Abstract
Immune-mediated drug hypersensitivity reactions (IDHR) may result from immuno-sensitization to a drug-induced neo-antigen. They rarely occur in patients and are usually not predicted preclinically using standard toxicity studies. To assess the potential of a drug to induce T-cell sensitization, trinitrophenyl (TNP)-Ficoll was used here as a bystander antigen in animal experiments. TNP-Ficoll will only elicit TNP-specific IgG antibodies in the presence of non-cognate T-cell help. Therefore, the presence of TNP-specific IgG antibodies after co-injection of drug and TNP-Ficoll was indicative of T-cell sensitization potential. This TNP-Ficoll-approach was used here to characterize T-cell help induced by oral exposure to diclofenac (DF) or carbamazepine (CMZ). DF or CMZ was administered orally to BALB/c mice and after 3 w, the mice were challenged in a hind paw with TNP-Ficoll and a dose of the drug that by itself does only elicit a sub-optimal popliteal lymph node assay (PLNA) response. T-cell-dependent responses were then evaluated in paw-draining popliteal lymph nodes (PLN). Also, shortly after oral exposure, mesenteric lymph nodes (MLN) were excised for evaluation of local responses. Both drugs were able to increase PLN cellularity and TNP-specific IgG1 production after challenge. Both DF and CMZ stimulated CD4+ and CD8+ T-cells and caused shifts of the subsets toward an effector phenotype. DF, but not CMZ, appeared to stimulate interferon (IFN)-γ production. Remarkably, depletion of CD8+, but not CD4+, T-cells reduced TNP-specific IgG1 production, and was more pronounced in CMZ- than in DF-exposed animals. Local responses in the MLN caused by DF or CMZ also showed shifts of CD4+ and CD8+-cells toward a memory phenotype. Together, the data indicate that oral exposure to CMZ and DF differentially induced neo-antigen-specific T-cell reactions in the PLNA.
Introduction
Immune-mediated drug hypersensitivity reactions (IDHR) are very difficult to predict in pre-clinical standard toxicity studies. They are an important reason for black box warnings or withdrawal of drugs from the market. IDHR are generally idiosyncratic in nature as multiple environmental and inherent factors contribute to their complexity (Gomes and Demoly Citation2005; Uetrecht Citation2007; Ulrich Citation2007; Chalasani & Björnsson Citation2010; Pichler et al. Citation2011; Zhang et al. Citation2011). Due to this idiosyncrasy, IDHR are often not detected before market introduction and widespread human use of a drug. This complexity is further accentuated by the fact several hypotheses have been described for induction of IDHR (Lee Citation2003; Uetrecht Citation2007; Zhang et al. Citation2011).
It has become clear that immuno-sensitization to small molecule drugs mostly involves both neo-antigen formation (e.g. hapten–protein conjugation, cryptic epitopes, pharmacological interaction with, e.g. MHC–peptide complexes) and co-stimulatory signals that provide adjuvant activity for efficient T-cell sensitization. The adjuvant activity may for instance result from induction of an inflammatory reaction and may also be sufficient to induce sensitization of auto-reactive T-cells. Related to these important key mechanisms, a smart panel of in vitro and in vivo methods can possibly help to identify the potential of a drug to induce IDHR. Among these models are local lymph node assays, such as the popliteal lymph node assay (PLNA).
Previously, we have shown the mouse PLNA combined with specific reporter antigens (RA) allows for discrimination between compounds that have co-stimulatory adjuvant activity, and compounds that produce neo-antigens including hapten–protein conjugates (Kammüller et al. Citation1989; Pieters et al. Citation2002. In this RA–PLNA-setup, a sub-sensitizing dose of the T-cell-dependent antigen trinitrophenyl-ovalbumin (TNP-OVA) or the T-cell-independent antigen TNP-Ficoll is subcutaneously co-injected into the hind footpad with the small molecule drug of interest (Albers et al. Citation1997; Gutting et al. Citation1999; Pieters & Albers Citation1999a,Citationb; Pieters Citation2001). TNP-specific IgG responses to TNP-OVA are indicative of cognate T-cell help, and because a sub-sensitizing dose of TNP-OVA is used, this response depends on sufficient co-stimulatory help induced by the tested drug. The polysaccharide molecule TNP-Ficoll; however, cannot be presented to T-cells, but by itself can activate B-cells to produce specific IgM antibodies and importantly the B-cell response to TNP-Ficoll is susceptible to non-cognate T-cell help. This means that TNP-specific IgG responses to TNP-Ficoll depend on additional T-cells that do not recognize TNP-Ficoll as antigen. Hence, IgG responses to TNP-Ficoll are indicative of non-cognate T-cell help, and of the presence of drug-induced neo-antigens.
Although the RA–PLNA can be used as an easy and fast first-screening method for new compounds, it uses subcutaneous footpad injection, reflecting a local response to subcutaneous injection but excluding presumably relevant gastrointestinal or hepatic metabolism and other processes, such as local immunoregulatory mechanisms. A previously described set-up using oral drug administration combined with TNP-OVA or TNP-Ficoll injection has shown that reporter antigens can indeed also be used combined with oral exposures. Furthermore, drugs that do not induce any IDHR did not induce immune responses in this model. Thus, showing the ability of this set-up to discriminate between immune sensitizing and non-sensitizing drugs (Gutting et al. Citation2002a; Nierkens et al. Citation2005; Kwast et al. Citation2011). The current study set out to further evaluate how two IDHR-inducing drugs, diclofenac (DF) and carbamazepine (CMZ), modulate IgG responses to TNP-Ficoll. Further, this study investigated the activation status of CD4+ and CD8+ T-cell responses following challenge with DF or CMZ.
The non-steroidal-anti-inflammatory drug (NSAID) DF is a widely used non-selective cyclo-oxygenase (COX) inhibitor that can occasionally induce severe hepatotoxicity, ulceration and gastrointestinal irritation. Metabolite formation has been described for this drug, resulting in protein-adduct formation (Wade et al. Citation1997; Boelsterli Citation2003; Aithal et al. Citation2004; Naisbitt et al. Citation2007). Conceivably, local irritation caused by DF can induce an intestinal and possibly systemic inflammatory environment, providing co-stimulatory signals that together with MHC-presentation of protein-adducts may trigger specific T-cell sensitization (Reuter et al. Citation1997; Gutting et al. Citation2002b; Bol-Schoenmakers et al. Citation2011). CMZ is an anti-epileptic drug that has been shown to cause liver toxicity, and also skin-related problems. Furthermore, the involvement of specific T-cells has been described (Naisbitt et al. Citation2003; Roychowdhury & Svensson Citation2005). Like DF, CMZ also has been shown to form reactive metabolites and protein-adducts (Lertratanangkoon and Horning Citation1982; Lillibridge et al. Citation1996; Ju & Uetrecht Citation1999; Kang et al. Citation2008; Njoku Citation2010). Further, in CMZ-sensitized patients, T-cells have been identified that were able to react to both the culprit drug as well as its reactive metabolites (Mauri-Hellweg et al. Citation1995).
The results of the study showed that oral exposure to DF or CMZ stimulated IgG1 responses to TNP-Ficoll. Furthermore, these CD4+ and CD8+ T-cell reactions included shifts in differentiation status in the popliteal lymph nodes (PLN) and in the mesenteric lymph nodes (MLN) shortly after oral exposure.
Materials and methods
Mice
Balb/c mice (female, 4–6-wk-old) were purchased from Charles River (Lyon, France). Mice were specific pathogen-free and upon arrival maintained under barrier conditions in filter-topped macrolon cages with woodchip bedding held in a facility maintained at 23 ± 2 °C, with a 50–55% relative humidity and a 12 h light/dark cycle. Drinking water and standard laboratory food pellets were provided ad libitum. All experiments were conducted according to the guidelines of, and with permission from, the animal experiments committee (IACUC) of Utrecht University.
Chemicals
Chemicals used for oral administration or footpad challenge were obtained from Sigma-Aldrich (Zwijndrecht, the Netherlands). TNP-Ficoll was obtained from Biosearch Technologies (Novarto, CA). DF for oral administration was dissolved in distilled water (Aqua B. Braun, Melsungen, Germany) and for footpad challenge in phosphate-buffered saline (PBS, pH 7.4). Carbamazepine for oral administration was dissolved in a 1% carboxymethylcellulose (CMC) solution in distilled water (Aqua B. Braun). Carbamazepine for footpad challenge was dissolved in a 25% PEG400 solution in distilled water. Tissue culture reagents were purchased from Invitrogen Life Technologies (Paisley, Scotland) or Greiner Bio-one (Frickenhausen, Germany).
Dose CMZ combined with TNP-Ficoll as reporter antigen
Mice received a subcutaneous injection into the hind footpad of 0.25, 0.5 or 1 mg CMZ combined with 10 μg TNP-Ficoll in a total volume of 50 μl. After 7 d, the mice were euthanized by cervical dislocation and PLN were isolated for analysis ().
Figure 1. Experimental set-up. (A) BALB/c mice (n = 6/group) were injected in the hind footpad with 0.25, 0.5 or 1 mg CMZ combined with 10 μg TNP-Ficoll. After 7 days, the mice were euthanized and their PLN isolated for further analysis. (B) Mice (n = 6–8 group) were orally exposed to 1 (75 mg/kg DF) or 7 consecutive (100 mg/kg CMZ) doses. On Day 21, the mice were challenged in the hind footpad with 0.5 mg/animal of DF/CMZ together with 10 μg of TNP-Ficoll. One week following this challenge, all mice were euthanized and their PLN isolated for analysis. For experiments including T-cell depletion, mice received IP injections with 300 μg/200 μl saline of CD4 + T-cell depleting antibody on Days −2 and 0 for DF-exposed animals and on Days −2, 0 and 3 for CMZ-exposed animals. CD8+ T-cell depleting antibody was injected on Days −2 and 0 in both experiments. (C) Mice (n = 6/group) were orally exposed to a single dose of DF (75 mg/kg) or seven consecutive doses of CMZ (100 mg/kg). Two, five and eight days following the (first) oral dose, mice were euthanized and their MLN isolated for further analysis.
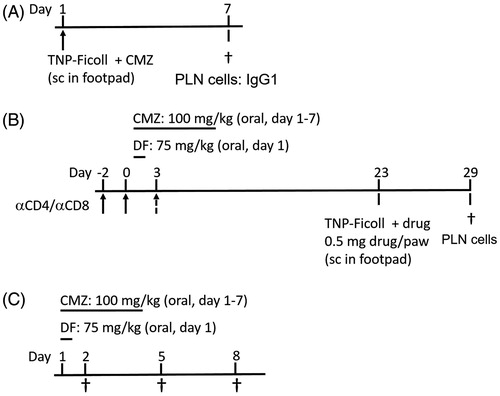
DF and CMZ with TNP-Ficoll Oral exposure protocol using TNP-Ficoll as reporter antigen
Mice were exposed according to the protocol noted in . In brief, mice received oral doses of vehicle, a single dose of DF (75 mg/kg), or seven consecutive daily doses of CMZ (100 mg/kg). The different oral regimens were chosen using the maximum tolerable dose as described in the datasheet of the compounds provided by the manufacturer (Sigma-Aldrich, Zwijndrecht, the Netherlands) and as used in previous experiments (Nierkens et al. Citation2005; Kwast et al. Citation2011). On Day 21, all mice were challenged subcutaneously in the hind footpad with 0.5 mg/animal of DF or CMZ together with 10 μg TNP-Ficoll [in 50 μl total volume]. One week following this challenge, the mice were euthanized and their PLN removed for further analysis.
CD4+ and CD8+ T-cell depletion
Mice were treated according to the oral protocol as described previously (). In addition, they each received intraperitoneal injections with 300 μg (in 200 μl saline) of αCD4+ T-cell depleting antibody (clone GK1.5) on Days −2, 0 and 3, or αCD8+ T-cell depleting antibody (clone YTS169) on Days −2 and 0. Each antibody had been purified from culture supernatants using ammonium sulfate gradients on thiophilic agarose resins. The concentrations used were determined previously to be capable of depleting CD4+ or CD8+ T-cells in Balb/c mice to at least 5% of normal levels (data not shown; Gocinski & Tigelaar Citation1990).
DF and CMZ in short exposure protocol
Mice were exposed to the protocol described in . In brief, mice received oral doses of vehicle, a single dose of DF (75 mg/kg), or seven consecutive daily doses of CMZ (100 mg/kg). The different oral regimens were chosen using the maximum tolerable dose as described in the datasheet of the compounds provided by the manufacturer (Sigma) and as used in previous experiments (Nierkens et al. Citation2005; Kwast et al. Citation2011). On Days 2, 5 and 8 following the first exposure, the mice were euthanized and their MLN removed for analysis.
Cell isolation PLN
After isolation, PLN were separated from adherent fatty tissue and placed in ice-cold complete RPMI 1640 medium (Invitrogen) supplemented with 2.5% fetal calf serum (FCS; Greiner Bio-one) and 1% penicillin/streptomycin (Invitrogen) (RPMI/2.5%). Single cell suspensions were prepared and cells were washed and re-suspended in 0.5 ml RPMI/2.5%. Cell numbers were determined using a Coulter Counter (Beckman Coulter, Woerden, the Netherlands) and adjusted to 106 live cells/ml.
Single cell preparation MLN
MLN were made into single cell suspensions by first placing them into ice-cold complete RPMI/2.5%. The tissues were mashed trough a 70-μm cell strainer and washed once with RPMI/2.5%. Red blood cells were then removed by incubating the cells in lysis buffer (0.16 M NH4Cl, 10 mM KHCO3, 0.1 mM Na2EDTA [pH 7.4]) on ice for 1 min followed by a single wash with PBS. Cells were then washed several times with RPMI/2.5% and re-suspended in RPMI/10% (RPMI 1640 supplemented with 10% FCS and 1% penicillin/streptomycin). Cell numbers were determined and adjusted to 106 cells/ml.
PLN cell re-stimulation and cytokine ELISA
PLN cell suspensions (in triplicate) at 1.5 × 105 cells in RPMI 1640/10% were seeded into 96-well culture plate and then incubated with 1 μg αCD3/ml and 1 μg αCD28/ml at 37 °C for 48 h. Acellular supernatant from each well was then collected and stored at −20 °C until analysis. Levels of interferon (IFN)-γ or interleukin (IL)-4 in samples were then estimated using commercially available ELISA kits (eBioscience, San Diego, CA), according to the manufacturer instructions. The level of sensitivity of the kits was 15 pg IFNγ/ml and 4 pg IL-4/ml.
Flow cytometry
For flow cytometric analysis, 2–5 × 105 cells were stained in FACS buffer (PBS containing 0.25% bovine serum albumin (BSA), 0.5 mM EDTA and 0.05% NaN3) containing fluorescein isothiocyanate (FITC)-, R-phycoerythrin (RPE)-, perinidin chlorophyll protein (PerCP)-, or allophycocyanin (APC)-labeled antibodies (purchased from eBioscience or BD-Pharmingen, Eerenbodegem, Belgium). In brief, cells were first pre-incubated for 20 min at 4 °C in FACS buffer containing αCD16/CD32 (2.4G2) to block Fc receptor binding. T-cell subsets were then characterized using anti-CD4-PerCP (clone RM4–5), -CD62L-APC (clone MEL-14), -CD8α-FITC (clone 53–6.7) and -CD44-PE (clone IM7) antibodies at manufacturer-recommended levels/2–5 × 105 cells and incubation on ice in the dark for 30 min. The cells were then fixed and stored in 0.4% formaldehyde until acquisition. Data were acquired on a FACS Calibur (Becton Dickinson, Breda, the Netherlands) and analysis was performed using Weasel (Walter and Eliza Hall Institute of Medical Research, Melbourne Australia). A minimum of 50 000 events/sample was acquired.
Statistical analysis
Multiple comparisons of group means were analyzed using a one-way analysis of variance (ANOVA) with a Bonferroni post-hoc test. A p value <0.05 was considered significantly different compared with controls. All analyses were performed using Prism 5 for Windows software (GraphPad, San Diego, CA)
Results
CMZ dose for footpad injection
The study here first determined the suboptimal dose to be used for CMZ footpad injection in combination with TNP-Ficoll. To that end, three doses of CMZ were tested in combination with TNP-Ficoll in naïve mice. A dose of 1 mg CMZ significantly enhanced PLN cellularity and the amount of IgG1 antibody secreting cells (ASC) (), but a dose of 0.5 mg appeared suitable as suboptimal challenge dose in future experiments. For DF, a suboptimal dose of 0.5 mg was used as determined previously (Gutting et al. Citation2002a; Nierkens et al. Citation2005). In the present work by the Nierkens group (performed in our laboratory), DF caused a nearly five-fold increase in IgG ASC after pre-exposure to DF when compared with after pre-exposure to saline. This was comparable to the increase induced by 0.5 mg CMZ.
Figure 2. Determination of suboptimal dose of CMZ. Mice (n = 6/group) were injected in hind footpad with 0.25, 0.5 or 1 mg CMZ together with 10 μg TNP-Ficoll. After 7 days, mice were euthanized, PLN isolated and (A) PLN cellularity and (B) TNP-Ficoll-specific IgG1 ASC were determined. Values shown are means ± SEM of vehicle- or drug-exposed group. **p < 0.01, ***p < 0.001; value significantly different vs. respective vehicle-exposed group.
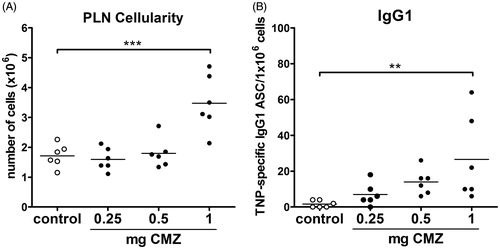
PLN cellularity and TNP-specific antibody production
To examine the ability of DF or CMZ to induce neo-antigen specific T-cell activation, mice were treated [according to the protocol described in ] with a combined footpad challenge of the low-dose of the drugs together with TNP-Ficoll. Challenges with DF or CMZ were seen to significantly increase PLN cellularity in mice orally pre-exposed to the respective drug (). Importantly, challenges with DF or with CMZ also significantly increased the amount of TNP-specific IgG1 ASC, which is, considering the use of TNP-Ficoll, indicative of a presence of non-cognate T-cell help ().
Figure 3. Cellularity, TNP specific IgG1, cytokine production, and amount of T-cells. Mice (n = 6–8/group) were pre-exposed orally to a single dose of 75 mg/kg DF or seven consecutive doses of 100 mg/kg CMZ. After 21 days, mice were challenged in hind footpad with the specific drug in combination with TNP-Ficoll. Seven days following this challenge, mice were euthanized, and PLN isolated. (A) PLN cellularity and (B) TNP-Ficoll-specific IgG1 cells were measured. (C) IL-4 and (D) IFNγ levels were determined in isolated PLN cells following 48 h stimulation with αCD3/αCD28. Amounts of (E) CD4+ and (F) CD8+ T-cells. Values shown are means ± SEM of the vehicle- or drug pre-exposed group. *p < 0.05, **p < 0.01 and ***p < 0.001; values significantly different vs. respective vehicle pre-exposed group.
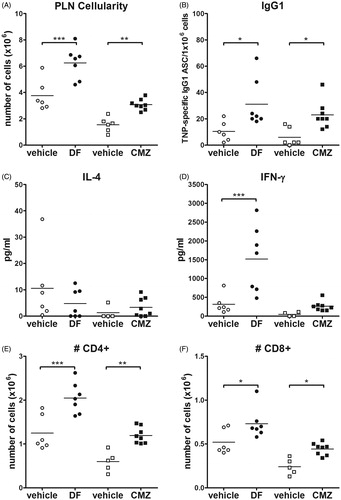
Cytokine production by re-stimulated PLN cells
PLN cells isolated after host challenge with the drugs and TNP-Ficoll were re-stimulated for 72 h with αCD3/αCD28 to investigate T-cell-specific cytokine production. The results indicate that PLN cells from DF-exposed, but not CMZ-exposed, mice showed significantly increased IFNγ production (). IL-4 production did not differ from control with cells obtained from any of the treatment groups ().
Changes in differentiation status of PLN T-cells
Both DF and CMZ challenge resulted in significant increases in CD4+ and CD8+ T-cell numbers in the PLN (). DF and CMZ did not change CD4+ T-cell levels as a percentage of total cells. However, DF challenge significantly reduced the percentage of CD8+ T-cells compared with controls, an outcome that was not observed in CMZ-challenged animals (). Differentiation status within both these T-cell populations was analyzed by discriminating between naïve (CD62L+ CD44−), effector (CD62L+ CD44+) and memory (CD62L−CD44+) T-cells. The data revealed that DF exposure significantly increased percentages of effector CD4+ T-cells, and decreased those of memory CD4+ T-cells. The differentiation status of CD8+ T-cells was unaltered by DF. CMZ, on the contrary, significantly increased the percentages of effector CD8+ T-cells and decreased those of memory CD8+ T-cells. The differentiation of CD4+ T-cells remained unaltered in CMZ-treated mice (). Naïve CD4+ T-cells were neither changed in DF- nor in CMZ-treated mice.
Figure 4. Differentiation status of CD4+ and CD8+ T-cells. Mice (n = 6–8/group) were orally exposed to a single (75 mg/kg DF) or seven consecutive (100 mg/kg CMZ) doses. On Day 21, mice were challenged in hind footpad with 0.5 mg/animal of DF/CMZ together with 10 μg TNP-Ficoll. Seven days after the challenge, mice were euthanized and their PLN isolated for analysis. The percentages of CD4+ and CD8+ T-cells and their subsequent differentiation status were determined. Naive (CD62L+ CD44−), effector (CD62L+ CD44+) and memory (CD62L−CD44+) subsets within all PLN CD4+ and CD8+ T-cell populations were determined. Values represent means ± SEM of the vehicle- or drug-exposed groups. *p < 0.05, **p < 0.01, ***p < 0.001; value significantly different vs. vehicle controls.
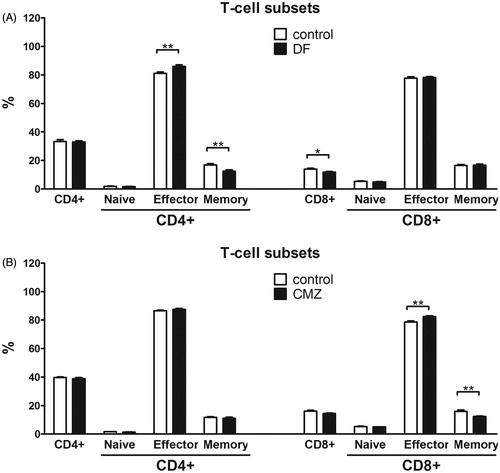
Changes in PLN cellularity and TNP-specific antibody production after T-cell depletion
Although DF and CMZ both activated CD4+ and CD8+ T-cells, each drug appeared to influence these cells differently. Therefore, the study set out to investigate the import of CD4+ and CD8+ T-cells on the immune response induced by these drugs by specifically depleting these cell types during the oral exposure phase. Again, the amount of TNP-specific IgG1 ASC was found to be significantly increased following DF or CMZ challenge. Only depletion of CD8+ T-cells appeared to prevent the induction of TNP-specific IgG1; depletion of CD4+ T-cells did not affect this challenge response (). In the case of CMZ, depletion of CD8+ T-cells appeared more effective in preventing induction of TNP-specific IgG1. In all, the recall reaction to both drugs seemed to be predominated by CD8+ T-cells.
Figure 5. T-cell depletion. Mice (n = 6–8/group) were exposed orally to a single dose of 75 mg/kg DF or seven consecutive daily doses of 100 mg/kg CMZ. During oral administration, CD4+ and CD8+ T-cells were depleted by IP injection of αCD4 or αCD8 depleting antibodies. On Day 21, mice were challenged in hind footpad with 0.5 mg/animal of DF/CMZ together with 10 μg TNP-Ficoll. Seven days after the challenge, mice were euthanized and their PLN isolated for analysis. Percentages of naïve (CD62L+ CD44−), effector (CD62L+ CD44+) and memory (CD62L−CD44+) subsets within all PLN CD4+ and CD8+ T-cells were determined. Values shown are means ± SEM of vehicle- or drug-exposed groups. *p < 0.05, **p < 0.01, ***p < 0.001; value significantly different vs. vehicle controls.
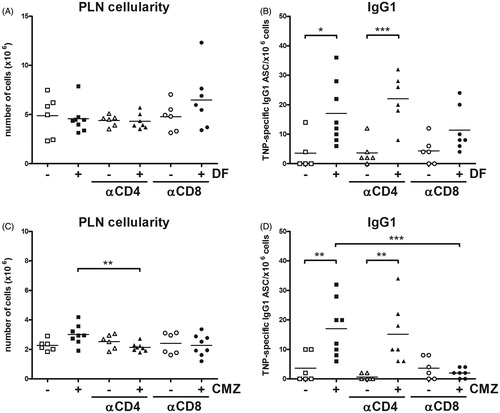
Changes in MLN T-cells
Since we were curious to know whether DF or CMZ also induced early local effects upon oral exposure, we also investigated the differentiation status of CD4+ and CD8+ T-cells in the MLN on Days 2, 5 and 8 following this oral administration. For this, naïve (CD62L+ CD44−), effector (CD62L+ CD44+) and memory (CD62L−CD44+) T-cell subsets were again discriminated. A single dose of DF significantly decreased the percentages of both CD4+ and CD8+ T-cells in the MLN on Day 8 following oral exposure ( and Supplementary Figure 1(A)). On Days 5 and 8, both CD4+ and CD8+ subsets displayed decreased effector and increased memory phenotypes. Naïve CD4+ and CD8+ cells remained unaltered after DF administration ( and Supplementary Figure 1(B)). Oral administration of CMZ did not alter CD4+ or CD8+ T-cell percentages in the MLN, but it significantly increased naïve, and decreased effector, CD8+ T-cells in the MLN after 8 days ( and Supplementary Figure 1C).
Figure 6. T-cells and activation status in MLN. Mice (n = 6/group) were exposed orally to a single dose of 75 mg/kg DF or seven consecutive doses of CMZ. Subsets of mice in each group were euthanized on Days 2, 5 and 8 following the first dose and subsequently MLN were isolated. The percentages of CD4+ and CD8+ T-cells and their activation status were determined in (A) DF- or (B) CMZ-exposed hosts. Values shown are the means ± SEM of vehicle- or drug-exposed groups. **p < 0.01, ***p < 0.001; value significantly different vs. respective vehicle-exposed group.
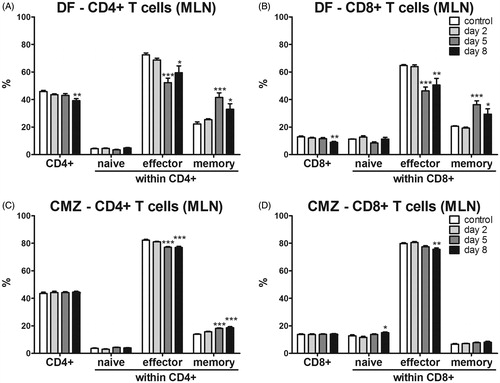
Discussion
The current study aimed to identify whether and what type of T-cells were induced by DF and CMZ upon oral exposure. For this, TNP-Ficoll was applied as a reporter antigen to be used to identify specific T-cells without the need to know their exact specificity. The results showed that challenge with DF or CMZ resulted in increased PLN cellularity and TNP-specific IgG1 ASC in drug pre-exposed animals. Also, the amounts of CD4+ and CD8+ T-cells were increased in drug pre-exposed groups. Further, where DF administration resulted in reduced CD8+ T-cell percentages and in shifts in differentiation status (memory–effector ratio) of CD4+ T-cells, CMZ pre-exposure imparted similar effects on CD8+ T-cells. Remarkably, responses induced by drugs seemed to be dependent on CD8+ T-cells, as only depletion of these cells resulted in decreased IgG1 ASC. Notably, short-term local effects as observed in MLN caused by DF exposure showed a shift in differentiation of both CD4+ and CD8+ T-cell subsets towards a memory phenotype, whereas CMZ exposure resulted in a similar shift but mostly in CD4+ T-cells.
It was shown previously that DF was able to induce both CD4+ and CD8+ T-cells in the PLNA (Gutting et al. Citation2002b). However, when T-cell-deficient mice were used in the same set-up, a minor PLN response was still observed, indicating that also a T-cell-independent component may be involved in immune responses to DF. In the present experiments, both T-cell subsets were not depleted simultaneously, but T-cell-independent innate cells (such as NK cells) may indeed be involved as it was recently observed that there was an increase in NK cells in the spleen upon oral exposure to DF but not to CMZ (Kwast et al. Citation2016). Furthermore, a reduction in the percentages of CD8+ T-cells was observed here. This suggested that possibly another (innate) immune cell type was increased, although which one in particular is not yet clear. Of note, the PLN cellularity varies sometimes between and even within experiments. The reason for this remains unclear but it was, apart from the relative irrelevance of cell numbers as an immune parameter, an important reason to use other immunologically more relevant parameters (such as e.g. ASC detection).
Interestingly, upon footpad challenge we observed shifts in the differentiation status of CD4+ T-cells in the PLN of DF-exposed animals, and shifts in the differentiation status of CD8+ T-cells in the PLN of CMZ-exposed animals. Shortly after exposure, we observed similar shifts in both CD4+ and CD8+ T-cells in the MLN after either DF or CMZ exposure. Apparently, different drugs are capable of affecting similar T-cell subsets shortly after exposure, but in time one or the other subset may become dominant. Regardless of this, CD8+ T-cells seemed to more pivotal both in the case of DF and in that CMZ, although the effect of CD8+ T-cell depletion was more moderate for DF. In several previously described models for contact sensitivity or atopic contact dermatitis, it was also shown that CD8+ T-cells predominated in the response to small molecules (Mauri-Hellweg et al. Citation1995; Martin et al. Citation2000; Vocanson et al. Citation2006). Thus, though immune responses to contact sensitizers differ from oral immune responses, CD8+ T-cell responses to small molecules seem to be important for both exposure routes. It was therefore remarkable that IgG1 responses were observed here as in others studies (Gutting et al. Citation2002b) outcomes which – according to general immunologic concepts – depended on CD4+ TH2 cell responses. Interestingly, in the present experiments, re-stimulated PLN cells isolated from DF-challenged mice showed increased production of the TH1 cytokine IFNγ but not TH2 cytokine IL-4 (or IL-5; data not shown). At the moment, it remains unclear what is causing this apparent discrepancy, but somehow IgG1 responses seem to be dependent on CD8+ T-cells and IFNγ.
The observation here of early changes in T-cells in the MLN warrants further analyses of immune phenomena induced locally after oral exposure. Such has already been done so far DF in combination with sensitization to peanut proteins in a mouse model of food allergy; that study found indeed that DF caused early disrupting effects on epithelial cells and was able to stimulate sensitization to the peanut proteins (Bol-Schoenmakers et al. Citation2011). It may therefore be interesting to include gut-associated lymphoid tissue, including the MLN, as read-out parameters in future experiments.
Clearly, both DF and CMZ may act as adjuvants (Kwast et al. Citation2011). This adjuvant effect may result in an autoimmune response, an immune response towards a neo-antigen (e.g. a hapten–protein conjugate), or a combination of the two. The formation of drug-specific neo-antigens in the form of hapten–protein adducts following drug exposure is often suspected but cannot be easily detected with current methods (Griem et al. Citation1998; Switzar et al. Citation2013). For both DF and CMZ, formation of multiple reactive metabolites has been described in different human and animal model systems (Lertratanangkoon & Horning Citation1982; Lillibridge et al. Citation1996; Wade et al. Citation1997; Ware et al. Citation1998; Ju & Uetrecht Citation1999; Boelsterli Citation2003; Aithal et al. Citation2004; Naisbitt et al. Citation2007; Kang et al. Citation2008; Njoku Citation2010). For newly developed drugs, metabolite formation and subsequent drug-adduct identification in vivo (e.g. in toxicity studies) remains a challenge. Therefore, the use of TNP-Ficoll is an interesting and helpful first screening tool in drug research to investigate these issues. The present study showed that the experimental approach used, i.e. combining oral exposure to drugs and footpad challenge with drug plus TNP-Ficoll, may help to further characterize underlying mechanisms of T-cell-dependent responses upon oral exposure.
Supplemental Figure 1. Gating strategy and phenotypes of T-cells in MLN
Download JPEG Image (793 KB)Disclosure statement
The authors declare no conflicts of interest. The authors alone are responsible for the content of this manuscript.
Funding
This project was funded by Dutch Top Institute Pharma project ??Towards novel translational safety biomarkers for adverse drug toxicity?? [D3-201-1] and the MIP-DILI project, a European Community grant under the Innovative Medicines Initiative (IMI) Program [115336].
References
- Aithal G, Ramsay L, Daly A, Sonchit N, Leathart J, Alexander G, Kenna J, Caldwell J, Day C. 2004. Hepatic adducts, circulating antibodies, and cytokine polymorphisms in patients with diclofenac hepatotoxicity. Hepatology. 39:1430–1440.
- Albers R, Broeders A, van der Pijl A, Seinen W, Pieters R. 1997. The use of reporter antigens in the popliteal lymph node assay to assess immunomodulation by chemicals. Toxicol Appl Pharmacol. 143:102–109.
- Boelsterli U. 2003. Diclofenac-induced liver injury: A paradigm of idiosyncratic drug toxicity. Toxicol Appl Pharmacol. 192:307–322.
- Bol-Schoenmakers M, Bleumink R, Rezende MM, Mouser E, Hassing I, Ludwig I, Smit JJ, Pieters RH. 2011. Diclofenac enhances allergic responses in a mouse peanut allergy model. Clin Exp Allergy. 41:424–433.
- Chalasani N, Björnsson E. 2010. Risk factors for idiosyncratic drug-induced liver injury. Gastroenterology. 138:2246–2259.
- Gocinski B, Tigelaar R. 1990. Roles of CD4+ and CD8+ T-cells in murine contact sensitivity revealed by in vivo monoclonal antibody depletion. J Immunol. 144:4121–4128.
- Gomes E, Demoly P. 2005. Epidemiology of hypersensitivity drug reactions. Curr Opin Allergy Clin Immunol. 5:309–316.
- Griem P, Wulferink M, Sachs B, Gonzalez J, Gleichmann E. 1998. Allergic and autoimmune reactions to xenobiotics: How do they arise? Immunol Today. 19:133–141.
- Gutting B, Schomaker S, Kaplan A, Amacher D. 1999. A comparison of the direct and reporter antigen popliteal lymph node assay for the detection of immunomodulation by low molecular weight compounds. Toxicol Sci. 51:71–79.
- Gutting B, Updyke L, Amacher D. 2002a. BALB/c mice orally pretreated with diclofenac have augmented and accelerated PLNA responses to diclofenac. Toxicology. 172:217–230.
- Gutting B, Updyke L, Amacher D. 2002b. Diclofenac activates T-cells in direct popliteal lymph node assay and selectively induces IgG(1) and IgE against co-injected TNP-OVA. Toxicol Lett. 131:167–180.
- Ju C, Uetrecht J. 1999. Detection of 2-hydroxyiminostilbene in the urine of patients taking carbamazepine and its oxidation to a reactive iminoquinone intermediate. J Pharmacol Exp Ther. 288:51–56.
- Kammüller M, Thomas C, De Bakker J, Bloksma N, Seinen W. 1989. The popliteal lymph node assay in mice to screen for the immune disregulating potential of chemicals – a preliminary study. Int J Immunopharmacol. 11:293–300.
- Kang P, Liao M, Wester M, Leeder J, Pearce R, Correia M. 2008. CYP3A4-mediated carbama-zepine (CBZ) metabolism: Formation of a covalent CBZ-CYP3A4 adduct and alteration of the enzyme kinetic profile. Drug Metab Dispos. 36:490–499.
- Kwast L, Fiechter D, Kruijssen L, Bleumink R, Ludwig I, Bol-Schoenmakers M, Smit J, Pieters R. 2016. Oral exposure to immunostimulating drugs results in early changes in innate immune parameters in the spleen. J Immunotoxicol. 15:1–13.
- Kwast L, Fiechter D, Hassing I, Bleumink R, Boon L, Ludwig I, Pieters R. 2011. Oral exposure to drugs with immune-adjuvant potential induces hypersensitivity responses to the reporter antigen TNP-OVA. Toxicol Sci. 121:312–319.
- Lee W. 2003. Drug-induced hepatotoxicity. N Engl J Med. 349:474–485.
- Lertratanangkoon K, Horning M. 1982. Metabolism of carbamazepine. Drug Metab Dispos. 10:1–10.
- Lillibridge J, Amore B, Slattery J, Kalhorn T, Nelson S, Finnell R, Bennett G. 1996. Protein-reactive metabolites of carbamazepine in mouse liver microsomes. Drug Metab Dispos. 24:509–514.
- Martin S, Lappin M, Kohler J, Delattre V, Leicht C, Preckel T, Simon J, Weltzien H. 2000. Peptide immunization indicates that CD8+ T-cells are dominant effector cells in trinitrophenyl-specific contact hypersensitivity. J Investig Dermatol 115:260–266.
- Mauri-Hellweg D, Bettens F, Mauri D, Brander C, Hunziker T, Pichler W. 1995. Activation of drug-specific CD4+ and CD8+ T-cells in individuals allergic to sulfonamides, phenytoin, and carbamazepine. J Immunol. 155:462–472.
- Naisbitt D, Britschgi M, Wong G, Farrell J, Depta J, Chadwick D, Pichler W, Pirmohamed M, Park B. 2003. Hypersensitivity reactions to carbamazepine: Characterization of the specificity, phenotype, and cytokine profile of drug-specific T-cell clones. Mol Pharmacol 63:732–741.
- Naisbitt D, Sanderson L, Meng X, Stachulski A, Clarke S, Park B. 2007. Investigation of the immunogenicity of diclofenac and diclofenac metabolites. Toxicol Lett. 168:45–50.
- Nierkens S, Aalbers M, Bol M, van Wijk F, Hassing I, Pieters R. 2005. Development of an oral exposure mouse model to predict drug-induced hypersensitivity reactions by using reporter antigens. Toxicol Sci. 83:273–281.
- Njoku D. 2010. Suppressive and pro-inflammatory roles for IL-4 in the pathogenesis of experimental drug-induced liver injury: A review. Expert Opin Drug Metab Toxicol. 6:519–531.
- Pichler W, Naisbitt D, Park B. 2011. Immune pathomechanism of drug hypersensitivity reactions. J Allergy Clin Immunol. 127: S74–S81.
- Pieters R. 2001. The popliteal lymph node assay: A tool for predicting drug allergies. Toxicology 158:65–69.
- Pieters R, Albers R. 1999a. Screening tests for autoimmune-related immunotoxicity. Environ Health Perspect. 107S:673–677.
- Pieters R, Albers R. 1999b. Assessment of autoimmunogenic potential of xenobiotics using the popliteal lymph node assay. Methods. 19:71–77.
- Pieters R, Ezendam J, Bleumink R, Bol M, Nierkens S. 2002. Predictive testing for autoimmunity. Toxicol Lett. 127:83–91.
- Reuter B, Davies N, Wallace J. 1997. Nonsteroidal anti-inflammatory drug enteropathy in rats: Role of permeability, bacteria, and enterohepatic circulation. Gastroenterology. 112:109–117.
- Roychowdhury S, Svensson C. 2005. Mechanisms of drug-induced delayed-type hypersensitivity reactions in the skin. AAPS J. 7:E834–E846.
- Switzar L, Kwast L, Lingeman H, Giera M, Pieters R, Niessen W. 2013. Identification and quantification of drug-albumin adducts in serum samples from a drug exposure study in mice. J Chromatogr B Analyt Technol Biomed Life Sci. 917–918:53–61.
- Uetrecht J. 2007. Idiosyncratic drug reactions: Current understanding. Annu Rev Pharmacol Toxicol. 47:513–539.
- Ulrich R. 2007. Idiosyncratic toxicity: A convergence of risk factors. Annu Rev Med.58:17–34.
- Vocanson M, Hennino A, Cluzel-Tailhardat M, Saint-Mezard P, Benetiere J, Chavagnac C, Berard F, Kaiserlian D, Nicolas J. 2006. CD8+ T-cells are effector cells of contact dermatitis to common skin allergens in mice. J Investig Dermatol. 126:815–820.
- Wade L, Kenna J, Caldwell J. 1997. Immunochemical identification of mouse hepatic protein adducts derived from the nonsteroidal anti-inflammatory drugs diclofenac, sulindac, and ibuprofen. Chem Res Toxicol. 10:546–555.
- Ware J, Graf M, Martin B, Lustberg L, Pohl L. 1998. Immunochemical detection and identification of protein adducts of diclofenac in the small intestine of rats: Possible role in allergic reactions. Chem Res Toxicol. 11:164–171.
- Zhang X, Liu F, Chen X, Zhu X, Uetrecht J. 2011. Involvement of the immune system in idiosyncratic drug reactions. Drug Metab Pharmacokinet. 26:47–59.