Abstract
Toxicological research for humans requires model animals that are physiologically and/or developmentally closely related to one another. The development of the immune system (IS) in the cynomolgus monkey (Macaca fascicularis) was assessed and compared with selected data of humans and mice with the aim of contributing arguments to the discussion of the most appropriate animal model in toxicological research. Details of the developing IS in the cynomolgus monkey from embryonic day 35 to birth are investigated utilizing cluster development (CD) antibodies. Early immunoreactivity with CD 68, CD 117, and HLA-DR antibody is apparent from days 40 and 45 onward. All principal cell lines—T-, B-, and NK-cells—are present on day 100 in both thymus and peripheral organs. We discuss investigations of the cynomolgus monkey IS development with the reported development in humans and mice and stress 4 topics of significant interspecies differences to be considered in the decision for the appropriate animal model in a toxicological study.
INTRODUCTION
Referring to “the development of the IS” means dealing with a variety of organs. Among them are transiently active embryo-fetal organs (intra- and extra-embryonic hemopoetic islets, yolk sac, placenta, liver), and the permanent key immune organs with primarily immune functions (thymus, lymph node), as well as multi functional organs with partial immune functions (spleen, bone marrow, skin, etc.). The classical immune functions are attributed to a considerable number of cell types and their various subtypes that are identified by a large number of specific molecules, receptors, or their activated synthetic machinery. In conclusion, the IS represents a highly complex system, not attributable to a restricted number of well defined organs but rather defined by its function: “the defence of toxic agents” (Mestas and Hughes, Citation2004).
In terms of development, the IS displays a “lifelong renewing system.” This means that studying the adult animal can provide access to the generative miracles and partly allows conclusions pertaining to the generative processes in fetal life. This is why the standard lymphopoetic schemes mostly highlight results gained from adult and not from embryonic animals. This becomes obvious to the reader when “bone marrow” is addressed as a source of premature immune cells, which does not actually appear prior to week 8–9 (see later). But do the adult generative mechanisms really completely mimic those observed in the premature embryogenetic phase? Focussing on this question the development of the IS in the cynomolgus monkey was studied and compared with selected developmental data from humans and mice.
Knowledge about the development of the immune system has predominantly been collected from rodents (Haley, Citation2003; Holsapple et al., Citation2003). However, in toxicology the main target of research is the human being and the discussion of suitability of the rodent animal model versus the necessity of a non-human primate (NHP) model needs to be conducted (Olson et al., Citation2000; Hendrickx et al., Citation2002; Makori et al., Citation2003). This article presents findings on the developing IS in the cynomolgus monkey as a contribution to this discussion.
METHODS
All procedures are in conformity with the requirements of the Animal and Welfare Act. The animals studied originated from female cynomolgus monkeys (Macaca fascicularis) that were imported from a Chinese breeding station. The present data were collected from 28 animals over a period of 15 years. Animals and organs investigated and reported were aged 35, 40, 45, 50, 60, 70, 85, 100, 130, or 150 days after gestation (gestational day 35; gd 35) (Buse et al., Citation2005). The organs studied were collected from 1 to 5 animals per age group. Embryos/fetuses were not selected by sex.
For studying developmental parameters of the immune system (IS), whole embryons or selected immune organs were fixed in formalin, embedded in paraffin wax, and microtomed in 7 μm slices. They were then histologically stained with either hematoxilin and eosin or trichrome according to Goldner as well as immunocytochemical stains using the antibodies listed in . All antibodies applied were tested for their reactivity with paraffin embedded cynomolgus monkey tissues.
TABLE 1 Antibodies applied to prenatal immune organs of cynomolgus monkeys
RESULTS
Key Spots in Embryo-Fetal Development of Immune Organs
Organogenesis in humans and non-human primates (NHP) are vastly similar particularly in the early developmental phase, whereas organ development in mice is considered to be relatively retarded (). Such view, however, refers to the hallmark of birth instead of the endpoints of functional development. Intra-uterine development lasts only 20 days in mice, compared with 165 intra-uterine days in cynomolgus monkeys and 267 days in humans. This, however, considerably impacts toxicological research, dealing in the case of mice with partly pre-natal and partly post-natal immune organ development and maturation, whereas in the case of humans and cynomolgus monkeys only with prenatal development. Here it may be mentioned that, in humans, the very maturation of the IS is considered to extend over the first decade of life.
FIG. 1. Selected landmarks in the immune system development in humans, Cynomolgus monkeys, and mice: The IS in primates develops intrauterine to the functional stage (even though the final maturity in humans is reached not before the first decade), whereas development in mice to the comparable functional state is mostly extra-uterine due to the short gestational time. Minor time deviations in organ development as deduced from literature do not substantially disturb this view. Color gradations refer to pregnancy—trimenons; light: first trimenon; middle: second trimenon; dark: third trimenon.
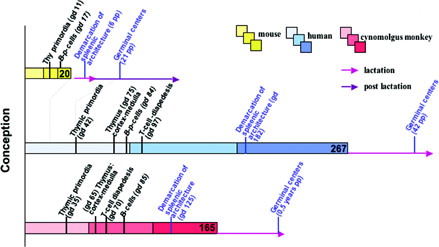
On gd 35, the developmental advance in the cynomolgus monkey grossly correlates with day 35 in humans and day 9 in mice. Hemopoesis is obvious from the presence of blood cells in heart and cardinal vessels as well as hematopoetic islets in the liver. The thymus bud is the first primary immune organ visible in a cranial medio-sagittal position (Buse et al., Citation2005).
On gd 40, lymphopoetic stem cells may be seen in the liver by means of CD117 immunoreactivity (IR), (), and blood macrophages by means of CD 68. On day 45 HLA-DR immune-positive cells are first seen among primordial lymphoid tissue at the level of the cisterna chyli ().
FIG. 2. (a) liver, gd 40, immunoreactive for CD117, indicative for HSC; (b) horizontal section through the cisterna chyli area with lymphatic parenchymia, focally reacting with HLA-DR antibody (arrows); (c) thymus on gd60 stained with HLA-DR antibody; there is a clear stain of the medullary area; the thymus is immunopositive for CD3 and immuno-negative for CD2, CD4, and CD8; inset: mesenchymal cells close to the thymus at day 50; (d) thymus on gd70 with medullary localized CD3 immunopositive cells (T-cells?); (e) thymus on gd70 with medullary localized HLA-DR immunopositive cells, corresponding to CD3+ distribution; (f) lymph node primordium on gd70 with an area of CD3 immuno-positive cells (T-cells?); (g) lymph node primordium on gd70 with few HLA-DR immunopositive cells.
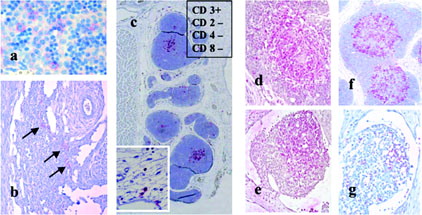
On gd 50, HLA-DR positive cells are present in liver, lymph nodes, lung, thymus, and thymus surrounding mesenchyme (). This clearly indicates early differentiation/-commitment of the prospective antigen presenting cells towards their premature commitment of nursing and of lymphoid T-cell selection and maturation (Wierzba-Bobrowicz et al., Citation2001). In the thymus as the major organ of premature T-cell commitment, distinct compartments are clearly characterized by medullary HLA-DR expression and cortex non-expressing cells. On day 50 the presence of the primordia of all organs furthermore marks the end of the embryonic period. Regarding the IS such is obvious by lymph node primordia in various body positions as well as bone cavities starting to open for invasion of bone marrow cell components (first indication in clavicle; Buse et al., Citation2003).
On gd 60, there is a first thymic appearance of CD3+-cells (T-cells), whereas the CD2 pan T-cell marker was not observed, and thus not in full agreement with literature. On day 60, the nursing function of thymic HLA-DR+-cells is in local agreement with the medullary CD3 T-cell expression, confirming the close interaction of both cell types (, , and ). On gd 70, CD3-positive cells were also discovered in the peripheral lymphatic organs such as lymph nodes in various body locations.
From gd 85 onward, the majority of the IS characterizing cells became faintly visible by use of various antibodies, and on day 100 were clearly seen in most organs (). In particular all of the expected principal cell lines–B-cells, NK cells and T-cells–were observed in the body periphery as well as in the thymus. Whereas T-helper cells (CD4+) were detected, the cytotoxic T-cells (CD8+) were not observed before birth.
TABLE 2 Immunoreactivities detected in the immune organs of gd 100 fetuses
B-cells (CD20+, initial immunoreactivity) on day 85 led to the question about B-cell activation, i.e., transformation into plasma cells and Ig synthesis. Plasma cells were investigated by means of CD138 IR. CD138 IR was observed on day 100 with prevalence in the lymph nodes. Their product, the IgM was consistently discovered from day 100 to birth at a serum level of 0.3–1.2 g/L (). This is in good agreement with IgM in fetal human serum shortly after appearance of the first B-cells and with data reported in the rhesus monkey (Makori et al., Citation2003). In the same time period (day 100 to birth) the IgG level had increased from 3 to 9 g/L. However, IgG is attributed to the maternal serum protein well known to be permeable through the placental barrier (Fujimoto et al., Citation1983). Concluding from the cellular and humoral basis, the IS was largely complete on day 100 and ready for further maturation and fine-tuning.
FIG. 3. (a) IgG measured from gd 92 to the 1-year-old animal; the pre-birth IgG is suspected to derive from the mother animals by transplacental permeation. Dotted lines give the IgG levels as suspected maternal vs. intrinsic origin; (b) IgM measured from gd 92 to the 1-year-old animal; the pre-birth IgM is suspected to derive from the fetus as it is not placenta permeable. Pre-natal data are collected from 8 individuals; post-natal data are won by repeated collection from 38 individuals.
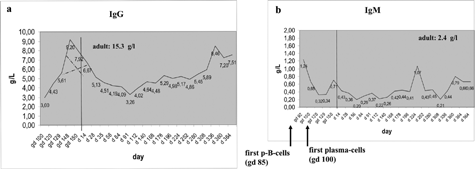
DISCUSSION
The data given elucidate the development of the cynomolgus monkey IS using humanized antibodies. The antibody reactivity in primates and their reactivity in humans clearly indicate the close relationship of both species, human (von Gaudecker, Citation1991) and, in addition, of further the rhesus monkey (Makori et al., Citation2003). Upon comparison with the developing rodent IS, four key topics have been identified to support decision making about species suitability for toxicological studies. The “yes” or “no” decision substantially depends on the “right question to the right system” and may principally include both primates and rodents. A “close relationship” does not necessarily imply the unique criterium for deciding on the first choice model.
TOPIC 1
Much basic and relevant knowledge for the human IS could be extracted from rodents, clearly underlining the appropriateness of rodents for basic research generally and for research in human toxicological specificially. The toxicologist can refer to extensive research with these animals.
TOPIC 2
The morphologic structure and the cellular composition of the IS in all three species: human, cynomolgus monkey, and mouse, is principally similar, and substantial differences have not been described. This is a further argument of the appropriateness of rodents in human toxicological research.
TOPIC 3
The intra-uterine versus extra-uterine development and maturation mark a serious contrast between humans and cynomolgus monkeys on the one hand and rodents on the other hand. The exclusively intra-uterine development to the neonatal ready-for-action-state of the IS at birth in humans and cynomolgus monkeys is in striking contrast to the highly immature IS in neonate mice/rodents. In the latter birth is followed by a 3-week extra-uterine weanling period until the ready-for-action state is reached. However, this fundamentally impairs toxicological research.
Human targeted compounds tested in cynomolgus monkeys reach and affect the developing IS after filtration through the placental blood-blood barrier (depending on its specific permeability). Such, however, can occur in the mouse only during the intrauterine phase of about day 10–20 (from barrier formation and fetal immune organ generation to birth. By day 20, however, the IS might only have passed half of its basic developmental advance, i.e., just expressing first T- and B-cells (Holsapple et al., Citation2003). As a consequence, thymectomy in the highly incomplete state of neonate rodents results in a life long irreversible lymphoid atrophy and severe immune defects (Barnett, Citation1996), whereas thymectomy in neonate humans (and presumably cynomolgus monkeys) has few medical effects and remains relatively inapparent during the entire life span. Well accepted thymectomy in humans has been concluded to derive from a well advanced IS with competent T- and B-cell populations already being both established in their peripheral destinations and ready for proliferation.
In the postnatal mouse the compounds have to be supplied via lactation and the gastrointestinal passage. However, it remains to be discussed whether or not transplacentar permeation and lactation leads to materno-fetal compound transitions with similar metabolisms, target organ access, and final compound levels. Rather, physiological differences in both rodents and primates must be considered pre- vs. postnatal: veneous/trans-placentar or oral application, fundamentally different kidney functions (clearance) and pulmonary O2-supply, haem exchange, or completely different germ spectra to which the IS becomes postnatal exposed.
TOPIC 4
Further relevant interspecies differences correlate with the so called “phylogenetic gap,” which amounts to 75 million years between human and mice, whereas it is only 25 million years between the human and cynomolgus monkey (Mestas and Hughes, Citation2004). It is well accepted that there exists a linear correlation of molecular interspecies changes and the timely distance to the points of phylogenetic radiation. Interspecies molecular differences are to be expected to be significant in mice and of lesser significance in monkeys; this has been confirmed in great detail by Mestas and Hughes (Citation2004). Upon demonstrating large molecular differences human vs. mice and minor differences human vs. primate; they clearly impact toxicological decision making. The molecular differences show that the similar goals of all ISs, namely the “defence of toxicological agents” as well as their general success, does not necessarily implicate the use of identical molecular trails to achieve the goals.
Are All Immune Systems the Same?
The development of the IS has been previously subdivided into five periods of vulnerability, the so called “windows of vulnerability” (Dietert et al., Citation2000; Landreth, Citation2002), which proved to be especially helpful tools in categorising IS development and illuminating species suitability in toxicological studies. Utilizing the five periods, mouse and cynomolgus monkey IS have been assessed with regard to the human target () (von Gaudecker, Citation1991; Neubert et al., Citation1996; Hirose et al., Citation2002; Robertson and Poznansky, 2002; Bomhardt et al., Citation2004).
TABLE 3 Windows of vulnerability in the developing immune system correlated in humans, cynomolgus monkeys, and mice
In conclusion, there is good evidence for human and rodent homologies. This should be especially relevant in the advanced IS research such as on adult rodents, and is underlined by further advantages of the small animals, i.e., fast generation cycles, easy housing, and fewer ethical problems. Based on the long history of relevant rodent studies their value, even under developmental aspects, is by no means questionable. However, drug effectiveness mechanisms are broken down to the molecular effect level that requires more detailed knowledge of the targets. With regard to early development, a number of serious arguments as to the appropriateness of rodents as animal models have to be considered pre-study.
In contrast, such considerations are easier to answer in case of macaques that closely meet the requirements of toxicological research in both molecular and developmental aspects. Such includes both meaningful macaque species, the rhesus monkey (Makori et al., Citation2003), and the cynomolgus monkeys, which in most cases certainly represent appropriate animal models in terms of the developing IS in human targeted toxicology.
REFERENCES
- Barnett J. B. Developmental immunotoxicology. Experimental Immunotoxicology, R. J. Smialowicz, M. P. Holsapple. CRC Press, Boca Raton, FL 1996; 47–62
- Bomhardt U., Beyer M., Hünig T., Reichardt H. M. Molecular and cellular mechanisms of T-cell development. Cell. Mol. Life Sci. 2004; 61: 263–280, [CSA]
- Buse E., Habermann G., Vogel F. Thymus development in macaca fascicularis (cynomolgus monkey): An approach for toxicology and embryology. J. Mol. Histol. 2005, (In press)[CSA]
- Buse E., Habermann G., Osterburg I., Korte R., Weinbauer G. Reproductive/ developmental toxicity and immunotoxicity assessment in the nonhuman primate model. Toxicology 2003; 221: 221–227, [CSA], [CROSSREF]
- Dietert R. R., Etzel R. A., Chen D., Halonen M., Holladay S. D., Jarabek A. M., Landreth K., Peden D. B., Pinkerton K., Smialowicz R. J., Zoetis T. Workshop to identify critical windows of exposure for children's health: Immune and Respiratory Systems Work Group summary. Environ. Health Perspect. 2000; 108(Suppl)483–490, [PUBMED], [INFOTRIEVE], [CSA]
- Fujimoto K., Terao K., Cho F., Honjo S. The placental transfer of IgG in the cynomolgus monkey. Japan. J. Med. Sci. Biol. 1983; 36: 171–176, [CSA]
- Haley P J. Species differences in the structure and function of the immune system. Toxicology 2003; 188: 49–71, [PUBMED], [INFOTRIEVE], [CSA], [CROSSREF]
- Hendrickx A. G., Makori N., Peterson P. The nonhuman primate as a model of developmental immunotoxicity. Human Exp. Toxicol. 2002; 21: 537–542, [CSA], [CROSSREF]
- Hirose J., Kouro T., Igarashi H., Yokota T., Sakaguchi N., Kincade P. W. A developing picture of lymphopoiesis in bone marrow. Immunol. Rev. 2002; 189: 28–40, [PUBMED], [INFOTRIEVE], [CSA], [CROSSREF]
- Holsapple M., West L. J., Landreth K. S. Species comparison of anatomical and functional immune system development. Birth Defects Res. (Part B) 2003; 68: 321–334, [CSA], [CROSSREF]
- Landreth K. S. Critical windows in development of the rodent immune system. Human Exp. Toxicol. 2002; 21: 493–498, [CSA], [CROSSREF]
- Makori N., Tarantal A. F., Lü F. X., Rourke T., Marthas M. L., McChesney M. B., Hendrickx A. G., Miller C. J. Functional and morphological development of lymphoid tissues and immune regulatory and effector function in Rhesus monkeys: Cytokine-secreting cells, immunoglobulin-secreting cells, and CD5+ B-1 cells appear early in fetal development. Clin. Diag. Lab. Immunol. 2003; 10: 140–153, [CSA], [CROSSREF]
- Mestas J., Hughes C. C. W. Of mice and not men: Differences between mouse and human immunology. J. Immunol. 2004; 224: 2731–2738, [CSA]
- Neubert R., Helge H., Neubert D. Nonhuman primates as models for evaluating substance-induced changes in the immune system with relevance for man. Experimental Immunotoxicology, R. J. Smialowicz, M. P. Holsapple. CRC Press, Boca Raton, FL 1996; 63–97
- Olson H., Betton G., Robinson D., Thomas K., Moro A., Kolaja G., Lilly P., Sanders J., Sipes G., Bracken W., Dorato M., Van Deun K., Smith P., Berger B., Heller A. Concordance of the toxicity of pharmaceuticals in humans and in animals. Regul. Toxicol. Pharmacol. 2000; 32: 56–67, [PUBMED], [INFOTRIEVE], [CSA], [CROSSREF]
- Robertson P., Poznansky M. C. T-lymphocyte development and models of thymopoetic reconstitution. Transplant Infect. Dis, 2003; 5: 38–42, [CSA]
- von Gaudecker B. Functional histology of the human thymus. Anat. Embryol. 1991; 183: 1–15, [PUBMED], [INFOTRIEVE], [CSA]
- Wierzba-Bobrowicz T., Kosno-Kruszewska E., Lewandowska E., Lechowicz W., Schmidt-Sidor B. Major histocompatibility complex class II (MHC II) expression during development of human fetal brain and haemopoietic organs. Basic and Clinical Immunology, A. Mackiewicz, M. Kurpisz, J. Zeromski. Kluwer Academic/Plenum Publishers, New York 2001