMSCs were placed on coverslips which were put in the bottom of all pores in 12 culture dishes. Forty-eight hours after transfection, cells were fixed with 4% paraformaldehyde and permeabilized with 1% Triton X-100 in 50 mmol/L Tris-HCl (pH 7.4) comprising 150 mmol/L NaCl and 1 mg/mL BSA. After 30-min incubation in blocking solution (#12411, Cell signalling technology, Boston, Mass, USA), non-specific binding was hindered. Afterwards, samples were incubated with the primary antibodies against KDM3A (ab243641, Abcam) overnight. Subsequently, samples were washed in PBS for three times, followed by incubation with secondary antibody (ab150116, Abcam) for 1 h. Cells were incubated in prepared block solution for 30 min at room temperature, followed by being washed twice in PBS. Later, DAPI was added for nucleus counterstaining. 6.5% BSA (10% FBS and 90% PBS) was obtained to mount coverslips on slides for 60 min and an inverted laser scanning confocal microscope (Axio-Imager LSM-800, Zeiss, Oberkochen, Germany) was utilized for capturing all images.
Statistical analysis
All relevant data in experiments were analysed using SPSS software. Each assay was independently repeated thrice. All data from assays encompassing three biological replications were displayed as the mean ± standard deviation (SD). Data analyses between groups were achieved utilizing student’s t-test (comparison for two groups) and one-way/two-way ANOVA (comparison for more than two groups). The p-value less than 0.05 was set as the threshold for statistical significance.
Results
USP2-AS1 promotes the osteogenic differentiation of HBMSCs
At first, we conducted flow cytometry assays to detect surface markers, including CD14, CD45, HLA-DR, CD29, CD44 and CD105 by using specific antibodies (CD14-PE, CD45-FITC, CD29-PE, HLA-DR-PE, CD44-FITC and CD105-FITC). As shown in Fig. S1A-F, we found that HBMSCs were negative for CD14, CD45 and HLA-DR but positive for CD29, CD44 and CD105. Therefore, we confirmed the purity of HBMSCs used in this study. Subsequently, we analysed GEO database (GSE113359) (https://www.ncbi.nlm.nih.gov/geo/query/acc.cgi?acc=GSE113359) to search for lncRNAs differentially expressed in HBMSCs at day 0 and day 10 of osteogenic differentiation stages by setting the conditions as adj.P.Val <0.005 and logFC > 2 (). Among the lncRNAs with highest logFC values, LINC00707 had already been proven to regulate the osteogenic differentiation of HBMSCs [Citation15,Citation16]. Therefore, other five lncRNAs, including USP2-AS1, HMMR-AS1, LINC02336, LINC01543 and NR2F1-AS1, were chosen for further analysis. According to RT-qPCR data, only USP2-AS1 was significantly up-regulated in OM-HBMSCs compared to PM-HBMSCs (). Thereafter, we chose USP2-AS1 to be our research object. Moreover, we applied RT-qPCR to detect the expression level of USP2-AS1 in OM-HBMSCs on day 0, 5, 10 and 15, and we found that the expression level of USP2-AS1 increased with time (). Subsequently, we knocked down USP2-AS1 in OM-HBMSCs with specific siRNAs and chose si1/2-USP2-AS1 for further use due to their higher knockdown efficiency (Fig. S2A). Afterwards, results of ALP staining assay showed that USP2-AS1 depletion led to the significant decrease in the ALP activity of OM-HBMSCs (). Results of ARS staining evidenced that the absorbance value of OM-HBMSCs decreased after USP2-AS1 silence, indicating that USP2-AS1 silence inhibited the formation of mineralized bone matrix in OM-HBMSCs (). Subsequently, we overexpressed USP2-AS1 by transfection of pcDNA3.1-USP2-AS1 (Fig. S2B), and found that pcDNA3.1-USP2-AS1 transfection could recover the inhibited ALP activity and decreased absorbance value induced by USP2-AS1 silence (Fig. S2C-D). Through RT-qPCR and western blot analyses, we found that expressions of osteogenesis-associated markers, including RUNX2, ALP, OSX and OCN were gradually increased after osteogenic induction, while USP2-AS1 depletion evidently reduced the expressions of the indicated markers (Fig. S2E-F & ). In addition, we performed subcellular fractionation and FISH assays and confirmed the distribution of USP2-AS1 in both cytoplasmic and nuclear parts of OM-HBMSCs (), which was in accordance with the predicted results on lncLocator (www.csbio.sjtu.edu.cn/bioinf/lncLocator) (Fig. S2G). The above findings indicated that USP2-AS1 promotes the osteogenic differentiation of HBMSCs.
Figure 1. USP2-AS1 promotes the osteogenic differentiation of HBMSCs.(A) Data from GEO database (GSE113359) showed differentially expressed lncRnas in HBMSCs on day 0 and day 10 during osteogenic differentiation. (B) RT-qPCR detected the expression levels of the selected lncRnas in PM-HBMSCs and OM-HBMSCs. (C) RT-qPCR detected the expression of USP2-AS1 in OM-HBMSCs on day 0, 5, 10 and 15 of osteogenic differentiation. (D) ALP staining assay examined the influence of USP2-AS1 silencing on ALP activity of OM-HBMSCs. (E) ARS staining assay verified the effect of USP2-AS1 knockdown on the formation of mineralized bone matrix in OM-HBMSCs. (F-G) RT-qPCR and western blot analysed the expression levels of osteogenesis-associated markers in USP2-AS1-depleted OM-HBMSCs. (H) subcellular fractionation and FISH assays determined the distribution of USP2-AS1 in OM-HBMSCs. **P < 0.01.
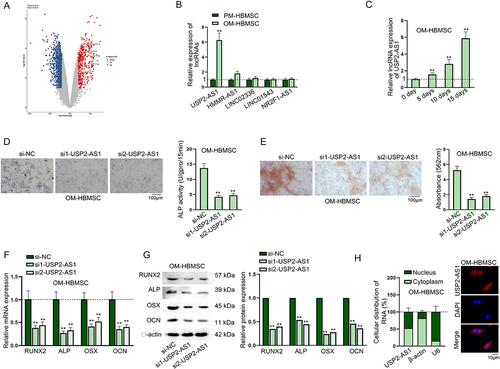
USP2-AS1 facilitates the osteogenic differentiation of HBMSCs by up-regulating USP2
As some previous reports have disclosed that lncRNAs can exert their functions via regulating the adjoining genes [Citation11–13], we speculated that USP2-AS1 could regulate the expression of its nearby gene USP2. We uncovered that USP2-AS1 and USP2 were co-localized both in cell nuclei and cytoplasm (, upper panel). Moreover, the genomic location of USP2-AS1 and USP2 obtained from UCSC (http://genome.ucsc.edu/) was shown (, lower panel). Then, we analysed the correlation between the expression levels of USP2-AS1 and USP2. As shown in , there existed a positive correlation between the expression levels of USP2-AS1 and USP2. We conducted RT-qPCR and western blot at day 14 after osteogenic induction and confirmed USP2-AS1 silencing could lead to decrease in USP2 expression in OM-HBMSCs (). Afterwards, we detected the overexpression efficiency of pcDNA3.1-USP2 in OM-HBMSCs (Fig. S3A). Subsequently, results of ALP staining indicated that USP2 up-regulation could rescue the ALP activity of OM-HBMSCs impaired by USP2-AS1 silencing (). Thereafter, ARS staining assay verified that co-transfection of pcDNA3.1-USP2 could completely countervail the inhibitory impacts of USP2-AS1 silence on absorbance value of OM-HBMSCs (). To further verify our conclusion, we detected the expression levels of RUNX2, ALP, OSX and OCN. As a result, co-transfection of pcDNA3.1-USP2 rescued the decreased levels of osteogenesis-associated markers caused by USP2-AS1 silencing (). Therefore, we confirmed that USP2-AS1 could promote the osteogenic differentiation of HBMSCs via enhancing USP2 expression.
Figure 2. USP2-AS1 induces the osteogenic differentiation of HBMSCs by up-regulating USP2.(A) FISH assay detected the co-localization of USP2-AS1 and USP2 in OM-HBMSCs. The genomic location of USP2-AS1 and USP2 obtained from UCSC was shown. (B) Based on RT-qPCR results of USP2-AS1 and USP2 expressions on day 0, 5, 10 and 15 after osteogenic differentiation, correlation analysis was completed. (C) RT-qPCR and western blot confirmed the effect of USP2-AS1 silencing on USP2 expression in OM-HBMSCs 14 days after osteogenic induction. (D) ALP staining examined the influence of USP2-AS1 depletion and USP2 overexpression on the ALP activity of OM-HBMSCs 14 days after cultivation. (E) ARS staining assay explored the role of USP2-AS1 and USP2 in the formation of mineralized bone matrix in OM-HBMSCs 14 days after cultivation. (F-G) RT-qPCR and western blot measured the expression levels of RUNX2, ALP, OSX and OCN in OM-HBMSCs with different transfection. (H) luciferase reporter assays verified the influence of USP2-AS1 overexpression on the activity of USP2 promoter. **P < 0.01.
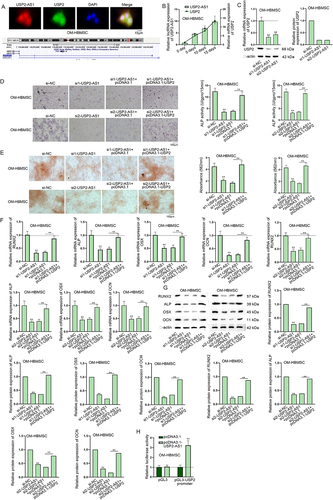
Many previous reports have unveiled that lncRNAs can interact with certain transcription factors in nucleus to affect the transcription of the downstream genes [Citation17–20]. In addition, lncRNAs can interact with RNA-binding proteins (RBPs) in cytoplasm to regulate their expression levels post-transcriptionally [Citation21,Citation22]. As we had confirmed the co-localization of USP2-AS1 and USP2 in the cytoplasm and nucleus of HBMSCs, we designed luciferase reporter assay to determine the underlying mechanism. From the results, we demonstrated that overexpression of USP2-AS1 could enhance the luciferase activity of pGL3-USP2 promoter rather than pmirGLO-USP2 3’UTR (Fig. S3B & ). To conclude, USP2-AS1 promotes the osteogenic differentiation of HBMSCs by positively regulating USP2.
ETS1 transcriptionally activates USP2
We continued to investigate the transcriptional regulation of USP2-AS1 on USP2. Firstly, we applied hTFtarget (http://bioinfo.life.hust.edu.cn/hTFtarget#!/) to predict putative transcription factors of USP2 in bone marrow tissues. Since the role of ETS1 [Citation23;] RUNX1 [Citation24;] CTCF [Citation25;] E2F4 [Citation26] and MYC [Citation27,Citation28] in osteogenic differentiation have been reported, we selected them as the research candidates (). Subsequently, we knocked down these transcription factors and detected the interference efficiency (Fig. S3C-G). Afterwards, we found that the expression of USP2 only significantly decreased upon ETS1 silencing (). Western blot analysis consistently indicated ETS1 knockdown could decrease USP2 protein level (). Therefore, we further conducted mechanism experiment to prove the interaction between ETS1 and USP2. Based on results of ChIP and DNA pull-down assays, we ascertained the binding between ETS1 and USP2 promoter in OM-HBMSCs (). Meanwhile, we searched on JASPAR (http://jaspar.genereg.net/) for putative binding sites of ETS1 on USP2 promoter (screening condition: score > 7) (). We overexpressed pcDNA3.1-ETS1 and confirmed the overexpression efficiency through RT-qPCR (Fig. S3H). Luciferase reporter assay revealed that the luciferase activity only kept unchanged in the group of pGL3-USP2 promoter site 1 Mut, reflecting that ETS1 could bind to USP2 promoter at site 1 (). To sum up, ETS1 transcriptionally activates USP2.
Figure 3. ETS1 transcriptionally activates USP2. (A) According to the data from hTftarget, transcription factors with potential to bind to USP2 were predicted. (B) RT-qPCR assay measured USP2 expression in OM-HBMSCs with knockdown of different transcription factors. (C) Western blot assay analysed the expression of USP2 in OM-HBMSCs with or without ETS1 knockdown. (D-E) ChIP and DNA pull-down assays verified the binding between ETS1 and USP2 promoter. (F) JASPAR predicted the binding sequences of ETS1 on USP2 promoter. (G) Schematic representation of ETS1 binding sites on USP2 promoter as well as mutated binding sites was presented, and luciferase reporter assay determined the specific binding sites between ETS1 and USP2 promoter. **P < 0.01.
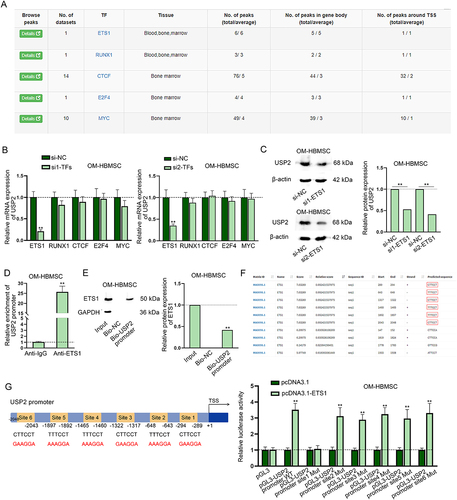
ETS1 up-regulates USP2 to induce the osteogenic differentiation of HBMSCs
Subsequently, we intended to figure out whether ETS1 could facilitate the osteogenic differentiation of HBMSCs by up-regulating USP2. Firstly, ALP staining results verified that USP2 overexpression could recover the decreased ALP activity in OM-HBMSC with ETS1 knockdown (). From the ARS staining results, we found USP2 up-regulation could countervail the decline in absorbance value in ETS1-depleted OM-HBMSC (). Moreover, co-transfection of pcDNA3.1-USP2 also counteracted the inhibiting effect of ETS1 silence on the expression levels of RUNX2, ALP, OSX and OCN (). Therefore, it is confirmed that ETS1 can promote the osteogenic differentiation of HBMSCs by up-regulating USP2.
Figure 4. ETS1 up-regulates USP2 to promote the osteogenic differentiation of HBMSCs.Experiments were carried out with OM-HBMSCs (14 days after osteogenic differentiation) with transfection of si-NC, si1-ETS1, si1-ETS1+pcDNA3.1 or si1-ETS1+pcDNA3.1-USP2. (A-B) ALP staining assay and ARS staining assay examined the influence of ETS1 knockdown and USP2 augment on the ALP activity and formation of mineralized bone matrix of OM-HBMSCs. (C-D) RT-qPCR and western blot detected RUNX2, ALP, OSX and OCN levels in OM-HBMSCs. **P < 0.01.
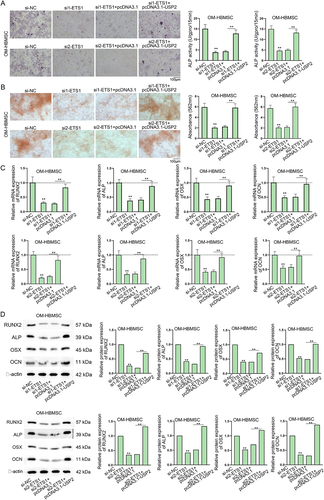
USP2-AS1 up-regulates USP2 via KDM3A-mediated demethylation of H3K9me2 in ETS1 promoter
Our subsequent assays were to verify whether USP2-AS1 positively regulated USP2 through modulating ETS1. First of all, we predicted the binding potential between USP2-AS1 and ETS1 on RPISeq website (http://pridb.gdcb.iastate.edu/RPISeq/) (). Then, we conducted RIP assay and the result indicated that USP2-AS1 did not directly interact with ETS1 in OM-HBMSCs (). Furthermore, ALP and ARS staining disclosed that USP2-AS1 overexpression could not rescue the inhibiting effect of ETS1 knockdown on osteogenic differentiation (Fig. S4A-B). Continuously, we conducted RT-qPCR to unveil the impact of USP2-AS1 silencing on ETS1 expression in HBMSCs on day 14 of osteogenic induction and we noticed that ETS1 expression was reduced with USP2-AS1 silencing (). According to recent studies, KDM3A can regulate ETS1 via the demethylation of H3K9me2 [Citation29–31], and KDM3A plays a critical role during the osteogenic differentiation of HBMSCs [Citation32]. Therefore, we speculated that KDM3A might participate in the USP2-AS1/ETS1 regulatory network. To validate our speculation, we silenced KDM3A (Fig. S5A) and found that ETS1 expression was significantly reduced after KDM3A knockdown (). Furthermore, RPISeq prediction showed a high binding potential between USP2-AS1 and KDM3A (). According to Hum-mPLoc 2.0 (http://www.csbio.sjtu.edu.cn/bioinf/hum-multi-2/) prediction, KDM3A existed in cell nucleus (Fig. S5B). Through FISH-IF assay, we observed that they were co-localized in cell nucleus (). Next, we determined the interaction between USP2-AS1 and KDM3A in OM-HBMSCs through RIP and RNA pull-down assays (). In addition, we identified that USP2-AS1 and KDM3A could not regulate each other, according to the results of RT-qPCR and western blot (Fig. S5C-D). Moreover, KDM3A knockdown induced ETS1 downregulation was found to be recovered after overexpression of USP2-AS1 (). Subsequently, we verified whether KDM3A up-regulated ETS1 via the H3K9me2 demethylation as previously validated [Citation29–31]. According to western blot analysis, KDM3A silencing led to the decreased level of ETS1 protein and the increased level of H3K9me2 (). ChIP assay further demonstrated the affinity of H3K9me2 and KDM3A in ETS1 promoter; moreover, knockdown of KDM3A decreased the H3K9me2 level in ETS1 promoter (). Finally, the RT-qPCR and western blot results revealed that the effect of KDM3A silencing on the expression of USP2 could be completely abolished by overexpression of ETS1 (). To conclude, USP2-AS1 up-regulates USP2 via KDM3A-mediated ETS1 upregulation.
Figure 5. USP2-AS1 up-regulates ETS1 via recruiting KDM3A.(A) The binding potential between USP2-AS1 and ETS1 was predicted on RPISeq website. (B) In RIP assay, RT-qPCR analysed the enrichment of USP2-AS1 in Anti-IgG and Anti-ETS1 in OM-HBMSCs. (C-D) RT-qPCR detected ETS1 expression upon USP2-AS1 silencing and KDM3A knockdown in OM-HBMSCs. (E) RPISeq website predicted the binding potential between USP2-AS1 and KDM3A. (F) FISH-IF assays determined the co-localization of USP2-AS1 and KDM3A in OM-HBMSCs. (G-H) RIP and RNA pull-down assays confirmed the binding relationship between USP2-AS1 and KDM3A. (I) RT-qPCR assay verified the influence of KDM3A depletion and USP2-AS1 overexpression on the expression of ETS1. (J) western blot detected protein levels of ETS1 and H3K9me2 in KDM3A-silenced OM-HBMSCs. (K) ChIP assay analysed the interaction between H3K9me2 and ETS1 promoter in OM-HBMSCs with or without KDM3A deficiency. (L-M) RT-qPCR and western blot quantified USP2 expression in OM-HBMSCs with different transfection. **P < 0.01; n.S.: no significance.
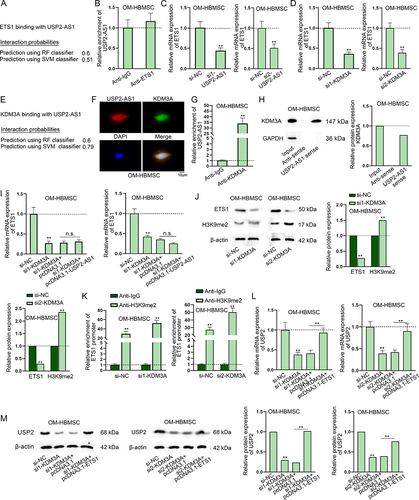
USP2 stabilizes β-catenin protein to activate the Wnt/β-catenin signalling pathway
Since USP2 is a deubiquitination protease, we continued to predict the proteins interacting with USP2. According to the prediction result of BioGRID (https://thebiogrid.org/), UBC, TRAF2, FASN, CTNNB1, MDM4 and RIPK1 were with highest confidence (). Among them, CTNNB1 (also named β-catenin) is a recognized participant of Wnt signalling pathway, which has been proven to be critical for osteogenic differentiation of HBMSCs [Citation33,Citation34]. Therefore, we chose β-catenin for further analysis. GST pull-down assay and Co-IP assay verified the interaction between USP2 and β-catenin (). Afterwards, we knocked down USP2 expression (Fig. S5E) and conducted IP-western blot assay to verify that USP2 down-regulation could promote the ubiquitination of β-catenin (). In the meantime, western blot results showed the significant decreased protein level of β-catenin in response to USP2 knockdown (). We further detected β-catenin protein level in OM-HBMSCs with or without USP2 knockdown at different time points after CHX treatment, and found that β-catenin protein was degraded more quickly in CHX-treated OM-HBMSCs after USP2 knockdown (). Afterwards, we observed from TOP/FOP flash assay that USP2 silencing significantly inhibited the activity of Wnt/β-catenin signalling pathway (). Finally, rescue assays revealed that overexpression of USP2 recovered the USP2-AS1 knockdown-induced downregulation of β-catenin protein (Fig. S5F). Consistently, USP2 overexpression counteracted the suppressing effect of USP2-AS1 silence on the activity of Wnt/β-catenin signalling pathway (Fig. S5G). Collectively, USP2-AS1 positively modulates USP2 to deubiquitinate β-catenin and induce Wnt/β-catenin pathway activation (Fig. S6).
Figure 6. USP2 stabilizes β-catenin protein to activate the Wnt/β-catenin signalling pathway.(A) Interacting proteins of USP2 were predicted on BioGRID database. (B) GST pull-down assay verified the interaction between USP2 and β-catenin. (C) CoIP assay further verified the interaction between USP2 and β-catenin. (D) western blot assay verified the influence of USP2 depletion on the ubiquitination of β-catenin. (E-F) western blot detected the impact of USP2 depletion on the protein level and stability of β-catenin. (G) TOP/FOP flash assay assessed the influence of USP2 on Wnt/β-catenin signalling pathway. **P < 0.01.
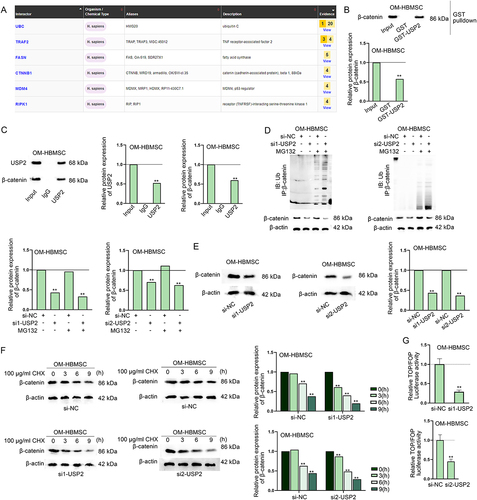
Discussion
HBMSCs are functional cells used for bone formation and regeneration, and the elucidation of the mechanisms underlying the osteogenic differentiation of stem cells can be conducive to developing suitable bone filling materials for patients requiring bone tissue regeneration [Citation35]. Accumulating researches have unveiled the critical roles of lncRNAs in osteogenic differentiation of HBMSCs [Citation36]. For example, lncRNA IGF2-AS enhances osteogenic differentiation of HBMSCs by targeting miR-3126-5p/KLK4 [Citation37;] lncRNA XIST inhibits the osteogenic differentiation of HBMSCs [Citation38]. Here, we selected USP2-AS1 as our research target due to its significant up-regulation in HBMSCs after osteogenic differentiation, according to the results of bioinformatics analysis and RT-qPCR. According to previous literatures, USP2-AS1 functions as an oncogenic factor in colon cancer and ovarian cancer [Citation8,Citation9]. The experimental results in the current study firstly verified the promoting effects of USP2-AS1 on the osteogenic differentiation of HBMSCs, which was evidenced by the results that USP2-AS1 knockdown led to the decreased ALP activity, reduced formation of mineralized bone matrix and lessened expression levels of osteogenesis-associated markers (RUNX2, ALP, OSX and OCN).
Recent studies have revealed that the interaction between lncRNAs and their nearby genes is essential to multiple cellular processes and cancer progression [Citation14]. In this study, we found that USP2-AS1 could positively regulate the expression of its nearby gene USP2. The former study disclosed that USP2 has ability to maintain cancer stem cell population by activating Bmi1 and epithelial-mesenchymal transition through up-regulating Twist [Citation39]. Our findings demonstrated that USP2 overexpression could completely countervail the suppressing impacts of USP2-AS1 silence on the osteogenic differentiation of HBMSCs. Furthermore, we verified the co-localization of USP2-AS1 and USP2 in both nucleus and cytoplasm, suggesting the possibility of both transcriptional and post-transcriptional modulation mechanisms. However, the results of luciferase reporter assay indicated that USP2-AS1 upregulated USP2 by activating its transcription. As previously reported, lncRNA GAS5 increases the expression of PARP1 by recruiting transcription factor E2F4 to PARP1 promoter in ovarian cancer cells [Citation40]. Similarly, PAXIP1-AS1 recruits transcription factor ETS1 to up-regulate KIF14, thus contributing to strengthened cell invasion and angiogenesis in glioma [Citation41]. Transcription factors including RUNX2, SOX9 and GATA4 act as the critical factors related to the osteogenic differentiation of mesenchymal stem cells [Citation42]. ETS1 is a transcription factor that plays a regulatory role in the osteogenic differentiation of MC3T3-E1 cells [Citation43]. In our study, we carried out assays and validated that ETS1 could bind to USP2 promoter region and activate USP2 transcription. Moreover, ETS1 promoted the osteogenic differentiation of HBMSCs by up-regulating USP2. USP2-AS1 upregulated EST1 expression but could not directly bind with it in OM-HBMSCs. The previous studies revealed that KDM3A can regulate ETS1 via the demethylation of H3K9me2 [Citation29–31] and KDM3A exerts critical influences during the osteogenic differentiation of HBMSCs [Citation32]. Similarly, we validated that USP2-AS1 interacted with KDM3A to regulate ETS1 via the demethylation of H3K9me2, and thereby enhanced USP2 expression.
Wnt/β-catenin signalling pathway serves as a critical effector for the osteogenic differentiation of HBMSCs [Citation33,Citation34]. For example, lncRNA LINC00707 promotes the osteogenic differentiation of HBMSCs through the Wnt/β-catenin pathway [Citation44]. Consistently, the present findings indicated that USP2 stabilized β-catenin protein to activate the Wnt/β-catenin signalling pathway.
In conclusion, our study proved that USP2-AS1 can facilitate the osteogenic differentiation of HBMSCs by recruiting KDM3A to activate USP2 transcription, and thus enhancing β-catenin protein stability to activate the Wnt/β-catenin signalling pathway. At present, lack of clinical data remains a major limitation of this study. Therefore, we will try to validate USP2-AS1/KDM3A/ETS1/USP2 axis in clinical samples in our future study.
Supplementary file 3.tif
Download TIFF Image (1.9 MB)Supplementary file 1.xlsx
Download MS Excel (13.5 KB)Figure S6.tif
Download TIFF Image (34.9 MB)Figure S4.tif
Download TIFF Image (27.1 MB)Supplementary file 7.tif
Download TIFF Image (11.6 MB)Figure S2.tif
Download TIFF Image (9.1 MB)Supplementary file 2.tif
Download TIFF Image (1.8 MB)Figure S5.tif
Download TIFF Image (6.2 MB)Supplementary file 5.tif
Download TIFF Image (2.2 MB)Figure S3.tif
Download TIFF Image (5.8 MB)Supplementary file 4.tif
Download TIFF Image (1.3 MB)Supplementary file 6.tif
Download TIFF Image (14.3 MB)Figure S1.tif
Download TIFF Image (6.2 MB)Acknowledgments
We thank all our experimenters for their efforts.
Disclosure statement
No potential conflict of interest was reported by the author(s).
Supplementary material
Supplemental data for this article can be accessed online at https://doi.org/10.1080/15476286.2023.2290771
Additional information
Funding
References
- Dragojevič J, Logar DB, Komadina R, et al. Osteoblastogenesis and adipogenesis are higher in osteoarthritic than in osteoporotic bone tissue. Arch Med Res. 2011;42(5):392–7. doi: 10.1016/j.arcmed.2011.08.005
- Yang X, Yang J, Lei P, et al. LncRNA MALAT1 shuttled by bone marrow-derived mesenchymal stem cells-secreted exosomes alleviates osteoporosis through mediating microRNA-34c/SATB2 axis. Aging. 2019;11(20):8777–91. doi: 10.18632/aging.102264
- Huang Y. The novel regulatory role of lncRNA-miRNA-mRNA axis in cardiovascular diseases. J Cellular Molecular Medi. 2018;22(12):5768–75. doi: 10.1111/jcmm.13866
- Tye CE, Boyd JR, Page NA, et al. Regulation of osteogenesis by long noncoding RNAs: an epigenetic mechanism contributing to bone formation. Connect Tissue Res. 2018;59(sup1):35–41.
- Zheng S, Wang YB, Yang YL, et al. LncRNA MALAT1 inhibits osteogenic differentiation of mesenchymal stem cells in osteoporosis rats through MAPK signaling pathway. Eur Rev Med Pharmacol Sci. 2019;23(11):4609–17.
- Shi ZL, Zhang H, Fan ZY, et al. Long noncoding RNA LINC00314 facilitates osteogenic differentiation of adipose-derived stem cells through the hsa-miR-129-5p/GRM5 axis via the Wnt signaling pathway. Stem Cell Res Ther. 2020;11(1):240.
- Wu H, Cao F, Zhou W, et al. Long noncoding RNA FAM83H-AS1 modulates SpA-Inhibited osteogenic differentiation in human bone mesenchymal stem cells. Mol Cell Biol. 2020;40(5):40.
- Li D, Bao J, Yao J, et al. lncRNA USP2-AS1 promotes colon cancer progression by modulating Hippo/YAP1 signaling. Am J Transl Res. 2020;12(9):5670–82.
- Guo B, Yu L, Sun Y, et al. Long non-coding RNA USP2-AS1 accelerates cell proliferation and migration in ovarian cancer by sponging miR-520d-3p and up-regulating KIAA1522. CMAR. 2020;12:10541–50. doi: 10.2147/CMAR.S268863
- Baek D, Park KH, Lee KM, et al. Ubiquitin-specific protease 53 promotes osteogenic differentiation of human bone marrow-derived mesenchymal stem cells. Cell Death Dis. 2021;12(3):238.
- Kong X, Duan Y, Sang Y, et al. LncRNA–CDC6 promotes breast cancer progression and function as ceRNA to target CDC6 by sponging microRNA-215. J Cell Physiol. 2019;234(6):9105–17.
- He J, Yu J. Long noncoding RNA FAM83A-AS1 facilitates hepatocellular carcinoma progression by binding with NOP58 to enhance the mRNA stability of FAM83A. Biosci Rep. 2019;39(11):39. doi: 10.1042/BSR20192550
- Pan L, Li Y, Jin L, et al. TRPM2-AS promotes cancer cell proliferation through control of TAF15. Int J Biochem Cell Biol. 2020;120:105683. doi: 10.1016/j.biocel.2019.105683
- Yang T, Chen WC, Shi PC, et al. Long noncoding RNA MAPKAPK5-AS1 promotes colorectal cancer progression by cis-regulating the nearby gene MK5 and acting as a let-7f-1-3p sponge. J Exp Clin Cancer Res. 2020;39(1):139.
- Jia B, Wang Z, Sun X, et al. Long noncoding RNA LINC00707 sponges miR-370-3p to promote osteogenesis of human bone marrow-derived mesenchymal stem cells through upregulating WNT2B. Stem Cell Res Ther. 2019;10(1):67. doi: 10.1186/s13287-019-1161-9
- Liu J, Wu M, Feng G, et al. Downregulation of LINC00707 promotes osteogenic differentiation of human bone marrow‑derived mesenchymal stem cells by regulating DKK1 via targeting miR‑103a‑3p. Int J Mol Med. 2020;46(3):1029–38. doi: 10.3892/ijmm.2020.4672
- Zhang Y, Huang YX, Wang DL, et al. LncRNA DSCAM-AS1 interacts with YBX1 to promote cancer progression by forming a positive feedback loop that activates FOXA1 transcription network. Theranostics. 2020;10(23):10823–37.
- Deng SJ, Chen HY, Ye Z, et al. Hypoxia-induced LncRNA-BX111 promotes metastasis and progression of pancreatic cancer through regulating ZEB1 transcription. Oncogene. 2018;37(44):5811–28.
- Gong J, Fan H, Deng J, et al. LncRNA HAND2-AS1 represses cervical cancer progression by interaction with transcription factor E2F4 at the promoter of C16orf74. J Cellular Molecular Medi. 2020;24(11):6015–27. doi: 10.1111/jcmm.15117
- Zheng S, Yang L, Zou Y, et al. Long non-coding RNA HUMT hypomethylation promotes lymphangiogenesis and metastasis via activating FOXK1 transcription in triple-negative breast cancer. J Hematol Oncol. 2020;13(1):17.
- Ma Y, Jin Y, Li C, et al. LncRNA MSC-AS1 motivates the development of melanoma by binding to miR-302a-3p and recruiting IGF2BP2 to elevate LEF1 expression. Exp Dermatol. 2021;30(12):1764–74. doi: 10.1111/exd.14427
- Zhang Y, Chen W, Pan T, et al. LBX2-AS1 is activated by ZEB1 and promotes the development of esophageal squamous cell carcinoma by interacting with HNRNPC to enhance the stability of ZEB1 and ZEB2 mRnas. Biochem Biophys Res Commun. 2019;511(3):566–72. doi: 10.1016/j.bbrc.2019.02.079
- Fan X, Teng Y, Ye Z, et al. The effect of gap junction-mediated transfer of miR-200b on osteogenesis and angiogenesis in a co-culture of MSCs and HUVECs. J Cell Sci. 2018;131. doi: 10.1242/jcs.216135
- Zhang W, Zhu Y, Chen J, et al. Mechanisms of miR‑128‑3p in inhibiting osteoblast differentiation from bone marrow‑derived mesenchymal stromal cells. Mol Med Rep. 2020;22(6):5041–52. doi: 10.3892/mmr.2020.11600
- Park KH, Choi Y, Yoon DS, et al. Zinc promotes osteoblast differentiation in human mesenchymal stem cells via activation of the cAMP-PKA-CREB signaling pathway. Stem Cells Dev. 2018;27(16):1125–35. doi: 10.1089/scd.2018.0023
- Zhang X, Chen J, Liu A, et al. Stable overexpression of p130/E2F4 affects the multipotential abilities of bone-marrow-derived mesenchymal stem cells. J Cell Physiol. 2018;233(12):9739–49.
- Wang Q, Cai J, Cai XH, et al. miR-346 regulates osteogenic differentiation of human bone marrow-derived mesenchymal stem cells by targeting the Wnt/β-catenin pathway. PLoS One. 2013;8(9):e72266. doi: 10.1371/journal.pone.0072266
- Piek E, Sleumer LS, van Someren EP, et al. Osteo-transcriptomics of human mesenchymal stem cells: accelerated gene expression and osteoblast differentiation induced by vitamin D reveals c-MYC as an enhancer of BMP2-induced osteogenesis. Bone. 2010;46(3):613–27.
- Sobral LM, Sechler M, Parrish JK, et al. KDM3A/Ets1/MCAM axis promotes growth and metastatic properties in Rhabdomyosarcoma. Genes Cancer. 2020;11(1–2):53–65.
- Sobral LM, Hicks HM, Parrish JK, et al. KDM3A/Ets1 epigenetic axis contributes to PAX3/FOXO1-driven and independent disease-promoting gene expression in fusion-positive Rhabdomyosarcoma. Mol Oncol. 2020;14(10):2471–86.
- Liu J, Li D, Zhang X, et al. histone demethylase KDM3A promotes cervical cancer malignancy through the ETS1/KIF14/Hedgehog axis. OTT. 2020;13:11957–73. doi: 10.2147/OTT.S276559
- Wu JC, Sun J, Xu JC, et al. Down-regulated microRNA-199a-3p enhances osteogenic differentiation of bone marrow mesenchymal stem cells by targeting Kdm3a in ovariectomized rats. Biochem J. 2021;478(4):721–34. doi: 10.1042/BCJ20200314
- Shen G, Ren H, Shang Q, et al. Foxf1 knockdown promotes BMSC osteogenesis in part by activating the Wnt/β-catenin signalling pathway and prevents ovariectomy-induced bone loss. EBioMedicine. 2020;52:102626. doi: 10.1016/j.ebiom.2020.102626
- Hang K, Ye C, Xu J, et al. Apelin enhances the osteogenic differentiation of human bone marrow mesenchymal stem cells partly through Wnt/β-catenin signaling pathway. Stem Cell Res Ther. 2019;10(1):189.
- Fan T, Qu R, Yu Q, et al. Bioinformatics analysis of the biological changes involved in the osteogenic differentiation of human mesenchymal stem cells. J Cellular Molecular Medi. 2020;24(14):7968–78.
- Yang Q, Jia L, Li X, et al. Long noncoding RNAs: new players in the osteogenic differentiation of bone marrow- and adipose-derived mesenchymal stem cells. Stem Cell Rev and Rep. 2018;14(3):297–308.
- Tang JZ, Zhao GY, Zhao JZ, et al. lncRNA IGF2-AS promotes the osteogenic differentiation of bone marrow mesenchymal stem cells by sponging miR-3,126-5p to upregulate KLK4. J Gene Med. 2021;23(10):e3372. doi: 10.1002/jgm.3372
- Chen X, Yang L, Ge D, et al. Long non‑coding RNA XIST promotes osteoporosis through inhibiting bone marrow mesenchymal stem cell differentiation. Exp Ther Med. 2019;17:803–11. doi: 10.3892/etm.2018.7033
- He J, Lee HJ, Saha S, et al. Inhibition of USP2 eliminates cancer stem cells and enhances TNBC responsiveness to chemotherapy. Cell Death Dis. 2019;10(4):285. doi: 10.1038/s41419-019-1512-6
- Long X, Song K, Hu H, et al. Long non-coding RNA GAS5 inhibits DDP-resistance and tumor progression of epithelial ovarian cancer via GAS5-E2F4-PARP1-MAPK axis. J Exp Clin Cancer Res. 2019;38(1):345.
- Xu H, Zhao G, Zhang Y, et al. Long non-coding RNA PAXIP1-AS1 facilitates cell invasion and angiogenesis of glioma by recruiting transcription factor ETS1 to upregulate KIF14 expression. J Exp Clin Cancer Res. 2019;38(1):486.
- Almalki SG, Agrawal DK. Key transcription factors in the differentiation of mesenchymal stem cells. Differentiation. 2016;92(1–2):41–51. doi: 10.1016/j.diff.2016.02.005
- Fan Q, Li Y, Sun Q, et al. miR-532-3p inhibits osteogenic differentiation in MC3T3-E1 cells by downregulating ETS1. Biochem Biophys Res Commun. 2020;525(2):498–504. doi: 10.1016/j.bbrc.2020.02.126
- Cai WL, Zeng W, Liu HH, et al. LncRNA LINC00707 promotes osteogenic differentiation of hBmscs through the Wnt/β-catenin pathway activated by LINC00707/miR-145/LRP5 axis. Eur Rev Med Pharmacol Sci. 2020;24(1):18–28. doi: 10.26355/eurrev_202001_19891