Abstract
Stem cell factor (SCF) is not only critical for mast cell development, but also an important mast cell functional regulator. However, roles of transcription factors involved in SCF-induced effects remain incompletely defined. Early growth response factor-1 (Egr-1) is a member of zinc-finger transcription factor family. Mouse bone marrow-derived mast cells (BMMC) were used to examine a role of Egr-1 in SCF-induced mast cell activation and growth. SCF induced a strong and rapid expression of Egr-1 mRNA as tested by real-time PCR analysis. SCF-induced Egr-1 nuclear translocation and DNA binding were demonstrated by electrophoretic mobility shift assay (EMSA) and immunofluorescence assay. To examine if Egr-1 is required for SCF-induced IL-13 expression, Egr-1-deficient BMMC were used. Levels of SCF-induced IL-13 mRNA and protein were reduced in Egr-1 deficient BMMC when compared with wild-type BMMC. Although Egr-1 is required for macrophage and lymphocyte development, SCF-induced mast cells growth was not affected by Egr-1 deficiency. Interestingly, SCF-induced Egr activation was blocked by a tyrosine kinase inhibitor PP2, suggesting a role of tyrosine phosphorylation in SCF-induced Egr-1 activation. Taken together, our results suggest that Egr-1 is required for SCF-induced IL-13 expression, but not mast cell growth.
Keywords :
INTRODUCTION
Mast cells originate from pluripotential progenitor cells in bone marrow and acquire mature phenotype in tissues through migration and differentiation (Metcalfe et al., Citation1997). They play a central role in allergic inflammation. Mast cells are characterized by expression of high affinity IgE receptor (Fc∑RI) and the stem cell factor (SCF) receptor, c-Kit (CD117) (Metcalfe et al., Citation1997). SCF is critical for the development, survival and proliferation of mast cells (Galli et al., Citation1994. Binding of SCF to its receptor c-Kit initiates a cascade of signaling events, leading to activation of Src kinase, phosphatidyl-inositol-3 (PI3)-kinase, phospholipase C (PLC)-γ, mitogen activated protein (MAP) kinase pathways and many others (Ronnstrand, Citation2004; Roskoski, Citation2005). It appears that each SCF-induced signaling pathway is associated with specific biological functions. For example, SCF-induced activation of the Src family of tyrosine kinases has been associated with gene transcription (Lennartsson et al., Citation1999; Bondzi et al., Citation2000) and mast cell chemotaxis (O'Laughlin-Bunner et al., Citation2001; Ronnstrand, Citation2004; Roskoski, Citation2005). SCF-induced PI3-kinase is involved in mast cell development (Fukao et al., Citation2002; Ronnstrand, Citation2004; Roskoski, Citation2005).
Mast cells are major effector cells in allergic inflammation through secretion of potent inflammatory mediators. Mast cell-derived mediators include the preformed mediators such as histamine, lipid mediators such as leukotrienes and various cytokines and chemokines, such as interleukin (IL)-13 (Metcalfe et al., Citation1997; Kinet, Citation1999). Although mast cells are able to produce all three categories of mediators, the amount, kinetic and type of particular mediators secreted are dependent upon the nature of individual stimuli (Galli et al., Citation2005). In vitro, SCF has been shown to regulate cytokine IL-4, tumor necrosis factor-α (TNF), or IL-13 production (Columbo et al., Citation1992; Coleman et al., Citation1993; Takaishi et al., Citation1994; Taylor et al., Citation1995; Gagari et al., Citation1997, Gibbs et al., Citation1997, Kobayashi et al., Citation1998, Garrington et al., Citation2000). In vivo, subcutaneous injection of recombinant SCF in human subjects induces mast cell hyperplasia and mast cell activation, as evidenced by a wheal-and-flare reaction at the injection site, electron microscopic findings of mast cell degranulation, and increased levels of mast cell tryptase and histamine metabolites (Costa et al., Citation1996). Transcription factors involved in SCF-induced mast cell functional regulation and growth remain incompletely defined.
Egr-1, a zinc finger transcription factor, is the prototype of the Egr family that includes Egr-1, Egr-2, Egr-3, Egr-4 and the Wilm's tumor product (Gashler and Sukhatme, Citation1995; Beckmann and Wilce, Citation1997). Members of Egr family have been associated in a large number of biological effects including cell growth and gene expression (Gashler and Sukhatme, Citation1995; Beckmann and Wilce, Citation1997). Importantly, the biological effects of Egr-1 appear to be cell type specific (Nguyen et al., Citation1993; Gashler and Sukhatme, Citation1995; Beckmann and Wilce, Citation1997; Faour et al., Citation2005). Depending upon the cell types, Egr-1 can either activate or inhibit gene expression or cell differentiation likely through interaction with other transcription factors or tissue specific factors (Nguyen et al., Citation1993; Gashler and Sukhatme, Citation1995; Beckmann and Wilce, Citation1997; Faour et al., Citation2005).
We used mast cells to examine a role of Egr-1 in SCF-induced IL-13 expression and mast cell growth. In this study, we demonstrated that SCF stimulated a rapid expression of Egr-1 mRNA and protein. SCF-induced IL-13 production at mRNA and protein levels is reduced in Egr-1-deficient mast cells suggesting that Egr-1 is required for SCF-induced mast cell activation. Inhibition of Src family kinases, but not PI3 kinase, protein kinase C (PKC), or MAP kinase blocked SCF-induced Egr-1 activation, suggesting a role for Src kinases in SCF-induced Egr-1 activation. Interestingly, SCF induced mast cell growth was not affected in Egr-1-deficient mast cells. Taken together, these results suggest that Egr-1 is involved in SCF-induced cytokine IL-13 production, but not mast cell growth.
MATERIALS AND METHODS
Antibodies and Reagents
Antibodies to Egr-1 (sc-189), Egr-2 (sc-20690), Egr-3 (sc-22801X), and Egr-4 (sc-19868X) were purchased from Santa Cruz Biotechnology (Santa Cruz, CA). Alexa 594–conjugated goat anti–rabbit IgG F(ab′)2 fragment was purchased from Molecular Probes (Eugene, OR). Fluorescein isothiocyanate (FITC) anti-mouse CD117 monoclonal antibody (mAb) (CL8936F), FITC rat IgG2a (CLCR2A01) were purchased from Cedarlane Laboratories Limited (Ontario, Canada). Recombinant murine stem cell factor (SCF, catalog no. 250-03) was purchased from PeproTech Incorporation (Rocky Hill, NJ).
Mast-Cell Culture and Stimulation
Egr-1–deficient mice and control C57BL/6NTac mice were purchased from Taconic Farms (Germantown, NY). The protocols were approved by the University Committee on Laboratory Animals, Dalhousie University, in accordance with the guidelines of the Canadian Council on Animal Care. Mouse bone marrow–derived mast cells (BMMC) were cultured as previously described (Li et al., Citation2006). After 4–5 wk of culture, mast-cell purity of greater than 98% was achieved as determined by toluidine blue staining (pH 1.0) of fixed cytocentrifuged preparations. BMMC (1× 106 cells/ml) were stimulated by addition of various concentrations of SCF (0.5 - 100 ng/ml) for various times (5 min – 24 hr). In the case of IgE+antigen stimulation, BMMC were sensitized with IgE directed against trinitrophenyl (TNP) as previously described (Li et al., Citation2006). TNP-bovine serum albumin (BSA; Biosearch Technologies, Inc., Novato, CA) was used as an antigen.
Preparation of Nuclear Proteins and Electrophoretic Mobility Shift Assay (EMSA)
Nuclear protein extracts were prepared by using a nuclear extract kit (catalog no. 40010; Active Motif, Carlsbad, CA), according to the manufacturer's protocol. All preparation procedures were carried out at 4°C. Total protein concentration was determined using the Bio-Rad protein assay (Bio-Rad Laboratories, Hercules, CA). EMSA was carried out as previously described (Li et al., Citation2006). The following double-stranded oligonucleotides were used: wild-type Egr-1 probe, 5′-GGA TCC AGC GGG GGC GAG CGG GGG CGA ACG-3′ (catalog no. 1200011; Geneka Biotechnology, Montreal, QC, Canada); mutant Egr-1 probe, 5′-GGA TCC AGC GGG GTA GAG CGG GTA CGA ACG-3′ (catalog no. 1400011; Geneka Biotechnology). Probe-labeling was accomplished by treatment with T4 poly kinase (catalog no. M410A; Promega, Madison, WI) in the presence of [32P]-adenosine 5′-triphosphate (catalog no. PB10218; Amersham Pharmacia Biotech, Piscataway, NJ). Labeled oligonucleotides were purified using a MicroSpin G-25 column (catalog no. 275325; Amersham).
Nuclear protein (8 μ g) was added to a total volume of 10 μ L binding reaction with 1 μ l (0.75 μ g/μ l) poly (dI-dC) (catalog no. 27-7880-02; Amersham) and incubated at room temperature for 15 minutes. Labeled oligonucleotides were added to each reaction mixture and incubated at room temperature for 30 minutes. Subsequently, samples were separated by electrophoresis on a 6% polyacrylamide gel in 0.5X Tris-boric acids–ethylenediaminetetraacetic acid (EDTA) buffer at room temperature for 1 hr at 300 V. Gels were vacuum-dried and subjected to autoradiography overnight at −80°C.
In some experiments, 1 μ l non-radiolabeled wild-type Egr-1, mutant Egr-1, Sp1 or Ap-1 oligonucleotides (50-fold excess of radiolabeled probe) were added and incubated for 15 min before addition of the radiolabeled probe (competition assay). For assays using antibodies to block the DNA–protein complex formation, nuclear extracts were incubated in a total volume of 20 μ l with 6 μ l appropriate polyclonal antibodies to Egr-1 (sc-189X), Egr-2 (sc-190X), Egr-3 (sc-22 801X), or Egr-4 (sc-19868X), respectively, for 2 hr on ice before the addition of the radiolabeled probe.
Real-Time Quantitative PCR
TRIZOL Reagent (catalog no. 15596-026; Invitrogen, Carlsbad, CA) was used to isolate total RNA from BMMC. Reverse transcription was carried out using SuperScript II RNase H-Reverse Transcriptase (catalog no. 18064-014; Invitrogen) according to the manufacturer's instruction. Real-time quantitative polymerase chain reaction (PCR) was performed using a 7000 Sequence detector (PE Applied Biosystems, Foster City, CA).
Specific quantitative assays for Egr-1 and IL-13 were performed using Assays-on-Demand reagents containing 6-FAM dye-labeled TaqMan minor groove binding (MGB) probes (Applied Biosystems). Glyceraldehyde-3-phosphate dehydrogenase (GAPDH) was used as an endogenous reference. Data were analyzed using relative standard curve method according to the manufacturer's protocol. An average value of each gene after GAPDH normalization at the timepoint showing highest expression was used as a calibrator to determine the relative levels of Egr-1, or IL-13 at different conditions as previously described (Li et al., Citation2006).
Fluorescence-Activated Cell Sorting (FACS) Analysis
To examine c-kit expression, BMMCs were stained with a FITC-conjugated rat anti–mouse CD117 (c-kit) mAb (IgG2a) for 30 min at 4°C. FITC-rat IgG2a was used as an isotypic control. Cells were analyzed by a FACScaliber flow cytometer (BD Biosciences). Fluorescence-labeled mast cells also were cytocentrifuged (Cytospin 3, Shandon, United Kingdom) onto slides at 4.5 × g (200 rpm) for 5 min. Slides were mounted with DAPI, a fluorescent groove-binding probe for DNA, in order to visualize cell nuclei. Cells were examined using a fluorescence microscope (Nikon Eclipse E600; Nikon, Tokyo, Japan). To examine FcεRI expression, BMMC were sensitized with IgE and then stained with FITC-conjugated rat anti-mouse IgE mAb (BD Biosciences) for 1 hr at 4°C. FITC-rat IgG1 was used as a control.
Cytokine IL-13 Assay
The level of IL-13 was measured by enzyme-linked immunosorbent assay (ELISA) using Quantikine Mouse IL-13 Immunoassay (for IL-13) from R&D Systems (Minneapolis, MN) according to the manufacturer's protocol.
RESULTS
Mouse BMMCs Express Egr-1 in Response to SCF Stimulation
Mouse BMMCs were stimulated with SCF at the concentration of 50 ng/ml for 15, 30, 60, or 180 min. Quantitative real-time PCR was used to determine Egr-1 expression. Egr-1 level was normalized to GAPDH in each sample. An average value of Egr-1 product after GAPDH normalization at the time point of 15 min (the highest Egr expression level) was used as a calibrator to determine the relative level of Egr-1 expression at various conditions (). Treatment of BMMC with SCF induced a rapid and transient expression of Egr-1 (, ). We also examined expression of Egr-2, Egr-3, Egr-4 and the Wilm's tumor product. A significant amount of Egr-2 expression was detected after SCF-stimulation, while little or no Egr-3, Egr-4 or the Wilm's tumor product can be found (data not shown). PCR products were also separated in agarose gels and visualized by ethidium bromide staining. Representative gels are presented in . A rapid and strong Egr-1 expression can be seen at 15 and 30 min after SCF treatment. Egr-1 and Egr-2 levels in the un-stimulated mast cells were undetectable.
FIG. 1 Mast cells express Egr-1 in response to SCF stimulation. Mouse bone marrow–derived mast cells (BMMCs) were stimulated with stem cell factor (SCF, 50 ng/ml) for 15, 30, 60, or 180 min. RNA isolated from these cells was reverse transcribed into cDNA and analyzed for Egr-1 expression by real-time quantitative PCR. GAPDH was used as an internal control. Egr-1 expression was normalized to endogenous control GAPDH. The data are expressed as relative mRNA levels compared with the average expression level in BMMC treated with SCF for 15 min (= 1) since at this time point Egr showed highest expression level (A). The PCR products were separated by 2% agarose gel and stained with ethidium bromide (B). Untreated BMMCs (time 0) showed no Egr-1 expression, whereas SCF significantly induced Egr-1 expression. Error bars represent standard errors from three independent experiments.
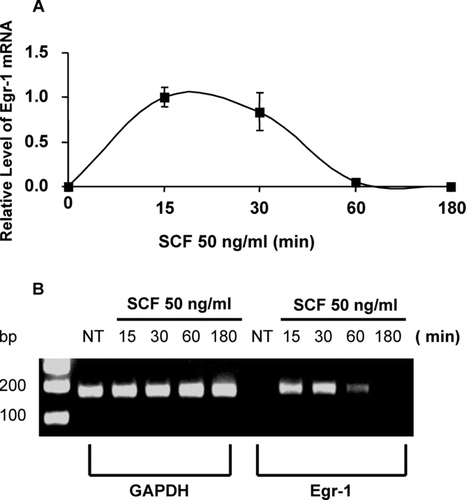
Expression of Egr-1 protein was determined by immunofluorescence assay. BMMC after treatment with SCF (100 ng/ml) for 2 hr were fixed, permeabilized and stained with anti-Egr-1 antibody (Ab). Alexa594-conjugated secondary Ab was used to visualize Egr-1 expression. Nuclei were stained by DAPI. SCF induced Egr-1 protein was found in the nucleus of the BMMC ().
FIG. 2 SCF-induced Egr-1 expression as determined by immunofluorescence staining. Mouse BMMCs from C57/BL6 mice were stimulated with SCF (100 ng/mL) for 2 hr or left untreated (no treatment). Cells were fixed, permeabilized, and then stained with anti–Egr-1 Ab or control rabbit serum. Alexa 594-conjugated goat anti–rabbit IgG F(ab′)2 was used as a secondary Ab. DAPI staining was carried out to visualize the nucleus of the cells. SCF stimulation induced expression of Egr-1, which is localized in the nucleus of the cells. Original magnification, × 40. Immunolabeled specimens were mounted in DAPI containing Vectashield (Vector Laboratories, Burlingame, CA). Cells were examined using a fluorescence microscope (Nikon E600; Nikon, Tokyo, Japan) equipped with a DMX1200 camera and a CFI Plan-Fluor DDL 40 × /0.75 objective lens. Images were processed using Adobe Photoshop 5.0 (Adobe Systems, San Jose, CA).
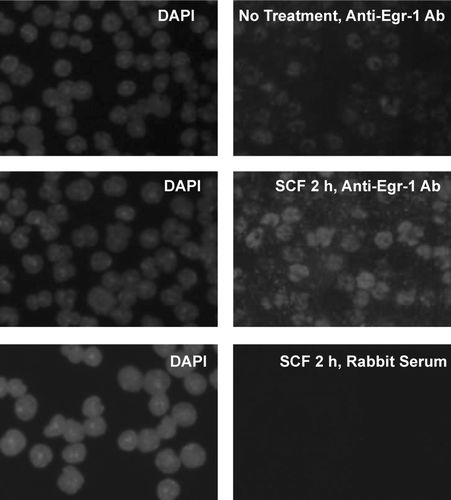
SCF-Stimulation Induces Egr Activation in Mouse BMMC
We determined if SCF stimulation induces Egr-1 activation using EMSA. Nucleus proteins from SCF-treated BMMC were isolated and subjected to analysis by EMSA. An Egr DNA probe which can bind Egr products (Egr-1, -2, -3, and -4) was used. Mast cells responded to SCF even at the very low concentration (0.5 ng/ml). A strong Egr binding was observed when BMMC were stimulated with 5 ng/ml of SCF (), a concentration close to the physiological SCF level of 3.3 ng/ml in human serum (Langley et al., Citation1993). Strong Egr binding was observed when BMMC were stimulated with SCF at the concentrations of 25–100 ng/ml (). Time course experiment showed treatment of BMMC with SCF (50 ng/ml) for 40, 60, and 120 min induced significant Egr DNA binding. Egr DNA binding activity declined after 3–6 hr ().
FIG. 3 Egr-1, but not Sp-1, is activated in mast cells following SCF stimulation. Egr-1 or Sp-1 activation was carried out using electrophoresis mobility shift assay (EMSA). Gels were analyzed by densitometry. (A) Mouse BMMCs were stimulated with SCF for 2 hr at the concentration of 0.5, 5, 25, 50, 100 ng/ml. (B) Mouse BMMCs were stimulated with SCF (50 ng/ml) for 5, 20, 40, 60, 120, 180, or 360 min. Nuclear proteins were isolated and subjected to EMSA analysis (see “Materials and methods”) using [32P]-labeled Egr-1– or Sp-1–specific probes. Blank, no nuclear proteins were added; NT, no treatment, nuclear proteins were isolated from BMMCs without SCF stimulation. (C) Nuclear proteins from SCF (50 ng/ml, 120 min) treated BMMCs (SCF) or from untreated BMMCs (NT) were subjected to DNA probe competition experiment using unlabeled probes or mutant probe to demonstrate specific Egr-1 binding. Fifty times concentrated unlabeled Egr-1 oligonucleotide was used to compete with [32P]-labeled Egr-1 oligonucleotide, whereas 50X concentrated unlabeled mutant Egr-1 (mEgr-1) or SP-1 oligonucleotides were used as nonspecific control probes. Nuclear proteins from no treatment group or SCF-treated cells were analyzed for Sp-1 activation by using [32P]-labeled Sp-1 probe. No Sp-1 binding was observed in SCF-stimulated BMMCs. (D) Anti-Egr-1 or anti-Egr-2 antibody but not anti-Egr-3 or anti-Egr-4 antibody blocked the DNA-protein complex formation. Nuclear proteins from SCF (50 ng/ml, 2 hr) treated BMMCs or from untreated BMMCs (NT) were incubated with or without specific antibodies to Egr-1, Egr-2, Egr-3, or Egr-4 for 2 hr on ice before EMSA experiment using [32P]-labeled Egr-1 oligonucleotide.
![FIG. 3 Egr-1, but not Sp-1, is activated in mast cells following SCF stimulation. Egr-1 or Sp-1 activation was carried out using electrophoresis mobility shift assay (EMSA). Gels were analyzed by densitometry. (A) Mouse BMMCs were stimulated with SCF for 2 hr at the concentration of 0.5, 5, 25, 50, 100 ng/ml. (B) Mouse BMMCs were stimulated with SCF (50 ng/ml) for 5, 20, 40, 60, 120, 180, or 360 min. Nuclear proteins were isolated and subjected to EMSA analysis (see “Materials and methods”) using [32P]-labeled Egr-1– or Sp-1–specific probes. Blank, no nuclear proteins were added; NT, no treatment, nuclear proteins were isolated from BMMCs without SCF stimulation. (C) Nuclear proteins from SCF (50 ng/ml, 120 min) treated BMMCs (SCF) or from untreated BMMCs (NT) were subjected to DNA probe competition experiment using unlabeled probes or mutant probe to demonstrate specific Egr-1 binding. Fifty times concentrated unlabeled Egr-1 oligonucleotide was used to compete with [32P]-labeled Egr-1 oligonucleotide, whereas 50X concentrated unlabeled mutant Egr-1 (mEgr-1) or SP-1 oligonucleotides were used as nonspecific control probes. Nuclear proteins from no treatment group or SCF-treated cells were analyzed for Sp-1 activation by using [32P]-labeled Sp-1 probe. No Sp-1 binding was observed in SCF-stimulated BMMCs. (D) Anti-Egr-1 or anti-Egr-2 antibody but not anti-Egr-3 or anti-Egr-4 antibody blocked the DNA-protein complex formation. Nuclear proteins from SCF (50 ng/ml, 2 hr) treated BMMCs or from untreated BMMCs (NT) were incubated with or without specific antibodies to Egr-1, Egr-2, Egr-3, or Egr-4 for 2 hr on ice before EMSA experiment using [32P]-labeled Egr-1 oligonucleotide.](/cms/asset/178c5cc3-877c-4561-927a-567aaf2687be/iimt_a_313127_uf0003_b.gif)
To confirm Egr DNA binding specificity, a competitive binding assay was performed. Un-labeled Egr probe, but not mutant Egr probe or Sp-1 probe successfully blocked [32P]-labeled Egr-1 probe binding (). Since Sp-1 and Egr-1 have similarity in their DNA binding sequences, we examined if SCF induced Sp-1 DNA binding. No Sp-1 activation can be observed in SCF-stimulated mast cells ().
We further confirmed Egr binding specificity by using antibodies to Egr-1, -2, -3, and -4 in an EMSA supershift assay. As shown in , anti-Egr-1 or anti-Egr-2 antibodies reduced Egr DNA binding activity, while anti-Egr-3 or anti-Egr-4 antibodies had little effects.
Egr-1 Deficiency Has No Effect on SCF-Induced Mast Cell Growth
Given that SCF plays an essential role in mast cell development, we determined if SCF-induced Egr-1 expression is required for mast cell growth. Bone marrow cells from Egr-1–deficient mice and wild-type control mice were cultured in WEHI-3B conditioned medium (as a source for IL-3) to differentiate into mast cells. Interestingly, Egr-1–deficient BMMC showed similar levels of c-kit and IgE receptor expression when compared to wild-type BMMC () (Li et al., Citation2006).
FIG. 4 Egr-1 deficiency has no effects on c-Kit and IgE receptor expression or mast-cell viability. (A) Bone marrow cells from wild-type mice or Egr-1–deficient mice were cultured in conditioned media in vitro for 4 wk and examined by flow cytometry for c-Kit and IgE receptor expression. For c-Kit analysis, BMMCs were stained with FITC conjugated rat anti-mouse c-Kit mAb or FITC conjugated rat IgG2a isotypic control. For analysis of IgE receptor expression, BMMC were sensitized with IgE overnight and then stained with FITC-conjugated anti-IgE antibody (mouse IgG1). No difference in c-Kit or IgE receptor expression was observed between Egr-1+/+ and Egr-1−/− BMMCs. (B) BMMC from Egr-1-deficient mice or wild-type mice cultured in complete media containing WEHI-3B supernatants (a source of IL-3) were healthy and showed ≥ 96% viability. To induce mast cell death, WEHI-3B supernatant was removed from culture media for various days. Mast cell viability was examined by trypan blue exclusion assay. Data were expressed as mean ± SD (n = 3 independent experiments).
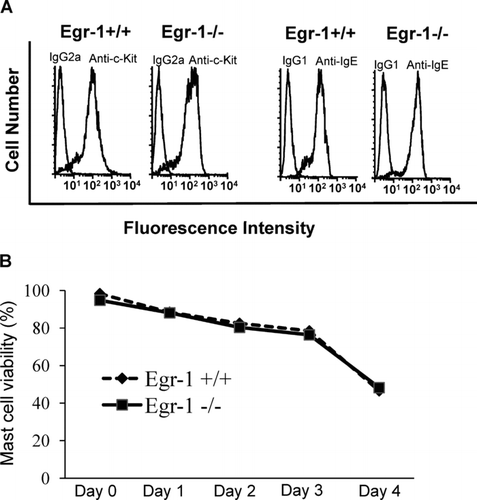
In addition, Egr-1–deficient BMMC and wild-type BMMC were labeled with carboxyfluorescein succinimidyl ester (CFSE) and treated with various concentrations of SCF (0.5, 5, 25, 50, or 100 ng/ml) for 5 d. Cells were then examined by flow cytometry. SCF induced a concentration dependent reduction of CFSE fluorescence intensity, suggesting cell proliferation. Egr-1 deficiency did not affect SCF-induced decrease of CFSE fluorescence intensity (mast cell proliferation) (data not shown). A similar trend of SCF-induced increase of mast cell numbers was observed in both Egr-1–deficient BMMC and wild-type BMMC. Furthermore, no morphological difference was observed between Egr-1–deficient and wild-type BMMC by toluidine blue staining or electron microscopic analysis (data not shown).
To determine if Egr-1 has a role in maintaining mast cell viability, cell death was induced by removal of WEHI-3B supernatant (a source for IL-3). Mast cell viability was examined by trypan blue exclusion. The withdrawal of WEHI-3B component from the culture media induced similar level of cell death in Egr-1–deficient and wild-type mast cells (). Thus, Egr-1 is not required for SCF-induced mast cell growth in vitro.
We also examined if Egr-1 is needed for mast cell development in vivo. Mast cells in the ear and back skin of Egr-1–deficient and wild-type mice were examined by alcian blue staining. Consistent with the in vitro results, no difference of mast cell number in these tissues was observed (data not shown).
Egr-1 Deficiency Leads to Decreased IL-13 Production Following SCF Stimulation
SCF regulates expression of various mast cell mediators. IL-13 is a major Type 2 cytokine involved in allergy. SCF has been shown to directly induce IL-13 expression by human mast cells (Hundley et al., Citation2004). We examined if Egr-1 is required for SCF-dependent IL-13 production. Wild-type BMMC and Egr-1-deficient BMMC were treated with SCF (50 ng/ml) for various times. Expression of IL-13 mRNA and protein was determined by quantitative real-time PCR analysis. As shown in , SCF-induced IL-13 mRNA expression was reduced in Egr-1 deficient BMMC when compared to wild-type BMMC. Analysis of IL-13 PCR product using agarose gel also confirmed the reduced IL-13 expression in Egr-1-deficient BMMC (). Furthermore, SCF-induced production of IL-13 at the protein level was also reduced in Egr-1-deficient BMMC comparing to wild-type BMMC ().
FIG. 5 Reduced IL-13 expression due to Egr-1 deficiency. (A-B) Wild-type or Egr-1–deficient BMMCs were stimulated with SCF (50ng/mL) for 15, 60 or 180 min. RNA from these cells was reverse transcribed to cDNA. Real-time quantitative PCR was performed to determine IL-13 expression. GAPDH was used as an internal control. Value of IL-13 mRNA was divided by that of GAPDH mRNA from the same sample. Error bars represent standard errors from three independent experiments (A). The PCR products were also separated by a 2% agarose gel and stained with ethidium bromide (B). (C) Wild-type or Egr-1–deficient BMMCs were stimulated with SCF (50 ng/ml) for 3, 6, 12, or 24 hr. Cell-free supernatants were used for examining IL-13 production by ELISA. Data were expressed as mean ± SE (n = 5).
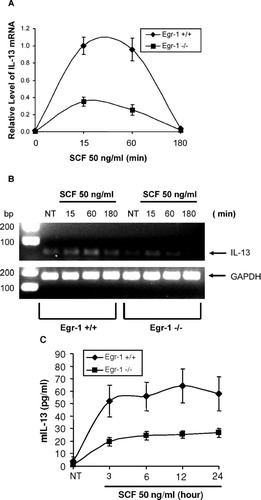
Protein Tyrosine Kinase Inhibitor PP2 Blocked SCF-Induced Egr Activation
Various inhibitors for different protein kinases and phosphatases were used to examine possible mechanisms involved in SCF-induced Egr activation. BMMC were pretreated for 1 hr with PP2 (tyrosine kinases), SB 203580 (p38 mitogen-activated protein kinase, p38 MAPK), PD 98059 (extracellular signal-regulated kinase, ERK), wortmannin (phosphatidylinositol 3-kinase, PI3 kinase), Ro 31-8220 (protein kinase C, PKC), and rapamycin (mammalian target of rapamycin, mTOR). BMMC were then stimulated with SCF (50 ng/ml) for an additional 2 hr. Nuclear proteins were examined for Egr-1 activation by EMSA. Tyrosine kinase inhibitor PP2 blocked SCF-induced Egr activation in a concentration dependent manner (). However, other kinase inhibitors including SB 203580, PD 98059, wortmannin, Ro 31-8220, or rapamycin had no effects (data not shown).
FIG. 6 Protein tyrosine kinase inhibitor PP2 inhibits SCF-induced Egr-1 activation. BMMCs were pretreated with various concentrations of PP2 (0.1, 0.5, 5, or 10 μ M) for 1 hr. Then cells were stimulated with SCF (50 ng/ml) for 2 hr. Nuclear proteins were isolated and subjected to EMSA for Egr-1 binding. Data were analyzed by densitometry.
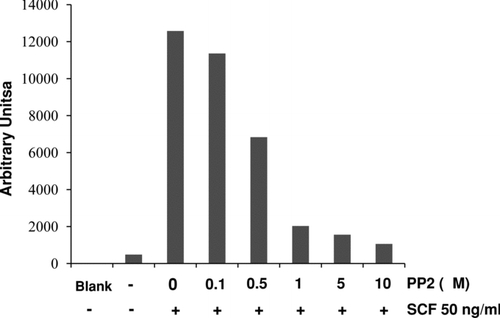
DISCUSSION
Mast cells express two characteristic receptors on their surface, FcεRI and SCF receptor c-Kit. SCF is a critical factor for both mast cell growth and functional activation (Galli et al., Citation1994). Mutations on the c-Kit or the SCF (steel) loci lead to mast cell deficiency in mice (Kitamura and Go, Citation1979; Geissler et al., Citation1988; Huang et al., Citation1990; Zsebo et al., Citation1990). In human, SCF is critical for in vitro generation of mast cells from CD34+ progenitors (Kirshenbaum et al., Citation1992; Valent et al., Citation1992). Major efforts have been made in an attempt to dissect molecular mechanisms involved in SCF-dependent mast cell development and SCF-mediated functional regulation. In this study, we showed that Egr-1 is involved in SCF-stimulated IL-13 production by mast cells. However, Egr-1 is dispensable for SCF-dependent mast cell growth although Egr-1 is critical for the development of a number of other cell types (Krishnaraju et al., Citation1995; Shao et al., Citation1997; Krishnaraju et al., Citation2001).
We demonstrated that Egr-1 mRNA and protein are induced in mast cells following SCF stimulation. SCF also strongly promotes Egr DNA binding activity. At 5 ng/ml, SCF showed a prominent effect on Egr DNA binding activity as determined by EMSA. SCF level in human serum averages 3.3 ± 1.1 ng/ml (Langley et al., Citation1993). It is possible that the SCF level at local tissues where mast cells reside is likely much higher. Thus, SCF is capable of stimulating Egr activation at a physiologically relevant concentration.
SCF appears to play an essential role in the regulation of IL-13 production (Kanbe et al., Citation1999). We further examined the functional role of Egr-1 in SCF-induced IL-13 production by mast cells. Using BMMC from Egr-1-deficient mice, we showed that SCF-induced IL-13 production was reduced in Egr-1-deficient mast cells suggesting that Egr-1 is required for SCF-induced IL-13 production. IL-13 is a 112-amino acid TH2 cytokine that has been shown to play a crucial role in the pathogenesis of asthma (Wills-Karp, 1990). Interestingly, Egr-1 has also been shown to play a key role in IL-13 induced biological responses in vivo and in vitro (Cho et al., Citation2006; Ingram et al., Citation2006). In addition, both TNF production and airway hyperresponsiveness were reduced in Egr-1-deficient mice (Silverman et al., Citation2001). Thus, the interplays between Egr-1 and IL-13 in the presence of SCF likely play a role in the pathogenesis of allergic inflammation.
Five members of Egr family have been reported including Egr-1, -2, -3, -4 and the Wilm's tumor product (Gashler and Sukhatme, Citation1995; Beckmann and Wilce, Citation1997). They share a highly homologous DNA-binding domain that recognizes an identical DNA response element (Beckmann and Wilce, Citation1997). Using real-time PCR analysis, we found that both Egr-1 and Egr-2 mRNA, but not Egr-3, Egr-4, or Wilm's tumor product, were induced in mast cells by SCF stimulation. The DNA binding activity of Egr-1 and Egr-2 was confirmed by using anti-Egr-1 Ab and anti-Egr-2 Ab in EMSA supershift assay. We showed reduced DNA binding activity after anti-Egr-1 and anti-Egr-2 Ab treatment. Although Egr family members possess structural similarities and share DNA binding sequence, their biological functions are likely distinct. Egr-1-deficient mice and Egr-2 deficient mice exhibit vastly different phenotypes. Mice carrying a germ-line knockout of Egr-1 mature to adulthood and do not show any morphological abnormalities (Lee et al., Citation1995).
In contrast, Egr-2-deficient animals show impaired brain development and death during the first two weeks after birth (Schneider-Maunoury et al., Citation1993). In our experiment, SCF induced a similar pattern of Egr-2 expression in Egr-1-deficient and wild-type mast cells (data not shown). A role for Egr-2 in SCF-induced mast cell development and function is unclear.
Previous reports showed that Egr-1 is important for the development of macrophages (Nguyen et al., Citation1993), lymphocytes (Shao et al., Citation1997), and neuronal cells (Beckmann and Wilce, Citation1997). A role of Egr-1 in SCF-dependent mast cell growth was examined. We showed that Egr-1 deficiency has no effects on SCF-induced BMMC growth, IgE receptor and c-Kit expression, as well as mast cell viability in vitro. In vivo, the number and morphology of mast cells in various tissues (ear, back skin or tongue) are similar between Egr-1-deficient and wild-type mice. The lack of effect of Egr-1 in SCF-induced mast cell growth is intriguing considering that SCF plays an essential role for mast cell development. These results support the concept that the effect of Egr-1 is cell-type specific.
We also attempted to examine the signaling molecules responsible for SCF-induced Egr-1 activation. Stimulation of c-Kit by its ligand SCF leads to activation of its intrinsic tyrosine kinase activity and phosphorylation of key tyrosine residues within the receptor. This phosphorylated c-Kit provides docking sites for a number of Src-homology 2 (SH2)-containing molecules leading to activation of multiple signaling pathways, including Src kinase pathway, PI3-kinase pathway, MAP kinase pathway, phospholipase C-γ pathway and JAK/STAT pathway (Ronnstrand, Citation2004; Roskoski, Citation2005). We found that Src kinase inhibitor PP2 blocked SCF-induced Egr-1 activation in a concentration dependent manner, suggesting that the Src pathway is likely responsible for SCF-induced Egr-1 activation in mast cells. This is consistent with previous studies demonstrating that activation of Src kinases is involved in SCF-dependent gene transcription (Lennartsson et al., Citation1999; Bondzi et al., Citation2000). Accordingly, it is likely that activation of SCF-Src-Egr-1 pathway leads to IL-13 production by mast cells.
SCF-activated specific signaling pathway is likely associated with its specific biological effects. PI3 kinase inhibitor wortmannin had no effect on SCF-induced Egr-1 activation, suggesting that PI3 kinase may not be involved in SCF-induced Egr-1 activation. This appears to be consistent with the lack of effect of Egr-1 on SCF-induced mast cell growth. Similarly, inhibitors for p38 MAP kinase (SB 203580), Erk kinase (PD 98059), PKC (Ro 318220) or mTOR (rapamycin) had no effect on Egr-1 DNA binding as tested by EMSA Thus, it is likely that these kinases may not be the upstream molecules responsible for SCF-induced Egr-1 activation.
In summary, we found that mast cells express Egr-1 in response to SCF stimulation. Egr-1 contributes to SCF-induced IL-13 production because Egr-1-deficient mast cells showed reduced level of these cytokines. Interestingly, Egr-1 is not required for SCF-induced mast cell growth. SCF-induced Egr-1 DNA binding is blocked by Src kinase inhibitor, suggesting a role of Src kinase in SCF-induced Egr-1 activation. Taken together, our results suggest that SCF binding to its receptor c-Kit induces Egr-1 activation through Src kinase, leading to IL-13 production. This finding has implications for the regulation of allergic inflammation.
The authors would like to thank Sandy Edgar for his excellent technical assistance in the real-time PCR study. This work was supported by grants from the Canadian Institutes of Health Research, Nova Scotia Health Research Foundation and Izaak Walton Killam Health Center to TJL. TJL is supported by a New Investigator Award from the Canadian Institutes of Health Research and an Investigatorship from Izaak Walton Killam Health Center.
Current address of Bo Li: Department of Immunology, Capital Medical University, Beijing 100069, China.
REFERENCES
- Beckmann A. M., Wilce P. A. Egr transcription factors in the nervous system. Neurochem. Int. 1997; 31: 477–510
- Bondzi C., Litz J., Dent P., Krystal G. W. Src family kinase activity is required for Kit-mediated mitogen-activated protein (MAP) kinase activation, however loss of functional retinoblastoma protein makes MAP kinase activation unnecessary for growth of small cell lung cancer cells. Cell Growth Differ. 2000; 11: 305–314
- Cho S. J., Kang M. J., Homer R. J., Kang H. R., Zhang X., Lee P. J., Elias J. A., Lee C. G. Role of early growth response-1 (Egr-1) in interleukin-13-induced inflammation and remodeling. J. Biol. Chem. 2006; 281: 8161–8168
- Coleman J. W., Holliday M. R., Kimber I., Zsebo K. M., Galli S. J. Regulation of mouse peritoneal mast cell secretory function by stem cell factor, IL-3 or IL-4. J. Immunol. 1993; 150: 556–562
- Columbo M., Horowitz E. M., Botana L. M., MacGlashan D. W., Jr, Bochner B. S., Gillis S., Zsebo K. M., Galli S. J., Lichtenstein L. M. The human recombinant c-kit receptor ligand, rhSCF, induces mediator release from human cutaneous mast cells and enhances IgE-dependent mediator release from both skin mast cells and peripheral blood basophils. J. Immunol. 1992; 149: 599–608
- Costa J. J., Demetri G. D., Harrist T. J., Dvorak A. M., Hayes D. F., Merica E. A., Menchaca D. M., Gringeri A. J., Schwartz L. B., Galli S. J. Recombinant human stem cell factor (kit ligand) promotes human mast cell and melanocyte hyperplasia and functional activationin vivo. J. Exp. Med. 1996; 183: 2681–2686
- Faour W. H., Alaaeddine N., Mancini A., He Q. W., Jovanovic D., Di Battista J. A. Early growth response factor-1 mediates prostaglandin E2-dependent transcriptional suppression of cytokine-induced tumor necrosis factor-α gene expression in human macrophages and rheumatoid arthritis-affected synovial fibroblasts. J. Biol. Chem. 2005; 280: 9536–9546
- Fukao T., Yamada T., Tanabe M., Terauchi Y., Ota T., Takayama T., Asano T., Takeuchi T., Kadowaki T., HataJi J., Koyasu S. Selective loss of gastrointestinal mast cells and impaired immunity in PI3K-deficient mice. Nat. Immunol. 2002; 3: 295–304
- Gagari E., Tsai M., Lantz C. S., Fox L. G., Galli S. J. Differential release of mast cell interleukin-6 via c-kit. Blood 1997; 89: 2654–2663
- Galli S. J., Kalesnikoff J., Grimbaldeston M. A., Piliponsky A. M., Williams C. M., Tsai M. Mast cells as “tunable” effector and immunoregulatory cells: Recent advances. Annu. Rev. Immunol. 2005; 23: 749–786
- Galli S. J., Zsebo K. M., Geissler E. N. The kit ligand, stem cell factor. Adv. Immunol. 1994; 55: 1–96
- Garrington T. P., Ishizuka T., Papst P. J., Chayama K., Webb S., Yujiri T., Sun W., Sather S., Russell D. M., Gibson S. B., Keller G., Gelfand E. W., Johnson G. L. MEKK2 gene disruption causes loss of cytokine production in response to IgE and c-Kit ligand stimulation of ES cell-derived mast cells. EMBO J. 2000; 19: 5387–5395
- Gashler A., Sukhatme V. P. Early growth response protein 1 (Egr-1): Prototype of a zinc-finger family of transcription factors. Prog. Nucleic Acid Res. Mol. Biol. 1995; 50: 191–224
- Geissler E. N., Ryan M. A., Housman D. E. The dominant-white spotting (W) locus of the mouse encodes the c-kit proto-oncogene. Cell 1988; 55: 185–192
- Gibbs B. F., Arm J. P., Gibson K., Lee T. H., Pearce F. L. Human lung mast cells release small amounts of interleukin-4 and tumor necrosis factor-α in response to stimulation by anti-IgE and stem cell factor. Eur. J. Pharmacol. 1997; 327: 73–78
- Huang E., Nocka K., Beier D. R., Chu T. Y., Buck J., Lahm H. W., Wellner D., Leder P., Besmer P. The hematopoietic growth factor KL is encoded by the Sl locus and is the ligand of the c-kit receptor, the gene product of the W locus. Cell 1990; 63: 225–233
- Hundley T. R., Gilfillan A. M., Tkaczyk C., Andrade M. V., Metcalfe D. D., Beaven M. A. Kit and FcεRI mediate unique and convergent signals for release of inflammatory mediators from human mast cells. Blood 2004; 104: 2410–2417
- Ingram J. L., Antao-Menezes A., Mangum J. B., Lyght O., Lee P. J., Elias J. A., Bonner J. C. Opposing actions of Stat1 and Stat6 on IL-13-induced up-regulation of early growth response-1 and platelet-derived growth factor ligands in pulmonary fibroblasts. J. Immunol. 2006; 177: 4141–4148
- Kanbe N., Kurosawa M., Yamashita T., Kurimoto F., Yanagihara Y., Miyachi Y. Cord-blood-derived human cultured mast cells produce interleukin-13 in the presence of stem cell factor. Int. Arch. Allergy Immunol. 1999; 119: 138–142
- Kinet J. P. The high-affinity IgE receptor (FcεRI): From physiology to pathology. Annu. Rev. Immunol. 1999; 17: 931–972
- Kirshenbaum A. S., Goff J. P., Kessler S. W., Mican J. M., Zsebo K. M., Metcalfe D. D. Effect of IL-3 and stem cell factor on the appearance of human basophils and mast cells from CD34+ pluripotent progenitor cells. J. Immunol. 1992; 148: 772–777
- Kitamura Y., Go S. Decreased production of mast cells in S1/S1d anemic mice. Blood 1979; 53: 492–497
- Kobayashi H., Okayama Y., Ishizuka T., Pawankar R., Ra C., Mori M. Production of IL-13 by human lung mast cells in response to Fcε receptor cross-linkage. Clin. Exp. Allergy 1998; 28: 1219–1227
- Krishnaraju K., Hoffman B., Liebermann D. A. Early growth response gene 1 stimulates development of hematopoietic progenitor cells along the macrophage lineage at the expense of the granulocyte and erythroid lineages. Blood 2001; 97: 1298–1305
- Krishnaraju K., Nguyen H. Q., Liebermann D. A., Hoffman B. The zinc finger transcription factor Egr-1 potentiates macrophage differentiation of hematopoietic cells. Mol. Cell. Biol. 1995; 15: 5499–5507
- Langley K. E., Bennett L. G., Wypych J., Yancik S. A., Liu X. D., Westcott K. R., Chang D. G., Smith K. A., Zsebo K. M. Soluble stem cell factor in human serum. Blood 1993; 81: 656–660
- Lee S. L., Tourtellotte L. C., Wesselschmidt R. L., Milbrandt J. Growth and differentiation proceeds normally in cells deficient in the immediate early gene NGFI-A. J. Biol. Chem. 1995; 270: 9971–9977
- Lennartsson J., Blume-Jensen P., Hermanson M., Ponten E., Carlberg M., Ronnstrand L. Phosphorylation of Shc by Src family kinases is necessary for stem cell factor receptor/c-kit mediated activation of the Ras/MAP kinase pathway and c-fos induction. Oncogene 1999; 18: 5546–5553
- Li B., Power M. R., Lin T. J. De novo synthesis of early growth response factor-1 is required for the full responsiveness of mast cells to produce TNF and IL-13 by IgE and antigen stimulation. Blood 2006; 107: 2814–2820
- Metcalfe D. D., Baram D., Mekori Y. A. Mast cells. Physiol. Rev. 1997; 77: 1033–1079
- Nguyen H. Q., Hoffman-Liebermann B., Liebermann D. A. The zinc finger transcription factor Egr-1 is essential for and restricts differentiation along the macrophage lineage. Cell 1993; 72: 197–209
- O'Laughlin-Bunner B., Radosevic N., Taylor Shivakrupa M. L., DeBerry C., Metcalfe D. D., Zhou M., Lowell C., Linnekin D. Lyn is required for normal stem cell factor-induced proliferation and chemotaxis of primary hematopoietic cells. Blood 2001; 98: 343–350
- Ronnstrand L. Signal transduction via the stem cell factor receptor/c-Kit. Cell. Mol. Life Sci. 2004; 61: 2535–2548
- Roskoski R., Jr. Signaling by Kit protein-tyrosine kinase - the stem cell factor receptor. Biochem. Biophys. Res. Commun. 2005; 337: 1–13
- Schneider-Maunoury S., Topilko P., Seitandou T., Levi G., Cohen-Tannoudji M., Pournin S., Babinet C., Charnay P. Disruption of Krox-20 results in alteration of rhombomeres 3 and 5 in the developing hindbrain. Cell 1993; 75: 1199–1214
- Shao H., Kono D. H., Chen L. Y., Rubin E. M., Kaye J. Induction of the early growth response (Egr) family of transcription factors during thymic selection. J. Exp. Med. 1997; 185: 731–744
- Silverman E. S., De Sanctis G. T., Boyce J., Maclean J. A., Jiao A., Green F. H., Grasemann H., Faunce D., Fitzmaurice G., Shi G. P., Stein-Streilein J., Milbrandt J., Collins T., Drazen J. M. The transcription factor early growth-response factor-1 modulates tumor necrosis factor-α, immunoglobulin E, and airway responsiveness in mice. Am. J. Respir. Crit. Care Med. 2001; 163: 778–785
- Takaishi T., Morita Y., Hirai K., Yamaguchi M., Ohta K., Noda E., Morita T., Ito K., Miyamoto T. Effect of cytokines on mediator release from human dispersed lung mast cells. Allergy 1994; 49: 837–842
- Taylor A. M., Galli S. J., Coleman J. W. Stem-cell factor, the kit ligand, induces direct degranulation of rat peritoneal mast cells in vitro andin vivo: Dependence of the in vitro effect on period of culture and comparisons of stem-cell factor with other mast cell-activating agents. Immunology 1995; 86: 427–433
- Valent P., Spanblochl E., Sperr W. R., Sillaber C., Zsebo K. M., Agis H., Strobl H., Geissler K., Bettelheim P., Lechner K. Induction of differentiation of human mast cells from bone marrow and peripheral blood mononuclear cells by recombinant human stem cell factor/kit-ligand in long-term culture. Blood 1992; 80: 2237–2245
- Wills-Karp M. Immunologic basis of antigen-induced airway hyperresponsiveness. Annu. Rev. Immunol. 1999; 17: 255–281
- Zsebo K. M., Williams D. A., Geissler E. N., Broudy V. C., Martin F. H., Atkins H. L., Hsu R. Y., Birkett N. C., Okino K. H., Murdock D. C., Jacobsen F. W., Langley K. E., Smith K. A., Takeish T., Cattanach B. M., Galli S. J., Suggs S. V. Stem cell factor is encoded at the Sl locus of the mouse and is the ligand for the c-kit tyrosine kinase receptor. Cell 1990; 63: 213–224