Abstract
The receptor tyrosine kinase (RTK) Met is the cell surface receptor for hepatocyte growth factor (HGF) involved in invasive growth programs during embryogenesis and tumorgenesis. There is compelling evidence suggesting important roles for c-Met in colorectal cancer proliferation, migration, invasion, angiogenesis, and survival. Hence, a molecular inhibitor of an extracellular domain of c-Met receptor that blocks c-Met-cell surface interactions could be of great thera-peutic importance. In an attempt to develop molecular inhibitors of c-Met, single chain variable fragment (scFv) phage display libraries Tomlinson I + J against a specific synthetic oligopeptide from the extracellular domain of c-Met receptor were screened; selected scFv were then characterized using various immune techniques. Three c-Met specific scFv (ES1, ES2, and ES3) were selected following five rounds of panning procedures. The scFv showed specific binding to c-Met receptor, and significantly inhibited proliferation responses of a human colorectal carcinoma cell line (HCT-116). Moreover, anti- apoptotic effects of selected scFv antibodies on the HCT-116 cell line were also evaluated using Annexin V/PI assays. The results demonstrated rates of apoptotic cell death of 46.0, 25.5, and 37.8% among these cells were induced by use of ES1, ES2, and ES3, respectively. The results demonstrated ability to successfully isolate/char-acterize specific c-Met scFv that could ultimately have a great therapeutic potential in immuno-therapies against (colorectal) cancers.
Introduction
Colorectal cancer (CRC) is one of the most prevalent forms of cancer with 1.2 million new cases diagnosed worldwide annually (van der Horst et al. Citation2009; Gao et al. Citation2015). Recent investigations have found many clinical and pathological biomarkers associated with the prognosis of CRC. Apart from identifying such markers, identification of critical receptors, and signaling pathways are also crucial for development of targeted therapies and improvement of therapeutic efficacy in patients with CRC (Sartore-Bianchi et al. Citation2009).
Many studies have reported on aberrant functions of the c-Met (Mesenchymal Epithelial Transition factor) receptor in the progression and metastasis of numerous human tumors, including carcinomas of the pancreas, stomach, prostate, ovary, breast, liver, and bones, in both melanoma and CRC (van der Horst et al. Citation2009; Watermann et al. Citation2015). The receptor tyrosine kinase (RTK) Met is the cell surface receptor for hepatocyte growth factor (HGF)/scatter factor (SF) (Eder et al. Citation2009). RTK c-Met regulates many processes in the development/progression of cancer cells and has an important role in the proliferation of tumor cells and angiogenesis during tumor maturation (Maulik et al. Citation2002). As such, developing therapeutic that act on the c-Met receptor might be useful in future treatments for CRC and/or other cancers (Jiang et al. Citation2005). Along these lines, various therapeutic strategies such as antagonism of ligand/receptor interactions, tyrosine kinase inhibition, and blocking receptor-effector interactions have been investigated in clinical trials (Eder et al. Citation2009).
It would be expected a c-Met antibody could be used for treatment of many cancer cells, especially CRC cells (Maulik et al. Citation2002; Jiang et al. Citation2005). Because of a smaller size compared to their intact IgG counterparts (∼30 kDa), single-chain variable fragments (scFv) antibodies against c-Met could be employed in imaging tumors, including their penetration and retention (Heo et al. Citation2006; Zehnder-Fjällman et al. Citation2007). Moreover, fragments such as Fab and scFv generated by phage display technology are often more useful in that the process overcomes some limitations inherent with generating whole immunoglobulins for instance cost and immunogenicity. Since prokaryotes such as Escherichia coli can produce fragments that retain antigen-binding capacities and the scFv are completely of human origin; accordingly, humanization steps are not necessary unlike with other monoclonal therapeutics (Carter Citation2001; Kim et al. Citation2008).
The present study utilized phage display technology to isolate single chain fragment variable [scFv] antibodies from a naïve phage library against one specific synthetic oligopeptide from the extracellular domain of a c-Met receptor. After panning, selected scFv were characterized using ELISA, DNA fingerprinting, and sequencing. Moreover, growth inhibitory effects of selected scFv antibodies on an Hct-116 human colon carcinoma cell line were evaluated using formazan (MTT) and Annexin V/PI assays.
Materials and methods
Reagents
One specific immunodominant oligopeptide from the extracellular domain of c-Met, i.e. AQLARQIGASLND (from Sema domain of c-Metm, a conserved ≈ 500 amino acid sequence) was purchased from JPT (Berlin, Germany). Purity was 95% as assessed by HPLC, with mass spectrometry (MS) confirmation of molecular mass. The peptide was re-suspended in water (at 0.2 mg/ml) and then aliquoted and stored at -20 °C. Human single-chain scFv libraries Tomlinson I + J were received from Dr Greg Winter at the MRC Laboratory of Molecular Biology and MRC Center for Protein Engineering (Cambridge, UK). Anti-M13 and anti-C-myc monoclonal antibodies conjugated with horseradish peroxidase (HRP) were bought from Roche (Mannheim, Germany). Isopropyl-β-d-thiogalactopyranoside (IPTG) and DAB (3,3′-diaminobenzidine) were purchased from Sigma (St. Louis, MO). MaxiSorpTM immune tubes and MaxiSorp ELISA plates were bought from Nunc (Roskilde, Denmark). Formazan (MTT) cell proliferation kits were purchased from Sigma. Annexin V/PI apoptosis assay kits were purchased from BD Pharmingen (San Diego, CA).
TG1 and Rosetta-Gami 2 Escherichia coli (Novagen, Madison, WI) were used as hosts for phagemid manipulation and expression of soluble scFv, respectively. An M13KO7 phage (BioScience, Nottingham, UK) was used as a helper phage. MvaI DNA restriction enzyme was purchased from Thermo Scientific (Waltham, MA). Ampicillin, kanamycin, phenyl-methyl-sulfonyl fluoride (PMSF), and triethylamine (TEA) were bought from Sigma. Tryptone, yeast extract, NaCl, and European bacteriological agar were purchased from Laboratorios Conda (Madrid, Spain). All other reagents were purchased from Sigma.
Phage amplification and panning process
Five hundred microliters of phagemid library were added and cultured in 200 ml of 2XYT containing 120 mg ampicillin/ml until the optical density at 600 nm of the suspension (i.e. OD600) reached ≈ 0.4–0.5 in an Eppendorf biophotometer (Hamburg, Germany). About 5 × 1011 PFU (plaque-forming units) of helper phage M13K07 were then added to each milliliter of bacterial culture and incubated for 30 min at 37 °C without agitation and then for another 30 min at 37 °C in a shaker (at 100 rpm). The bacteria were then collected by centrifugation (10 min, 4 °C, 4000 rpm), re-suspended in 100 ml fresh 2XYT containing ampicillin (120 μg/ml) and kanamycin (50 μg/ml), and then incubated for 14–16 h at 30 °C with shaking (200 rpm). There-after, phage were purified according to standard PEG/NaCl precipitation methods (Maniatis et al. Citation1982).
Human single-fold scFv libraries Tomlinson I + J were used to screen high-affinity phage clones against a specific peptide of c-Met. In brief, 10 μg/ml of specific peptide in PBS (phosphate-buffered saline, pH 7.4) or 50 μg/ml of bovine serum albumin (BSA; Sigma) in PBS were coated onto dedicated immunotubes by incubating at 4 °C for 14 h. The tubes were then washed three times with PBS and blocked by incubation with 5% MPBS (5% skim milk in PBS) for 90 min at 37 °C, with gentle shaking. Prepared phage from the library (1012 PFU/ml) in 4 ml of 5% MPBS (Input 1) were then added to the BSA-coated immunotubes and incubated for 1 h at 37 °C. Thereafter, supernatants containing phages that had not bound to BSA were collected and added to c-Met peptide-coated tubes and incubated at 37 °C for 30 min with gentle shaking (50 rpm) and then for a further 30 min without shaking. Subsequently, each immunotube was washed 10 times with PBST (PBS +0.5% [v/v] Tween-20), 10 times with PBS alone, and then twice with double-distilled water. Bound phages were then eluted with 2 ml TEA (triethylamine, 100 mM, pH 10.0). After being held at room temperature (RT) for 10 min, 2 ml Tris-HCl (0.5 M, pH 7.4) buffer was added to neutralize the eluted phage solution (Output 1).
To perform phage amplification, log-phase TG1 (OD600 = 0.5) was infected with eluted phage and M13K07 helper phage; subsequently, propagated phages were collected from the culture supernatants as outlined above (and termed Input 2). Determination of enrichment was performed by estimating the number of rescued phages after each round of panning by estimating the colony forming units (CFU) of the infected E. coli TG1 before the next round of bio-panning was begun. After five rounds of panning, the phage clones were randomly selected to culture for ELISA screening (Sharifzadeh et al. Citation2013).
Polyclonal phage ELISA
Anti-c-Met scFv produced at five rounds of panning can be screened for binding by ELISA to identify "polyclonal" phage antibodies. In brief, MaxiSorp ELISA plates were coated (100 μl/well) with c-Met peptide (50 μg/ml) in PBS (as used for selection) and incubated overnight at 4 °C. Bovine serum albumin (BSA) was also coated on selected wells as negative control in the plates; antigen-free wells were used to assess background binding of c-Met scFv to the plate. The wells were then washed twice with PBS before undergoing blocking, i.e. 250 μl/well of 3% BSA in PBS were added and the plates were incubated 90 min at 37 °C. The wells were then washed once with PBS before 100 μl of PEG-purified phages (1012/well) diluted in 3% BSA-PBS were added and the plate incubated for 30 min with very gentle shaking (50 rpm) and then for 30 min without shaking at 37 °C. The wells were then washed for four times with PBS-0.1% Tween-20. Afterward, a 1:5000 dilution of HRP-conjugated anti-M13 antibody in 0.1% BSA-PBS was added to each well and the plates were incubated again for 60 min at 37 °C [with shaking, 50 rpm]. After three washes with PBS-0.05% Tween 20 and one wash with PBS, 100 μl TMB substrate was added to each well and the plates were incubated at RT in the dark for up to 20 min. Reactions were stopped by an addition of 50 μl 1 M sulfuric acid to each well. The absorbance value in each well was then measured at 450 nm in an ELISA plate reader (Bio-Rad, Hercules, CA). All experiments were carried out in duplicate.
Screening of monoclonal phage-scFv
Phage outputs from the third, fourth, and fifth rounds (pannings) were used to infect exponentially-growing TG1 by incubating at 37 °C for 30 min with shaking (150 rpm) and 30 min without shaking. Infected cells were then spread over LB agar plates containing ampicillin (120 μg/ml) and the plates were incubated overnight at 37 °C. Fifty-six randomly selected phage clones were then picked, the phage were rescued using standard protocols (Sharifzadeh et al. Citation2013), and the isolated scFv were tested for binding to c-Met peptide by phage ELISA as above. An anti-BSA-specific scFv clones were employed as a positive control in the ELISA procedure. Wells coated with BSA but not specific c-Met peptides were used as negative controls.
Expression of soluble c-Met scFv
To produce soluble scFv, E. coli Rosetta-Gami 2 (a non-suppressor strain) was infected with phages that were most reactive in the monoclonal phage ELISA. The expressed scFv could then be used in ELISAs. In briefly, 15 μl competent Rosetta-Gami 2 was incubated in 7 ml LB broth containing 120 mg tetracycline/ml until an OD600 = 0.6 was attained. Subsequently, 50 μl purified scFv-phages were added and the mixture was incubated for 30 min at 37 °C without agitation and then another 30 min on a shaker platform (150 rpm). Thereafter, 7 ml LB broth containing 120 mg ampicillin/ml was added until an OD600 = 0.9 was reached. scFv expression was induced with IPTG. A total of 200 μl of IPTG (1 mmol/l) was then added and the mixture incubated 18 h at 37 °C with shaking (200 rpm). The culture was then centrifuged (3700 rpm, 20 min) and the pellet dissolved in 10 μl PMSF (1 mmol/l). To produce periplasmic extract, each pellet was re-suspended in TES buffer (30 mM Tris-HCl, 1 mM EDTA, 20% Sucrose [pH 8.0]) and the mixture was incubated 60 min at 4 °C with shaking (150 rpm). Thereafter, TES buffer was added and the mixture was incubated 120 min at 4 °C with shaking (150 rpm). A bacterial extract was then generated by centrifuging the culture at 10 000 rpm for 20 min. The supernatant containing the periplasmic protein fraction was collected and analyzed for presence of soluble scFv by ELISA as described above, with the exception of anti-c-Myc antibody conjugated to HRP (1:2000 dilution) to detect soluble scFv fragments. An anti-BSA-specific scFv clone was employed as a positive control in the ELISA procedure. Wells coated with BSA but not specific c-Met peptides were used as negative controls. The absorbance in each well was then measured at 450 nm in an ELISA plate reader (Bio-Rad, Hercules, CA). All experiments were carried out in duplicate.
Identification of c-Met-specific scFv clones by DNA fingerprinting and DNA sequencing
Colony PCR (using primer pairs LMB3 [5′-CAGGAAACAGCTATGAC-3′] and pHEN [5′-CTATGCGGCCCCATTCA-3′]) was performed to investigate the presence of bands corresponding to the full-length VH and Vκ genes. PCR products were examined by electrophoresis over 1.5% agarose gels containing Simply Safe (EURx, Warsaw, Poland) in standard TAE (Tris acetate-EDTA) buffer. After checking the insert size, DNA fingerprinting with Mva-I restriction enzyme was employed to identify scFv patterns (Younesi & Nejatollahi Citation2014). For this, 20 μl each PCR product was mixed with 2 μl restriction enzyme buffer/1 μl frequent-cutting Mva I, and the sample then incubated/digested for 2 h at 37 °C. The samples were then resolved over a 2% agarose gel. Subsequently, individual clones with the most frequent pattern were selected and these clones were then analyzed by DNA sequencing.
Specificity of soluble scFv
To evaluate any cross-reactivity of selected scFv with various antigens, ELISA was employed. In brief, 50 μg/ml of specific peptides, i.e. those of c-Met, insulin-like growth factor 1 receptor (IGF-1R), 4–1BB receptor (CD137), receptor tyrosine kinase-like orphan receptor 1 (ROR1), and 0.5 μg/ml programmed cell death protein 1 (PD-1), skimmed milk, and BSA were individually coated onto dedicated wells (i.e. overnight, 4 °C as above) within 96-well ELISA plates. Wells were then washed, blocked (as above), and then treated with selected scFv. After incubation at 37 °C for 90 min, scFv bound to given wells were detected by treatment of the wells with HRP-anti C-myc (1:2000 dilution) in 0.1% BSA-PBS and incubation for 60 min at 37 °C. After washing the wells three times with PBS-0.05% Tween 20 and once with PBS, 100 μl TMB substrate solution was added to each well and the plate incubated in the dark at RT for up to 20 min. After addition of 50 μl stop solution to each well, the absorbance at 450 nm was assessed in the plate reader. All experiments were done in triplicate.
Western blot analyses
Anti-c-Met scFv antibodies existing in periplasmic extracts from E. coli Rosetta-Gami 2 were isolated by standard osmotic shock protocol (Sonoda et al. Citation2011). Total protein content was determined using a Bradford assay and all samples were adjusted to the same protein concentration using PBS to enable loading of equal amounts of protein (1 μg) per sample onto 10.5% SDS-PAGE gels. Resolved proteins were then electrotransferred to nitrocellulose membranes that were in turn blocked at 4 °C overnight in 5% MPBS. Each membrane was then incubated for 2 h at 37 °C (with gentle agitation) in a solution of PBS containing a 1:2000 dilution of HRP-anti C-myc. Each membrane was then washed six times with PBS-Tween 0.1% buffer and signals revealed by treatment with 3,3′-diaminobenzidine substrate (DAB). The membranes were then analyzed using densitometry (1D Image Analysis Software, Kodak, Rochester, NY) to quantify signal intensities.
Cell proliferation assay
Activated c-Met is known to trigger cancer cell proliferation (He et al. Citation2014). Hct-116 human colon carcinoma cells were obtained from the Pasture Institute cell bank (Tehran, Iran) and cultured in RPMI 1640 supplemented with 10% fetal bovine serum (FBS), 100 μg strepto-mycin/ml, and 100 U penicillin/ml (Gibco, Grand Island, NY) at 37 °C in a humidified 5% CO2 incubator). For use in the assays, Hct-116 cells were seeded into 96-well plates (14 000 cells/well) in 200 μl RPMI supplemented with 15% FBS and 100 ng HGF/ml) and the plates were incubated at 37 °C for 24 h. The next day, medium in each well was replaced with medium containing 5% FBS and 100 ng HGF/ml. The cells were then treated with various amounts of selected anti c-Met scFv (5000 or 10 000 scFv/cell) or 10 μl DMSO (positive control) for 24 h at 37 °C. Cell proliferation was then determined using an MTT assay, according to manufacturer’s instructions. Absorbance in each well was measured in the plate reader at 570 nm. Each experiment was repeated a minimum of three times.
Annexin V-FITC/PI assay
Apoptosis was assessed using an Annexin V-FITC apoptosis detection kit according to manufacturer’s protocols. In brief, HCT-116 cells were seeded into 24-well culture plates (density of 2 × 104 cells/well) and treated with HGF (100 ng/ml) overnight at 37 °C. The medium in the well was then replaced with fresh medium containing 100 ng HGF/ml and selected scFv (5000 scFv/cell) or irrelevant scFv (5000 scFv/cell) and the plates incubated for a further 24 h at 37 °C. The cells were then harvested and washed twice with ice-cold PBS and then re-suspended in a solution containing with Annexin V-FITC and PI (each in kit-provided binding buffer) in the dark for 15 min at room temperature. Immediately thereafter, the cells were analyzed in a FACS Calibur system (Becton Dickinson, Mountain View, CA) using system-associated FloMax software (TreeStar Inc., Ashland, OR). Each assay was repeated three times. A minimum of 10 000 events were captured for each sample analysis.
Statistical analysis
All results are expressed as means ± SD. All analyses of the data were performed using a one-way analysis of variance (ANOVA) and t-tests. All evaluations were performed using Prism software (Graph Pad, San Diego, CA). A p value <0.05 was accepted as statistically significant.
Results
Selection of phage-displayed scFvs
After five rounds of affinity selection, by counting the colony forming units (CFU) of the infected E. coli TG1 was estimated as the phage titer. The data show that the phage titer of the fifth round was 937-fold greater than that of the first round ().
Figure 1. (A) Titration results of c-Met-specific phages after bio-panning of the Tomlinson library. (B) Polyclonal phage and (C) Monoclonal Phage ELISA of selected scFvs against c-MET peptides. From 56 clones, 26 presented with positive reactivity to c-Met peptide. Data shown are means [± SD] of OD values from duplicate experiments. An arbitrary cutoff 2.5 times greater than the negative control was considered positive. Positive control: anti BSA scFv, negative control: BSA.
![Figure 1. (A) Titration results of c-Met-specific phages after bio-panning of the Tomlinson library. (B) Polyclonal phage and (C) Monoclonal Phage ELISA of selected scFvs against c-MET peptides. From 56 clones, 26 presented with positive reactivity to c-Met peptide. Data shown are means [± SD] of OD values from duplicate experiments. An arbitrary cutoff 2.5 times greater than the negative control was considered positive. Positive control: anti BSA scFv, negative control: BSA.](/cms/asset/5586c12e-5590-4c27-b2fc-a450b1edeae7/iimt_a_1251512_f0001_b.jpg)
Polyclonal and monoclonal phage ELISA
As shown in , the optical density (OD) values of wells coated with IGF-IR peptide were higher than those of uncoated wells and, further, increased round by round, indicating the effectiveness of the panning strategy. Fifty-six phage clones from Outputs 3, 4, and 5 were randomly selected and assessed for c-Met binding activity in the monoclonal phage ELISA. The data showed that 26 different clones were identified that specifically bound to c-Met, but not to BSA control protein (an OD >2.5-fold greater than the OD associated with the negative control) ().
Screening of clones by soluble phage ELISA
Anti c-Met scFv were expressed in soluble forms in non-suppressor E. coli Rosetta-Gami-2. Preparations of soluble scFv from periplasmic extracts were evaluated using indirect ELISA for reactivity to c-Met peptide. As shown in , 16 out of 26 preparations of the soluble scFv reacted with c-Met.
Figure 2. Production of soluble scFv. Binding of soluble scFv to c-Met and BSA wells was assessed by ELISA. Data are presented as means [± SD] of OD values from duplicate experiments. An arbitrary cutoff 2.5 times greater than the negative control was considered positive. Positive control: anti BSA scFv, negative control: BSA.
![Figure 2. Production of soluble scFv. Binding of soluble scFv to c-Met and BSA wells was assessed by ELISA. Data are presented as means [± SD] of OD values from duplicate experiments. An arbitrary cutoff 2.5 times greater than the negative control was considered positive. Positive control: anti BSA scFv, negative control: BSA.](/cms/asset/657baba7-439a-4014-9e4a-2e1b7ac192c2/iimt_a_1251512_f0002_b.jpg)
Identification of c-Met-specific scFv clones by DNA fingerprinting and DNA sequencing
scFv genes from these 16 selected clones were amplified using LMB3 and pHEN primers, and PCR products were analyzed in agarose gel to confirm the presence of the desired bands corresponding to the VH and Vκ inserts (≈ 935 bp). Of the 16 clone, 9 presented a band with the corresponding size (). DNA fingerprinting with Mva-I restriction enzyme showed there were four different digestion patterns (). Nucleotide sequencing revealed unique sequences in 3 out of 4 clones (). These scFv - thereafter referred to as ES1, ES2 and ES3 - were employed for further characterization and functionality analysis.
Figure 3. (A) Amplification of scFv fragments by PCR (VH and Vκ insert, ≈ 935 bp). Lane M: 100 bp +3K DNA ladder. Lanes 1–9: amplified scFv gene in 9 clones. (B) Mva-I DNA finger-printing of scFv clones, scFv genes were amplified by PCR then digested with Mva-I (BstNI) and separated over an agarose gel. Lane M: 100 bp +3K DNA ladder. Lanes 1–9: single clones digested by Mva-I. Two common patterns were seen in lanes 1, 3, and 7, and in lanes 2, 4, 8 and 9. (C) Western blot analysis of expressed scFv. Lane 1, 2, and 3: expressed products of ES1-scFv, ES2-scFv, and ES3-scFv, respectively. Lane 4: total lysate from non-induced E. coli Rosetta-Gami 2. A representative blot is shown.
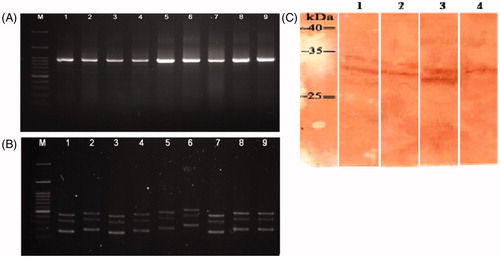
Table 1. VDJ genes and CDR3 sequences of anti c-Met scFv.
Specificity
For evaluating the specific binding of soluble scFv, cross-reactivity ELISA was used by c-Met, IGF-1R, CD137, ROR1 peptide antigens and different proteins including PD-1, skimmed milk, and BSA. As indicated in , all the selected scFv reacted to specific peptides. However, no evident reactivity to a panel of irrelevant proteins was seen.
Table 2. Specificity of soluble scFv.
Western blot analyses
To evaluate the expression of soluble scFv protein, immunoblotting assays was done using anti-C-Myc antibody. Representative results demonstrated a specific reactivity of anti-C-Myc tag antibody with scFv protein at the expected target protein (∼30 kDa) molecular weight ().
Functional inhibition assay
The anti-proliferative effects of anti-c-Met scFv antibodies in HCT-116 cell line are shown in . The percentages of the growth inhibition after 24 h treatments of HCT-116 cells were 76.84% for ES1, 72.11% for ES2 and 65.59% for ES3 when compared to the untreated cells. The results obtained following the treatment of the HCT-116 cell line with an unrelated scFv (as negative control) had significantly lower growth compared to that by cells treated with ES1, ES2, and ES3 (p = 0.001, p = 0.003, and p = 0.02, respectively).
Figure 4. Functional inhibition assay. (A) Proliferation of HCT-116 cells in presence of HGF (100 ng/ml) (p = 0.05 vs. untreated cells). (B) Proliferation inhibited by ES1, ES2 and ES3 (77%, 72%, and 66%, respectively. (p = 0.001, p = 0.003, p = 0.002, respectively, vs. effect from an unrelated scFv). Data shown are means ± SD of OD of three independent experiments.
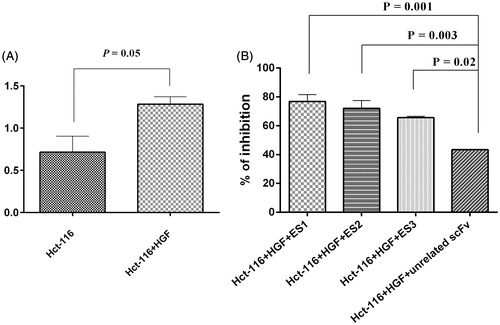
Annexin V/PI apoptosis assay
Apoptosis was assessed by Annexin V-FITC/PI staining. The results presented in illustrate that after 24 h, ≈ 58.3% of all untreated HCT-116 cells were alive (Annexin V-/PI-), and that 16.5 (± 1.00)% of the cells were characterized as early (Annexin V+/PI−)/late (Annexin V+/PI+) apoptotic cells. After a 24 h treatment with anti-c-Met scFv (ES1), 46.0 (± 1.09)% of the cells were apoptotic (p < 0.001 vs. control level); results of the study using ES2 and ES3 were that 25.5 (± 0.86) and 37.8 (± 0.90)% of the cells were now in this state (p < 0.001). In HCT-116 cells that had been treated with an unrelated scFv (anti-BSA scFv) for 24 h, the percentage of apoptotic cells was just 7.2% was obtained ( and ).
Figure 5. Annexin V/PI apoptosis assay in HCT-116 cells treated with anti-c-Met scFv. Apop-totic death was analyzed in cells treated for 24 h with unrelated scFv (negative control), HGF, ES1, ES2, or ES3. Apoptotic cell death was characterized by Annexin V+/PI- and Annexin V+/PI+ staining status using FACS. (A) Untreated cells. Cells treated with (B) unrelated scFv, (C) ES1scFv, (D) ES2 scFv, or (E) ES3 scFv.
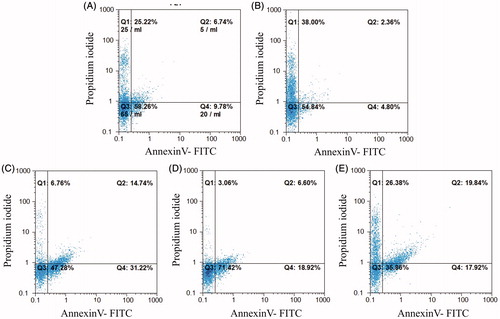
Discussion
Dysregulation of HGF/c-Met signaling has been described in a wide variety of human cancers. The involvement of HGF/c-Met function in tumor formation, progression, and dissemination suggests targeting c-Met signaling pathway is a significant potential in the therapeutic strategy (Gherardi et al. Citation2012). c-Met has been proposed as a therapeutic target for colorectal cancer (CRC) in several studies (Gao et al. Citation2015; van der Horst et al. Citation2009). Over-expression of c-Met has been observed in 30–70% of CRC tumors (Takeuchi et al. Citation2003; Zeng et al. Citation2004). In CRC, c-Met expression at the mRNA and protein levels has been reported in 30–91% of samples assayed by Northern blot and reverse transcription polymerase chain reaction (RT-PCR) and 57–100% by Western blot and IHC analyses (Takeuchi et al. Citation2003).
Many studies have reported a significant over-expression of c-Met mRNA and protein in CRC tumors compared with levels in adjacent normal colon mucosa. For example, Zeng et al. (Citation2004) reported that 69% of primary CRCs demonstrated c-Met protein over-expression. Moreover, using c-Met Monoclonal antibodies (MAb) to investigate the distribution of c-Met protein in human normal and neoplastic tissues, it was revealed that the receptor was less detectable in the normal tissues (Prat et al. Citation1991). Because of its over-expression in CRC - but low expression levels in normal tissues, c-Met has emerged as a promising target for the CRC treatment. Therefore, there is an essential need to develop inhibitors of c-Met receptor that may be useful in the management of CRC. Along these lines, targeting of growth factor receptors using MAb is an effective strategy in cancer therapy because of an inherent increased specificity and low toxicity (Nahta et al. Citation2003). Some strategies like those that employed anti-c-Met MAb have entered clinical trials for treatment of CRC (Greenall et al. Citation2012; van der Horst et al. Citation2009).
Phage antibody display is an effective in vitro method for the selection of tumor-specific recombinant human antibodies (Zhao et al. Citation2016). Recombinant human single chain variable fragments (scFv) have been developed against various therapeutic targets for cancer treatments (Tao et al. Citation2010). Because of some advantages of scFv over whole (intact) antibodies, scFv have been utilized as tools for targeting cancer biomarkers, and it is expected that anti-c-Met scFv are likely to be beneficial in the clinical treatment or imaging of many cancer cells (Edwardraja et al. Citation2010; Gupta et al. Citation2010).
In the present study, scFv antibodies (i.e. ES1, ES2, and ES3) against specific oligo-peptides from the extracellular domain of the c-Met receptor were isolated and characterized for their tumor binding and inhibitory effects in a colorectal cancer cell line. Multiple panning processes (that included a pre-absorption step at each round) were employed to reduce the number of false-positive phage clones. After five rounds of panning, the phage titer was ≈1000-fold increased relative to that after just one round. Characterizations showed the phages to be c-Met specific. PCR analyses confirmed the presence of full length VH and Vκ inserts and DNA fingerprinting with Mva-I restriction enzyme revealed three distinct VH and Vκ sequences among four clones (ES1, ES2, and ES3). Functionality of these scFv was confirmed by assessing their impact on growth of HCT-116 human colon cancer cells that - upon HGF stimulation - have a high level of phosphorylated c-Met (Kwon et al. Citation2015). The results showed ES1 and ES2 effectively inhibited HCT-116 proliferation; equivalent concentrations of irrelevant scFv had no significant effect on the cell proliferation. Anti-apoptotic effects of the selected scFv on HCT-116 cell lines were also investigated. The results of Annexin V/PI assays here were consistent with those of the formazan (MTT) cell proliferation assays. Although a minority of untreated and unrelated scFv treated cells were apoptotic, significant apoptosis effect was observed in cells treated with the anti-c-Met scFv. Thus, the proliferative and apoptotic effects of ES1, ES2, and ES3 scFv on the HCT-116 cells suggested they were, in fact, efficient c-Met receptor inhibitors.
Studies have demonstrated that growth inhibition and induction of apoptosis pathways in specific cell lines was caused by specific binding of anti c-Met scFv to c-Met (Liu et al. Citation2013; Lu et al. Citation2011). In a similar study (van der Horst et al. Citation2009), the effect of two fully human anti-MET monoclonal antibody (R13 and R28) on the proliferation of various colon tumor xenograft models, was investigated. R13 and R28 synergistically inhibited HGF binding to MET and cell-based phosphorylation assays indicated R13 and R28 abolished HGF-induced activation of MET, AKT1, and ERK1/2, as well as HGF-induced migration and proliferation. Onartuzumab (Met mAb), a recombinant humanized monoclonal monovalent anti-c-Met antibody that binds the extracellular domain of c-Met, blocks ligand binding and subsequent activation by HGF, resulting in suppressed growth of multiple cancer models (Bendell et al. Citation2013; Surati et al. Citation2011). In another study by Martens et al. (Citation2006), the effect of humanized anti-c-Met antibody one-armed 5D5 (OA-5D5) on proliferation of and apoptosis in U87 glioblastoma cells was investigated. The authors identified multiple potential mechanisms for the effect of this antibody, including both direct effects on tumor cells as well as upon tumor vasculature/vascularization. Their findings suggested that anti-c-Met antibody one-armed 5D5 had anti-proliferative and apoptotic effects that contributed to the inhibition of tumor growth in-vivo. At this point, the in situ efficacy (as well as effects on other cell lines) of the novel scFv remains to be determined.
In comparison to previously studied anti-c-MET antibodies, which were discussed above, the new c-MET scFv here showed more anti-proliferative and apoptotic effects against the human colorectal carcinoma cell line (HCT-116). Moreover, this scFv was isolated from a fully human phage display library. As such, there is less risk it would elicit immune responses in a treated subject in comparison to as has been the case previously with chimeric and humanized antibodies. Other advantages of the novel scFv here over intact antibodies are its smaller size, high affinity to c-Met receptor, low cost, and ease of production. Moreover, human scFv fragments show better penetration into tumor tissues and they also clear rapidly from the blood and may have lower renal retention than monoclonal antibodies or Fabs. These additional factors make it suitable for potential use in targeting c-Met in colorectal cancer therapies (Abdolalizadeh et al. Citation2013; Jhanwar & Divgi Citation2005; Nejatollahi et al. Citation2013; Sadreddini et al. Citation2015; Younesi & Nejatollahi Citation2014).
In summary, this study developed a protocol that helped to introduce a novel human anti-c-Met scFv. The major conclusion to be drawn from this study was that ES1, ES2, and ES3 appear to possess in vitro properties well-suited for c-Met receptor targeting. Nevertheless, further investigations need to be done to establish whether the selected scFv have (other) in vitro and in vivo efficacies to act as anti-tumor agents.
Disclosure statement
The authors declare no conflicts of interest. The authors alone are responsible for the content and writing of the paper.
Additional information
Funding
References
- Abdolalizadeh J, Nouri M, Majidi Zolbanin J, Barzegari A, Baradaran B, Barar J, Coukos G, Omidi Y. 2013. Targeting cytokines: Production and characterization of anti-TNF-α scFvs by phage display technology. Curr Pharm Des. 19:2839–2847.
- Bendell J, Ervin T, Gallinson D, Singh J, Wallace J, Saleh M, Vallone M, Phan S, Hack S. 2013. Treatment rationale and study design for a randomized, double-blind, placebo-controlled Phase II study evaluating onartuzumab (Met MAb) in combination with bevacizumab plus mFOLFOX-6 in patients with previously untreated metastatic colorectal cancer. Clin Colo-Rectal Cancer. 12:218–222.
- Carter P. 2001. Improving the efficacy of antibody-based cancer therapies. Nat Rev Cancer. 1:118–129.
- Eder J, Woude G, Boerner S, LoRusso P. 2009. Novel therapeutic inhibitors of the c-Met signaling pathway in cancer. Clin Cancer Res. 15:2207–2214.
- Edwardraja S, Neelamegam R, Ramadoss V, Venkatesan S, Lee S. 2010. Redesigning of anti-c-Met single chain Fv antibody for the cytoplasmic folding and its structural analysis. Biotechnol. Bioeng. 106:367–375.
- Gao H, Guan M, Sun Z, Bai C. 2015. High c-Met expression is a negative prognostic marker for colorectal cancer: A meta-analysis. Tumour Biol. 36:515–520.
- Gherardi E, Birchmeier W, Birchmeier C, Woude G. 2012. Targeting MET in cancer: rationale and progress. Nat Rev Cancer. 12:89–103.
- Greenall S, Gherardi E, Liu Z, Donoghue J, Vitali A, Li Q, Murphy R, Iamele L, Scott A, Johns T. 2012. Non-agonistic bivalent antibodies that promote c-MET degradation and inhibit tumor growth and others specific for tumor related c-MET. PLoS One. 7:e34658. doi: 10.1371/journal.pone.0034658.
- Gupta P, Han S, Holgado-Madruga M, Mitra S, Li G, Nitta R, Wong J. 2010. Development of an EGFRvIII specific recombinant antibody. BMC Biotechnol. 10:72. doi: 10.1186/1472-6750-10-72.
- He C, Ai J, Xing W, Chen Y, Zhang H, Huang M, Hu Y, Ding J, Geng M. 2014. Yhhu3813 is a novel selective inhibitor of c-Met kinase that inhibits c-Met-dependent neoplastic phenotypes of human cancer cells. Acta Pharmacol Sinica. 35:89–97.
- Heo M, Kim S, Kim S, Kim Y, Chung J, Oh M, Lee S. 2006. Functional expression of single-chain variable fragment antibody against c-Met in the cytoplasm of Escherichia coli. Protein Express Purif. 47:203–209.
- Jhanwar Y, Divgi C. 2005. Current status of therapy of solid tumors. J Nuclear Med. 46:141S–150S.
- Jiang W, Martin T, Parr C, Davies G, Matsumoto K, Nakamura T. 2005. Hepatocyte growth factor, its receptor, and their potential value in cancer therapies. Crit Rev Oncol Hematol. 53:35–69.
- Kim Y, Neelamegam R, Heo M, Edwardraja S, Paik H, Lee S. 2008. Improving the productivity of single-chain Fv antibody against c-Met by rearranging the order of its variable domains. J. Microbiol Biotechnol. 18:1186–1190.
- Kwon Y, Smith B, Zhou Y, Kaufman M, Godwin A. 2015. Effective inhibition of c-MET-mediated signaling, growth and migration of ovarian cancer cells is influenced by the ovarian tissue microenvironment. Oncogene. 34:144–153.
- Liu Z, Feng Z, Zhu X, Xu W, Zhu J, Zhang X, Fan Z, Ji G. 2013. Construction, expression, and characterization of an anti-tumor immunotoxin containing the human anti-c-Met single-chain antibody and PE38KDEL. Immunol Lett. 149:30–40.
- Lu R, Chang Y, Chen M, Wu H. 2011. Single chain anti-c-Met antibody conjugated nanoparticles for in vivo tumor-targeted imaging and drug delivery. Biomaterials. 32:3265–3274.
- Maniatis T, Fritsch E, Sambrook J, editors. 1982. Molecular cloning: A laboratory manual. Cold Spring Harbor, NY: Cold Spring Harbor Laboratory.
- Martens T, Schmidt N, Eckerich C, Fillbrandt R, Merchant M, Schwall R, Westphal M, Lamszus K. 2006. A novel one-armed anti-c-Met antibody inhibits glioblastoma growth in vivo. Clin Cancer Res. 12:6144–6152.
- Maulik G, Shrikhande A, Kijima T, Ma P, Morrison P, Salgia R. 2002. Role of the hepatocyte growth factor receptor, c-Met, in oncogenesis and potential for therapeutic inhibition. Cytokine Growth Factor Rev. 13:41–59.
- Nahta R, Hortobagyi G, Esteva FJ. 2003. Growth factor receptors in breast cancer: Potential for therapeutic intervention. Oncologist. 8:5–17.
- Nejatollahi F, Abdi S, Asgharpour M. 2013. Anti-proliferative and apoptotic effects of a specific anti-prostate stem cell single chain antibody on human prostate cancer cells. J Oncol. 2013:831–839.
- Prat M, Narsimhan R, Crepaldi T, Rita Nicotra M, Natali P, Comoglio P. 1991. The receptor encoded by the human c-MET oncogene is expressed in hepatocytes, epithelial cells and solid tumors. Int J Cancer 49:323–328.
- Sadreddini S, Seifi-Najmi M, Ghasemi B, Kafil H, Alinejad V, Sadreddini S, Younesi V, Jadidi-Niaragh F, Yousefi M. 2015. Design and construction of immune phage antibody library against tetanus neurotoxin: Production of single chain antibody fragments. Human Anti-Bodies. 23:73–79.
- Sartore-Bianchi A, Di Nicolantonio F, Nichelatti M, Molinari F, De Dosso S, Saletti P, Martini M, Cipani T, Marrapese G, Mazzucchelli L. 2009. Multi-determinants analysis of molecular alterations for predicting clinical benefit to EGFR-targeted monoclonal antibodies in colorectal cancer. PLoS One. 4:e7287. doi: 10.1371/journal.pone.0007287.
- Sharifzadeh Z, Rahbarizadeh F, Shokrgozar M, Ahmadvand D, Mahboudi F, Jamnani F, Bakhtiari S. 2013. Development of oligoclonal nanobodies for targeting the tumor-associated glycoprotein 72 antigen. Mol Biotechnol. 54:590–601.
- Sonoda H, Kumada Y, Katsuda T, Yamaji H. 2011. Effects of cytoplasmic and periplasmic chaperones on secretory production of single-chain Fv antibody in Escherichia coli. J Biosci Bioeng. 111:465–470.
- Surati M, Patel P, Peterson A, Salgia R. 2011. Role of MetMAb (OA-5D5) in c-MET active lung malignancies. Expert Opin Biol Ther. 11:1655–1662.
- Takeuchi H, Bilchik A, Saha S, Turner R, Wiese D, Tanaka M, Kuo C, Wang H, Hoon D. 2003. c-MET expression level in primary colon cancer: A predictor of tumor invasion and lymph node metastases. Clin Cancer Res. 9:1480–1488.
- Tao J, Xiang J, Li D, Deng N, Wang H, Gong Y. 2010. Selection and characterization of a human neutralizing antibody to human fibroblast growth factor-2. Biochem Biophys Res Commun. 394:767–773.
- van der Horst E, Chinn L, Wang M, Velilla T, Tran H, Madrona Y, Lam A, Ji M, Hoey T, Sato A. 2009. Discovery of fully human anti-MET monoclonal antibodies with anti-tumor activity against colon cancer tumor models in vivo. Neoplasia. 11:355–364.
- Watermann I, Schmitt B, Stellmacher F, Müller J, Gaber R, Kugler C, Reinmuth N, Huber R, Thomas M, Zabel P. 2015. Improved diagnostics targeting c-MET in non-small cell lung cancer: Expression, amplification, and activation? Diagn Pathol. 10:130. doi: 10.1186/s13000-015-0362-5.
- Younesi V, Nejatollahi F. 2014. Induction of anti-proliferative and apoptotic effects by anti-IL-25 receptor single chain antibodies in breast cancer cells. Int Immunopharmacol. 23:624–632.
- Zehnder-Fjällman A, Marty C, Halin C, Hohn A, Schibli R, Ballmer-Hofer K, Schwendener R. 2007. Evaluation of anti-VEGFR-3 specific scFv antibodies as potential therapeutic and diagnostic tools for tumor lymph-angiogenesis. Oncol Reports. 18:933–941.
- Zeng Z, Weiser M, D’Alessio M, Grace A, Shia J, Paty P. 2004. Immunoblot analysis of c-Met expression in human colorectal cancer: Over-expression is associated with advanced stage cancer. Clin Exp Metastasis. 21:409–417.
- Zhao A, Tohidkia M, Siegel D, Coukos G, Omidi Y. 2016. Phage antibody display libraries: A powerful antibody discovery platform for immunotherapy. Crit Rev Biotechnol. 36:276–289.