Abstract
Eosinophilic inflammation is frequently observed in response to nanoparticle (NP) exposure in airway rodent models of allergies where the number of eosinophils is increased in lungs. Despite this, it is surprising that the potential cytotoxic effect of NP, as well as their direct role on eosinophils is poorly documented. The present study investigated how different NP can alter the biology of the human eosinophilic cell line AML14.3D10. It was found that among NP forms of CeO2, ZnO, TiO2, and nanosilver of 20 nm (AgNP20) or 70 nm (AgNP70) diameters, only ZnO and AgNP20 induced apoptosis. Caspases-7 and -9 were not activated by the tested NP while caspase-3 was activated by AgNP20 only. However, both ZnO and AgNP20 induced cytoskeletal breakdown as evidenced by the cleavage of lamin B1. Using an ELISArray approach for the simultaneous detection of several analytes (cytokines/chemokines), it was found that only ZnO and AgNP20 increased the production of different analytes including the potent pro-inflammatory CXCL8 (IL-8) chemokine. From the data here, we conclude that toxic effects of some NP could be observed in human eosinophil-like cells and that this could be related, at least partially, by induction of apoptosis and production of cytokines and chemokines involved in inflammation. The results of this study also indicate that distinct NP do not activate similarly human eosinophils, since ZnO and AgNP20 induce apoptosis and cytokine production while others such as TiO2, CeO2, and AgNP70 do not.
Introduction
The use of engineered nanoparticles (NP) in a variety of sectors, including electronics, biomedical sciences, pharmaceutical industry, cosmetics, etc., is in rapid expansion. Consequently, the probability of human exposure to NP has risen (Oberdorster et al. Citation2005). Because NP could enter human body via inhalation, ingestion, and dermal exposure, they can reach the blood circulation and come in close contact with immune cells. Therefore, it is crucial to evaluate the risk that NP may represent to humans.
One of the major toxic effects observed following NP exposure in a variety of in vitro and in vivo models is inflammation, based especially on two criteria: (i) an increased production of cytokines in in vitro and in vivo models of inflammation; and (ii) an increased number of granulocytes observed in lungs and/or bronchoalveolar lavages following administration of NP via inhalation, intratracheal instillation, nebulization, etc. (Chen et al. Citation2006, Citation2011; Cho et al. Citation2010; Rossi et al. Citation2010; Srinivas et al. Citation2011; Sahu et al. Citation2013). Of note, an increase number of granulocyte eosinophils are especially observed in response to NP exposure in airway rodent models of allergy (Cho et al. Citation2010; Larsen et al. Citation2010; Brandenberger et al. Citation2013; Roy et al. 2013).
Despite the fact that high number of neutrophils and/or eosinophils is observed in several studies following NP exposure, the role of NP in the biology of human neutrophils and eosinophil is poorly understood. However, in the past few years, direct interactions between NP and human neutrophils have been investigated, leading to the observation that several neutrophil responses can be altered in response to NP exposure. These include respiratory burst, degranulation, cytokine production, and apoptosis (Goncalves et al. Citation2010; Abrikossova et al. Citation2012; Babin et al. Citation2013; Poirier et al. Citation2014). Unlike neutrophils, there is presently no study reporting the direct roles of NP on human eosinophils. Accordingly, the present study utilized the human eosinophilic cell line AML14.3D10, a good model for studying eosinophil cell biology or at least to obtain information on cancerous cells of eosinophilic origin (Baumann & Paul Citation1998), to investigate effects of a panel of well-characterized NP. Among the NP agents analyzed were titanium dioxide (TiO2), cerium oxide (CeO2), zinc oxide (ZnO), as well as nanosilver of initial diameter of 20 nm (AgNP20) or 70 nm (AgNP70) for their effects on apoptosis and cytokine production by the AML14.3D10 cells.
Materials and methods
Nanoparticles, chemicals, agonists, and antibodies
TiO2, CeO2, and ZnO NP were obtained from Vivenano (Toronto, ONT, Canada). TiO2 anatase crystals, in aqueous suspension stabilized by polyacrylate sodium (≤ 10-nm particle size, 90%); metal content: 97% TiO2 determined by inductively coupled plasma (ICP); cerium oxide (CeO2) water-dispersible and non-aggregating (crystalline CeO2) in aqueous suspension; carboxy-functionalized and stabilized by sodium polyacrylate, ≤ 10 nm particle size, 90%) with a metal content of 90% Ce/10% Pr as determined by (ICP) and ZnO water-dispersible and non-aggregating ZnO (crystalline ZnO) carboxy-functionalized in aqueous suspension stabilized by sodium polyacrylate (≤ 20 nm particle size, 90%) with metal content of 90% Zn as determined by (ICP).
RPMI 1640, HEPES, and penicillin/streptomycin (P/S) were purchased from Gibco BRL (Grand Island, NY). Bovine serum albumin (BSA), Viscum album agglutinin-1 (VAA-I), and monoclonal antibodies to human cytoskeletal proteins (anti-gelsolin antibody, clone GS-2C4 and anti-vinculin, clone Vin 11-5) were purchased from Sigma (St. Louis, MO). The silver NP of 20 nm or 70 nm of diameter, i.e., AgNP20 and AgNP70, (Pelco® citrate Biopure™ silver) were purchased from Ted Pella (Redding, CA). Anti-vimentin (clone V9), polyclonal anti-lamin B1, and rabbit polyclonal anti-GAPDH antibody were from Santa Cruz Biotechnologies (Santa Cruz, CA). Horseradish peroxidase (HRP)-conjugated goat anti-mouse and goat anti-rabbit antibodies were purchased from Jackson Immunoresearch (West Grove, PA). Mouse mAb anti-caspase-3 (3G2), anti-caspase-7 (C7), and anti-capase-9 (C9) were purchased from Cell Signaling (Danvers, MA).
Size distribution and zeta potential measurements
The size distribution and the surface charge (zeta potential) of NP were determined by dynamic light scattering using a Malvern Zetasizer Nano-ZS (model ZEN3600 from Malvern Instruments Inc. (Westborough, MA). Measurements were performed in the same experimental conditions that NP were used in vitro: at 37 °C and at a concentration of 100 μg NP/ml in RPMI-1640 medium supplemented with 10% fetal calf serum and 1% penicillin– streptomycin (Gibco) with 1 mM sodium pyruvate. Before performing the analysis, the sterility of all NP used was checked.
Since the classical Limulus amebocyte lysate assay used to determine endotoxin level is a method in which NP could interfere (Neun & Dobrovolskaia Citation2011), sterility was instead tested as previously published (Goncalves & Girard Citation2013). In brief, NP suspensions were incubated in Lysogeny broth agar plates for 48 h and the plates then observed for the presence or absence of colonies. As a positive control, a suspension of intentionally contaminated TiO2 NP (strictly reserved to perform this kind of analysis) was used. In contrast to the positive control, all the NP used in this study appears to be negative for endotoxin in the limit of this approach, since no colonies were observed (data not shown).
Cell line
Human eosinophilic AML14.3D10 cells (referred to as 3D10 cells hereafter) were generated and kindly provided by Drs. C. C. Paul and M. Baumann (Wright State University, Dayton, OH) (Baumann & Paul Citation1998). The non-adherent 3D10 cells were cultured at 37 °C in a 5% CO2 atmosphere in the same buffer as above. Cells were cultivated at a density between 2 × 105 and 1 × 106/ml, and the medium was changed three times per week. Before performing the different experiments, the cell viability was systematically verified by trypan blue exclusion and was found to routinely be >98%.
Cell viability and apoptosis determination
3D10 cells were incubated for up to 24 h in the presence or absence of 100 μg/ml of TiO2, CeO2, ZnO, AgNP20, or AgNP70 and cell viability was then determined by trypan blue exclusion at 6, 12, 18, and 24 h. The concentration of 100 μg/ml was selected based on previous studies performed with human granulocytes (Goncalves et al. Citation2010; Babin et al. Citation2013; Goncalves & Girard Citation2014; Poirier et al. Citation2014, Citation2016). For apoptosis, cells were incubated for 24 h and apoptosis then evaluated by flow cytometry by counting the numbers of FITC-Annexin-V-positive cells. In some experiments, apoptosis was confirmed by cytology from cyto-centrifuged sample preparations of cells using a Cytotek® centrifuge (Miles Scientific, Naperville, IL) and processed as documented previously (Lavastre et al. Citation2005). Cells were examined by light microscopy at 400× final magnification.
Caspase activation et cytoskeletal protein expression
Cells (106 cells/ml) were incubated in the presence or absence of buffer, VAA-I, or the test NP for 24 h and the expression of caspase and cytoskeletal proteins were then evaluated by Western blot as published previously (Lavastre et al. Citation2002). In brief, cells were harvested for the preparation of cell lysates in 1× Laemmli sample buffer. Aliquots corresponding to 106 cells were loaded onto 10% sodium dodecyl sulfate-polyacrylamide gels, electrophoresed, and then their proteins electrotransferred to polyvinylidene difluoride membranes. Non-specific binding sites on the membranes were blocked with blocking solution (5% BSA in Tris-buffered saline-Tween [25 mM Tris-HCl (pH 7.8), 190 mM NaCl, 0.15% Tween-20]) for 1 h at room temperature. Membranes were then washed and incubated with anti-human pro-caspase or cyto-skeletal antibodies (all at 1:1000) overnight at 4 °C. After several washes with Tris-buffered saline-0.1% Tween 20, the membranes were incubated with HRP-labeled goat anti-mouse IgG antibodies (1:20 000) or HRP-labeled goat anti-rabbit IgG antibody (1:20 000) for 1 h at room temperature in blocking solution. At the end of the experiments, the membranes were stripped and re-probed with anti-GAPDH antibody (1:500) to verify equal protein loading. In all cases, bands were revealed with an enhanced chemiluminescence-Western blotting detection system and assessed in a ChemidocTM MP imaging system (both Bio-Rad, Hercules, CA).
Cytokine and chemokine detection
Supernatants from four different batches of 3D10 cells treated separately were harvested and pooled for the simultaneous detection of several cytokines and chemokines using two Multi-Analyte ELISArrays (Qiagen Inc.-Canada, Toronto, Canada). The first kit allowed for simultaneous detection of cytokines and chemokines including interleukin (IL)-1α, IL-1β, IL-2, IL-4, IL-6, IL-8, IL-10, IL-12, IL-17A, interferon (IFN)-γ, tumor necrosis factor (TNF)-α, and GM-CSF. The second kit allowed for detection of chemokines IL-8, MCP-1, RANTES, MIP-1α, MIP-1β, IP-10, I-TAC, MIG, Eotaxin, TARC, MDC, and GR α. Only IL-8 was common to both kits. The measurement of IL-8 production was also determined using a commercially available ELISA kit (Invitrogen Canada Inc., Toronto, Canada) as per the instructions of the manufacturer. The level of sensitivity of the kit was < 5 pg IL-8/ml.
Statistical analysis
A one-way analyses of variance (ANOVA) followed by Dunnett's test was used to evaluate for significance differences between controls and each sample. Statistical analyses were performed using Prism for Windows (v5.0, GraphPad Software, San Diego, CA). Statistical significance was set at p < 0.05.
Results
Characterization of NP
As indicated in , the size distribution of AgNP20 and AgNP70 was higher than the starting material with a proportion of ≈100% of the NP suspensions with a mean diameter of 158.9 ± 6.2 nm and 113.2 ± 2.6% nm, respectively. The presence of large aggregates was noted for CeO2, ZnO, and TiO2 NP suspensions. The zeta potential varies among the NP where the values (mean ± SEM, n = 3) were −10.1 ± 0.5, −9.2 ± 1.2, −11.0 ± 0.5, 0.01 ± 1.20, and −12.1 ± 0.8 mV for AgNP20, AgNP70, CeO2, ZnO, and TiO2, respectively.
Table 1. Characterization of the NP used in this studyTable Footnotea.
Evaluation of cell viability and apoptosis in human 3D10 eosinophil cells in response to NP
illustrates that none of the tested NP induced necrosis in > ≈17% of cells after 6, 12, 18, or 24 h as well as the potent pro-apoptotic plant lectin VAA-I (VAA). Thus, the tested NP did not appear more cytotoxic than VAA-I. Because apoptotic cells exclude trypan blue and are, therefore, considered viable, apoptosis was next determined. As illustrated in , ZnO and AgNP20 were found to significantly increase apoptosis from 5.7 ± 0.7% (mean ± SEM, n = 4 different cell batches) to 11.5 ± 0.5% and to 15.9 ± 0.6%, respectively, according to the determination of FITC-Annexin-V+ cells. Of note, VAA-I also significantly increased apoptosis to a level of 54.9 ± 1.3%. These results were confirmed by cytology in two separate experiments where, among the tested NP, only ZnO and AgNP20 were found to induce apoptosis ().
Figure 1. Evaluation of cell viability and apoptosis in human 3D10 eosinophil cells in response to nanoparticles. 3D10 eosinophil cells (1 × 106 cells/ml, 2 ml/well) were incubated in the presence of buffer (Ctrl), 1 μg/ml of the pro-apoptotic plant lectin VAA-I (VAA) or 100 μg/ml of the indicated NP for 6-24 h (A) or 24 h (B and C) and cell viability (A) was assessed by trypan blue exclusion assay and apoptosis was evaluated by flow cytometry (B) or cytology (C). (A) Two independent kinetic experiments are illustrated. (B) Results are means ± SEM (n = 4). (C) Results are from two separate experiments (Exp. 1 and Exp. 2). Inset, typical images illustrating that most of the cells are normal in Ctrl when compared with incubation with VAA where the vast majority of cells are apoptotic (arrows). *p < 0.05 versus control (Ctrl).
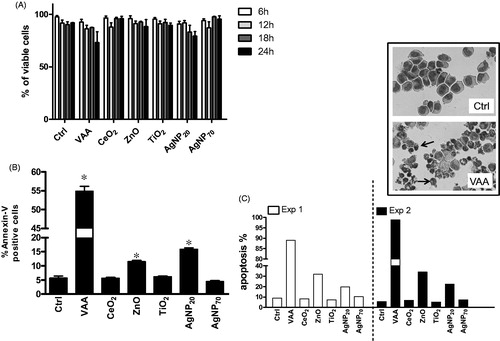
Caspase activation in NP-treated 3D10 cells
The study next determined whether or not both ZnO and AgNP20 could induce processing of pro-caspases-3, -7, and -9 in comparison with VAA-I known to induce apoptosis in these cells by a caspase-dependent mechanism (Lavastre et al. Citation2005). In contrast to VAA-I that markedly induced pro-caspase-3 processing, AgNP20 but not ZnO slightly-to-moderately activated caspase-3 (). The other NP tested did not alter the basal level of pro-caspase-3. Similar results were observed for casp-7, a closely caspase-3-related caspase. Caspase-9, highly expressed in 3D10 cells, was found to be activated by VAA-I as evidenced by its cleavage while ZnO and AgNP20 did not, or minimally activate it, respectively.
Figure 2. Effect of NP on caspase activation. (A) 3D10 eosinophil cells (1 × 106 cells/ml; 2 ml/well) were incubated in the presence of buffer (ctrl), 1 μg/ml VAA-I (VAA) or with 100 μg/ml of the indicated NP for 24 h and expression of pro-caspase-3, 7 or 9 was then assessed by Western blot (see Materials and methods section). Membranes were then stripped and re-probed with an anti-GAPDH antibody to verify protein loading. Results shown are from one representative experiment out of at least three. (B) Corresponding densitometry.
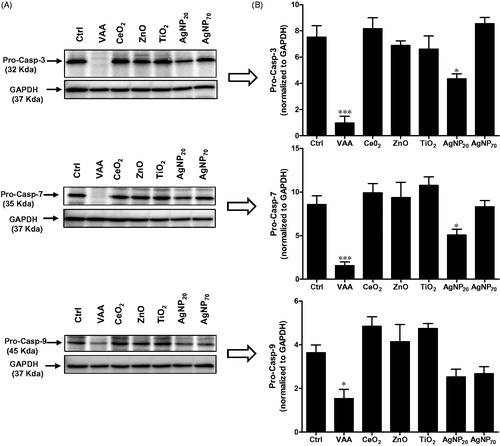
ZnO and AgNP20 induce degradation of cytoskeletal lamin B1 protein in 3D10 cells
Knowing that ZnO and AgNP20 induced apoptosis but only minimally caspases, the study attempted to verify if these NP would alter the cytoskeleton, expecting they would not. Unexpectedly, both ZnO and AgNP20, but not the other NP, were found to cleave lamin B1 onto the characteristic p46 fragment (). As expected, VAA-I was found to markedly cleave lamin B1 to the p46 fragment. However, unlike VAA-I, none of the tested NP were found to induce degradation of vimentin. Gelsolin was only minimally cleaved by ZnO when compared to VAA-I. Vinculin was also not cleaved by any of the test NP.
Figure 3. Expression of cytoskeletal proteins in response to NP. (A) 3D10 eosinophil cells (1 × 106 cells/ml; 2 ml/well) were incubated for 24 h in the presence of buffer (Ctrl), 1 μg/ml VAA-I (VAA), or with 100 μg/ml of the indicated NP and the expression of vimentin, lamin B1, gelsolin, and vinculin was then determined by Western blot analyses. Membranes were then stripped and re-probed with an anti-GAPDH antibody to verify protein loading. Results shown are from one representative experiment out of at least two. (B) Corresponding densitometry.
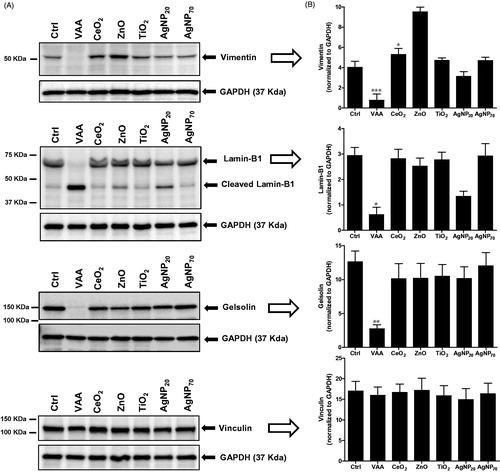
Production of cytokines and chemokines in NP-treated 3D10 cells
illustrates the results obtained (with first kit) that showed that production of different cytokines was increased when compared with controls only with ZnO or AgNP20. More precisely, ZnO increased 3D10 cell production of IL-8, MCP-4, RANTES, MIP-1α, MIP-1β, IP-10, and ITAC while AgNP20 also increased, but to a lesser extent, production of IL-8, RANTES, MIP-1α, MIP-1β, and IP-10, but not of MCP-4, IP-10, or ITAC. The second kit allowed detection of other analytes, but IL-8 was also included in this kit and the results testified to reproducibility of the technique (see IL-8 in ). Of note, only the production of TNFα was increased by treatment with ZnO or AgNP20. Production of all other analytes evaluated was not affected by any of the test NP.
Figure 4. Cytokine and chemokine production in NP-induced eosinophils. 3D10 eosinophil cells (1 × 106 cells/ml; 2 ml/well) were incubated for 24 h in the presence of buffer or with 100 μg/ml of the indicated NP and the supernatants from different cell batches were then pooled and used for the antibody array assay to detect production of indicated cytokines/chemokines. CTRL-and CTRL+ are, respectively, technical negative and positive controls included in the kit.
ZnO and AgNP20 increase the production of IL-8
To confirm the results obtained using the ELISarrays, and since IL-8 is an important pro-inflammatory molecule, amounts of IL-8 released in the supernatants of NP-treated 3D10 cells were evaluated by ELISA. As shown in , only ZnO and AgNP20 significantly increased the production of IL-8 from 18.5 ± 7.5 (mean ± SEM, n = 4) for control cells to 2084 ± 245 or 252 ± 47 pg/ml, respectively, for cells treated with ZnO or AgNP20. Treatment with the other NP never gave rise to levels that exceeded 27 pg/ml.
Figure 5. ZnO and AgNP20 induce IL-8 production in eosinophils. 3D10 eosinophil cells (1 × 106 cells/ml; 2 ml/well) were incubated in the presence of buffer (Ctrl), 1 μg/ml VAA-I (VAA) or with 100 μg/ml of the indicated NP and supernatants were tested by ELISA to quantify the production of IL-8. *p < 0.05 versus control (Ctrl).
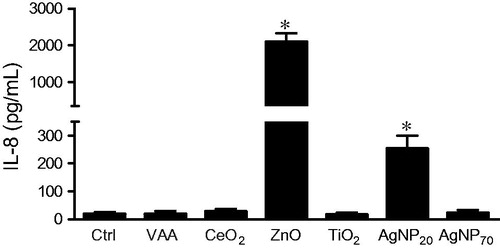
Discussion
The results of the present study indicated that the biology of human eosinophil-like 3D10 cells could be altered by NP. To the best of our knowledge, this is the first study investigating interactions between NP and human cells of eosinophilic origin. Accumulation of eosinophils is a common feature of allergic airway inflammation and there are evidences for a role of these cells in pulmonary diseases, including asthma. In addition, some NP were found to exert some pro-inflammatory activity in airway diseases in vivo (Larsen et al., Citation2010; Brandenberger et al., Citation2013). Here, among the tested NP, it was interesting to note that only ZnO and AgNP20 were found to activate these cells. Therefore, NP does not necessarily act similarly on eosinophils and, according to the present data, induction of apoptosis is a potential mechanism of toxicity of ZnO and AgNP20. The presence of large aggregates in AgNP20 is not without any precedent since, as previously documented for human neutrophils (Poirier et al. Citation2014), and as for these cells, we cannot rule out here the possibility that such aggregates can induce the observed effect in 3D10 cells. Interestingly, we recently documented that AgNP70 and AgNP20 alter differently the biology of human neutrophils (Poirier et al. Citation2016). The fact that AgNP20 were more potent than AgNP70 could be related to their ability to penetrate more easily inside cells. Therefore, it is tempting to speculate here that because AgNP20 possess more biological activity in 3D10 cells than AgNP70, this could also be linked to differences in cellular nanoparticle uptake. This remains to be determined.
Of note, in the present study, 3D10 cells were incubated with NP for only 24 h. This was not like several studies where cells or cell lines were incubated with NP for up to 72 h and even longer in some cases (Zhang et al. Citation2011; Al-Qubaisi et al. Citation2013; Chuang et al. Citation2013; Hou et al. Citation2013; Kimura et al. Citation2013; Tsai et al. Citation2013). Nevertheless, in experimental similar conditions to those used in the present study (100 μg/ml for 24 h), TiO2 NP were found to be anti-apoptotic in human neutrophils (Goncalves et al. Citation2010), whereas AgNP20 were rather pro-apoptotic (Poirier et al. Citation2014). Therefore, a given NP can act similarly on different cell types (e.g., AgNP20) or not (e.g., TiO2). Several pro-apoptotic NP, including those investigated here, are known to activate caspases in different cell types (Kang et al. Citation2009; Lanone & Boczkowski Citation2011; Guo et al. Citation2013).
In contrast, in the present study, the tested caspases were not, or only minimally, activated by ZnO and AgNP20. Despite this, lamin B1 was cleaved by both ZnO and AgNP20. These results are in agreement with our previous observation that the pro-apoptotic molecule VAA-I cleaved several cytoskeletal proteins, including lamin B1 in 3D10 cells (Lavastre et al. Citation2005). However, the ability of VAA-I to cleave lamin B1 was found to be a caspase-dependent process since pre-treatment of cells with the pan-caspase inhibitor z-VAD, completely reversed the degradation/cleavage of lamin B1 by VAA-I. Therefore, it is tempting to speculate that ZnO and AgNP20 induce lamin B1 degradation by proteases other than caspases. For example, calpains were recently shown to be involved in eosinophil apoptosis. In primary human eosinophils, lamin B degradation was found to be a caspase-3- and 6-dependent process (Ilmarinen-Salo et al. Citation2010). We did not investigate here the potential role of caspase-6 in ZnO- or AgNP20-induced lamin B1 degradation in leukemia eosinophils, since, unlike primary human eosinophils (Ilmarinen-Salo et al. Citation2010), there is no evidence yet that 3D10 cells express this caspase.
Interestingly, among the tested NP, ZnO and AgNP20 were not only the two NP found to induce apoptosis but also the only ones that increased cytokine production in 3D10 cells. However, it is difficult to make a link between the pro-apoptotic activity of ZnO and AgNP20 with their ability to increase cytokine production. For example, the fact that among the tested analytes, only TNFα is known to mediate apoptosis in a variety of cells. In addition, the TNFα production is increased only by ZnO and not by AgNP20 that are both pro-apoptotic in 3D10 cells. Also, it was previously demonstrated that addition of TNFα to IL-15 in human eosinophil cultures did not promote or accelerate their rate of apoptosis (Hoontrakoon et al. Citation2002) and, moreover, TNFα was found to be anti-apoptotic by itself in these cells (Tsukahara et al. Citation1999). Although ZnO and AgNP20 increased the production of other analytes in the present study, these latter, including IL-8, MIP-1α, and MIP-1β are known to be potent chemoattractants and not as pro-apoptotic agents. This concords with potential pro-inflammatory activity of ZnO and/or AgNP20. In this sense, ZnO NP was recently found to increase the production of IL-8 in human bronchial epithelial cells (Wu et al. Citation2010). Interestingly, ZnO NP are known to provoke metal fume fever (Kuschner et al. Citation1995), where an increased number of certain analytes, especially TNFα, IL-6, and IL-8, are observed in bronchoalveolar fluids (Kuschner et al. Citation1997). The current results are also in agreement with this observation since ZnO was found to increase the production of both TNFα and IL-8 in human 3D10 cells, but not of IL-6.
The pro-inflammatory activity of nanosilver NP is less documented than ZnO NP and the results are not clear. In a subacute murine inhalation model, nanosilver was found to induce minimal lung toxicity or inflammation (Stebounova et al. Citation2011). However, the average nano-silver NP size used in this study was ≈10 nm rather than 20 nm. In another study, nanosilver particles less than 100 nm and microsilver particles (250 μm) were implanted into the back muscles of rats and inflammation response levels measured based on patho- and morphological changes (Chen et al. Citation2007). After 90 and 180 d, local inflammation reaction induced by the implanted nanosilver particles was more serious than the microsilver particles. More recently, a higher propensity for citrate 20 nm NP to generate acute neutrophilic inflammation in the lung and to produce some cytokines was reported (Wang et al. Citation2013). In particular, the production of LIX and MCP-1 was increased in response to citrate 20 nm NP. Unfortunately, LIX, a chemokine usually associated with cell migration and activation of neutrophils, was not an analyte among the ones tested in the present study. Also, although we observed an increase production of MCP-1 in response to ZnO, we did not observe this for AgNP20. However, as measured by ELISA, it was clear that human eosinophils could be directly activated by both NP to produce large amounts of IL-8, a potent pro-inflammatory molecule know to attract leukocytes, especially neutrophils (Kobayashi, Citation2008).
Conclusions
The results of this study indicate that NP do not activate similarly human eosinophils, since some (ZnO and AgNP20) can induce apoptosis and cytokine production while others (TiO2, CeO2, and AgNP70) do not have such effects. Moreover, NP belonging to a common group, namely metallic oxides such as TiO2, CeO2, and ZnO also impact differently on eosinophil cell biology. This is also true for a given NP with a different starting diameter; AgNP20 induce apoptosis and cytokine production, but not AgNP70. The fact that some NP can directly activate eosinophils to release chemokines that possess the capacity to attract leukocytes, including eosinophils themselves, support a role of these cells in cytokine networking leading to allergic airway inflammation.
Funding information
This study was partially supported by grants from Natural Sciences and Engineering Research Council of Canada (NSERC) and the Institut de Recherche Robert-Sauvé en Santé et en Sécurité du Travail (IRSST).
Disclosure statement
The authors declare no conflicts of interest. The authors alone are responsible for the content of this manuscript.
References
- Abrikossova N, Skoglund C, Ahren M, Bengtsson T, Uvdal K. 2012. Effects of gadolinium oxide nanoparticles on the oxidative burst from human neutrophil granulocytes. Nanotechnology. 23:275101.
- Al-Qubaisi MS, Rasedee A, Flaifel MH, Ahmad SH, Hussein-Al-Ali S, Hussein MZ, Eid EE, Zainal Z, Saeed M, Ilowefah M, et al. 2013. Cytotoxicity of nickel zinc ferrite nanoparticles on cancer cells of epithelial origin. Int J Nanomed. 8:2497–2408.
- Babin K, Antoine F, Goncalves DM, Girard D. 2013. TiO2, CeO2, and ZnO nanoparticles and modulation of the degranulation process in human neutrophils. Toxicol Lett. 221:57–63.
- Baumann MA, Paul CC. 1998. The AML14 and AML14.3D10 cell lines: A long-overdue model for the study of eosinophils and more. Stem Cells. 16:16–24.
- Brandenberger C, Rowley NL, Jackson-Humbles DN, Zhang Q, Bramble LA, Lewandowski RP, Wagner JG, Chen W, Kaplan BL, Kaminski NE, et al. 2013. Engineered silica nanoparticles act as adjuvants to enhance allergic airway disease in mice. Part Fibre Toxicol. 10:26.
- Chen D, Xi T, Bai J. 2007. Biological effects induced by nanosilver particles: In vivo study. Biomed Mater. 2:S126–S128.
- Chen EY, Garnica M, Wang YC, Chen CS, Chin WC. 2011. Mucin secretion induced by titanium dioxide nanoparticles. PLoS One. 6:e16198.
- Chen HW, Su SF, Chien CT, Lin WH, Yu SL, Chou CC, Chen JJ, Yang PC. 2006. Titanium dioxide nanoparticles induce emphysema-like lung injury in mice. FASEB J. 20:2393–2395.
- Cho WS, Duffin R, Poland CA, Howie SE, MacNee W, Bradley M, Megson IL, Donaldson K. 2010. Metal oxide nanoparticles induce unique inflammatory footprints in the lung: Important implications for nanoparticle testing. Environ Health Perspect. 118:1699–1606.
- Chuang SM, Lee YH, Liang RY, Roam GD, Zeng ZM, Tu HF, Wang SK, Chueh PJ. 2013. Extensive evaluations of the cytotoxic effects of gold nanoparticles. Biochim Biophys Acta. 1830:4960–4973.
- Goncalves DM, Chiasson S, Girard D. 2010. Activation of human neutrophils by titanium dioxide (TiO2) nanoparticles. Toxicol In Vitro. 24:1002–1008.
- Goncalves DM, Girard D. 2013. Evidence that polyhydroxylated C60 fullerenes (fullerenols) amplify the effect of lipopolysaccharides to induce rapid leukocyte infiltration in vivo. Chem Res Toxicol. 26:1884–1892.
- Goncalves DM, Girard D. 2014. Zinc oxide nanoparticles delay human neutrophil apoptosis by a de novo protein synthesis-dependent and reactive oxygen species-independent mechanism. Toxicol In Vitro. 28:926–931.
- Guo D, Bi H, Wu Q, Wang D, Cui Y. 2013. Zinc oxide nanoparticles induce rat retinal ganglion cell damage through bcl-2, caspase-9 and caspase-12 pathways. J Nanosci Nanotechnol. 13:3769–3777.
- Hoontrakoon R, Chu HW, Gardai SJ, Wenzel SE, McDonald P, Fadok VA, Henson PM, Bratton DL. 2002. Interleukin-15 inhibits spontaneous apoptosis in human eosinophils via autocrine production of granulocyte macrophage-colony stimulating factor and NF-κB activation. Am J Respir Cell Mol Biol. 26:404–412.
- Hou Y, Lai M, Chen X, Li J, Hu Y, Luo Z, Ding X, Cai K. 2013. Effects of mesoporous SiO, FeO, and TiO nanoparticles on the biological functions of endothelial cells in vitro. J Biomed Mater Res A. 102:1726–1736.
- Ilmarinen-Salo P, Moilanen E, Kankaanranta H. 2010. Nitric oxide induces apoptosis in GM-CSF-treated eosinophils via caspase-6-dependent lamin and DNA fragmentation. Pulm Pharmacol Ther. 23:365–371.
- Kang SJ, Kim BM, Lee YJ, Hong SH, Chung HW. 2009. Titanium dioxide nanoparticles induce apoptosis through the JNK/p38-caspase-8-Bid pathway in phytohemagglutinin-stimulated human lymphocytes. Biochem Biophys Res Commun. 386:682–687.
- Kimura R, Rokkaku T, Takeda S, Senba M, Mori N. 2013. Cytotoxic effects of fucoidan nanoparticles against osteosarcoma. Mar Drugs. 11:4267–4278.
- Kobayashi Y. 2008. The role of chemokines in neutrophil biology. Front Biosci. 13:2400–2407.
- Kuschner WG, D'Alessandro A, Wintermeyer SF, Wong H, Boushey HA, Blanc PD. 1995. Pulmonary responses to purified zinc oxide fume. J Invest Med. 43:371–378.
- Kuschner WG, D'Alessandro A, Wong H, Blanc PD. 1997. Early pulmonary cytokine responses to zinc oxide fume inhalation. Environ Res. 75:7–11.
- Lanone S, Boczkowski J. 2011. Titanium and gold nanoparticles in asthma: The bad and the ugly. Eur Respir J. 37:225–227.
- Larsen ST, Roursgaard M, Jensen KA, Nielsen GD. 2010. Nano-titanium dioxide particles promote allergic sensitization and lung inflammation in mice. Basic Clin Pharmacol Toxicol. 106:114–117.
- Lavastre V, Chiasson S, Cavalli H, Girard D. 2005. Viscum album agglutinin-I induces apoptosis and degradation of cytoskeletal proteins via caspases in human leukaemia eosinophil AML14.3D10 cells: Differences with purified human eosinophils. Br J Hematol. 130:527–535.
- Lavastre V, Pelletier M, Saller R, Hostanska K, Girard D. 2002. Mechanisms involved in spontaneous and Viscum album agglutinin-I-induced human neutrophil apoptosis: Viscum album agglutinin-I accelerates the loss of antiapoptotic Mcl-1 expression and the degradation of cytoskeletal paxillin and vimentin proteins via caspases. J Immunol. 168:1419–1427.
- Neun BW, Dobrovolskaia MA. 2011. Detection and quantitative evaluation of endotoxin contamination in nanoparticle formulations by LAL-based assays. Meth Mol Biol. 697:121–130.
- Oberdorster G, Oberdorster E, Oberdorster J. 2005. Nanotoxicology: An emerging discipline evolving from studies of ultrafine particles. Environ Health Perspect. 113:823–839.
- Poirier M, Simard JC, Antoine F, Girard D. 2014. Interaction between silver nanoparticles of 20 nm (AgNP20) and human neutrophils: Induction of apoptosis and inhibition of de novo protein synthesis by AgNP aggregates. J Appl Toxicol. 34:404–412.
- Poirier M, Simard JC, Girard D. 2016. Silver nanoparticles of 70 nm and 20 nm affect differently the biology of human neutrophils. J. Immunotoxicol. 13:375–385.
- Rossi EM, Pylkkanen L, Koivisto AJ, Vippola M, Jensen KA, Miettinen M, Sirola K, Nykasenoja H, Karisola P, Stjernvall T, et al. 2010. Airway exposure to silica-coated TiO2 nanoparticles induces pulmonary neutrophilia in mice. Toxicol Sci. 113:422–433.
- Roy R, Kumar S, Verma AK, Sharma A, Chaudhari BP, Tripathi A, Das M, Dwivedi PD. 2014. Zinc oxide nanoparticles provide an adjuvant effect to ovalbumin via a TH2 response in Balb/c mice. Int Immunol. 26:159–172.
- Sahu D, Kannan GM, Vijayaraghavan R, Anand T, Khanum F. 2013. Nanosized zinc oxide induces toxicity in human lung cells. ISRN Toxicol. 2013:316075.
- Srinivas A, Rao PJ, Selvam G, Murthy PB, Reddy PN. 2011. Acute inhalation toxicity of cerium oxide nanoparticles in rats. Toxicol Lett. 205:105–115.
- Stebounova LV, Adamcakova-Dodd A, Kim JS, Park H, O'Shaughnessy PT, Grassian VH, Thorne PS. 2011. Nanosilver induces minimal lung toxicity or inflammation in a subacute murine inhalation model. Part Fibre Toxicol. 8:5.
- Tsai SW, Liaw JW, Kao YC, Huang MY, Lee CY, Rau LR, Huang CY, Wei KC, Ye TC. 2013. Internalized gold nanoparticles do not affect the osteogenesis and apoptosis of MG63 osteo-blast-like cells: A quantitative in vitro study. PLoS One. 8:e76545.
- Tsukahara K, Nakao A, Hiraguri M, Miike S, Mamura M, Saito Y, Iwamoto I. 1999. Tumor necrosis factor-α mediates anti-apoptotic signals partially via p38 MAP kinase activation in human eosinophils. Int Arch Allergy Immunol. 1:54–59.
- Wang X, Ji Z, Chang CH, Zhang H, Wang M, Liao YP, Lin S, Meng H, Li R, Sun B, et al. 2013. Use of coated silver nanoparticles to understand the relationship of particle dissolution and bioavailability to cell and lung toxicological potential. Small. 10:385–398.
- Wu W, Samet JM, Peden DB, Bromberg PA. 2010. Phosphorylation of p65 is required for zinc oxide nanoparticle-induced IL-8 expression in human bronchial epithelial cells. Environ Health Perspect. 118:982–987.
- Zhang XQ, Yin LH, Tang M, Pu YP. 2011. ZnO, TiO2, SiO2, and Al2O3 nanoparticles-induced toxic effects on human fetal lung fibroblasts. Biomed Environ Sci. 24:661–669.