Abstract
In recent years, progress has been made in understanding how peptides presented by MHC Class I molecules were generated, in particular which proteases are involved in this process and how intracellular pathways influence antigen presentation in professional antigen-presenting cells and various types of tumor cells. This review will give an overview of MHC Class I abnormalities in malignancies and their underlying molecular mechanisms. Dependent on the tumor types structural alterations in particular of the MHC Class I heavy chain, β2-m and the TAP1 subunit have been found at a low frequency, whereas dysregulation of MHC Class I antigen processing components appears to be the major mechanism of MHC Class I down-regulation in tumors of distinct origin. This could occur at the epigenetic, transcriptional and/or post-transcriptional level. The lack or suppression of MHC Class I surface expression due to antigen-processing deficiencies are accompanied by reduced recognition and lysis by antigen-specific cytotoxic T-lymphocytes, which is further often associated with disease progression.
Abbreviations: | ||
APC, | = | antigen presenting cell; |
APM, | = | antigen processing machinery; |
APN, | = | aminopeptidase N; |
BLH, | = | bleomycin hydrolase; β2-m, β2-microglobulin; |
LOH, | = | loss of heterozygosity; |
CSF, | = | colony stimulating factor; |
CTL, | = | cytotoxic T lymphocytes; |
DC, | = | dendritic cells; |
ER, | = | endoplasmic reticulum; |
ERAP, | = | ER aminopeptidase associated with antigen processing; |
GM-CSF, | = | granulocyte-macrophage colony stimulating factor; |
HC, | = | heavy chain; |
IFN, | = | interferon; |
IL, | = | interleukin; |
LAP, | = | leucin aminopeptidase; |
LMP, | = | low molecular weight proteins; |
LOH, | = | loss of heterozygosity; |
luc, | = | luciferase; |
MHC, | = | major histocompatibility complex; |
NK, | = | natural killer; |
PDI, | = | protein disulfide isomerase; |
PLA, | = | proteasome activator; |
PLC, | = | peptide loading complex; |
RCC, | = | renal cell carcinoma; |
TAP, | = | transporter associated with antigen processing; |
TPPII, | = | tripeptidyl peptidase II; |
Treg, | = | regulatory T-lymphocytes; |
TSA, | = | trichostatin A; |
VPA, | = | valproic acid. |
Introduction
The tumor development is a multifactorial process which involves various genetic alterations including activation of oncogenes, inactivation of tumor suppressor genes as well as modification of immunosurveillance molecules resulting in aberrant cell cycle control, instability of genomic integrity and induction of tolerance. Therefore, the tumor host interaction is regulated by a fine balance between tumor cells, professional antigen presenting cells (APC) such as dendritic cells (DC) and B-lymphocytes, tumor cells and other immune effector cells like T-lymphocytes, NK cells, regulatory T-lymphocytes (Treg), macrophages as well as NKT cells (Dunn et al., Citation2004a, Citation2004b). Furthermore, it has been recently demonstrated that the tumor stroma in particular endothelial cells and the tumor microenvironment plays a key role in this process (Dunn et al., Citation2005).
So far, there exist three lines of evidence of cancer immunosurveillance. These include (i) an increased incidence of non-viral tumors in immunosuppressed transplanted patients, (ii) a high frequency of immune cell infiltration within the tumor as well as (iii) the development of spontaneous innate and acquired immune responses. However the immune system can act as a double-edge sword: on one hand tumor growth could induce anti-tumor immune responses, whereas on the other hand proper immune responses could also result in cancer progression due to the selection of immune escape variants which could not be recognized by immune effector cells (Seliger, Citation2005; Buy and Schreiber, Citation2007; Chang and Ferrone, Citation2007).
Tumors have developed different strategies to escape immune response. These include for example (i) the secretion of immune suppressive cytokines like transforming growth factor-β (TGFβ) and interleukin (IL)-10, thereby inhibiting proper T-lymphocyte responses, (ii) FAS expression causing T-lymphocyte apoptosis, (iii) lack of co-stimulatory or (iv) expression of co-inhibitory molecules blocking the secondary, co-stimulatory signal essential for T-lymphocyte activation, (v) expression of non-Classical HLA antigens, like HLA-G known to inhibit both T-lymphocyte and NK cell responses and (vi) lack or down-regulation of MHC Class I antigen processing components. This review focuses on the frequency, function and relevance of MHC Class I abnormalities in tumors.
The complex MHC class I antigen processing pathway
One major task of the immune system is the monitoring of MHC Class I antigen complexes exposed on the cell surface. When MHC Class I molecules do present self-antigens immune tolerance is induced, whereas presentation of foreign antigens such as antigens derived from either transformed or viral proteins results in CD8+ cytotoxic T-lymphocyte (CTL)-mediated lysis. In contrast, the lack of MHC Class I surface antigens caused an insensitivity of CTLs to recognize and lyse tumor cells, but NK cells have the capacity to eliminate these MHC Class I-negative cells. Thus, for a proper T-lymphocyte recognition an effective MHC Class I antigen processing and presentation is required. This is pursued by the complex MHC Class I antigen processing machinery (APM), which has been well characterized during the last two decades. It consists of four major steps: (i) peptide generation/trimming; (ii) peptide transport; (iii) MHC Class I assembly and (iv) antigen presentation (; Yewdell, Citation2005; Jensen, Citation2007).
Figure 1. Schematic diagram of MHC Class I antigen processing machinery (APM). Ubiquitinated proteins were cleaved into peptides by the proteasome and further trimmed by cytosolic peptidases. The peptides are then transported via the TAP heterodimer from the cytosol into the ER. In the ER, MHC Class I heavy chain (HC) and β2-m are stabilized and associate which is assisted by the chaperones calnexin and calreticulin. A multimeric peptide loading complexes (PLC) is yielded consisting of the TAP subunits, MHC HC, β2-m, tapasin, calreticulin and ERp57. Upon peptide loading the PLC dissociates and the peptide/MHC Class I/β2-m complex is transported via the trans-Golgi to the cell surface.
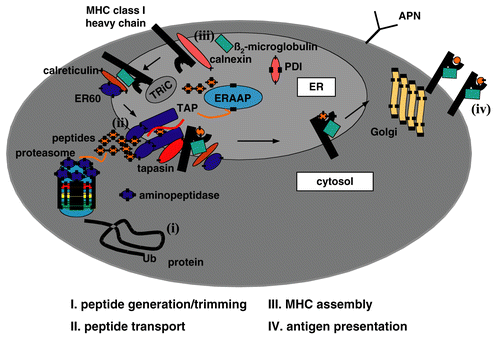
Briefly, endogenously synthesized proteins were ubiquitinated and then subjected to degradation by the multicatalytic proteasome complex. In particular the interferon (IFN)-γ-inducible proteasome subunits, the low molecular weight proteins (LMP) 2 and 7 are involved in this process. The proteasome yields the correct C-terminus of the peptide, whereas the N-terminus is further trimmed by peptidases localized in the cytosol such as the tripeptidyl peptidase II (TPPII), the bleomycin hydrolase (BLH) and the leucin aminopeptidase (LAP)3 and in the endoplasmic reticulum (ER), like the ER aminopeptidases associated with antigen processing (ERAP) 1 und 2 (Hammer et al., Citation2007). The trimmed peptides are then transported via the heterodimeric transporter associated with antigen processing TAP1 and TAP2 from the cytosol into the ER. In the ER the MHC Class I assembly occurs, which is assisted by the chaperones calnexin, calreticulin, the protein disulfide isomerase (PDI) as well as ERp57. Furthermore, the chaperone tapasin as a component of the multimeric peptide loading complex (PLC) is required for the stabilization of TAP and facilitates peptide loading onto MHC Class I molecules (Cabrera, Citation2007). Upon peptide loading the PLC dissociates and the trimer consisting of the MHC Class I heavy chain (HC), β2-microglobulin (β2-m) and antigen is released and transported via the trans Golgi to the cell surface and there exposed to the CD8+ CTL.
Physiological expression and regulation of MHC class I APM components
MHC Class I antigens and the various APM components are constitutively expressed in all normal tissues and cell lines, but not expressed in embryonic cells, testis, and ovar. However, there exists a heterogeneous expression pattern under physiological conditions, which might be due to tissue specificity, differentiation-dependence, cell cycle control and changes in the microenvironment thereby altering the repertoire of T-lymphocyte epitopes presented. An association of MHC Class I APM component expression and cell cycle has been demonstrated for TAP1 and LMP2 mRNA (Alpan et al., 1997). Furthermore, a p53-mediated induction of TAP was found by Zhu and co-authors (Citation1999).
Regarding the differentiation, the APM expression pattern was analysed during maturation of dendritic cells (DC). DC was obtained from human blood or buffy coat and CD14+ monocytes were isolated. These cells were treated on Days 1, 3 and 5 with GM-CSF and IL-4. At Day 5, immature DCs were generated and upon their cultivation with a maturation cytokine cocktail, mature DC were obtained at Day 7. These mature DC were characterized by the expression of the proper DC-specific markers such as the co-stimulatory molecules CD80, CD86, MHC Class I and Class II antigens and the DC marker CD83. The APM component expression pattern of immature (Day 5) and mature (Day 7) DC was compared at the mRNA and protein level. While the LMP subunits were highly expressed in immature DC when compared to mature DC, an inverse expression was found for the components mainly involved in antigen presentation ( Li et al., Citation2001; Randolph and Jakubzik, 2007).
Figure 2. Heterogeneous APM component expression in DCs during the differentiation process. Protein from immature and mature DC was extracted and subjected to Western blot analysis using anti-APM component-specific antibodies. The control represent the human keratinocyte cell line HaCat.
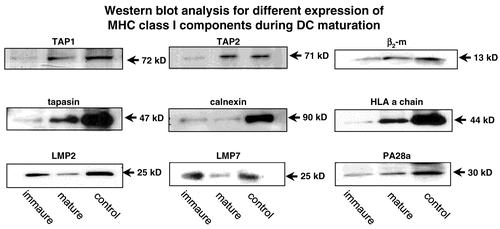
The expression of MHC Class I HC, β2-m, tapasin, and the TAP1/TAP2 heterodimer is more pronounced in the mature DC than in their immature counterpart (Li et al., Citation2001). These data demonstrate a differentiation-dependent APM component regulation reflecting the major functional activity of DCs during maturation . In addition, differentiation of tumor cells by retinoic and retinoic acid (RA) (Vertuani et al., Citation2003; Citation2007) as well as modulation of the epigenetic control mechanisms by demethylating substances like 2´5´-azadesoxycytidine and deacetylating agents such as the valproic acid (VPA) and trichostatin A (TSA) are able to induce the expression of some MHC Class I APM components. Beside the effect of different substances on APM components stress parameters like heat shock, irradiation and oxidative stress could also modulate their expression (Deng et al., Citation2003; Theoh and Davies, Citation2004; Reits et al., Citation2006).
Figure 3. Association of the differentiation-dependent APM component expression in DC with their function.
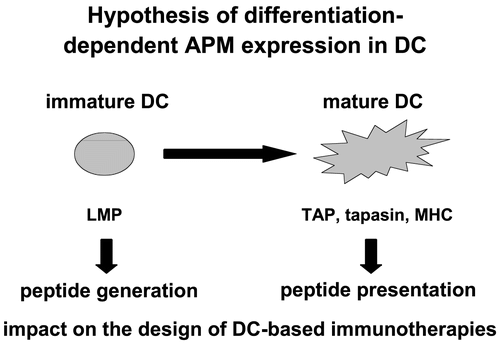
Concerning the modulation of the MHC Class I APM component expression by cytokines, IFNs, tumor necrosis factor (TNF)-α, granulocyte macrophage colony stimulations factor (GM-CSF) and IL-4 could up-regulate the expression of the major APM components, whereas IL-10 has an inhibitory activity CitationFruci et al., 1999; Hallermalm et al., 2001; Kurte et al., Citation2004; Petersson et al., Citation1998). The most potent stimulator of APM component expression is IFNγ, which up-regulates the LMP subunits, the proteasome activator (PA)28, TAP1, TAP2, MHC Class I, HC, β2-m, ERAP1, ERAP2 as well as LAP3, whereas no effect of this cytokine was found on the expression of calnexin, calreticulin and protein disulfide isomerase (PDI). In addition, there exist also synergistic effects of treatment combinations in particular for example IFNα and IFNγ strongly enhanced APM component expression when compared to a single treatment.
MHC class I deficiencies in tumors
It has been demonstrated that human tumors of distinct histology often expressed low or down-regulated MHC Class I surface antigens, which could be due to modulation and/or inhibition of the expression of various MHC Class I APM components (Aptsiauri et al., Citation2007; Bangia and Ferrone, Citation2006; Cabrera et al., Citation2007; Chang et al., Citation2003; Seliger et al., Citation2000, 2006a and b). The distinct frequency of MHC Class I abnormalities could be due to total HLA Class I antigen loss, HLA Class I down-regulation as well as loss or down-regulation of HLA Class I allo-specificities (Mendez et al., Citation2008). However, the frequency and mode of these defects significantly varied between the types of tumors analysed (Atkins et al., Citation2004a, Citation2004b; Mendez et al., Citation2008). Mutations or deletions in β2-m were detected in colon carcinoma (21%), melanoma (15%) and in other tumors (>5%). The mutations in β2-m were found on one copy the gene due to a base pair substitution or deletion suggesting a hot spot in this region. This alteration is associated with a loss of the second wild-type gene due a total or partial loss chromosome 15 (Chang et al., Citation2006). Concerning the haplotype-specific loss, this has been associated with loss of heterozygosity (LOH) on chromosome 6p21. It has been detected in different tumors and tumor cell lines (Jimenez et al., Citation1999).
Using a combination of immunhistochemistry with molecular analyses and microdis-section, such alterations are easier to detect (Ramal et al., Citation2000). For example haplotype loss was shown in head and neck squamous cell carcinoma with a frequency of 36%, whereas in renal cell carcinoma (RCC) LOH only occurs in approximately 12% of tumor lesions analysed (Maleno et al., Citation2004). The locus-specific down-regulation, which results in lack of the sensitivity to HLA-restricted CTL-mediated lysis, has been demonstrated in particular in colorectal, cervical and laryngeal carcinoma as well as in melanoma. It could be caused by the loss of transcription factor binding due to oncogenic or viral transformation for example by ras, HER-2/neu, myc and HPV16 E6 (Atkins et al., Citation2004a, Citation2004b). Allele-specific loss is caused by structural alterations in the MHC Class I HC involving in particular HLA-A11, -A2, -A42 and HLA-B15. Beside these abnormalities down-regulation of APM components such as LMP and TAP could result in inefficient MHC Class I surface expression. Whereas MHC Class I HC and β2-m is not affected in RCC, a strong down-regulation of LMP2, LMP7, TAP and tapasin was found in this tumor type. Furthermore, the frequency of APM deficiencies differed between the RCC subtypes analysed () and was more pronounced in metastatic lesions when compared to the primary tumor (Seliger et al., Citation1996a and Citationb; ).
Table 1. Highly-variable expression of LMP2, LMP7 and TAP1 in different RCC subtypes
Figure 5. Immunohistochemical analysis of primary and metastatic RCC lesions. Immuno-histochemistry of a benign oncocytoma and of RCC lesions was performed using anti-APM-specific antibodies.
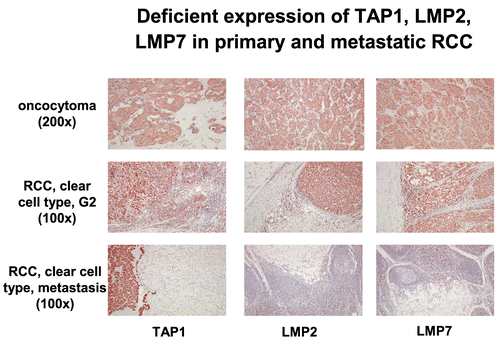
In some cancers, MHC Class I deficiencies correlated with a reduced survival rate of patients suggesting that APM abnormalities is have a clinical relevance (Meissner et al., Citation2005; Ramnath et al., Citation2006). These abnormalities are further associated with a diminished sensitivity to CTL-mediated lysis, but lack of destruction by NK cells. In most cases, this phenotype is IFNγ-inducible. However, there also exists a loss of IFNγ inducibility despite the lack of APM deficiencies, which can be caused by abnormalities in the IFN signal transduction pathway (Rodriguez et al., Citation2007). Concerning chaperones there exist little information regarding the expression pattern of tapasin, calnexin, calreticulin, ERp57 and PDI in human tumors compared to the normal counterpart. This could be reflected by either the lack of analyses of these components or by the low frequency and significance of such deficiencies. So far, calreticulin and PDI is unaltered in all tumor samples analysed, whereas calnexin loss or down-regulation has only been found in cervical carcinoma. Monitoring of ERp57 has not yet been performed. A down-regulation of tapasin was detected in RCC, melanoma, neuroblastoma, hepatocellular carcinoma as well as in head and neck squamous cell carcinoma.
Mechanisms of MHC class I abnormalities in tumors of distinct origin
The molecular mechanisms underlying the APM component down-regulation could occur at different levels including structural alterations and dysregulation due to epigenetic control, transcriptional and posttranscriptional modulation. So far, mutations of the MHC I HC, β2-m, TAP1 have been described in human tumors (Chen et al., Citation1996; Seliger et al., Citation2001), but appear to be a rare event. In addition we have recently identified tapasin and LMP mutations in neuroblastoma. Based on the low frequency of structural alterations it has been suggested that APM components are mainly regulated at the epigenetic, transcriptional, and/or post-transcriptional level in human tumors . These different steps alone or in combination control MHC Class I surface expression. However it is noteworthy that there can also exist a loss of MHC Class I APM inducibility by IFN due to defects in the IFN signal transduction pathway (Rodriguez et al., Citation2007).
In order to determine the differential regulatory mechanisms a number of distinct approaches were employed. First, the various APM promoters were cloned and hooked to the luciferase (luc) reporter gene. These APM promoter luc constructs were then transiently transfected in human tumor cell lines of distinct origin . A distinct promoter activity was detected in the tumor cell lines analysed suggesting a transcriptional and/or a post-transcriptional regulation of APM component expression as shown in representatively for the dual TAP1 and LMP2 promoter in melanoma cells. In addition, in some esophageal squamous cell carcinoma, colon carcinoma, RCC, and melanoma cell lines a methylation of tapasin was found which could be reverted by demethylating agents like 2´5´desoxyazacytidine (Nie et al., Citation2001; Manning et al., Citation2008; Seliger, unpublished). However, TAP1 and TAP2 appear not to be directly methylated, but treatment with 2´5´desoxyazacytidine was able to revert their expression suggesting that factors regulating the TAP1 and TAP2 promoter DNA become demethylated.
Figure 7. Strategy of determination of APM promoter activity. Upon cloning of the APM promoters they were linked to the luciferase gene and these APM promoter luc constructs were then transiently transfected into the respective cell lines. The cells were either left untreated or treated with IFNγ before the luciferase activity was determined.
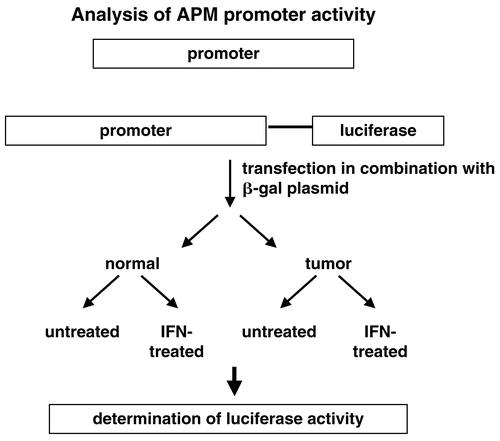
Figure 8. Transcriptional and posttranscriptional regulation of the TAP1/LMP2 promoter activity in distinct melanoma cells. The dual TAP1/LMP2 promoter was transfected into different melanoma cell lines and the promoter activity was determined demonstrating a heterogeneous promoter activity in the cell lines tested and often an unexpected discordant regulation of the TAP1 and LMP2 promoter activity.
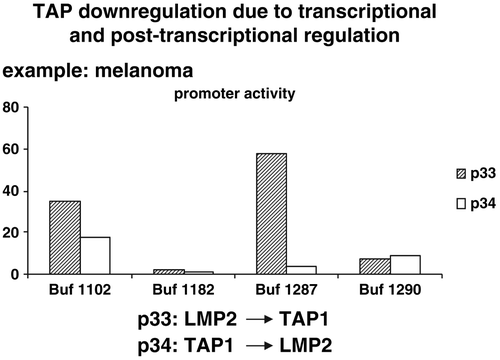
Association of peptidase expression with HLA class I surface expression
It has been recently suggested that peptidases, in particular amino- and ectopeptidases, modulate the anti-tumor T-lymphocyte responses. These peptidases could be found in the cytosol, in the ER as well as on the cell surface. A failure to customize the peptide MHC Class I repertoire has profound immunological consequences. A distinct expression pattern of both cytosolic as well as ER-resident peptidases have been described in some tumors, but only a few lesions have so far been analysed (Fruci et al., Citation2006). The aberrant expression of these peptidases has an influence on MHC Class I expression and the antigen repertoire presented. Therefore, we determined the expression pattern of BLH, TPPII, ERAP1 and ERAP2 in a large series of RCC as well as melanoma cell lines.
A heterogeneous mRNA and/or expression profile of these peptidases was detected in the different tumor cell lines analysed, but the role and underlying mechanisms of their differential expression has still to be evaluated. Concerning the membrane-bound aminopeptidases the expression of aminopeptidase N(APN)/CD13 was monitored. Beside its different expression in human tumors, over-expression of APN in MHC-Class I-negative colon carcinoma cells results in an increased MHC Class I surface expression which is directly associated with an up-regulation of the transcription and translation of the major APM components including MHC Class I HC, β2-m, TAP1, TAP2 as well as the LMP subunits (Seliger, personal communication). Furthermore, this APN-mediated up-regulation of MHC Class I surface expression was associated with an increased recognition by CD8+ CTL.
Perspectives
As summarized in this article MHC Class I abnormalities occur in human tumors at a high frequency and were often associated of the metastatic potential of the disease. The frequency of deficiencies were dependent on the component and tumor type analysed and varied from 15 to 95%. These abnormalities further result in impaired host anti-tumor immunity. In addition, it has been suggested that the disappointing efficacy of T-lymphocyte-based immunotherapies might be due to the down-regulation or lack of MHC Class I surface antigen expression on tumor cells, which is associated with reduced CTL recognition, Therefore, the assessment of MHC Class I APM components might identify (i) patients at risk for metastatic dissemination and (ii) patients which might respond to immunotherapy. Based oh this information, prospective studies have to be performed to validate the value of determination of the MHC Class I abnormalities for prognosis and prior to immunotherapy. However, this is difficult since in most studies the patients’ follow-up is not obtained. Concerning the already published results the statistical power of these analyses is limited, due to the low number of tumor samples analysed so far in this respect. In this context also immunotherapeutic trials have to be revisited regarding the MHC Class I APM expression in tumor cells prior to vaccination.
References
- Alpan, R. S., Zhang, M., Pardee, A. B. 1996. Cell cycle-dependent expression of TAP1, TAP2, and HLA-B27 messenger RNAs in a human breast cancer cell line. Cancer Res. 56:4358–4361.
- Aptsiauri, N., Cabrera, T., Mendez, R., Garcia-Lora, A., Ruiz-Cabello, F., Garrido, F. 2007. Role of altered expression of HLA Class I molecules in cancer progression. Adv. Exp. Med. Biol. 601:123–131.
- Atkins, D., Breuckmann, A., Schmahl, G. E., Binner, P., Ferrone, S., Krummenauer, F., Storkel, S., Seliger, B. 2004a. MHC Class I antigen processing pathway defects, ras mutations and disease stage in colorectal carcinoma. Int. J. Cancer 109:265–273.
- Atkins, D., Ferrone, S., Schmahl, G. E., Storkel, S., Seliger, B. 2004b. Down-regulation of HLA Class I antigen-processing molecules: An immune escape mechanism of renal cell carcinoma? J. Urol. 171:885–889.
- Bangia, N., Ferrone, S. 2006. Antigen presentation machinery (APM) modulation and soluble HLA molecules in the tumor microenvironment: Do they provide tumor cells with escape mechanisms from recognition by cytotoxic T-lymphocytes? Immunol. Invest. 35:485–503.
- Bui, J. D., Schreiber, R. D. 2007. Cancer immunosurveillance, immunoediting, and inflammation: Independent or interdependent processes? Curr. Opin. Immunol. 19:203–208.
- Cabrera, C. M. 2007. The double role of the endoplasmic reticulum chaperone tapasin in peptide optimization of HLA Class I molecules. Scand. J. Immunol. 65:487–493.
- Cabrera, T., Maleno, I., Collado, A., Lopez Nevot, M. A., Tait, B. D., Garrido, F. 2007. Analysis of HLA Class I alterations in tumors: Choosing a strategy based on known patterns of underlying molecular mechanisms. Tissue Antigens 69(S1):264–268.
- Chang, C. C., Ferrone, S. 2007. Immune selective pressure and HLA Class I antigen defects in malignant lesions. Cancer Immunol. Immunother. 56:227–236.
- Chang, C. C., Campoli, M., Ferrone, S. 2003. HLA Class I defects in malignant lesions: What have we learned? Keio. J. Med. 52:220–229.
- Chang, C. C., Ogino, T., Mullins, D. W., Oliver, J. L., Yamshchikov, G. V., Bandoh, N., Slingluff, C. L., Jr., Ferrone, S. 2006. Defective human leukocyte antigen Class I-associated antigen presentation caused by a novel β2-microglobulin loss-of-function in melanoma cells. J. Biol. Chem. 281:18763–18773.
- Chen, H. L., Gabrilovich, D., Tampe, R., Girgis, K. R., Nadaf, S., Carbone, D. P. 1996. A functionally-defective allele of TAP1 results in loss of MHC Class I antigen presentation in a human lung cancer. Nat. Genet. 13:210–213.
- Deng, X. L., Chen, W., Cai, M. Y., Wei, D. P. 2003. Expression of Class I MHC molecule, HSP70 and TAP in human hepatocellular carcinoma. World J. Gastroenterol. 9:1853–1855.
- Dunn, G. P., Ikeda, H., Bruce, A. T., Koebel, C., Uppaluri, R., Bui, J., Chan, R., Diamond, M., White, J. M., Sheehan, K. C., Schreiber, R. D. 2005. Interferon-γ and cancer immunoediting. Immunol Res. 32:231–245.
- Dunn, G. P., Old, L. J., Schreiber, R. D. 2004a. The immunobiology of cancer immunosurveillance and immunoediting. Immunity 21:137–148.
- Dunn, G. P., Old, L. J., Schreiber, R. D. 2004b. The three Es of cancer immunoediting. Annu. Rev. Immunol. 22:329–360.
- Fruci, D., Ferracuti, S., Limongi, M. Z., Cunsolo, V., Giorda, E., Fraioli, R., Sibilio, L., Carroll, O., Hattori, A., van Endert, P. M., Giacomini, P. 2006. Expression of endoplasmic reticulum aminopeptidases in EBV-B cell lines from healthy donors and in leukemia/ lymphoma, carcinoma, and melanoma cell lines. J. Immunol. 176:4869–4879.
- Garcia-Lora, A., Algarra, I., Garrido, F. 2003. MHC Class I antigens, immune surveillance, and tumor immune escape. J. Cell. Physiol. 195:346–355.
- Hammer, G. E., Kanaseki, T., Shastri, N. 2007. The final touches make perfect the peptide-MHC Class I repertoire. Immunity 26:397–406.
- Jensen, P. E. 2007. Recent advances in antigen processing and presentation. Nat. Immunol. 8:1041–1048.
- Jimenez, P., Canton, J., Collado, A., Cabrera, T., Serrano, A., Real, L. M., Garcia, A., Ruiz-Cabello, F., Garrido, F. 1999. Chromosome loss is the most frequent mechanism contributing to HLA haplotype loss in human tumors. Int. J. Cancer 83:91–97.
- Kurte, M., Lopez, M., Aguirre, A., Escobar, A., Aguillon, J. C., Charo, J., Larsen, C. G., Kiessling, R., Salazar-Onfray, F. 2004. A synthetic peptide homologous to functional domain of human IL-10 down-regulates expression of MHC Class I and transporter associated with antigen processing 1/2 in human melanoma cells. J. Immunol. 173:1731–1737.
- Li, J., Schuler-Thurner, B., Schuler, G., Huber, C., Seliger, B. 2001. Bipartite regulation of different components of the MHC Class I antigen-processing machinery during dendritic cell maturation. Int. Immunol. 13:1515–1523.
- Maleno, I., Cabrera, C. M., Cabrera, T., Paco, L., Lopez-Nevot, M. A., Collado, A., Ferron, A., Garrido, F. 2004. Distribution of HLA Class I altered phenotypes in colorectal carcinomas: High frequency of HLA haplotype loss associated with loss of heterozygosity in chromosome region 6p21. Immunogenetics 56:244–253.
- Manning, J., Indrova, M., Lubyova, B., Pribylova, H., Bieblova, J., Hejnar, J., Simova, J., Jandlova, T., Bubenik, J., Reinis, M. 2008. Induction of MHC Class I molecule cell surface expression and epigenetic activation of antigen-processing machinery components in a murine model for human papilloma virus 16-associated tumours. Immunology 123:218–227.
- Meissner, M., Reichert, T. E., Kunkel, M., Gooding, W., Whiteside, T. L., Ferrone, S., Seliger, B. 2005. Defects in the human leukocyte antigen Class I antigen processing machinery in head and neck squamous cell carcinoma: Association with clinical outcome. Clin. Cancer Res. 11:2552–2560.
- Mendez, R., Rodriguez, T., Del Campo, A., Monge, E., Maleno, I., Aptsiauri, N., Jiménez, P., Pedrinaci, S., Pawelec, G., Ruiz-Cabello, F., Garrido, F. 2008. Characterization of HLA Class I altered phenotypes in a panel of human melanoma cell lines. Cancer Immunol. Immunother. 57:719–729.
- Nie, Y., Yang, G., Song, Y., Zhao, X., So, C., Liao, J., Wang, L. D., Yang, C. S. 2001. DNA hypermethylation is a mechanism for loss of expression of the HLA Class I genes in human esophageal squamous cell carcinomas. Carcinogenesis 22:1615–1623.
- Petersson, M., Charo, J., Salazar-Onfray, F., Noffz, G., Mohaupt, M., Qin, Z., Klein, G., Blankenstein, T., Kiessling, R. 1998. Constitutive IL-10 production accounts for the high NK sensitivity, low MHC Class I expression, and poor transporter associated with antigen processing (TAP)-1/2 function in the prototype NK target YAC-1. J. Immunol. 161:2099–2105.
- Ramal, L. M., Maleno, I., Cabrera, T., Collado, A., Ferron, A., Lopez-Nevot, M. A., Garrido, F. 2000. Molecular strategies to define HLA haplotype loss in microdissected tumor cells. Hum. Immunol. 61:1001–1012.
- Ramnath, N., Tan, D., Li, Q., Hylander, B. L., Bogner, P., Ryes, L., Ferrone, S. 2006. Is down-regulation of MHC Class I antigen expression in human non-small cell lung cancer associated with prolonged survival? Cancer Immunol. Immunother. 55:891–899.
- Reits, E. A., Hodge, J. W., Herberts, C. A., Groothuis, T. A., Chakraborty, M., Wansley, E. K., Camphausen, K., Luiten, R. M., de Ru, A. H., Neijssen, J., Griekspoor, A., Mesman, E., Verreck, F. A., Spits, H., Schlom, J., van Veelen, P., Neefjes, J. J. 2006. Radiation modulates the peptide repertoire, enhances MHC Class I expression, and induces successful antitumor immunotherapy. J. Exp. Med. 203:1259–1271.
- Rodriguez, T., Mendez, R., Del Campo, A., Jimenez, P., Aptsiauri, N., Garrido, F., Ruiz-Cabello, F. 2007. Distinct mechanisms of loss of IFNγ-mediated HLA Class I inducibility in two melanoma cell lines. BMC Cancer 7:34.
- Seliger, B. 2005. Strategies of tumor immune evasion. BioDrugs 19:347–354.
- Seliger, B., Hohne, A., Knuth, A., Bernhard, H., Ehring, B., Tampe, R., Huber, C. 1996a. Reduced membrane major histocompatibility complex Class I density and stability in a subset of human renal cell carcinomas with low TAP and LMP expression. Clin. Cancer Res. 2:1427–1433.
- Seliger, B., Hohne, A., Knuth, A., Bernhard, H., Meyer, T., Tampe, R., Momburg, F., Huber, C. 1996b. Analysis of the major histocompatibility complex Class I antigen presentation machinery in normal and malignant renal cells: Evidence for deficiencies associated with transformation and progression. Cancer Res. 56:1756–1760.
- Seliger, B., Maeurer, M. J., Ferrone, S. 2000. Antigen-processing machinery breakdown and tumor growth. Immunol. Today 21:455–464.
- Seliger, B., Ritz, U., Abele, R., Bock, M., Tampe, R., Sutter, G., Drexler, I., Huber, C., Ferrone, S. 2001. Immune escape of melanoma: first evidence of structural alterations in two distinct components of the MHC Class I antigen processing pathway. Cancer Res. 61:8647–8650.
- Seliger, B., Ritz, U., Ferrone, S. 2006. Molecular mechanisms of HLA Class I antigen abnormalities following viral infection and transformation. Int. J. Cancer 118:129–138.
- Teoh, C. Y., Davies, K. J. 2004. Potential roles of protein oxidation and the immunoproteasome in MHC Class I antigen presentation: the ‘PrOxI’ hypothesis. Arch. Biochem. Biophys. 423:88–96.
- Vertuani, S., De Geer, A., Levitsky, V., Kogner, P., Kiessling, R., Levitskaya, J. 2003. Retinoids act as multi-step modulators of the major histocompatibility Class I presentation pathway and sensitize neuroblastomas to cytotoxic lymphocytes. Cancer Res. 63:8006–8013.
- Vertuani, S., Dubrovska, E., Levitsky, V., Jager, M. J., Kiessling, R., Levitskaya, J. 2007. Retinoic acid elicits cytostatic, cytotoxic and immunomodulatory effects on uveal melanoma cells. Cancer Immunol. Immunother. 56:193–204.
- Yewdell, J. W. 2005. The seven dirty little secrets of major histocompatibility complex Class I antigen processing. Immunol. Rev. 207:8–18.
- Zhu, K., Wang, J., Zhu, J., Jiang, J., Shou, J., Chen, X. 1999. p53 induces TAP1 and enhances the transport of MHC Class I peptides. Oncogene 18:7740–7747.