Abstract
Monocrotaline (MCT) is a pyrrolizidine alkaloid found in a variety of plants. The main symptoms of MCT toxicosis in livestock are related to hepato- and nephrotoxicity; in rodents and humans, the induction of a pulmonary hypertensive state that progresses to cor pulmonale has received much attention. Although studies have shown that MCT can cause effects on cellular functions that would be critical to those of lymphocytes/macrophages during a normal immune response, no immunotoxicological study on MCT have yet to ever be performed. Thus, the aim of the present study was to evaluate the effect of MCT on different branches of the immune system using the rat – which is known to be sensitive to the effects of MCT - as the model. Rats were treated once a day by gavage with 0.0, 0.3, 1.0, 3.0, or 5.0 mg MCT/kg for 14 days, and then any effects of the alkaloid on lymphoid organs, acquired immune responses, and macrophage activity were evaluated. No alterations in the relative weight of lymphoid organs were observed; however, diminished bone marrow cellularity in rats treated with the alkaloid was observed. MCT did not affect humoral or cellular immune responses. When macrophages were evaluated, treatments with MCT caused no significant alterations in phagocytic function or in hydrogen peroxide (H2O2) production; however, the MCT did cause compromised nitric oxide (NO) release by these cells.
Introduction
Pyrrolizidine alkaloids are common constituents of hundreds of plant species around the world (Roeder, Citation1995). About 600 pyrrolizidine alkaloids and their N-oxide derivatives are known and are found in > 6000 plants in three families—Boraginaceae, Compositae, and Leguminosae - with Crotalaria spectabilis one of the most representative species of the last family (Nobre et al., Citation1994). In many places worldwide, Crotalaria spp is cultivated for use as a natural fertilizer, inasmuch as it fixes nitrogen in the soil (Bokhtiar et al., Citation2003). Livestock are often at risk of poinsoning by this plant when have access to Crotalaria spp.-treated areas, especially during the dry season.
Humans can also be readily exposed to these plants and their alkaloids. This is primarily a problem for individuals who drink herbal teas and/or home-brewed medications derived from the foliage/seeds of Crotalaria spp. in the form of “bush tea” (Srivastava and Mohabbat, Citation1977) or through the consumption of grains contaminated with Crotalaria spp seeds (Eloff et al., Citation2003). Moreover, foodstuffs, e.g., milk, honey, grains, herbal medicines, dietary supplements, can also be contaminated by the alkaloids (Byron, Citation1998; Prakash et al., Citation1999; Steenkamp et al., Citation2000). Regardless of the means of consumption by the livestock or humans, the main active principle in Crotalaria spp is the pyrrolizidine alkaloid monocrotaline (MCT). However, this agent must be transformed into a pyrrole compound, dehydromonocrotaline (MCTP), by microsomal liver enzymes in order to become the active toxic molecule that possesses a strong bonding affinity for nucleophilic groups (Huxtable, Citation1990; Niwa et al., Citation1991; Wang et al., Citation2005).
The cardinal toxic effects observed in livestock intoxicated with plants that contain MCT are related to hepato- and nephrotoxicity, as well as gastrointestinal edema, diarrhea, rectal prolapse, ascites, photosensitization, and sluggishness (Cheeke, Citation1998). Histologically, hepatic periacinar necrosis, megalocytosis of hepatocytes with neutrophilic infiltration, glomerulonephritis, and alterations in renal tubular epithelium cells have also been observed (Figueredo et al., Citation1987; Wilson et al., Citation1992). In addition to many of these pathologies, rodents and humans intoxicated with MCT have been observed to display progressive pulmonary hypertension with vascular lesions (vasculitis), extracellular matrix secretion in pulmonary arteries, increased smooth muscle growth in pulmonary arterioles, and interstitial inflammation that progresses to cor pulmonale (Chesney et al., Citation1974; Pan et al., Citation1993). For these latter reasons, MCT has been extensively used as a laboratory tool to study pulmonary hypertension development.
The immune system is dependent on cell-cell signaling between membrane proteins and receptors to induce lymphocyte activation, proliferation, and finally, establishment of a vigorous functional immune response. Shah et al. (Citation2005) suggested that one toxic mechanism employed by MCTP is interference in protein/receptor trafficking from the Golgi apparatus to the plasma membrane. Mukhopadhyay et al. (Citation2006) proposed that Golgi impairment induced by MCTP could result in a block of mitotic activity, leading to cellular alterations, including megalocytosis. Despite the lack of reported immunosuppression arising from natural MCT toxicosis, we hypo-thesized that this alkaloid could cause a decrease in host immune responses as a result, in part, of the effects delineated in the cited recent investigations. The present study was undertaken to evaluate the effects of low doses of MCT on different branches of the immune responses in rats, a laboratory species known to be susceptible to the development of Crotalaria spp. toxicosis.
Materials and methods
MCT extraction, purification and dosage preparation
Powdered Crotalaria spectabilis seeds (1 kg), defatted with hexane, were exhaustively extracted by maceration with 92% ethanol. The combined extracts were filtered and concentrated under reduced pressure to remove the solvent. The residue was dissolved in a mixture of ethanol-water (1:1), applied to a column of Amberlite IR-120B (H+ form), and extracted with 0.5 M ammonium hydroxide (NH4OH). The resulting eluate was concentrated under reduced pressure, resulting in a white residue that was, in turn, re-crystallized from methanol (three times) to yield colorless crystals (2.3 g). These crystals were then compared with standard MCT (Sigma, St. Louis, MO) using thin-layer chromatography (Molyneux and Roitman, Citation1980), as well as elemental and spectral (1H and 13C NMR) analyses (all performed at Analytical Laboratory of the Institute of Chemistry, University of São Paulo).
The final crystal products (0.23% yield) generated from extracts of the C. spectabilis seeds showed strong similarity to the standard MCT. The elemental composition calculated for MCT (C16H23NO3) is C =59.06; H = 7.13, and N = 4.31% and analysis of the product showed C = 59.05, H = 6.80, and N = 4.39%. The spectra data of 1H and 13C of the MCT obtained fully agreed with those previously reported by Culvenor and Smith (Citation1963) and Molyneux et al. (Citation1982); from these results, the purity of the extracted product was determined to be 99.26%.
To prepare the doses used in the present studies, MCT was first dissolved and mixed for 15 min in 1% phosphoric acid solution. The pH was adjusted to 7 with NaOH and distilled water was then added to achieve the final concentrations of 0.3; 1.0; 3.0; 5.0 and 7.0 mg MCT/ml for use herein.
Animals
Male adult Wistar rats (10 wk-of-age) were obtained from our colony in the Department of Pathology in the School of Veterinary Medicine and Animal Science. All rats received food and water ad libitum and were maintained under controlled conditions of temperature (22–25°C), relative humidity (50–65%) and lighting (12 hr/12 hr light/dark cycle). Food consumption and body weight gain were measured every other day. Experiments were carried out in accordance with the ethical principles for animal research adopted by the Bioethics Committee of the School of Veterinary Medicine and Animal Science, University of São Paulo.
Evaluation of MCT toxicity (Study 1a)
Twenty-four rats were randomly divided into one control and three experimental groups. Rats from the experimental groups were treated once a day with 0, 1.0, 3.0, or 7.0 mg MCT/kg of body weight (BW) by gavage, for 14 d. The initial mean rats body weights (± SEM) in each group were 202.6 ± 5.1, 202.5 ± 6.9, 210.5 ± 7.2, and 198.8 ± 6.6, respectively. On Day 14, all the rats were killed and the trachea of each was exposed and cannulated to perform a lung lavage with a total volume of 10 ml of phosphate-buffered saline (PBS; 8 mM Na2HPO4, 1.5 mM KH2PO4, 2.7 mM KCl, 0.14 M NaCl). The lavage fluid collected was centrifuged at 450 g (10 min, 4°C) and the pulmonary lactate dehydrogenase (LDH) levels of the supernatants were determined. In addition, representative samples of lung, heart, liver, and kidneys were harvested, fixed in 10% formalin, embedded in paraffin, sectioned at 5 μm thickness, and stained with hematoxylin and eosin (H&E) for histopathological examination.
Immunopathology (Study 1b)
Forty rats were divided into one control and three experimental groups that received 0, 0.3, 1.0, or 3.0 mg MCT/kg by gavage once a day for 14 d. Rats from the control group received only vehicle the same way over the same period. The initial mean rats body weights (± SEM) in each group were 243.2 ± 4.4, 245.4 ± 4.0, 242.8 ± 4.1, and 243.3 ± 3.6, respectively. At the end of the experimental period, the thymus and spleen were removed from euthanized rats and weighed. The spleen was disrupted with two pieces of ground glass and the red blood cells were removed by lysis, resulting in a single splenocyte suspension in cold RPMI-1640 culture medium. Bone marrow cell suspensions were achieved by flushing the marrow cavity of the left femur of each rat with ice-cold RPMI-1640 medium using a sterile syringe with a 26-gauge needle. Viability was assessed via a trypan blue dye exclusion test and cells were counted in a Neubauer chamber.
Each thymus was fixed in 10% buffered formalin, embedded in paraffin, sectioned at 5 μm thickness, and stained with H&E. Image Pro Software (Media Cybernetic, Bethesda, MD) was used for section analysis and the ratio of cortical to medullar areas (C/M) was determined and calculated by the formula C/M = Total cortex area/[(Total area) − (Total cortex area)].
Humoral immune response (Study 2a)
Forty rats were divided into four equal groups (one control and three experimental groups) that received 0, 0.3, 1.0, or 3.0 mg MCT/kg by gavage once a day for 14 d. On Day 7 of their regimens, subsets of rats (n = 10/treatment group) were immunized by intraperitoneal (IP) injection of 2.0 × 109 sheep red blood cell (SRBC) in 0.9% saline (normal saline). Seven days later, blood samples were harvested from the euthanized rats and were allowed to clot in a vertical tube at room temperature for 45 min and then centrifuged at 3200 g for 10 min. The serum was collected and anti-SRBC antibody titer was estimated as follows: 25 μl of two-fold diluted serum in normal saline was challenged with 25 μl of 1% (v/v) SRBC suspension in a microtiter plate. The plate was incubated at 37°C for 1 hr and then checked for hemagglutination. The highest dilution causing hemagglutination was taken as the antibody titer. Antibody titers were expressed in a graded manner, with the minimum dilution (i.e., 1/2) being ranked as “1” against which the mean ranks of different groups were compared.
A Plaque-Forming Cell assay (PFC) was also used to assess the status of each host’s humoral immune response. Briefly, each rat’s spleen used to generate a corresponding single cell suspension (107 cells/ml) in RPMI-1640 medium at 4°C. Splenocyte suspension, SRBC, and guinea pig complement were added to a 1.0 cm2 PFC well to achieve a final concentration of 2.0 × 106 splenocytes/ml, 7% SRBC, and 10% complement in a final volume of 50 μl; duplicate wells were prepared for each rat sample. The well was then covered with a glass slide (22 × 22 mm) and sealed with varnish. Each PFC well was incubated at 37°C for 3 hr before the number of lysate plaques produced by the 105 splenocytes in the well was counted under a light microscope (40X magnification). Data are expressed as total PFC/105 splenocytes.
Cellular immune response: DTH assay (Study 2b)
Forty rats were divided into four equal groups that received 0, 1.0, 3.0, or 5.0 mg MCT/kg by gavage once a day for 14 d. The initial mean rat body weights (± SEM) in each group were 232.5 ± 7.6, 233.7 ± 10.0, 233.2 ± 7.6, and 228.3 ± 8.6, respectively. Sensitization with keyhole limpet hemocyanin (KLH) was performed as described by Exon et al. (Citation1990). Briefly, KLH (5.0 mg/ml) was injected in the intracaudal tail fold (in 200 μl volume of sterile water). Primary and secondary sensitizations were performed on Days 1 and 7 of MCT treatment. At the end of the experimental period (Day 14), all the rats were challenged with heat-aggregated (80°C for 1 hr) 20 mg/ml KLH in 0.1 ml saline. The challenge antigen was injected into one footpad with the other footpad receiving sterile saline. Swelling was measured with a metric tape and calculated by subtracting the saline-injected left footpad thickness from the KLH-injected right footpad thickness. The swelling was then plotted as a percentage of left foot thickness.
Innate immunity: Macrophage activity (Study 3)
Forty rats were divided into four equal groups (one control and three experimental groups) that received 0, 1.0, 3.0, or 5.0 mg MCT/kg by gavage once a day for 14 d. The initial mean rat body weights and SEM in each group were 311.4 ± 9.3, 307.3 ± 8.8, 315.3 ± 6.6, and 312.3 ± 6.5, respectively. At the end of the experimental period, peritoneal macrophage activity (phagocytosis, hydrogen peroxide [H2O2], and nitric oxide [NO] production) was evaluated using the protocols previously described by Rabinovitch and Destefano (Citation1973a, Citationand b).
To evaluate phagocytosis by its peritoneal macrophages, at sacrifice, each rat’s peritoneal cavity was lavaged with cold (4°C) PBS. Cells in the fluid were isolated by centrifugation (450 × g, 4°C, 10 min) and then resuspended in RPMI medium at 2.0 × 106 cells/ml. To study macrophage phagocytosis, 200 μl of each suspension was placed (in duplicate) on a glass slide held in a 6-well tissue culture plate (Corning Costar, Corning, NY). Over a period of 20 min, the wells were washed several times with cold PBS; RPMI-1640-supplemented medium was added to each well, followed by 1.0 mg zymosan A solution (5.0 mg/ml). The culture plate was incubated for 60 min at 37°C in a humidified atmosphere containing 5% CO2. After incubation, each well was rinsed with cold PBS and the adherent cells were fixed in 0.5% glutaraldehyde for 10 min. The cells were then counted using a phase contrast microscope (Nikon Inc., Melville) at 40X magnification. Using an ocular grid, 200 macrophages were scored as round or spread. The phagocytosis index (PI) was then calculated as PI = 100 × (number of macrophages with phagocytic activity/200 adherent cells counted); i.e., PI = percentage of macrophages presenting phagocytized zymosan particles. The mean of four counts obtained from two slides from each rat was used to determine the PI.
Spontaneous and phorbol myristate acetate solution (PMA)-induced H2O2 release from these macrophages were measured by the method of Russo et al. (Citation1989). Briefly, peritoneal cells adjusted to 2.0 × 106 cells/ml were centrifuged for 10 min and resuspended in 1.0 ml phenol red solution (PRS; containing 140 mM NaCl, 10 mM potassium-phosphate buffer [pH 7.0], 0.5 mM dextrose, 0.28 mM phenol red, and 8.5 U/ml horseradish peroxidase for H2O2 detection). Aliquots (100 μl) of the suspension were then added to the wells of a 96-well flat-bottom microplate and incubated at 37°C for 1 hr in a humidified 5% CO2 atmosphere. Subsequently, wells containing PRS received 10 μl 1 N NaOH to stop the reaction and H2O2-dependent phenol red oxidation was measured at 620 nm using a Multiskan EX plate reader (Thermo Scientific, Milford, MA). The same procedure was used to determine H2O2 release after stimulation with PMA, with 10 μl of 10 ng/ml PMA added to each well prior to incubation. H2O2 levels were calculated as previously described (Pick and Mizel, Citation1981). Experiments involving spontaneous and PMA-induced H2O2 production were repeated four times for each rat.
The concentrations of NO in the supernatants of cultures of macrophages incubated with LPS (100 ng/ml) or vehicle for 24 hr were measured using the Griess reagent (Green et al., Citation1982). In brief, 100 μl of Griess reagent (freshly prepared) was mixed with an equal amount of cell culture supernatant and then incubated at room temperature for 10 min. The absorbance of the samples was then measured at 540 nm in the Multiskan EX reader. All experiments for NO measurements were performed in triplicate.
Statistical analyses
Data were analyzed by one-way analysis of variance (ANOVA), with post-hoc analysis using Dunnett’s test. Differences between the control and the experimental groups were considered to be statistically significant when p < 0.05. Data are presented as means ± standard error of mean (SEM). Bone marrow cellularity data was analyzed by Kruskal–Wallis analysis of variance for nonparametric data, followed by the Dunn test for multiple comparisons, with the level of significance set at p < 0.05 for all comparisons.
Results
Evaluation of food intake/body weight gain
In Study 1a, while it appeared there was a reduction in food consumption in rats treated with the higher MCT doses, their consumption levels were not significantly different from that of control rats (). Nevertheless, these rats displayed significantly diminished body weight gains relative to the controls. Diarrheic feces were only found in the cages of rats treated with 7.0 mg MCT/kg; no other clinical symptoms were manifest by these (or the other MCT-treated) rats. A somewhat similar pattern of results was noted with the rats in Study 2b except that here, the rats that were treated with 5.0 mg MCT/kg showed significant reductions in food consumption in conjunction with those in body weight gain (). It is only in Study 3 that rats in the 3.0 mg MCT/kg group displayed both of these significant reductions.
Table 1. Total food consumption, body weight gain, lactate dehydrogenase level and average severity of histological lesions of liver and kidney sections of rats treated with monocrotaline in different experimental designs.
MCT toxicity (Study 1a)
Analyses of the lungs from all rats showed that only those rats treated with the highest dose of MCT (i.e., 7.0 mg MCT/kg) had a significant elevation in LDH enzyme levels compared to the controls (). Histopathological evaluations revealed that only rats belonging to this group had morphological changes in their: lungs, i.e., elevated number of macrophages in alveolar lumen and alveolar epithelial Type II cell hyperplasia; liver, i.e., megalocytosis (but no other alterations); and, kidneys, i.e., sporadic congestion foci of mild-to-moderate intensity (). Even at this dose, no morphologic alterations were observed in the heart of each rat.
Figure 1. Tissue samples from rats treated with 7.0 mg MCT/kg by gavage daily for 14 days. (A) The pulmonary alveolar lumen reveals enhanced quantities of macrophages (arrows). (B) In the liver, an increased presence of megalocytosis within hepatocytes (arrows) was observed. Finally, in the renal samples (C), only a mild presence of diffused congestion is visible. Bar = 35 μm.
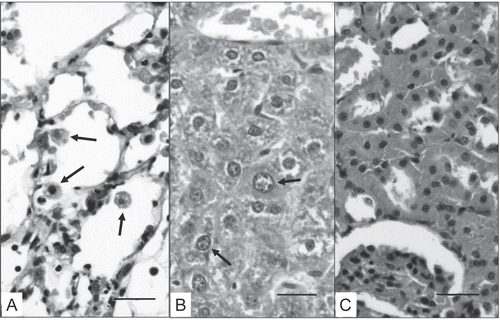
The immunopathology studies (Study 1b) revealed there was diminished bone marrow cellularity in the rats treated with 3.0 mg/kg of the alkaloid (). No other parameter analyzed, i.e., splenic and thymic indices, splenic cellularity, ratio of thymic cortical to medullar areas (C/M), was significantly affected by any of the MCT treatments.
Table 2. Thymic and splenic indices, thymus cortex/medulla ratio, spleen and bone marrow cellularity.
Evaluation of immune function: Humoral and cell-mediated immunity endpoints (Study 2)
In both assays used to assess humoral immune response (antibody titer measurement and the PFC assay), no statistically-significant differences were observed between the control rats and any of the rats treated with MCT for 14 d (). Similarly, when a dose as high as 5.0 mg MCT/kg was tested in a study to assess potential effects on one type of cell-mediated immune response (i.e., DTH), no statistically significant alteration in response due to treatment with MCT was observed.
Table 3. Anti-SRBC titers, plaque-forming cell (PFC) levels, and delayed type hypersensitivity (DTH) responses.
Evaluation of immune function: Innate immunity (Study 3)
When peritoneal macrophage activities were assessed, no significant effects from MCT upon phagocytic activity or H2O2 formation/release (spontaneous and PMA-induced) were observed (). Conversely, NO production was found to be significantly decreased in the cells from rats treated with both 1.0 and 5.0 mg MCT/kg doses. The NO level produced by cells from rats treated with the 3.0 mg MCT/kg dose were also reduced, but to a level that failed to reach statistical significance (p = 0.07). Though not statistically significant, after activation with LPS, NO production levels by the macrophages from all MCT-treated rats were still lower than those by activated macrophages from control rats.
Figure 2. Effects on (A) phagocytosis, (B) spontaneous and phorbol myristate-acetate-induced H2O2 release, and (C) spontaneous or lipopolysaccharide-induced nitric oxide production by resident peritoneal macrophages of rats treated with vehicle (control), 1.0; 3.0 or 5.0 mg/kg of MCT for 14 days (n = 10/group). Results represent means ± SEM. *p < 0.05; **p < 0.01 versus control group.
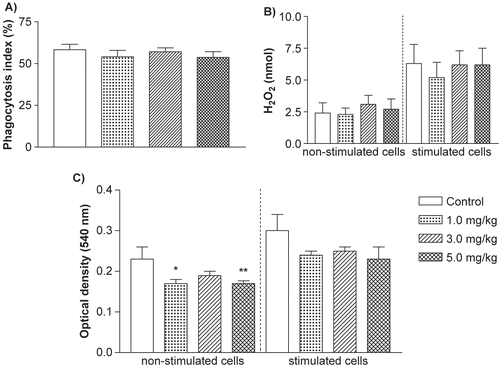
Discussion
When in vivo studies are performed regarding the toxic effect of xenobiotics on the immune system, it is important to verify whether the doses used in the experimental design do not cause exacerbated toxicity and/or clinical symptoms in the hosts. This is because it is well known that certain physiological disorders can promote immune alterations. For example, fever can cause stimulation of certain immune responses (Heron and Berg, Citation1978; Roberts, Citation1991) and stress can give rise to increased circulating levels of immunosuppressive (gluco)corticoids (Idova et al., Citation1997). Because pulmonary hypertension is a major toxic effect of MCT in rats (see early studies by Lalich and Merkow, Citation1961), it was critical that the MCT doses used here did not promote this deleterious effect that could, in turn, induce physiologic stress in the rats. When the toxicity of the different MCT doses used here was evaluated, it was seen that only rats that were treated with 7.0 mg MCT/kg showed any evidence of pulmonary disorder, i.e., high levels of LDH enzyme (a marker of pulmonary toxicity; Henderson et al., Citation1985) in conjunction with large quantities of macrophages in the alveolar lumen and hyperplasia of alveolar epithelial Type II cells. Based on these results, the use here of the other MCT doses (0.3 up to 5.0 mg/kg) to assess immune functions provided a measure of confidence that no indirect effects from hypertension would be a factor in any observed results.
As the immune system is also very sensitive to changes in the nutritional status of a host (Cunningham-Rundle et al., Citation2005), another concern in these studies would be any MCT-induced anorexic effect and consequent reductions in body weight or host weight gain rate. Although rats treated with 3.0 mg/kg of the alkaloid revealed reduced food consumption trends and significant decreases in body weight gain (relative to the control rats), none presented with symptoms of MCT toxicosis, histological lesions, increases in pulmonary LDH levels, or any modification in the specific humoral and cell-mediated immune responses analyzed. In addition, because: the DTH response was not seen to be affected even at an MCT dose that clearly led to significant reductions in both feed consumption and weight gain; effects on macrophage functions were evident even at MCT doses where no effects on those parameters were evident; and, the point at which significant effects on macrophage function (specifically, NO formation) and bone marrow cellularity were apparent occurred when levels of net change/loss of body weight (i.e., − 1.24% ± 1.15 and − 5.14% ± 0.59 (mean across all studies [± SEM]) for 1.0 and 3.0 mg MCT/kg, respectively) were still far below that level (i.e., − 17%) shown to be associated with significant changes in both endpoints in a study of the immunomodulatory effects of induced protein/caloric malnutrition (Ruffmann et al., Citation1985, Citation1987), we are confident that each of the effects observed here was primarily due to factors other than host nutritional status on Day 14 of the regimens.
The finding of induced alterations in bone marrow cellularity in rats that received the 3.0 mg MCT/kg dose is not completely unexpected. The biotransformation of MCT by hepatic microsomal P450 3A (Kasahara et al., Citation1997) into the highly reactive metabolite MCTP results in increased DNA binding, DNA cross-linking, and DNA-protein crosslinking events (Petry et al., Citation1984; Hincks et al., Citation1991; Kim et al., Citation1993; Pereira et al., Citation1998; Fu et al., Citation2001), all of which can readily lead to a cell attaining an anti-mitotic state. This anti-mitotic property of MCTP is shared by the pyrrole metabolites of other pyrrolizidine alkaloids such as indicine-N-oxide (contained in Heliotropium indicum L. [a plant in Boraginaceae family]) that is administered to patients with leukemia and solid tumors (Poster et al., Citation1981; Whitehead et al., Citation1990).
Like other anticancer medications, this alkaloid presents side effects typical of chemotherapeutics, including myelosuppression (Letendre, Citation1981; Lee et al., Citation2005). Thus, it is plausible to hypothesize that MCT, like indicine-N-oxide, promotes diminished bone marrow cellularity and that this myelotoxic effect might be relevant to induction of immunomodulation in MCT-treated hosts. In this context, during an immune response that requires substantial rates of lymphoid cell proliferative activity, administration of MCT should promote immunosuppression due, in part, to the anti-mitotic effect.
However, when antibody anti-SRBC responses were evaluated in the current studies, both by assessment of serum titers (antibody formation against SRBC antigen) and the PFC assay, no immunotoxic effect was observed for MCT. Nevertheless, discarding possible effects of this alkaloid on humoral immunity at this time could be a mistake. In the experimental design used here, only a single SRBC sensitization was performed in the middle of the experimental period and the antibody anti-SRBC (IgM isotype) levels were assessed 7 d later. Considering that a single exposure to SRBC would not promote a high rate of proliferation among T- and B-lymphocytes participating in this type of immune response, it is possible to infer that the impact of the anti-mitotic effect of MCT could not be detected by these assays as currently designed. Future experiments are required to clearly verify whether the anti-mitotic effects of MCT interfere in secondary humoral immune responses as opposed to primary ones only.
The results of the studies of the effects of MCT on the functionality of host macrophages after MCT treatments were somewhat contradictory. When phagocytosis and hydrogen peroxide production by peritoneal macrophages from the alkaloid-treated rats were studied, it was seen that MCT treatment yielded no toxicity with regard to these parameters. The finding that phagocytic activity was unimpeded helps explain why there was little effect from the alkaloid on the DTH, PFC, and anti-SRBC antibody formation endpoints. If this function had been significantly impacted, then processing of the SRBC and/or the KLH would have been modified, and each of those endpoints modulated in turn.
In contrast to those two parameters, when NO release by the macrophages was measured, a reduction in NO production—both spontaneous and induced by LPS—was noted. In a study performed by Sasaki et al. (Citation2007), pulmonary artery endothelial cells of rats exposed to MCT were shown to be incapable of producing NO due to a decrease in L-arginine availability (secondary to accelerated L-arginase activity). In addition, these investigators noted an elevation in levels of cytoplasmic methylarginine that competes with L-arginase (by eNOS), thereby synergistically interfering in pulmonary NO depletion.
Taking these data in total, it is reasonable to hypothesize that peritoneal macrophages, known to possess iNOS to synthesize NO (Tapiero et al., Citation2002), also had an enhanced arginase enzymatic activity (like the pulmonary endothelial cells) and, possibly, elevated levels of cyto-plasmic methylarginine, each of which interfered in the NO production by these inflammatory cells. Ongoing studies are attempting to verify whether MCT induced increased levels of these two substances in the MCT-exposed rats. Nonetheless, to our knowledge, the results obtained in the present work are the first to highlight that the NO production capacity of macrophages in rats exposed to MCT was suppressed. As it is well known that NO plays an important role in inflammatory processes, including initiating vasodilatation, this inhibiting effect could be a critical factor underlying the increased risk of pulmonary hypertension and aberrant inflam-matory responses in MCT-exposed hosts.
In conclusion, the present work using rats as a model to evaluate MCT immunotoxicity, showed that low doses of the alkaloid gave rise to myelotoxic effects and interference in the formation of at least one product critical to the inflammatory process. Future experiments will be conducted to more clearly determine which bone marrow cell lines are affected by MCT, the roles of iNOS and NO in the inflammatory process, and whether effects of this compound on alveolar macrophages could be a factor in the pulmonary hypertension known to be induced by this alkaloid.
Acknowledgment
Declaration of interest: The authors report no conflicts of interest.
References
- Bokhtiar, S. M., Gafur, M. A., and Rahman, A. B. 2003. Effects of Crotalaria and Sesbania aculeata green manures and N-fertilizer on soil fertility and the productivity of sugarcane. J. Agric. Sci. 140:305–309.
- Byron, J. 1998. Pyrrolizidine alkaloids in eggs: New alkaloid found in potatoes. Food Chem. News 14:6–7.
- Cheeke, P. R. (Ed.) 1998. Natural Toxicants in Feeds, Forages and Poisonous Plants. Danville: Interestate Publishers, pp. 479.
- Chesney, C. F., Allen, J. R., and Hsu, I. C. 1974. Right ventricular hypertrophy in monocrotaline pyrrole-treated rats. Exp. Mol. Pathol. 20:257–268.
- Culvenor, C. C., and Smith, L. W. 1963. Alkaloids of Crotalaria crispara F. Muell. ex Benth., the structures of cripatine and fulvine. Aust. J. Chem. 16:239–245.
- Cunningham-Rundles, S., McNeeley, D. F., and Moon, A. 2005. Mechanisms of nutrient modulation of the immune response. J. Allergy Clin. Immunol. 115:1119–1128.
- Eloff, J. N., Jansen Van Rijssen, F. W., Naude, T. W., and Joubert, J. P. J. 2003. What levels of potentially toxic seed should be allowed in grains in South Africa? I. Background and pyrrolizidine alkaloid-containing plants. S. Afr. J. Sci. 99:337–344.
- Exon, J. H., Bussiere, J. L., and Mather, G. G. 1990. Immunotoxicity testing in the rat: An improved multiple assay model. Int. J. Immunopharmacol. 12:699–701.
- Figueredo, M. L. A., Rodriguez, J., and Alfonso, H. A. 1987. Pathology of experimental acute intoxication of Crotalaria retusa and Crotalaria spectabillis in chickens. Rev. Cubana Ciências Vet. 18:63–71.
- Fu, P. P., Chou, M. W., Xia, Q., Yang, Y. C., Yan, J., Doerge, D. R., and Chan, P. C. 2001. Genotoxic pyrrolizidine alkaloids and pyrrolizidine alkaloid N-oxides mechanisms leading to DNA adduct formation and tumorigenicity. Environ. Carcinog. Ecotoxicol. Rev. 19:353–386.
- Green, L. C., Wagner, D. A., Glogowski, J., Skipper, P. L., Wishnok, J. S., and Tanneubaum, S. R. 1982. Analysis of nitrate, nitrite and [15N] nitrite in biological fluids. Anal. Biochem. 126:131–138.
- Henderson, R. F., Benson, J. M., Hahn, F. F., Hobbs, C. H., Jones, R. K., Mauderly, J. L., McClellan, R. O., and Plckrell, J. A. 1985. New approaches for evaluation of pulmonary toxicity: Bronchoalveolar lavage fluid analysis. Fundam. Appl. Toxicol. 5:451–458.
- Heron, I., and Berg, K. 1978. The actions of interferon are potentiated at elevated temperature. Nature 274:508–510.
- Hincks, J. R., Kim, H. Y., Segall, H. J., Molyneux, R. J., Stermitz, F. R., and Coulombe, R. A. 1991. DNA cross-linking in mammalian cells by pyrrolizidine alkaloids: Structure–activity relationships. Toxicol. Appl. Pharmacol. 111:90–98.
- Huxtable, R. J. 1990. Activation and pulmonary toxicity of pyrrolizidine alkaloids. Pharmacol. Ther. 47:371–389.
- Idova, G., Cheido, M., and Devoino, L. 1997. Modulation of the immune response by changing neuromediator systems activity under stress. Int. J. Immunopharmacol. 19:535–540.
- Kasahara, Y., Kiyatake, K., Tatsumi, K., Sugito, K., Kakusaka, I., Yamagata, S. I., Ohmori, S., and Kuriyama, T. 1997. Bioactivation of monocrotaline by P450 3A in rat liver. J. Cardiovasc. Pharmacol. 30:124–129.
- Kim, H. Y., Stermitz, F. R., Molyneux, R. J., Wilson, D. W., Taylor, D., and Coulombe, R. A. 1993. Structural influences on pyrrolizidine alkaloid-induced cytopathology. Toxicol. Appl. Pharmacol. 122:61–69.
- Lalich, J. J., and Merkow, L. 1961. Pulmonary arteritis produced in rats by feeding Crotolaria spectabilis. Lab. Invest. 10:744–750.
- Lee, D. P., Skolnik, J. M., and Adamson, P. C. 2005. Pediatric phase I trials in oncology: An analysis of study conduct efficiency. J. Clin. Oncol. 23:8431–8441.
- Letendre, L., Smithson, W. A., and Gilchrist, G. S. 1981. Activity of indicine N-oxide in refractory acute leukemia. Cancer 47:437–441.
- Molyneux, R. J., and Roitman, J. N. 1980. Specific detection of pyrrolizidine alkaloids on thin-layer chromatograms. J. Chromatog. 195:412–415.
- Molyneux, R. J., Roitman, J. N., Benson, M., and Lundin, R. E. 1982. [13C]-NMR spectroscopy of pyrrolizidine alkaloids. Phytochemistry 21:439–443.
- Mukhopadhyay, S., Shah, M., Patel, K., and Sehgal, P. B. 2006. Monocrotaline pyrrole-induced megalocytosis of lung and breast epithelial cells: Disruption of plasma membrane and Golgi dynamics and an enhanced unfolded protein response. Toxicol. Appl. Pharmacol. 211:209–220.
- Niwa, H., Ogawa, T., Okamoto, O., and Yamada, K. 1991. Alkylation of nucleosides by dehydromonocrotaline, the putative toxic metabolite of the carcinogenic pyrrolizidine alkaloid monocrotaline. Tetrahedron Lett. 32:927–930.
- Nobre, D., Dagli, M. L., and Haraguchi, M. 1994. Crotalaria juncea intoxication in horses. Vet. Human Toxicol. 36:445–448.
- Pan, L. C., Wilson, D. W., Lame, M. W., Jones, A. D., and Segall, H. J. 1993. Cor pulmonale is caused by monocrotaline and dehydromonocrotaline, but not by glutathione or cysteine conjugates of dihydropyrrolizine. Toxicol. Appl. Pharmacol. 118:87–97.
- Pereira, T. N., Webb, R. I., Reilly, P. E., Seawright, A. A., and Prakash, A. S. 1998. Dehydromonocrotaline generates sequence-selective N-7 guanine alkylation and heat and alkali stable multiple fragment DNA crosslinks. Nucleic Acids Res. 26:5441–5447.
- Petry, T. W., Bowden, G. T., Huxtable, R. J., and Sipes, I. G. 1984. Characterization of hepatic DNA damage induced in rats by the pyrrolizidine alkaloid monocrotaline. Cancer Res. 44:1505–1509.
- Pick, E., and Mizel, D. 1981. Rapid microassays for the measurement of superoxide and hydrogen peroxide production by macrophages in culture sing an automatic enzyme immunoassay. J. Immunol. Meth. 46:211–226.
- Poster, D. S., Bruno, S., Penta, J., and MacDonald, J. S. 1981. Indicine-N-oxide: A new anti-tumor agent. Cancer Treat. Rep. 65:1–2.
- Prakash, A. S., Pereira, T. N., Reilly, P. E., and Seawright, A. A. 1999. Pyrrolizidine alkaloids in human diet. Mutat. Res. 443:53–67.
- Rabinovitch, M., and Destefano, M. J. 1973a. Macrophage spreading in vitro: I. Inducers of spreading. Exp. Cell Res. 77:323–334.
- Rabinovitch, M., and Destefano, M. J. 1973b. Macrophage spreading in vitro: II. Manganese and other metals as inducers or as co-factors for induced spreading. Exp. Cell Res. 79:423–430.
- Roberts, N. J., Jr. 1991. Impact of temperature elevation on immunologic defenses. Rev. Infect. Dis. 13:462–472.
- Roeder, E. 1995. Medicinal plants in Europe containing pyrrolizidine alkaloids. Pharmazie 50:83–98.
- Ruffmann, R., Schlick, E., Tartaris, T., Budzynski, W., and Chirigos, M. A. 1987. Protein deficiency reduces natural anti-tumor immunity. Cancer Detect. Prevent. Suppl. 1:15–27.
- Ruffmann, R., Schlick, E., Tartaris, T., Gruys, E., Welker, R. D., Saito, T., and Chirigos, M. A. 1985. Effects of protein depletion on NK cell cytotoxicity and bone marrow cellularity. Med. Oncol. Tumor Pharmacother. 2:195–202.
- Russo, M., Teixeira, H. C., Marcondes, M. C., and Barbuto, J. A. 1989. Superoxide independent hydrogen peroxide release by activated macrophages. Braz. J. Med. Biol. Res. 22:1271–1273.
- Sasaki, A., Doi, S., Mizutani, S., and Azuma, H. 2007. Roles of accumulated endogenous nitric oxide synthase inhibitors, enhanced arginase activity, and attenuated nitric oxide synthase activity in endothelial cells for pulmonary hypertension in rats. Am. J. Physiol. Lung Cell Mol. Physiol. 292:L1480–1487.
- Shah, M., Patel, K., and Sehgal, P. B. 2005. Monocrotaline pyrrole-induced endothelial cell megalocytosis involves a Golgi blockade mechanism. Am. J. Physiol. Cell Physiol. 288:850–862.
- Srivastava, R. N., and Mohabbat, O. 1977. Veno-occlusive disease of the liver. Am. Heart J. 94:665.
- Steenkamp, V., Stewart, M. J., and Zuckerman, M. 2000. Clinical and analytical aspects of pyrrolizidine poisoning caused by South African traditional medicines. Ther. Drug Monit. 22:302–306.
- Tapiero, H., Mathe, G., Couvreur, P., and Tew, K. D. 2002. I Arginine. Biomed. Pharmacother. 56:439–445.
- Wang, Y. P., Yan, J., Beger, R. D., Fu, P. P., and Chou, M. W. 2005. Metabolic activation of the tumorigenic pyrrolizidine alkaloid, monocrotaline, leading to DNA adduct formation in vivo. Cancer Lett. 226:27–35.
- Wilson, D. W., Segall, H. J., Pan, L. C., Lame, M. W., Estep, J. E., and Morin, D. 1992. Mechanisms and pathology of monocrotaline pulmonary toxicity. Crit. Rev. Toxicol. 22:307–325.
- Whitehead, V. M., Bernstein, M. L., Vega, R., Vats, T., Dyment, P., Vietti, T. J., and Krischer, J. 1990. Phase I trial of indicine-N-oxide in children with leukemia and solid tumors: A Pediatric Oncology Group study. Cancer Chemother. Pharmacol. 26:377–379.