Abstract
Particulate matter exposures have been linked to increased mortality and morbidity that may be associated with immune dysfunction. Therefore, Lupus-prone New Zealand mixed mice (NZM) were intranasally instilled with either 30 μ l saline or 30 μ l saline suspensions of 500 μ g acid-washed PM 1648, PM 1648 or PM2.5 collected in Houston, TX, once a week for 4 weeks. Lung injury, mortality, and various immune dysfuntions were assessed. Accelerated mortality was observed in the PM 1648 and PM2.5 instilled mice compared to the acid-washed PM 1648 and saline-instilled mice. PM 1648 and PM2.5 significantly suppressed the natural development of anti-nuclear antibodies in NZM mice at 16 weeks. IgG serum levels were significantly increased in the acid-washed PM 1648 instilled mice at 8 weeks following PM instillation compared to the saline-instilled group. In contrast, IgG serum levels were significantly decreased in the PM 1648 and PM2.5 instilled mice at 8 weeks post-PM instillation as compared to the acid-washed PM 1648 instilled group. There were increases in the amount of immune cell infiltration, fibrosis and collagen deposition within the lungs of PM 1648 and PM2.5 groups in comparison within the saline- and acid-washed PM 1648 instilled mice. These results demonstrate that PM has an immunosuppressive effect on the development of anti-nuclear autoantibodies and modulates the IgG responses in this model of autoimmune disease.
INTRODUCTION
Particulate matter is a complex mixture of organic, inorganic, and biological compounds including diesel exhaust particles (DEP), polycyclic aromatic hydrocarbons (PAHs), endotoxin, minerals and metals that differs in mass, size, and chemical constituents (Huang, Hsu, and Chan, Citation2003; Pozzi et al., Citation2003). These particles are categorized either in 3 size ranges, PM1.0 (diameters < 1 μ m), PM1.0−2.5 (diameters between 1.0–2.5 μ m), and PM2.5−10 (diameters between 2.5–10 μ m) (Huang, Hsu, and Chan, Citation2003) or as coarse particles ranging from 2.5–10 μ m and fine fraction including particles with an aerodynamic diameter of about 0.1–2.5 μ m (PM2.5) (Pozzi et al., Citation2003). The coarse fraction consists of particles that are derived mainly from natural sources such as dust and other geogenic material, while the fine fraction is a mixture of carbonaceous material derived from anthropogenic emissions (Pozzi et al., Citation2003). The effects of airborne particulate matter on the immune system have been most widely studied in the respiratory tract, however there is lack of data on the effects of air pollution on the immune system (Albright and Goldstein, Citation1996; Leonardi et al., Citation2000). Particulate matter could result in local or systemic immune responses that can be manifested as either immune enhancement or immunosuppression (Albright and Goldstein, Citation1996). For example, polycyclic aromatic hydrocarbons (PAHs) are important organic components of particulate matter that can affect the immune system (Albright and Goldstein, Citation1996; Huang, Hsu, and Chan, Citation2003). PAHs may function as potent immunosuppressive agents that can act directly on B and T lymphocytes (Albright and Goldstein, Citation1996).
There is a clear underlying genetic basis for the susceptibility to immunologic disorders resulting from exposure to pollutants (Albright and Goldstein, Citation1996). Lupus-prone New Zealand mixed mice provide an appropriate model to assess the effects of environmental agents on the progression of autoimmune diseases such as systemic lupus erythematosus (SLE) in a sensitive population (Rudofsky and Lawrence, Citation1999; Brown et al., Citation2003). NZM mice are a moderately lupus-prone strain with reduced disease penetrance, therefore an environmental influence on SLE in these mice would be more readily observed (Rudofsky and Lawrence, Citation1999; Brown et al., Citation2003). These mice spontaneously develop pathologic hallmarks of SLE between 6 months and 1 year of age (Rudofsky and Lawrence, Citation1999; Brown et al., Citation2003). The most characteristic of these traits are the development of anti-ssDNA, anti-dsDNA, anti-histone, anti-nuclear antigen (ANA), and anti-IgG autoantibodies as well as increased levels of circulating immune complexes (Rudofsky and Lawrence, Citation1999; Brown et al., Citation2003).
A study by Kleinman et al. (Citation2000) demonstrated that fine particle (PM2.5) exposure can result in pulmonary injury and impaired host defenses in aged rats. There is also epidemiological evidence, which indicates that exposure to elevated levels of particulate matter exacerbates viral pulmonary infections (Lambert et al., Citation2003). Lambert et al., investigated the possible immunomodulatory effect of particle exposure on immune responses to respiratory syncytial virus (RSV), which is the major cause of bronchiolitis and pneumonia in infants (Lambert et al., Citation2003). Their study indicated that preexposure to ultrafine particles suppresses IFN-γ production that is necessary for microbial defense in the lungs of these mice at 1 and 2 days after infection (Lambert et al., Citation2003).
The present study was designed to test the hypothesis that particulate matter exerts immunomodulatory effects on the natural progression of systemic autoimmune disease in SLE-prone NZM mice. The disease course was investigated by following the development of autoantibodies, serum immunoglobulins and T-helper type 1 and 2 cytokines, proteinuria, and pulmonary fibrosis.
MATERIALS AND METHODS
Mice
Male and female New Zealand Mixed (NZM 2410) mice were obtained from Taconic (Germantown, NY) and maintained in microisolator containers in accordance with the Guide for the Care and Use of Laboratory Animals, prepared by the Institute of Laboratory Animal Resources, National Resource Council. The animal room is set on 12-hour light/dark cycles with food and water provided ad libitum.
Particle Matter
Standard reference material 1648 (PM 1648) was obtained from the National Institute of Standards and Technology (NIST). These particles were collected in St. Louis, MO, over a 24-month period (1974–1976) in a bag-house designed especially for the purpose. The collected particulate material was removed from the filter bags, combined into a single lot, screened through a fine-mesh sieve to remove extraneous material, and blended thoroughly (NIST, 1998).
In order to obtain acid-washed PM 1648 with a different surface composition as compared to PM 1648, a fraction of PM 1648 was treated with acid. A 0.5 g aliquot of PM was extracted with acid (HNO3) to remove both metallic and organic components. The acid-treated particles were digested in a microwave oven with concentrated HNO3 for 10 minutes, and the particles were treated as described by Obot et al. (Citation2002).
The PM2.5 (mass median diameter [MMD] ≤2.5 μ m) was collected over a 6-month period in Houston, TX, using model 400 microenvironmental monitors with a 2.5-μ m size-selective impactor (MSP Corporation, Shoreview, MN). The samplers were located at the U.S. Environmental Protection Agency (EPA) site number 48-201-1035 (CAMS113, Clinton Monitoring Site). PM2.5 samples were collected on Poretics 2-μ m, 37-mm polyester filters (Osmonics, Inc., Minnetonka, MN) at an average flow rate of 10 L/min. The duration of sampling varied depending on filter particle load, so that variability in flow rate would be maintained at ± 10%. Individual filters were stored frozen until PM extraction. Removal of particles was done by cup-horn sonication of pooled filters immersed in sterile water. The PM2.5 suspension was concentrated by a series of sequential ultracentrifugations in an Optima TLX (Beckman Coulter, Fullerton, CA). Finally, the recovered PM2.5 was dried in a Speed Vac concentrator (Savant, Thermo Forma) and weighed.
Treatment of Mice
At 6 weeks of age, equal numbers of male and female NZM mice were anesthetized with ketamine (1.25 mg/kg) and intranasally instilled with 30 μ L saline (n = 6) or 30 μ L saline suspensions of 500 μ g acid-washed PM 1648 (n = 6), PM 1648 (n = 6), or PM2.5 (n = 6) collected in Houston, TX. All mice received 4 instillations 1 week apart in order to represent several exposures over a period of time. Blood was drawn from the mice by a saphenous vein bleed to collect sera every 2 weeks until sacrificed at 17 weeks postexposure to monitor autoantibody and Ig levels. Upon sacrifice lungs were removed for histology.
Detection of Serum Autoantibodies
ANA and anti-histone antibodies were detected by ELISA kits (Alpha Diagnostics, San Antonio, TX). Sera were diluted 100-fold prior to assay and manufacturer's protocol was followed.
Serum Immunoglobulin Quantification
Serum IgM, IgG, IgG1, IgG2a, IgG2b, and IgG3 levels were quantified by ELISA. Then, 96-well Polysorp Nalge-Nunc ELISA plates (Fisher) were coated with 100 μ L of 1 μ g/ml goat anti-mouse kappa light chain antibody (Southern Biotechnology Associates, Birmingham, AL) diluted in phosphate-buffered saline (PBS) and incubated overnight at 4°C. Plates were blocked with 200 μ L PBS-1% bovine serum albumin (BSA) for 1 hour at room temperature followed by 3 washes with PBS-0.1% Tween 20. Sera were diluted in PBS-0.1% Tween 20/1% BSA. Standard curves were generated using unlabeled IgG (Southern Biotechnology Associates) starting at 0.5 μ g/ml, unlabeled IgM (Sigma Chemical, St. Louis, MO) starting at 1 μ g/ml, unlabeled IgG1 (Southern Biotechnology Associates) starting at 0.1 μ g/ml, unlabeled IgG2a (Sigma) starting at 0.25 μ g/ml, unlabeled IgG2b (Southern Biotechnology Associates) starting at 0.05 μ g/ml, unlabeled IgG3 (Sigma) starting at 0.5 μ g/ml. Diluted sera were added to wells and incubated in duplicate for 1 hour at room temperature followed by 3 washes with PBS-0.1% Tween 20. HRP-conjugated goat anti-mouse IgG (Fc-fragment specific) (Jackson ImmunoResearch Laboratories, West Grove, PA), IgM, IgG1, IgG2a, IgG2b and IgG3 (Caltag Laboratories, Burlingame, CA) were diluted 2,000-fold in PBS-0.1% Tween 20/1% BSA and incubated for 1 hour at room temperature followed by 3 washes with PBS-0.1% Tween 20. Then, 100 μ L One Step TMB substrate (Zymed, San Francisco, CA) was added and incubated for 15 minutes followed by the addition of 100 μ L 2N H2SO4 to stop the reaction. The optical density was read at 450 nm and serum immunoglobulin concentration was calculated by extrapolation from the linear portion of the standard curve. All samples were tested in duplicate.
Urinary Protein
Proteinuria was measured every 2 weeks by Chemstrip 2 GP test strips as described by the manufacturer (Boehringer Mannheim Diagnostics, Indianapolis, IN). Milligram protein per deciliter was measured among groups following the provided scale (0 = negative, trace; 1+ = 30 mg/dl; 2+ = 100 mg/dl; 3+ = 500 mg/dl).
Cytokine Analysis
Cytokine levels were quantified by use of a Luminex based immunoassay from Linco Research (St. Charles, MO). A 5-plex assay measuring IFN-γ, IL-4, IL-10, and IL-12 was used with serum from saline, acid-washed PM 1648, PM 1648, and PM2.5 treated NZM mice 17 weeks following instillation. The protocol provided by Linco Research was followed and the samples were analyzed on a Luminex 100 instrument (Luminex, Austin, TX).
Histology
Animals were given a lethal injection of sodium pentobarbital (5 mg i.p.) and the lungs were perfused with Histochoice fixative (Amresco Inc, Solon, IL). Lungs were removed and routinely processed using an automated processor (ThermoShandon, Pittsburgh, PA). The lungs were embedded in paraffin wax and sectioned 10-μ m thick, then collected on poly L-lysine coated slides (Sigma). Using an automated stainer (ThermoShandon) lung samples were stained with hematoxylin and eosin for general cellular morphology and Gomori Trichome for collagen deposition. Samples were blinded and examined and scored under light microscopy. Lungs were scored according to the following scale for inflammation and fibrosis: 1 = none, 2 = minimal, 3 = moderate, 4 = severe.
Statistical Analysis
Differences between saline-treated and -acid washed PM 1648, PM 1648, or PM2.5 treated mice were assessed using 1-factor analysis of variance (1-way ANOVA), Dunnett and Tukey multiple comparison tests. All values are reported as mean ± SEM; p ≤ 0.05 was considered significant.
RESULTS
Effects of Particulate Matter on Mortality, Proteinuria and Pulmonary Toxicity in NZM Mice
A consistent association between levels of particulate matter in the ambient air and increases in mortality due to respiratory and cardiovascular complications has been reported (Salvi et al., Citation1999; Kleinman et al., Citation2000; Huang, Hsu, and Chan, Citation2003; Pozzi et al., Citation2003). Therefore, the effects of acid-washed PM 1648, PM 1648, PM2.5, and saline on mortality in NZM mice were examined. Mortality was accelerated in the PM 1648 and PM2.5 instilled animals compared to the acid-washed PM 1648 and saline-instilled groups at 12 and 14 weeks following instillation, respectively (). The number of mice in the PM 1648 and PM2.5 groups were decreased by 1 and 2 animals respectively due to premature deaths that occurred during instillations of PM material. Mortality in the saline-exposed NZM mice did not begin until 16 weeks and no mortality was observed in the acid-washed PM 1648 instilled mice that continued to live until sacrificed at 17 weeks following exposure (). Although the number of mice used in this study was limited, these results suggest that PM 1648 and PM2.5 exposure in NZM mice could accelerate mortality in this model.
1 Survival of saline (█) (n = 6), acid-washed PM 1648 (▴) (n = 6), PM 1648 (▾) (n = 5) and PM2.5 (♦) (n = 4) exposed NZM mice. AW PM 1648 = Acid-washed PM 1648.
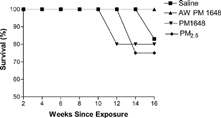
A rapid onset of glomerulonephritis with high proteinuria levels (greater than 500 mg/dl) in both male and female NZM mice have been previously reported (Rudofsky and Lawrence, Citation1999; Brown et al., Citation2003). In this study, none of the particulate or saline instilled NZM mice developed high proteinuria levels within 17 weeks following exposure (data not shown).
To investigate possible lung pathological changes from PM exposure, saline-instilled and acid-washed PM 1648, PM 1648, and PM2.5 treated mice were sacrificed at 17 weeks following exposure and lung samples were collected for histology. Lung sections were stained with hematoxylin and eosin and Gomori's trichrome to examine the development of fibrosis. Histological examination of stained lung sections of PM 1648 and PM2.5 instilled NZM mice demonstrated increases in fibrosis and collagen deposition compared to the saline exposed NZM mice that had minimal collagen deposition (). H & E staining showed increased immune cell infiltration in the PM 1648 and PM2.5 treated animals compared to the saline-exposed mice (). shows blinded scoring of lung sections indicating a slight increase in pulmonary fibrosis and inflammation.
2 Representative examples of Gomori trichrome staining of (a) saline, (b) acid-washed PM 1648, (c) PM 1648, and (d) PM2.5 exposed NZM mice 17 weeks following exposure. The PM 1648 and PM2.5 exposed NZM mice showed increases in fibrosis and collagen deposition represented by the blue staining of collagen. Saline exposed NZM mice had minimal collagen deposition.
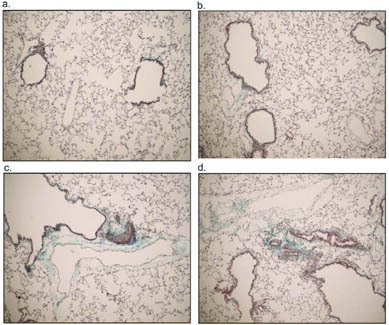
3 Representative examples of H&E staining of (a) saline, (b) acid-washed PM 1648, (c) PM 1648, and (d) PM2.5 exposed NZM mice 17 weeks following exposure. The PM 1648 and PM2.5 exposed NZM mice showed increases in inflammatory cell infiltration. Saline and AW PM1648 had minimal levels of inflammation. The arrows indicate PM within the lungs 17 weeks following instillation.
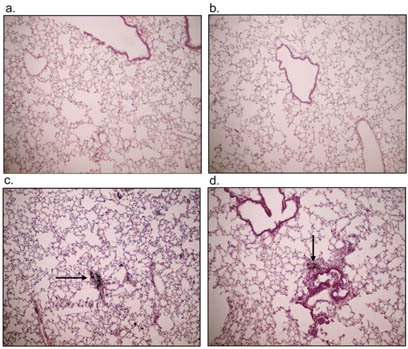
Scoring of lung sections for inflammation and fibrosis
Effects of Particulate Matter on Autoantibody Levels in NZM Mice
One hallmark characteristic of NZM mice is development of high levels of autoantibodies such as anti-histone and anti-nuclear antibodies (ANA) within 6 months of age (Rudofsky and Lawrence, Citation1999; Brown et al., Citation2003). Therefore, these biomarkers were examined in NZM mice following instillation of saline or saline suspensions of acid-washed PM 1648, PM 1648, and PM2.5 at 16 weeks when they should be prevalent. ANA levels measured by ELISA were significantly suppressed in the PM 1648 and PM2.5 treated animals compared to the saline group at 16 weeks postinstillation (0.138 ± 0.042 and 0.152 ± 0.070 versus 0.469 ± 0.090, P ≤ 0.05, respectively) (). Anti-histone antibody levels were not significantly suppressed in the acid-washed PM 1648, PM 1648 and PM2.5 exposed NZM mice compared to the saline-treated mice at 16 weeks post-PM instillation (0.228 ± 0.04, 0.397 ± 0.041 and 0.329 ± 0.095 versus 0.48 ± 0.168, respectively) suggesting that the decrease in ANA is not due to a decrease in anti-histone autoantibodies (). Taken together, these results demonstrate that particulate matter can suppress the natural development of anti-nuclear autoantibodies in NZM mice.
4 Development of (A) anti-nuclear antigen (ANA) autoantibodies and (B) anti-histone autoantibodies in saline and particulate matter exposed NZM mice measured by ELISA at 16 weeks post-PM instillation. ANA levels were significantly suppressed in the PM 1648 (n = 4) and PM2.5 (n = 3), but not in acid-washed PM 1648 (n = 6) treated animals compared to the saline group (n = 5). * (p ≤ 0.05). No significant differences were observed in the levels of anti-histone autoantibodies. Values reported are means ± SEM. Single-factor analysis of variance (1-way ANOVA) and post hoc Dunnett test were performed. AW 1648 = Acid-washed PM 1648.
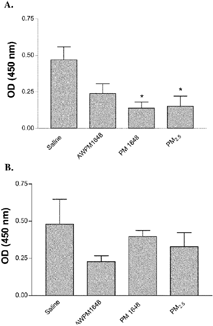
Effects of Particulate Matter on Serum Immunoglobulin Levels in NZM Mice
Due to the observed decreases in the levels of anti-nuclear antibodies (ANA), serum immunoglobulin levels were measured at different time points following PM exposure in NZM mice. IgG, IgM, IgG1, IgG2a, IgG2b, and IgG3 were analyzed. IgM levels were measured at 2, 4, 6, and 16 weeks following PM exposure; however, there were no differences among the groups (data not shown). IgG levels were measured from 2 to 16 weeks following PM exposure (). IgG serum levels were significantly increased in the acid-washed PM 1648 instilled mice at 8 weeks following PM instillation as compared to the saline-instilled group (7951.12 ± 1503.03 μ g/ml versus 4218.3 ± 740.75 μ g/ml, p ≤ 0.05) (). In contrast, IgG serum levels were significantly decreased in the PM 1648 and PM2.5 instilled mice at 8 weeks post-PM instillation as compared to the acid-washed PM 1648 (1293.24 ± 360.27 μ g/ml and 2409.87 ± 1032.77 μ g/ml versus 7951.12 ± 1503.03 μ g/ml, p ≤ 0.001 and p ≤ 0.05, respectively) ().
5 IgG serum levels (μ g/ml) of saline (█) (2–14 weeks, n = 6; 16 weeks, n = 5), acid-washed PM 1648 (▴) (n = 6), PM 1648 (▾) (2–10 weeks, n = 5; 12–16 weeks, n = 4) and PM2.5 (♦) (2–12 weeks, n = 4; 14–16 weeks, n = 3) exposed NZM mice measured by ELISA in NZM mice 2–16 weeks following particulate matter exposure. Serum levels of IgG were significantly increased in the acid-washed PM 1648-instilled mice at 8, weeks following particulate matter instillation as compared to the saline-instilled group. *(p ≤ 0.05). IgG serum levels were significantly decreased in the PM 1648 and PM2.5 instilled mice at the same time point as compared to the acid-washed PM 1648 instilled group. + < eqid1 > + (p ≤ 0.001) and + (p ≤ 0.05). Values reported are means ± SEM. Single-factor analysis of variance (1-way ANOVA) and post hoc Dunnett and Tukey tests were performed. AW PM 1648 = Acid-washed PM 1648.
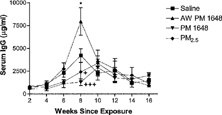
To verify the nature of the observed particulate matterinduced immune responses serum levels of IgG subclasses (IgG1, IgG2a, IgG2b, and IgG3) were measured. However, no significant differences were observed between saline-exposed mice and PM 1648 and PM2.5 exposed mice with regards to the IgG isotypes at 4, 8, and 16 weeks following exposure (data not shown). Taken together, the observed decrease in ANA at 16 weeks following PM exposure is possibly due to an earlier decrease in IgG levels at 8 weeks.
Effects of Particulate Matter on Serum T-Helper Type 1 and 2 Cytokines
IFN-γ, IL-4, IL-10, and IL-12 were measured using a Luminex-based mouse cytokine assay to examine whether differences in serum cytokine profiles would suggest a Th2 type immune response as seen with the previous immunoglobulin levels. However, there were no statistically significant differences in serum cytokine levels between saline- and particulate-matter-treated NZM mice (data not shown).
DISCUSSION
Airborne pollutants including particulate matter (diesel exhaust, aromatic hydrocarbons, etc.) enter via the respiratory tract and subsequently interact with the immune system. This interaction may result in local and systemic responses (Albright and Goldstein, Citation1996). Lupus-prone New Zealand mixed mice provide a model to evaluate the effects of environmental agents on immune dysfunction as well as the progression of autoimmune diseases such as SLE in a sensitive population (Rudofsky and Lawrence, Citation1999; Brown et al., Citation2003). Therefore, in this study we investigated the immunomodulatory effects of particulate matter on the progression of systemic autoimmune disease in SLE prone NZM mice by assessing mortality and following the development of autoantibodies, serum immunoglobulins, T-helper type 1 and 2 cytokines, proteinuria, and pulmonary fibrosis.
We observed accelerated mortality in the PM 1648 and PM2.5 instilled animals compared to the saline instilled animals. Brown et al. (Citation2003) showed a rapid development of high titers of circulating immune complexes, proteinuria and immune complex deposition within the kidneys of silica-exposed NZM mice. They also demonstrated a possible association between development of glomerulonephritis and proteinuria and increased mortality in silica, exposed NZM mice (Brown et al., Citation2003). However, the PM- and saline-exposed NZM mice in this study did not develop high levels of proteinuria. Therefore, the observed acceleration in mortality resulting from PM 1648 or PM2.5 exposure was not due to renal failure. However, pulmonary toxicity and fibrosis have been reported with urban particles with the greatest toxicity seen with PM2.5 (Churg and Brauer, Citation2000; Schwartz and Neas, Citation2000; van Zijverden et al., Citation2001; Buerke et al., Citation2002). Also, there has been a consistent link between increased incidence of mortality and morbidity due to cardiopulmonary complications and elevated levels of particulate matter in the air (Salvi et al., Citation1999; Kleinman et al., Citation2000; Huang, Hsu, and Chan, Citation2003; Pozzi et al., Citation2003). We also demonstrated that PM 1648 and PM2.5 exposed NZM mice showed increases in fibrosis, collagen deposition, and immune cell infiltration in the lungs. Therefore, the observed acceleration in mortality in PM 1648 and PM2.5 instilled mice might have been caused by PM-induced inflammation and complications other than autoimmunity. Whether the PM-induced accelerated mortality is somewhat unique to the NZM model remains to be determined.
Previous studies have reported development of high levels of autoantibodies including anti-histone and anti-nuclear antibodies (ANA) in NZM mice within 6 months to 1 years of age (Rudofsky and Lawrence, Citation1999; Brown et al., Citation2003). Brown et al. (Citation2003) demonstrated that silica significantly elevated levels of serum ANA and anti-histone autoantibodies in NZM mice at 16 weeks following exposure. Therefore, we examined serum ANA at 16 weeks and reported that the saline instilled mice developed high levels of autoantibodies to nuclear antigen that should be seen normally in this model, whereas PM 1648 and PM2.5 instilled mice did not develop high levels of anti-nuclear autoantibodies. Therefore, PM 1648 and PM2.5 significantly suppressed the natural development of ANA in NZM mice. The anti-histone autoantibody levels were not significantly suppressed following PM exposure, therefore, it appears that the decrease in ANA levels was not due to alterations in anti-histone autoantibodies. It appears that particulate matter, unlike silica, suppresses the development of anti-nuclear autoantibody production.
Due to the observed decreases in the levels of anti-nuclear antibodies (ANA), we examined serum levels of immunoglobulins. There were no differences in IgM levels among the groups. Leonardi et al. (Citation2000) reported increased serum levels of total IgG in blood samples collected from children in 25 urban areas of Central Europe, representing a wide range of exposure to outdoor air pollution, with increasing concentration of PM especially PM2.5. On the other hand, we demonstrated a suppressive effect of PM 1648 and PM2.5 on the serum IgG levels in NZM mice. Silica has also been shown to significantly lower serum levels of IgG in NZM mice (Brown et al., Citation2003; Brown, Citation2004). This immunomodulatory effect of silica in NZM mice has been explained by its ability to compromise the immune system (Brown et al., Citation2003). We observed a consistent decrease in both serum levels of IgG and autoantibodies to nuclear antigen with PM 1648 and PM2.5 in NZM mice suggesting an overall suppressive effect on the natural development of autoantibodies in NZM mice.
Due to the decreased IgG levels, we examined immunoglobulin isotypes and alterations in the Th1/Th2 cytokine balance within serum from saline- and PM-treated NZM mice. However, there were no significant changes in serum IgG isotypes or levels of IFN-γ, IL-4, IL-10, or IL-12 at 17 weeks following particulate matter exposure (data not shown). Since we measured the levels of these cytokines 17 weeks following particulate matter exposure, it is possible that the levels of these cytokines had been normalized by then or serum was not the optimum environment to measure the levels of these cytokines. Consistent with these results, silica also did not change IFN-γ, IL-4, IL-10 serum or bronchoalveolar lavage fluid (BALF) levels in NZM mice (Brown, Citation2004).
The mechanism responsible for the PM-induced decrease in anti-nuclear autoantibodies remains unclear. However, potent immunosuppressive effects and airway inflammation have been reported with polycyclic aromatic hydrocarbons (PAHs), an organic component of PM (Albright and Goldstein, Citation1996; Fahy et al., Citation1999). Therefore, one potential mechanism of the observed immnuosupressive effects of PM on the development of ANA autoantibodies and serum levels of IgG in NZM mice may be related to the PAH component of particulate matter. In contrast to PM 1648 and PM2.5, acid-washed PM 1648 did not cause significant suppressive effects on the development of ANA autoantibodies in NZM mice. It also did not show suppressive effects of PM 1648 and PM2.5 on serum IgG levels. This might in part be explained by the fact that acid treatment of PM 1648 removed organic components of PM including PAHs. Therefore, it is possible that acid-washed PM 1648 has lost its immunosuppressive ability due to the lack of PAH component.
In conclusion, particulate matter exposure in the NZM 2410 mouse model of SLE appears to have a potential immunosuppressive effect on the development of anti-nuclear autoantibodies and IgG levels, therefore, possibly decreasing the severity of autoimmune disease in this model. Finally, the ability of both PM 1648 and freshly generated PM2.5 to cause a similar effect suggests that the active component is on both particles and PM 1648 is a good representative of PM2.5 exposure.
ACKNOWLEDGMENTS
We extend our appreciation to Raymond Hamilton for statistical guidance.
This study was supported by U.S. National Institute of Environmental Health Sciences grants ES-11120, RR-017670, and AFPE predoctoral fellowship for JMB.
REFERENCES
- Albright J. F., Goldstein R. A.. 1996. Airborne pollutants and the immune system. Otolaryngol. Head Neck Surg.. 114(2)232–238. [PUBMED], [INFOTRIEVE]
- Brown J. M., Archer A. J., Pfau J. C., Holian A.. 2003. Silica accelerated systemic autoimmune disease in lupus-prone New Zealand mixed mice. Clin. Exp. Immunol.. 131(3)415–421. [CROSSREF], [PUBMED], [INFOTRIEVE]
- Brown J. M., Pfau J. C., Holian A.. 2004. Immunoglobulin and lymphocyte responses following silica exposure in New Zealand mixed mice. Inhal. Toxicol.. 16: 1–7
- Buerke U., Schneider J., Rosler J., Woitowitz H. J.. 2002. Interstitial pulmonary fibrosis after severe exposure to welding fumes. Am. J. Ind. Med.. 41(4)259–268. [CROSSREF], [PUBMED], [INFOTRIEVE]
- Churg A., Brauer M.. 2000. Ambient atmospheric particles in the airways of human lungs. Ultrastruct. Pathol.. 24(6)353–361. [PUBMED], [INFOTRIEVE]
- Fahy O., Tsicopoulos A., Hammad H., Pestel J., Tonnel A. B., Wallaert B.. 1999. Effects of diesel organic extracts on chemokine production by peripheral blood mononuclear cells. J. Allergy Clin. Immunol.. 103(6)1115–1124. [PUBMED], [INFOTRIEVE]
- Huang S. L., Hsu M. K., Chan C. C.. 2003. Effects of submicrometer particle compositions on cytokine production and lipid peroxidation of human bronchial epithelial cells. Environ. Health Perspect.. 111(4)478–482. [PUBMED], [INFOTRIEVE]
- Kleinman M. T., Bufalino C., Rasmussen R., Hyde D., Bhalla D. K., Mautz W. J.. 2000. Toxicity of chemical components of ambient fine particulate matter (PM 2.5) inhaled by aged rats. J. Appl. Toxicol.. 20(5)357–364. [CROSSREF], [PUBMED], [INFOTRIEVE]
- Lambert A. L., Trasti F. S., Mangum J. B., Everitt J. I.. 2003. Effect of preexposure to ultrafine carbon black on respiratory syncytial virus infection in mice. Toxicol. Sci.. 72(2)331–338. [CROSSREF], [PUBMED], [INFOTRIEVE]
- Leonardi G. S., Houthuijs D., Steerenberg P. A., Fletcher T., Armstrong B., Antova T., Lochman I., Lochmanova A., Rudnai P., Erdei E., Musial J., Jazwiec-Kanyion B., Niciu E. M., Durbaca S., Fabianova E., Koppova K., Lebret E., Brunekreef B., van, Loveren H.. 2000. Immune biomarkers in relation to exposure to particulate matter: A cross-sectional survey in 17 cities of Central Europe. Inhal. Toxicol.. 12(Suppl 4)1–14. [PUBMED], [INFOTRIEVE]
- Obot C. J., Morandi M. T., Beebe T. P., Hamilton R. F., Holian A.. 2002. Surface components of airborne particulate matter induce macrophage apoptosis through scavenger receptors. Toxicol. Appl. Pharmacol.. 184(2)98–106. [CROSSREF], [PUBMED], [INFOTRIEVE]
- Pozzi R., De, Berardis B., Paoletti L., Guastadisegni C.. 2003. Inflammatory mediators induced by coarse (PM2.5–10) and fine (PM2.5) urban air particles in RAW 264.7 cells. Toxicology. 183(1-3)243–254. [CROSSREF], [PUBMED], [INFOTRIEVE]
- Rudofsky U. H., Lawrence D. A.. 1999. New Zealand mixed mice: A genetic systemic lupus erythematosus model for assessing environmental effects. Environ. Health Perspect.. 107(Suppl 5)713–721. [PUBMED], [INFOTRIEVE]
- Salvi S., Blomberg A., Rudell B., Kelly F., Sandstrom T., Holgate S. T., Frew A.. 1999. Acute inflammatory responses in the airways and peripheral blood after short-term exposure to diesel exhaust in healthy human volunteers. Am. J. Respir. Crit. Care Med.. 159(3)702–709. [PUBMED], [INFOTRIEVE]
- Schwartz J., Neas L. M.. 2000. Fine particles are more strongly associated than coarse particles with acute respiratory health effects in schoolchildren. Epidemiology. 11(1)6–10. [CROSSREF], [PUBMED], [INFOTRIEVE]
- van, Zijverden M., de, Haar C., van, Beelen A., van, Loveren H., Penninks A, Pieters R.. 2001. Coadministration of antigen and particles optimally stimulates the immune response in an intranasal administration model in mice. Toxicol. Appl. Pharmacol.. 177(3)174–178. [PUBMED], [INFOTRIEVE]