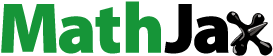
ABSTRACT
This position stand aims to provide an evidence-based summary of the energy and nutritional demands of tactical athletes to promote optimal health and performance while keeping in mind the unique challenges faced due to work schedules, job demands, and austere environments. After a critical analysis of the literature, the following nutritional guidelines represent the position of the International Society of Sports Nutrition (ISSN).
General Recommendations
Nutritional considerations should include the provision and timing of adequate calories, macronutrients, and fluid to meet daily needs as well as strategic nutritional supplementation to improve physical, cognitive, and occupational performance outcomes; reduce risk of injury, obesity, and cardiometabolic disease; reduce the potential for a fatal mistake; and promote occupational readiness.
Military Recommendations
Energy demands should be met by utilizing the Military Dietary Reference Intakes (MDRIs) established and codified in Army Regulation 40-25. Although research is somewhat limited, military personnel may also benefit from caffeine, creatine monohydrate, essential amino acids, protein, omega-3-fatty acids, beta-alanine, and L-tyrosine supplementation, especially during high-stress conditions.
First Responder Recommendations
Specific energy needs are unknown and may vary depending on occupation-specific tasks. It is likely the general caloric intake and macronutrient guidelines for recreational athletes or the Acceptable Macronutrient Distribution Ranges for the general healthy adult population may benefit first responders. Strategies such as implementing wellness policies, setting up supportive food environments, encouraging healthier food systems, and using community resources to offer evidence-based nutrition classes are inexpensive and potentially meaningful ways to improve physical activity and diet habits. The following provides a more detailed overview of the literature and recommendations for these populations.
1. Introduction
Military personnel, law enforcement officers, and firefighters perform some of our society’s most physically demanding jobs [Citation1,Citation2–4]. For instance, essential job tasks related to these occupations may include lifting and carrying heavy and odd objects and tools, casualty extraction, moving rapidly to obtain a tactical advantage or maintain safety, and forcible entry, as well as high cognitive demand, during life and death decisions under high stress and austere conditions. Typically, these tasks are performed while wearing personal protective equipment (PPE) weighing approximately 10–50 kg [Citation5,Citation6]. Based on the occupational demands and the need for performance-based training, these individuals are often referred to as ‘Tactical Athletes’ [Citation7] ().
In recent years, there has been an increased emphasis on developing evidence-based physical training methodologies and strategies aimed at maintaining and improving occupational and physical performance, as well as reducing the risk of injury and disease within these populations. Subsequently, the importance of providing nutritional guidance and identifying best practices for fueling tactical athletes has become paramount. This position stand aims to provide practical guidance and recommendations for tactical athletes with respect to evidence-based nutritional strategies that promote health and occupational performance.
2. Methods
International Society of Sports Nutrition (ISSN) position stands are invited papers the ISSN Editors and Research Committee identify as being of interest to scientists and practitioners. Editors and/or the Research Committee identify a lead author or team of authors to perform a comprehensive literature review. A review of the scientific literature was conducted related to the tactical athlete, tactical athlete nutrition, warfighters, first responders, supplementation, health, performance, and operational readiness. This was accomplished by conducting key word searches related to the tactical athlete, as well as nutrition, on each topic summarized using the National Institutes for Health National Library of Medicine PubMed.gov search engine. The draft is then sent to leading scholars for review and comment. The paper is then revised as a consensus statement and reviewed and approved by the Research Committee and Editors as the official position of the ISSN.
3. Part I – Military
3.1. Physiological and Energy Expenditure Demands of Military
3.1.1. Duty-Specific Tasks and Common Stressors
The physiological demands placed upon military personnel vary considerably based on the physical requirements demanded of the operator (i.e. military occupation specialty [MOS]), the mission of the unit, and deployment status. Military populations are heterogeneous as some military personnel work on primarily sedentary jobs (i.e., financial management technician), while others may engage in near-continuous physical tasks (i.e., deploying special operations Operator). Regardless, it is important for the warfighter to be physically and mentally ready when deployments are initiated and the pace of daily operations increases (i.e., operational tempo [OPTEMPO]). The modern-day warfighter faces a barrage of occupational challenges such as load carriage, heavy lifting, offensive and defensive combat/maneuvers, sprinting, and casualty evacuations [Citation8]. Given the varying or unknown challenges of multi-domain operations (and future warfare), it is important for military personnel to be both anaerobically and aerobically conditioned, with most military operations requiring steady-state effort and occupational-specific tasks for a protracted period of hours or days.
Prolonged operations may leave warfighters without adequate energy intake, which can lead to poor physical performance and decision-making [Citation9]. Warfighters are commonly exposed to hypo-energetic states, particularly during sustained periods of training or combat operations, which may lead to large energy deficits (i.e., 2,500–4,500 kcal/day) [Citation10,Citation11]. The United States Army Research Institute of Environmental Medicine (USARIEM) conducted studies demonstrating decrements in muscular strength and power following severe weight loss among participants in the U.S. Army Ranger School [Citation10,Citation12]. These physiological stressors are typically coupled with sleep deprivation, which can further exacerbate decrements in physical and mental performance [Citation13]. Indeed, Nindl et al. [Citation12] reported the approximate caloric deficit to be 3,000 kcal/day during these periods of training and operations. This imbalance was largely responsible for the observed loss of body mass (~3.1%), poor sleep (~3.6 h over a 72-h duration), and self-reported increases in depression, anger, fatigue, tension, and confusion [Citation12]. While unpredictable, warfighters should seek and exploit opportunities to maximize the delivery of nutrient-dense foods while continuing with regular physical training to maximize operational readiness. In general, implementing strategies to combat negative energy balance status will best prepare the warfighter during sustained periods of training and combat and other protracted periods of tactical-specific activities.
Military personnel are subjected to environmental stressors, such as heat, cold, and high altitude, which may impair their ability to perform occupational tasks. Unacclimated individuals may be required to travel to geographical locations on short notice with severe environmental conditions. While having ‘boots on the ground’ is important to establish a military presence in locations of conflict, warfighters might be subjected to limited time or opportunity to acclimatize to new environments. In this respect, inadequate heat adaptation can leave the warfighter more susceptible to heat-related illnesses such as exertional heat stroke [Citation14,Citation15]. Similar to other athletes, physically fit warfighters fare much better in terms of physiological adaptations and acclimatization [Citation16,Citation17], specifically those who are aerobically well trained [Citation18]. In terms of high-altitude exposure (i.e., 1,500–3000 m, 3,000–5,000 m, and 5,000 m or above are considered high-, very high-, and extreme altitude levels), aerobic/submaximal activities can be compromised at higher altitudes due to decreases in barometric pressure. High-altitude cerebral edema, high altitudes pulmonary edema, and acute mountain sickness are all potential conditions that can impact the performance of military personnel conducting operations at high altitudes [Citation19–21]. Common symptoms associated with acute mountain sickness include disorientation, headaches, nausea, vomiting, fatigue, dizziness, and irritability, which can all negatively affect physical and cognitive performance [Citation22], while altitudes greater than 3,000 m have been demonstrated to impair psychomotor performance, reaction time, vigilance, memory, and logical reasoning [Citation23,Citation24]. Lastly, physical and cognitive performance decrements may also result from cold exposure (e.g., reductions in muscular strength) [Citation20]. Military field operations conducted in cold environments may result in a reduction in physical performance (e.g., anaerobic power) [Citation25]. Furthermore, the risk of dehydration in cold environments is a major concern [Citation20]. It is important to note that these environmental stressors exacerbate these physiological responses in warfighters. These changes do not include the additional physical burden associated with load carriage requirements.
3.1.2. Physical fitness considerations and energy expenditure
While Service members often engage in long work periods at submaximal intensities, various tasks may be high intensity in nature and include heavy load carriage; thus, it is also important that military personnel to possess adequate levels of muscular endurance, strength, and power. Traditionally, warfighters have been subjected to heavy load carriage, and that load has progressively increased over the past 10 to 20 years [Citation26]. Loads include equipment (i.e., weapon systems, ammunition, and provisions) and PPE, such as ballistic helmets, body armor, load-bearing vests, chest rigs, patrol packs, and boots [Citation27]. Energy cost [Citation28,Citation29], altered gait mechanics [Citation4,Citation30,Citation31], and risk of injury [Citation4,Citation32,Citation33] all increase with a concomitant decrease in neuromuscular function [Citation34] as a result of the increased load carriage demands. The context in which the load is carried also plays an important part in energy cost. For example, the speed at which a warfighter is moving while carrying a heavy load may increase the energy cost of the task (depending on duration and terrain gradients) [Citation29,Citation35,Citation36]. Moreover, warfighters with lower body mass might be more disadvantaged in carrying absolute loads due to increased cardiovascular strain and reduced neuromuscular function [Citation34]. Given these occupational-specific demands, warfighters may be subject to high energy and neuromuscular function costs when engaged in tactical operations.
Studies have reported a range of energy requirements, as low as 9.8 MJ/day (2,342 kcals) among female combat support personnel [Citation37] to as high as 29.8 MJ/day (7,122 kcals) for male Marines [Citation38]. Special Operations Forces (SOF) have been reported to have much higher daily average energy requirements in comparison to the average support soldier (4,099 ± 740 kcals/day vs. 3,361 ± 939 kcal/day, respectively) [Citation39]. As a result, it is likely that the daily energy expenditure of SOF likely exceeds the energy requirements as suggested by the military dietary reference intake (MDRI). Moreover, energy expenditures through specific training ranged from ~17,150 kJ/day (~4,100 kcals/day) [Citation40] during Army Ranger School to ~21,750 kJ/day (~5,200 kcals/day) [Citation41] during US Army Special Forces Assessment and Selection (SFAS) have been reported. Barringer et al. [Citation42] identified high daily energy demands of various SOF depending on the types of training conducted. For example, Marine Corps Forces Special Operations Command (MARSOC), the Ranger Assessment and Selection Program (RASP), and US Army Special Forces Small Unit Tactics Training groups had a predicted daily energy expenditure of 6,317 kcal/day, 4,264 kcal/day, and 5,215 kcal/day, respectively [Citation42]. Prediction of SOF energy requirements can aid dietetic personnel in planning an appropriate feeding regimen to meet operational demands and optimize warfighter health and performance [Citation42].
3.1.3. Duty-specific health and performance burdens
Much like the general population, warfighters are susceptible to various cardiometabolic diseases, such as heart disease [Citation43]. Similar to first responders, warfighters are exposed to various physiological, psychological, and extreme environmental stressors that can exacerbate the development and progression of cardiometabolic disease, among other adverse health issues (i.e., exertional heat illness) [Citation44]. Like greater segments of the general population, the prevalence of overweight and obese military personnel has increased [Citation45], which is associated with various chronic conditions, such as cardiovascular disease, type 2 diabetes, and other inflammatory conditions, as well as the occurrence of a mental health challenge (i.e., major depressive disorder and anxiety). Although the military has a fitness standard in place to determine employability and occupational specialty, the US Army reported 17.3% of warfighters as being obese, in addition to 52.9% being classified as overweight [Citation46]. This is problematic for multiple reasons, as warfighter readiness is likely compromised due to overweight and/or obesity classifications, and warfighters with obesity disproportionately use more healthcare services compared to those of normal weight [Citation47], with musculoskeletal issues among the most common diagnostic codes identified. A recent cross-sectional study regarding the fiscal year 2015 military claims and treatment facility data noted obese warfighters utilized approximately 30% of the recorded healthcare services for musculoskeletal issues compared to non-obese warfighters [Citation47]. Moreover, chronic sleep restriction can further exacerbate challenges associated with obesity among warfighters [Citation48]. For example, chronic sleep restriction is associated with decreases in leptin and increases in ghrelin [Citation49] as well as increased consumption of high-fat and high-carbohydrate foods [Citation49–51]. Chronic sleep restriction also increases insulin resistance and impairs glucose tolerance [Citation49,Citation52–54]. In this respect, poor long-term sleep practices can lead to an increased risk of cardiovascular disease [Citation55], musculoskeletal injuries [Citation56], substance abuse [Citation57], and post-traumatic stress disorder (PTSD) [Citation58].
In terms of load carriage, heavy loads (31–44 kg) [Citation59] routinely carried by warfighters consistently lead to injuries, most commonly to the lower back, which heavily impacts occupational performance, mission success, and readiness [Citation60,Citation61]. An association between fitness scores on the Army Physical Fitness Test (APFT) and lower back pain [Citation62] has been established – specifically, a score below 200 may be predictive of lower back pain. On the contrary, higher fitness levels are likely protective against lower back pain and other musculoskeletal injuries [Citation63]. While highly fit individuals are still susceptible to musculoskeletal injuries due to overuse, warfighters who are overweight or obese are at a greater risk of injury due to additional load (bodyweight) and physiological challenges [Citation64,Citation65]. Ultimately, strategies to prevent or reduce the risk of musculoskeletal injuries are important considerations for the warfighter, as injury will reduce occupational readiness and performance. Although there are physical fitness and body composition standards in place, military personnel are subjected to overuse and obesity-related issues that can reduce occupational readiness, physical performance, and overall health.
3.1.4. Key points: physiological and energy expenditure demands of military
The physiological demands military personnel face varies considerably depending on their intended mission; however, all warfighters should be prepared for high operational tempos and sustained training and combat conditions.
The energy requirements of Special Operation Forces are not well understood. Greater research is needed to best prepare appropriate fueling regimens to meet the operational demands and optimize warfighter performance based on operational tasks and the environment in which they are working.
Load carriage, chronic sleep loss, and environmental stressors impact warfighter health and performance and should be considered when developing fueling regimens to optimize mission success.
3.2. General energy and macronutrient guidelines for military personnel
Adequate nutrient intake can improve health as well as physical and occupational performance outcomes among warfighters [Citation66]. Given that warfighters are often engaged in high-volume physical training, MDRIs have been established and codified in Army Regulation 40-25 to provide guidance on feeding for military personnel [Citation67] and are based on moderate levels of activity suggested to be appropriate for personnel in garrison. In this regard, the recommended energy intake is 3,400 kcal/day for males and 2,300 kcal/day for females [Citation67]. Based on Army Regulation 40-25, protein intakes for military personnel are suggested to be between 0.8 and 1.6 g/kg/day for both males and females and should be increased during periods of intense physical exertion and increased metabolic demand [Citation67]. The reader is encouraged to review the ISSN position stands regarding protein intake for those engaged in intense training, as the recommendations are between 1.4 and 2.0 g/kg/day for exercising individuals [Citation68,Citation69]. The warfighter is likely to benefit from the ISSN’s recommended range, especially those who are engaged in regular exercise training. The current recommendations for carbohydrates and fats are based on well-established sports nutrition guidelines. In terms of carbohydrates, during prolonged periods of intense physical demand, it is recommended that warfighters consume between 4 and 8 g/kg/day [Citation67]. Assuming adequate energy intake, warfighters who consume 50 to 55% of their total calories as carbohydrates will meet the recommended carbohydrate intake. Fat intake should be approximately 30% or less of total energy intake. Additionally, military personnel are advised to include the consumption of linoleic acid (17 g/day and 12 g/day for males and females, respectively) and alpha-linolenic acid (ALA: 1.6 g/day and 1.1 g/day for males and females, respectively) within their daily fat intake [Citation67]. The MDRIs for energy intake and macronutrients are based upon height and weight measurements, representative of the 50th percentile for military males and females and must be adjusted for body size, physical activity, environmental factors, clothing, equipment, and terrain, to be applicable to different Service members’ needs.
3.2.1. Key points: general energy and macronutrient guidelines for military personnel
The provision and timing of adequate amounts of energy, macronutrients, and micronutrients best promote optimal occupational performance outcomes and reduce injury and disease risk.
Army Regulation 40-25 provides guidance on feeding for the warfighter.
3.3. Special nutritional considerations for military personnel
3.3.1. Combating protein loss during energy deficit
Warfighters endure a combination of unique physiological, psychological, and environmental stressors during any period of sustained training and combat operations, which routinely result in a negative energy balance. As the magnitude of energy deficit increases, so does the rate of catabolism of whole-body and skeletal muscle protein [Citation70]. Consuming adequate energy (via combat rations) to match energy expenditures and recommended protein intake [Citation71,Citation72] during ongoing training or combat would largely negate this protein catabolism. However, warfighters rarely achieve energy balance and optimal protein recommendations when subsisting on combat rations. During sustained training or combat, the operational tempo dictates what the warfighter normally eats and what is carried on their person. Energy deficits from increased energy demand can largely be attenuated with the provision and consumption of high calorie rations. While this dietary strategy is effective, it is an oversimplification of the well-recognized logistical and physiological barriers imposed upon the warfighter. These barriers include operational time constraints, appetite suppression, the inability to carry more food, or prioritizing operational items over rations. Thus, warfighters rarely achieve energy balance and optimal protein recommendations when subsisting on combat rations alone. This caloric deficit can lead to substantive losses in whole-body and skeletal muscle protein (i.e., negative protein balance) and, thus, profound performance decrements, especially with frequent and repeated exposures to energy deficits.
Depending on the length of the training or combat period, the extent of energy deficit can routinely achieve 40–70%. Negative protein balance has been largely unavoidable during these times and is further exacerbated by sub-optimal dietary protein intake (both quantity and quality) and the effects of limited energy intake [Citation73,Citation74]. Prolonged negative protein balance and concomitant muscle loss may compromise physical performance and increase injury risk and lost duty time, which further diminishes warfighter readiness [Citation12,Citation74]. While additional protein intake is effective in mitigating compromises in body protein homeostasis, especially when combined with resistance exercise [Citation75], as the caloric deficit increases (~40% [Citation73,Citation75,Citation76]) the anabolic effects of protein are absent, and the constituent essential amino acids (EAA) are prioritized as carbon skeletons for energy production.
Skeletal muscle sensitivity to anabolic capability is diminished in favor of whole-body protein homeostasis during caloric deficit [Citation77,Citation78]. For example, diet-induced energy deficits of 30% revealed that an EAA dose of 0.3 g/kg maintained whole-body protein balance and stimulated muscle protein synthesis, while a lower dose of 0.1 g/kg was only able to maintain protein balance [Citation78]. A later study delivered 24 g of EAA after military-related tasks/exercises via three isonitrogenous formats (EAA-enriched whey protein formula, whey protein alone, or a mixed nutrient meal) and showed that muscle protein synthesis was not different between the groups; however, the EAA-enriched whey protein formula produced a significantly greater whole-body protein balance, followed by whey protein alone, and then the mixed nutrient meal [Citation77]. Evidence encompassing a wide range of protein/EAA intake formats clearly indicates that the more easily digested formats can rapidly deliver EAA to the periphery for optimal stimulation of protein synthesis [Citation79], while pragmatically this format may be better suited for a warfighter due to it being largely indestructible and resistant to spoilage, takes up little volume, and weighs very little. Taken together, these data strongly suggest that a supplemental format would be beneficial for the warfighter in the preservation of body protein homeostasis during extended periods of training, combat, and energy deficit.
3.3.2. Key points: special considerations for military personnel
Requirements of EAA increase with caloric deficit, such that supplementation should be considered.
A greater peripheral delivery of EAA results in greater stimulation of both whole-body and muscle protein synthesis.
Simpler EAA ingestion formats (i.e., free-form and protein alone) may provide for greater peripheral delivery of EAA and protein kinetic responses while also doubling as a more pragmatic consideration.
3.4. Hydration
3.4.1. Considerations for hydration among military
Mission hydration recommendations from the Academy of Nutrition and Dietetics and the American College of Sports Medicine [Citation80] suggest that the warfighters (1) consume 5 to 7 mL (~0.25 cups) per kilogram of body mass of water or sports drink 4 h prior to a mission, (2) limit dehydration (to <2% loss in body weight) during missions by consuming adequate amounts of fluids, and (3) consume 450 to 675 mL (2–3 cups) per 0.45 kg (1 lb) of body weight lost upon completion of the mission. Environmental demands may place additional fluid and electrolyte needs upon the warfighter and can compromise fluid homeostasis. Adequate fluid intake is critical in hot and humid environments to avoid heat-related injuries and early fatigue, which can hinder occupational performance while also posing a risk to overall health. Moreover, cold environments might reduce thirst [Citation81]; thus, fluid intake may be reduced. This is even more problematic in high-altitude environments because hypoxia-induced increases in ventilation and diuresis can exacerbate fluid imbalance and likely are coupled with poor thirst sensation. The three-step approach to hydration, outlined by Cheuvront and Sawka [Citation82] (see the Fist Responder Hydration section: Part 2), may be a helpful aid to the warfighter in minimizing the risk of dehydration.
3.5. Nutritional supplementation in warfighters
As highlighted throughout, daily training and operations of warfighters create physical and nutritional challenges that can compromise health, performance, and recovery. For these reasons, efficacious regimens of nutritional supplementation are commonly considered. As outlined in
, several nutritional supplements are commonly used by tactical athletes. In terms of promoting general health, the warfighter should consider ingesting a daily low-dose multivitamin (see ) to ensure sufficient levels of vitamins and minerals are met in the diet [Citation83]. While nutritional supplements are not intended to replace the role of whole foods within the tactical athlete’s diet, supplementation should be used as means to augment dietary intake with the purpose of achieving a health or performance benefit. Although most lack robust scientific support, beta-alanine, caffeine, and creatine monohydrate all have demonstrated merit in terms of safety, ergogenic potential, enhancing mental readiness, and neuroprotection.
Table 1. Commonly Used Supplements Consumed by Tactical Athletes.
3.5.1. Beta-alanine
Beta-alanine is the rate-limiting precursor to carnosine, a powerful physiological buffer that is also linked to focus, alertness, and cognitive function during a period of stress and fatigue. The majority of research involving beta-alanine has focused on its ergogenic potential [Citation87]. Briefly and in this respect, review papers that have summarized the literature for beta-alanine generally support its efficacy as an ergogenic aid in brief, high-intensity activities that span durations of 60–300 s [Citation87–90]. Beyond these conclusions, one study has assessed the impact of beta-alanine supplementation (6 g/day for 4 weeks) on both physical and cognitive performance among military personnel [Citation91]. Following a battery of physically demanding tasks, 20 warfighters were subjected to shooting tasks and cognitive assessments, whereby shooting accuracy, marksmanship, and target engagement speed improved following the 4-week supplementation period, with no observed changes in cognitive function [Citation91]. Another study by Hoffman and colleagues [Citation92] demonstrated that 30 days of beta-alanine supplementation significantly increased muscle carnosine content as well as improved military-specific performance assessed via a 50-m casualty carry. Additionally, significant improvements were noted for cognitive performance assessed via a 2-min serial subtraction test [Citation92], which was performed at a shooting range while continuous fire was directed at targets nearby. Moreover, this finding highlights the ability to maintain focus during stressful, anxiety-inducing conditions (i.e., close-by gunfire) following the 30 day of beta-alanine supplementation. Future research should continue to examine beta-alanine’s potential ability to improve shooting performance and decision-making in a warfighter population.
3.5.2. Caffeine
Considering its consumption in coffee, tea, cocoa, soft drinks, and other foods and beverages, caffeine is arguably the most popular nutritional supplement in the world, and this popularity is also present among military personnel [Citation93–95]. Caffeine is a central nervous system stimulant that has the potential to enhance exercise performance and reduce fatigue [Citation96]. Key outcomes of this substance have been critically detailed in the International Society of Sports Nutrition (ISSN) position stand on caffeine [Citation97]. Military personnel have reported an average daily consumption of 212–285 mg/day of caffeine [Citation95]. The Army Regulation 40-25 recommends 100 to 200 mg (100 mg per 2 h or 200 mg per 4 h) of caffeine for operational utility and during periods of sleep deprivation [Citation67]. Operational ration components (i.e., caffeinated gum) contain 100 to 200 mg per serving and are intended to help promote optimal cognitive and physical performance among warfighters [Citation67]. In military populations, caffeine has been reported to improve time to exhaustion during military-specific tasks [Citation98] following sleep deprivation. Specifically, Kamimori et al. [Citation99] demonstrated that a total daily caffeine dose of 800 mg may aid in effectively overcoming adverse effects from successive periods of extended wakefulness when optimal sleep periods are not practical.
Beyond the physical benefits, it is well documented that caffeine enhances cognitive performance and mood when appropriate doses are used [Citation100–103]. Moreover, evidence continues to accumulate that supports caffeine’s ability to aid the warfighter during or after periods of sleep deprivation [Citation99,Citation104–106], particularly in terms of improving attention and vigilance, reaction time, and ability to perform complex activities, problem-solving, and reasoning [Citation105]. Several studies have illustrated caffeine’s ability to improve marksmanship following sleep deprivation [Citation106–108]. For example, Lieberman et al. [Citation108] demonstrated that 200 to 300 mg caffeine doses attenuated the decrements in correct hits on a four-choice visual reaction time assessment (73% and 81%, respectively) and premature errors (98% and 88%, respectively) compared to placebo [Citation108] during Navy SEAL candidates’ Hell Week training. This study also demonstrated that a 200 mg dose of caffeine improved traditional indicators of marksmanship: reduced distance from center of mass (23%), reduced misses (44%), increased shot group tightness (47%), and increased sighting time (23%) compared to placebo [Citation108]. Improved sighting time was also demonstrated by Tharion et al. [Citation106], which is a particularly relevant outcome that for this population can make the difference between life or death or correctly identifying friend versus foe.
Any individual (military operator or not) must be cognizant of the increased association of elevations in blood pressure, heart rate, heart palpitations, and general feelings of anxiousness or jitteriness that occur in some individuals after caffeine is consumed [Citation97,Citation109,Citation110]. In addition, caffeine’s ability to promote wakefulness can contradict the human body and mind’s need for quality periods of rest and sleep [Citation111,Citation112]. This is not problematic when ingestion occurs in the morning or afternoon but can have very negative implications on both the amount and quality of sleep that an individual can expect to achieve if caffeine is consumed in the late afternoon or in the evening. Thus, careful consideration should be made by individuals who consume any dosage of caffeine within a few hours (e.g., ≤6 h) of needing to fall asleep, particularly if the dose exceeds 200 mg and/or the individual is known to exhibit sharp sensitivity to caffeine’s presence. Finally, in terms of energy drinks, data indicate that one in six warfighters reported high use (i.e., 24 oz or more/day of energy drinks consumption) and significant associations to mental health issues, aggressive behaviors, and fatigue [Citation113]. In fact, reports suggest that military personnel consume energy drinks at a greater frequency than the civilian population [Citation114]. Further research is warranted to assess relationships between energy drink use and these health-related variables in a longitudinal sense. Moderation is encouraged for those consuming energy drinks, and further emphasis should be given to the aforementioned considerations regarding caffeine doses [Citation69,Citation115].
3.5.3. Creatine monohydrate
Recent reviews, including the ISSN Position Stand on creatine monohydrate, have outlined the ergogenic potential of creatine supplementation [Citation116,Citation117]. These reviews highlight creatine’s documented potential to increase high-intensity exercise capacity, peak power, maximal strength, repetitions completed before fatigue, and fat-free mass while undergoing physical training. While research, to date, is limited in military populations, the ergogenic outcomes observed from the use of creatine monohydrate in athletic populations are relevant to warfighters. First, Cooke et al. [Citation118] reported that creatine supplementation might help to improve recovery and mitigate damage to muscle tissue after challenging stressful bouts of exercise. Additionally, prolonged supplementation with creatine monohydrate in competitive collegiate-level athletes has demonstrated a lower incidence of muscle-related injuries/issues, such as cramping, strains/pulls, heat illness, and total injuries [Citation119,Citation120]. Creatine monohydrate supplementation has also been shown to increase intracellular water and reduce heart rate, rectal temperature, and sweat rate during prolonged exercise in the heat [Citation121]. This enhanced tolerance to performance in the heat is an important benefit to the warfighter when operating in hot environmental conditions. Recent findings also indicate that creatine monohydrate supplementation confers benefits to cognitive processes during periods of neural vulnerability, which occurs amidst stress and/or sleep deprivation [Citation122] conditions clearly documented to occur for many military populations [Citation123,Citation124]. Lastly, some emerging evidence has demonstrated the ability of creatine supplementation to increase brain creatine levels by about 5–10% [Citation122,Citation125].
This explains some of the published outcomes supporting creatine’s ability to provide neuroprotection from traumatic brain injuries and even potential function in a prophylactic manner in terms of symptom reduction [Citation122,Citation126,Citation127]. Moreover, other evidence exists to support the potential for creatine to support brain health and other associated outcomes of interest to the warfighter [Citation124,Citation128,Citation129]. Most studies show that higher daily doses of creatine over a longer duration (i.e., 10–20 g/day for 2 or more weeks) may be needed to increase the brain creatine content [Citation125,Citation130]. Long-term high-dose creatine monohydrate supplementation (e.g., 20–25 g/day for 12 weeks and 5–10 g/day for up to 21 months) has been studied in athletic populations and have been shown to be safe and lower the incidence and severity of an injury [Citation119,Citation120,Citation131,Citation132]. Additionally, clinical populations have been studied with doses 10–30 g/day for up to 5 years [Citation69,Citation116,Citation133]. The interested reader is highly encouraged to read the following reviews to further understand the potential health and performance implications of creatine monohydrate supplementation, including data that summarizes any potential safety concerns for its use [Citation116,Citation117,Citation127,Citation134].
3.5.4. Key Points: Ergogenic Supplementation in Warfighters
During sustained training and combat operations, warfighters may supplement with 200 to 300 mg/day of caffeine to enhance or combat decrements in military-specific performance.
Available evidence suggests total daily caffeine amounts of 800 mg (200 mg doses administered with 2–3-h intervals between doses over a 24-h period) may help attenuate the negative effects of successive and chronic sleep loss (i.e., during sustained training and combat operations).
Creatine monohydrate supplementation of 0.3 g/kg/day for 5–7 days and ~5 g/day (or 0.1 g/kg/day) thereafter is an ergogenic aid that can enhance occupational, physical, and cognitive performance, with growing evidence that it may function in a neuroprotective fashion (with daily doses of 10–20 g/day needed).
3.6. Preserving Whole-Body Muscle and Protein Status in Warfighters
3.6.1. Essential Amino Acids (EAA)
Seminal work performed on muscle protein synthesis has highlighted the critical role that delivering an efficacious dose of EAA has on maximally stimulating muscle protein synthesis [Citation135], while other non-protein factors also impact key components of nutrient and assimilation of skeletal muscle and other tissues [Citation79,Citation136]. Many studies have confirmed the benefits of EAA intake in various settings regarding skeletal muscle anabolism [Citation137,Citation138], lean mass [Citation139,Citation140], and functional outcomes [Citation139,Citation140] (particularly in older populations), as well as rehabilitative mitigation of muscle loss after surgery [Citation141,Citation142]. Further, it has long been demonstrated that free-form EAA supplementation in conjunction with exercise confers a significant anabolic effect on skeletal muscle [Citation143] and other factors such as when the dose is ingested may also augment observed muscle protein synthesis rates [Citation144–147]. To date, over 70 clinical trials have been conducted, indicating the benefits of EAA ingestion and/or supplementation. Further, increasing EAA ingestion has recently been demonstrated efficacious in Warfighters during periods of caloric deficit for the maintenance of body protein (see below).
Pragmatic and ergogenic reasons are evident for suggesting the inclusion of free-form EAA into the warfighter ration complement. Pragmatically, commercially available free-form EAA products are now available that are water-soluble, highly palatable, and packaged into lightweight and compact delivery formats. These formulas are suited to the warfighters who must carry their operational requirements on their person while carefully considering weight and space requirements. From an anabolic standpoint, the consolidation of research indicates that doses as small as 3–6 g are efficacious, especially when combined with exercise [Citation148]. A maximal response with free-form EAA ingestion is derived between 10 and 15 g [Citation135,Citation149]. This finding is consistent with studies utilizing protein ingestion and the prevalent result that 25–30 g of protein, when combined with exercise, provides a maximal stimulation of muscle protein synthesis [Citation150]. However, it is important to note that ingestion of free-form EAA results in greater stimulation of synthesis than an equivalent amount in high-quality protein [Citation79].
For these reasons, warfighters are advised to consume a daily diet that delivers optimal amounts of EAA at each meal and across the entire day, irrespective of whether this occurs via intact protein sources in the form of food, isolated protein powders, or free-form amino acids. An efficacious dose of EAA is considered between 10 and 12 g [Citation68]. Importantly, many sources of protein exist that can deliver the required amino acids [Citation151], but an ever-present challenge for warfighters is their ability to carry large amounts of provisions and oftentimes limited access to refrigeration, while also dealing with spontaneous, chaotic changes in schedule. For these reasons and others, utilizing free-form EAA might also be a suitable alternative. Toward this aim, Church et al. [Citation79] recently highlighted that peak EAA concentrations and EAA area under the curve are the strongest predictors of changes in the fractional synthesis rates (FSR) of muscle proteins, postprandial FSR, and whole-body protein synthesis. As such, any combination of dose, timing, source, and form in conjunction with pragmatic considerations for the warfighter that maximizes these outcomes should be most strongly considered.
For the warfighter, the EAA requirement to stimulate protein anabolism is altered in the presence of caloric deficits that often occur during periods of sustained training or combat. Largely due to the metabolic stress of exercise/high workloads and energy deficit, muscle anabolic potential is mitigated in favor of the maintenance of whole-body protein homeostasis. For example, Gwin et al. [Citation78] demonstrated in young subjects (23 ± 5 years) that 5 days of 30% energy deficit increased the body’s need for EAA to maintain body protein balance. Under identical conditions, two EAA doses (0.10 g/kg, ~8 g/dose or 0.30 g/kg) were provided immediately after single-leg exercise. While whole-body protein homeostasis was achieved with both doses, the higher dose achieved greater rates of whole-body protein synthesis, which ultimately were two-fold greater when compared to the lower dose [Citation78]. Follow-up work by the same research group again instituted a 30% energy deficit over 5 days [Citation77], and this time provided a 35-g protein dose in three delivery formats: a free-form EAA + whey protein formula, whey protein alone, or a mixed macronutrient meal. A key point of this study was that muscle and whole-body protein metabolism were assessed before and after approximately 50 min of military task performance, and the authors reported that whole-body protein synthesis and net protein balance were greater with the EAA/whey combination [Citation77]. These results demonstrate that combining optimal EAA amounts with other nutrients may further heighten the observed responses in terms of muscle protein synthesis rates and whole-body protein metabolism [Citation152]. In addition to these outcomes, other studies have provided support in both civilian and military contexts that adding protein to the diet (which will optimize EAA delivery) in the face of energy deficits of 30–40% can mitigate lean tissue loss and optimize loss of fat tissue [Citation153,Citation154].
Taken together, the data indicate that efficient delivery of EAA may be beneficial to the warfighter, especially when considering the inherent operational stressors of caloric deficit, performance requirements, and the limited ability to carry nutritional intake. Adequate EAA, whether it is delivered in free-form or intact protein sources such as isolated protein powders or foods, will protect whole-body protein homeostasis during challenging physiological requirements. Thus, consideration by the warfighter should be given to effective and efficient delivery of EAA to preserve whole-body muscle and protein status.
3.6.2. Key points: preserving whole-body muscle and protein status in warfighter
A larger peripheral delivery of EAA results in greater stimulation of both whole-body and muscle protein synthesis.
EAA ingestion formats (i.e., free-form or intact isolated proteins) provide for greater peripheral delivery of EAA and protein kinetic responses.
Optimal delivery of EAA in the warfighter’s diet, particularly when faced with a prolonged energy deficit, can help mitigate the loss of whole-body and muscle protein and sustain higher rates of protein metabolism.
3.7. Additional nutritional supplement considerations
Without question, adequate nutrition and hydration are paramount when discussing nutritional considerations for tactical and service professionals. An additional area that continues to capture more interest is that of cognitive health and performance, especially as it relates to occupational performance. As outlined previously for various physical conditions, acute sleep deprivation, chronic sleep restriction, extreme environmental conditions, inadequate nutrition, and high rates of physical exertion also challenge cognitive demands and function. Beyond these challenges, the incidences of suicide [Citation155,Citation156] and traumatic brain injuries (TBI) [Citation157] in military populations are two additional areas where enhancing nutrient delivery may afford positive outcomes. In this respect, interest has grown toward understanding and optimizing the potential benefits of various forms of nutritional supplementation in reference to cognitive function. While much more research is needed, two ingredients will briefly be discussed: omega-3 fatty acids and L-tyrosine for their potential to positively impact cognitive performance and health.
3.7.1. Omega-3 fatty acids
Omega-3 (n-3) fatty acids are polyunsaturated fatty acids obtained through the diet or in supplemental forms and typically contain the fatty acids eicosapentaenoic acid (EPA) and docosahexaenoic acid (DHA). Researchers have referred to n-3 polyunsaturated fatty acid intake as a potential ‘nutritional armor’ for cognitive health and performance [Citation158,Citation159]. Briefly, eicosapentaenoic acid (EPA; 20:5 n-3) and docosahexaenoic acid (DHA; 22:6 n −3) are long-chain n-3 fatty acids endogenously synthesized from ALA. Since EPA and DHA are downstream metabolic products of ALA, they are not considered essential fatty acids. However, the conversion of ALA to EPA or DHA is inefficient and somewhat limited in humans [Citation160]. The physiological adaptations associated with n-3 fatty acids are primarily mediated by the incorporation of EPA and DHA into tissue membrane phospholipids (e.g., skeletal, cardiac, or neural tissue). Thus, obtaining EPA and DHA from the consumption of oily fish or dietary supplements (e.g., fish oil or algal oil) is the most effective strategy to improve membrane and circulating n-3 fatty acid status.
In the brain, DHA is one of the primary structural components of the neuronal cell membrane and accounts for 10% and 97% of the total lipid and n-3 fatty acid content, respectively [Citation161,Citation162]. As such, preclinical models have shown that TBI reduces DHA brain content, and prophylactic DHA supplementation consistently enhances resilience to TBI, including multiple mild TBIs. Since military personnel are routinely exposed to combat and training environments that increase susceptibility to head trauma, severe and mild TBIs and the associated negative sequalae continue to be a concern for the military. While minimal human data exists, recent studies in athletes exposed to repetitive head impacts may offer some insight into the nutritional factors available to reduce the prevalence and severity of TBIs. As such, DHA supplementation (≥2 g∙d−1) has been shown to reduce a reliable marker of head trauma, neurofilament light chain (Nf-L), in American football players subjected to repetitive head impacts [Citation163]. Since there are notable divergent and even competitive mechanisms of DHA and EPA, a follow-up study was conducted to determine the effect of a more standard n-3 fatty acid supplement. Heileson et al. [Citation164] reported similar reductions in Nf-L and, thus head trauma, over the course of an American college football season with a supplement containing multiple n-3 fatty acids (2 g DHA, 0.56 g EPA, and 0.32 g DPA). In athletes, a population not unlike the Military personnel, n-3 fatty acid supplementation has also been implicated in other cognitive outcomes such as improved mood, sleep quality, episodic memory, and reaction time efficiency [Citation165–168]. Further, evidence exists that deficiencies in n-3s, particularly DHA, may elevate deployment-induced stress that can manifest as depression and suicide [Citation169]. Warfighters with low DHA levels are 62% more likely to have completed suicide [Citation169]. Hallahan et al. [Citation170] reported a 45% reduction in suicidal ideation and a 30% reduction in depression for individuals who consumed 2 g·d−1 of EPA+DHA for at least 12 weeks, and these data are thoroughly summarized in a review by Hibbeln and Gow [Citation171–174]. Finally, these recommendations were also firmly supported by a military expert panel who concluded that it would be unethical not to attempt to increase the n-3 status among U.S. military personnel [Citation175].
One of the most consistent benefits associated with n-3 fatty acid supplementation is recovery from rigorous training or exercise-induced muscle damage [Citation173,Citation176]. While the majority of studies used laboratory-controlled models of muscle damage, Black et al. [Citation165] studied the effects of n-3 fatty acid supplementation during pre-season in male rugby players. Omega-3 supplementation, as a part of a protein-based drink, attenuated lower-body muscle soreness and fatigue and improved neuromuscular performance. Since recent studies have continued to report similar results such as blunted vertical jump height decrements [Citation177], reduced delayed-onset muscle soreness [Citation177,Citation178], and greater range of motion [Citation179], it is clear that n-3 fatty acids may positively influence muscle recovery following rigorous training environments.
Since Warfighters endure a high degree of physiological and psychological stress and exposure to high-risk environments with heightened susceptibility to TBIs and post TBI-associated sequelae (e.g. depression, anxiety), prophylactic n-3 fatty acid supplementation appears to be a pragmatic strategy to potentially enhance cognition protection and performance and muscular recovery. Similarly, a recent expert military panel unanimously concluded that it would be unethical to not attempt to increase the n-3 status among U.S. military personnel [Citation175]. While the evidence for long-chain n-3 supplementation in military populations is limited (which should be a high priority area of research), there is a strong rationale for supplementation in military personnel, especially those that consume less than 2–3 servings of fatty fish per week [Citation67].
3.7.2. L-tyrosine
Tyrosine is a proteogenic amino acid that is the precursor for the synthesis of the catecholamine dopamine (DA), norepinephrine (NE), and epinephrine (E). Since tyrosine is synthesized from phenylalanine, it is not considered an essential amino acid. Importantly, however, there is evidence that the brain does not convert enough tyrosine from phenylalanine to meet its demands. This is especially true in brain-vulnerable states that arise with stress and sleep loss. In these vulnerable states, neural catecholamine activity increases and becomes more precursor sensitive [Citation180]. Accordingly, in situations where there is increased environmental and psychological stressors or ongoing sleep loss, warfighters might find that L-tyrosine supplementation can aid with optimal cognitive functioning. Indeed, several studies have reported that various doses of L-tyrosine supplementation preserve cognitive processing under stressful conditions. For example, Mahoney et al. [Citation181] reported that 150 mg/kg L-tyrosine supplementation prior to two 90-min cold-water immersions demonstrated improved speed and accuracy of information processing, while Shurtleff et al. indicated that a similar dosage of L-tyrosine improved matching accuracy following 30-min cold exposure [Citation182]. Also, Coull et al. [Citation148] demonstrated improved vigilance (9% increase) following 300 mg/kg body mass of L-tyrosine supplementation among soccer players exercising in a hot environment.
The cognitive processes affected by L-tyrosine supplementation are largely carried out through pre-frontal cortex (PFC) activity. PFC DA activity is particularly critical for cognitive processes such as working memory, decision making, task switching, attention, and general executive control functions [Citation183]. In addition, relative to other brain areas, the PFC is more sensitive to catecholamine activity due to the PFC-unique density and activity of DA transporters (DATs) and NE transporters (NETs) [Citation184]. It is important to note that PFC DA activity does not linearly improve cognitive processing. There is an inverted U-shaped relationship between DA activity and PFC-dependent cognitive processing such that too little or too much dopamine impairs performance [Citation185]. An additional consideration in L-tyrosine supplementation for cognitive processing is that tyrosine is transported across the blood–brain barrier via a general transport mechanism for large neutral amino acids (LNAA) and thus competes with other LNAA, such as tryptophan [Citation180]. Currently, more research that specifically seeks to identify if L-tyrosine supplantation can improve or preserve cognitive processing in warfighter environments is needed, particularly as previous research has indicated that prolonged exposure to physically demanding tasks and/or extreme conditions (sleep loss, heat, cold, altitude, etc.) can alter catecholamine production [Citation186].
3.7.3. Key points: additional nutritional supplement considerations
Warfighters engaged in sustained training and combat likely experience decrements in cognitive performance and brain health.
Limited but promising initial research has been completed in warfighter populations for omega-3 fatty acids and L-tyrosine. Each of these supplements currently has limited literature bases and well-controlled high-quality research investigations are strongly advised to better understand any potential benefit they may afford to warfighter’s health and readiness.
Well-controlled investigations are needed to better understand the potential impact and translatability of the current research regarding warfighter cognition health and performance as well as mental health.
4. Part 2 – First responders
4.1. Demands of law enforcement
4.1.1. Job-specific tasks and common stressors
The physiological demands of law enforcement personnel may vary considerably, ranging from those that are relatively mundane to those that are immediately life-threatening. For instance, calls for response tend to occur sporadically, which may require a law enforcement officer to quickly shift from a sedentary state to one that requires maximal physical exertion (i.e. from seated in their car or at the station to sprinting, jumping, defensive tactics, and arrest control) [Citation2,Citation187]. In this respect, law enforcement officers are required to pursue and apprehend suspects, enter unfamiliar scenarios (at times forcefully), engage in hand-to-hand self-defense, lift and carry heavy objects, and maneuver quickly on foot [Citation188]. The energy demands of these activities are further exacerbated by the burden of PPE (10–40 kg) [Citation5,Citation189,Citation190], which creates considerable biomechanical (i.e. postural issues, ranges of motion, and gait kinematics) and physiological constraints, subsequently also increasing the risk for musculoskeletal injury [Citation190–193]. These challenges, when compounded over an entire career, are viewed to be primary contributors to the documented increases in injury, cardiometabolic disease, and premature death [Citation194] in law enforcement populations.
4.1.2. Physical fitness considerations and energy expenditure
During academy training, recruits are exposed to occupation-specific procedures, skills, and expected behaviors and values of a law enforcement officer [Citation195] as well as regular physical and occupational-specific training to aid in the early development of their law enforcement career. Due to the prescriptive nature of this training period, nutritional needs, education, and shortcomings may be easier to assess. As a result, practitioners should take into consideration their physical training schedules for not only optimal physical performance but also to better prepare the recruit for real-life, on-the-job feeding scenarios. Toward this end, an emphasis on healthy eating patterns and behaviors will help promote good cardiovascular and metabolic health over an entire career. Currently, no universally accepted strength, flexibility, or aerobic capacity fitness standards exist for law enforcement, but some feel it is likely similar to the established standard for a firefighter (i.e., ~42 ml·kg−1·min−1), given the similarities in anticipated job task while dressed in full PPE and exposed to environmental stressors [Citation196]. Further, PPE increases the metabolic cost (v O2; L/min) of various occupational tasks by ~6–21% depending on the weight, mode, and intensity of the work among law enforcement officers [Citation197,Citation198]. Specialist law enforcement personnel (such as those assigned to Special Weapons and Tactics (SWAT) officers) likely have higher aerobic fitness demands and energy expenditure due to more complex tasks and absolute load carriage (~40 kg vs. ~10 kg loads carried by nonspecialist forces, respectively) [Citation199]. However, the specific energy needs when performing these essential job tasks is unknown, and future investigations should explore the caloric costs of performing job tasks while wearing PPE within general duty and specialist teams. Based on the daily variability in energy expenditure, the energy needs of recruits, officers, and special team members must be analyzed separately and considered when making nutritional recommendations across the occupational life span.
4.1.3. Job-specific health and performance burdens
Previous research indicates that law enforcement officers are twice as likely to suffer from cardiovascular disease (CVD) compared to the general population [Citation200]. Varvarigou et al. noted that stressful occupation-specific duties were associated with a greater risk of sudden cardiac death while on duty; however, most sudden cardiac deaths (~77%) occurred during non-routine tasks, which can all be characterized by sudden increases in cardiovascular demand from a combination of extreme physical exertion and psychological stress [Citation201]. Similar to firefighters [Citation202], extended sedentary periods followed by acute heavy physical exertion are occupational factors that increase the risk for sudden cardiac death and CVD in this population [Citation201], particularly when coupled with low fitness levels [Citation203]. Like the general population, the combination of sedentary physical activity patterns and poor dietary habits increases the risk of a law enforcement officer developing obesity [Citation204]. Obesity rates are significantly associated with reduced overall fitness among police officers [Citation205,Citation206], while Williford and Scharf-Olson [Citation207] noted that higher body fat % (BF%) was linked to poorly simulated job-specific performance. Other work has demonstrated that law enforcement personnel with higher BF% have lower aerobic capacity and reduced capacity to carry external loads compared to their leaner counterparts [Citation208]. Moreover, Dawes et al. [Citation209] found negative correlations between the sum of skinfolds and muscular endurance in SWAT officers, with other studies consistently showing that performance is negatively impacted by a higher BF% in addition to being at greater risk for cardiometabolic disease [Citation210,Citation211]. While discordance exists in the literature [Citation212,Citation213], it is likely that leaner law enforcement officers will perform better compared to those with excess fat mass. Thus, it is important to consider interventions (i.e., dietary and training) to 1) prevent accrual of body fat, 2) attenuate the potential negative performance effects of higher BF%, and 3) improve the overall health and fitness profile of the law enforcement officer.
4.2. Demands of Firefighting
4.2.1. Job-specific tasks and common stressors
The job demands of firefighters can be physically arduous and environment-dependent. Structural firefighters routinely carry heavy equipment, advance unwieldy and heavy charged hose lines, climb stairs with equipment, drag/carry victims, and perform forcible entry, salvage, overhaul, and search and rescue [Citation214], while wildland firefighters routinely build fire lines, operate a chainsaw, and hike with external loads over wildland terrain in adverse conditions [Citation215]. Additionally, fire-resistant PPE must be worn, which adds an additional load (~26 kg for structural and ~6–20 kg for wildland firefighters) and may adversely impact both physical and occupational performance while increasing metabolic demands and cardiovascular strain [Citation216–221]. In fact, decrements of 1.5 ± 0.43% per kg of PPE in physical ability have been noted among these personnel [Citation221,Citation222]. Moreover, firefighting is sporadic and unpredictable and requires performance in extreme environmental conditions [Citation215,Citation223]. Previous work has indicated that structural firefighting routinely yields heart rate (HR) values between 84% and 100% HRmax, oxygen consumption values of 60% to 97% in response to various fire suppression or occupational-specific tasks [Citation224–227], and peak blood lactate levels ranging from 6 to 13 mmol/L during simulated fire ground tasks [Citation224,Citation228–230]. Similarly, wildland firefighters achieve HR values from 62% to 71% HRmax [Citation215], with oxygen uptake levels being reported to be around 50% peak oxygen uptake (~22.2 ml·kg−1·min−1) [Citation231] and as high as 34 ml·kg−1·min−1 during hikes [Citation232].
It is well established that firefighters experience significant cardiac events at a rate higher than the general population [Citation202,Citation233,Citation234]. Adverse cardiovascular events among firefighters most commonly occur when responding to an active structural fire [Citation235]. It has been reported that the risk of death is 10- to 100-times greater following active fire suppression compared to non-emergency response calls [Citation235]. The reasons for these events are certainly multifactorial, with acute and systemic changes in catecholamines, cortisol, and cytokines, such as interleukin-6 (IL-6), tumor necrosis factor-alpha (TNF-α), and interleukin-1β [Citation236,Citation237], which are known primary factors contributing to cardiovascular dysfunction [Citation238]. Another key contributor is heat exposure during fire suppression training, which directly leads to fluid loss and increases in core temperature, collectively placing additional strain on the cardiovascular system [Citation236,Citation237,Citation239]. Furthermore, Hunter et al. [Citation239] reported impairments in vascular function, a 73% increase in thrombus production, and a 7% increase in platelet-monocyte binding 2 h after completion of fire suppression activities. Additionally, several other outcomes are considered to be indicative of increased cardiac stress (e.g. increases in fibrinolytic capacity, asymptomatic myocardial ischemia, and cardiac troponin I, decreases in left ventricle contractility, and stroke volume) have been shown to occur in response to varying firefighting environments [Citation240,Citation241]. Finally, one cannot overlook the contribution of hypokinesis, obesity, and extreme environmental conditions toward CVD among firefighters [Citation242].
4.2.2. Physical fitness considerations and energy expenditure
The current minimum standard for aerobic capacity to perform structural fire rescue tasks is 42 ml·kg−1·min−1 (with a range of 39–45 ml·kg−1·min−1) [Citation227,Citation230,Citation243], and possessing this level of aerobic fitness increases the efficiency of occupational task completion and results in less fatigue during these events [Citation230,Citation243]. Alternatively, aerobic demands during wildland firefighting tasks can range from 10 to 34 ml·kg−1·min−1 when performing tasks such as hiking, fire-line construction, brush removal, and chain sawing [Citation231,Citation232,Citation244]. Additionally, several studies have reported relationships between muscular strength, power, and anaerobic endurance with a firefighter’s ability to perform essential occupational tasks such as a victim drag, equipment hoist, ladder carry, stair climbing, and hose deployment [Citation245–247]. Thus, high levels of both aerobic and muscular fitness have been recommended for adequate occupational performance for both structural and wildland firefighters [Citation248].
4.2.3. Job-specific health and performance burdens
As outlined in previous sections, firefighters routinely engage in high-stress activities (such as active fire suppression) that are oftentimes countered with extended periods of low physical activity. This dichotomy, combined with poor nutritional choices [Citation249,Citation250], ongoing and intermittent elevations in stress hormones [Citation251–253], increased rates of obesity [Citation254], and poor fitness levels [Citation255], are all considered to be leading contributors to poor long-term health of a firefighter.
4.2.4. Key Points: Demands of First Responders
First responders are exposed to various physiological, psychological, and environmental stressors that increase their risk for cardiorespiratory and metabolic disease.
First responders are routinely sleep deprived and suffer long-term consequences of sleep deprivation, which impact both their mental and physical health.
Adequate physical fitness levels are required for optimal occupational performance and overall health, an outcome that commonly is not achieved.
First responders need to be ready at a moment’s notice, shifting from a sedentary state to a state of high-intensity or maximal physical exertion.
First Responders’ specific energy needs are difficult to plan against because of the unknown essential occupational tasks energy needs and the inevitable increased energy demand from PPE, load carriage, and environmental stressors (i.e. heat exposure).
4.3. Current health status and nutrition habits of first responders
The primary nutritional consideration for tactical athletes, particularly firefighters and law enforcement officers, is to actively take steps to first improve one’s health. Thus, it must be stressed that tactical athletes should establish and maintain a nutritious eating plan as their foundation and build toward strategies aimed at performance optimization.
4.3.1. Law enforcement officers
Despite less research on this occupation, law enforcement personnel appear to exhibit similar health challenges in terms of obesity and CVD when compared to firefighters. In 2014, Can and Hendy indicated that as much as 40.5% of US law enforcement officers may be obese [Citation256]. Currently, no consensus exists as to which approach commonly used to assess obesity (i.e., BMI, percent body fat, or waist circumference) is considered best practice for law enforcement populations. While some feel BMI may be appropriate, other data in this population suggest that BMI fails to appropriately categorize individuals as obese compared to when using BF% and waist circumference [Citation257–259]. Additionally, CVD appears to be more prevalent among law enforcement officers, current and retirees, than among the general population (odds ratio = 1.70, 95% confidence interval = 1.03 to 2.79) [Citation260,Citation261]. These findings suggest that while fueling for occupational performance is important within these populations, nutritional and physical activity strategies aimed at improving general health should be prioritized as the first line of defense against the incidence of premature morbidity and mortality with these groups.
4.3.2. Firefighters
For firefighters, heart attacks are the leading cause of mortality (~45% of on-duty deaths and 30% of deaths overall) [Citation255,Citation262,Citation263]. Furthermore, other data suggest that firefighters have a high prevalence of CVD risk factors, with ~75% of career and volunteer firefighters being overweight or obese [Citation255,Citation264,Citation265]. In addition, it has been reported that obese firefighters demonstrate higher total cholesterol, lower HDL cholesterol, higher triglycerides, higher blood pressure, and lower fitness and aerobic capacity compared to those firefighters who are of healthy weight [Citation264]. Further, Soteriades et al. [Citation262] found that in U.S. firefighters, CVD events in the line of duty occurred exclusively in those with underlying (non-job-related) CVD. These findings support the notion that CVD mortality in firefighters is strongly connected to poor lifestyle habits such as inadequate levels of regular physical activity and poor nutritional habits as opposed to stresses experienced during the job.
4.3.3. Key Points: Current Health Status and Nutrition Habits of First Responders
First responders demonstrate a higher prevalence of obesity, CVD, cancer, and other chronic diseases when compared to the general population.
Nutrition practitioners are advised to focus efforts on healthy eating habits and strategies with the goal of supporting long-term health while simultaneously optimizing occupational performance.
Like many aspects of the general population, first responders are faced with several challenges that they must overcome to improve their physical activity and improve the quality of their diet.
Strategies such as implementing wellness policies, setting up supportive food environments, encouraging healthier food systems, and using community resources to offer evidence-based nutrition classes are inexpensive and potentially meaningful ways to improve dietary habits.
4.4. General energy and macronutrient guidelines for first responders
Similar to the general population, first responders should strive to consume a daily diet that provides appropriate amounts of energy, macronutrients, and micronutrients in accordance with their daily energy and nutrient needs. While little is known about the nutrient intake of first responders, guidance can be found in nutritional recommendations intended for the general population. Currently, no accurate assessment of caloric expenditure is established for on-duty first responders, and this is an area of critical need for more research. Recommended approaches to estimate total energy expenditure include using the Mifflin St. Jeor [Citation266] or Harris-Benedict Equations [Citation267,Citation268] (see ) with 1) the appropriate activity factor for activities of daily living and 2) the additional calories to account for those expended through physical activity (leisure and occupational) using pre-established metabolic equivalents (METs) [Citation269] or some form of commercial heart rate and physical activity monitor that can estimate energy expenditure. However, it is important to note that these commercially available wearable devices vary considerably, and measurements of energy expenditure may be inaccurately estimated. Additional recommendations for estimating total energy expenditure would include referring to the Compendium of Physical Activity. However, the 2011 Compendium of Physical Activities lists only four specific activities for law enforcement officers and firefighters. Future research should further assess common occupational tasks to expand the Compendium of Physical Activity specific to first responder populations. The International Society of Sports Nutrition position stands outline energy and macronutrient intake guidelines for individuals engaged in general fitness programs, as well as increasing intensities of exercise [Citation69,Citation270], which may aid first responders depending on the intensity and volume of their exercise training. outlines recommendations for nutritional strategies depending on the training involvement of the individual (i.e. intermediate, advanced, or endurance training) (). Additionally, the Acceptable Macronutrient Distribution Ranges (AMDRs) for the general healthy adult population are also recommendations first responders should consider [Citation271].
Table 2. Basal Energy Expenditure Equations.
Table 3. Recommended Nutritional Strategies for Individuals Involved in Intermediate Training.
Table 4. Recommended nutritional strategies for athletic populations (Advanced/Endurance Training).
4.5. Hydration
4.5.1. Considerations for first responder hydration
Optimal hydration supports vital physiological processes (i.e., hydrolysis, heat absorption, joint lubrication, waste removal, etc.), while dehydration has progressive and potentially severe implications for physical performance and cognition [Citation272]. The Institute for Medicine recommends that 2.7 L (~11.5 cups) and 3.7 L (~15.5 cups) of total water per day are sufficient to meet the adequate intake values in 19- to 50-year-old females and males, respectively [Citation273]. Although precise hydration guidelines have yet to be established for tactical athletes, these recommendations do appear to meet the needs of insensible water loss routes, which occur during increased respiration and perspiration when exposed to elevated temperatures. Ideally, the tactical athlete would incorporate hydration strategies to prevent dehydration beyond ~2% of bodyweight, as anything beyond this threshold has been proposed to significantly impair physical performance and cognition [Citation274,Citation275]. However, people, in general, are poor evaluators of their sweat loss, with recent data demonstrating that approximately one out of every two athletes (168 out of 297) underestimated their sweat loss in hot environmental conditions [Citation276]. Although hydration guidelines are based on approximate sweat loss estimations, firefighters, and tactical athletes alike, can incorporate practical hydration strategies within their respective units from previously collected data. Cheuvront and Sawka [Citation82] suggest a three-step approach for assessing and correcting potential hypohydration states by having athletes each morning (1) evaluate if they are thirsty, (2) monitor their urine color using the urine color chart by Armstrong et al. [Citation277,Citation278], and (3) weigh themselve each morning to determine if body mass is lower than normal. Based on these assessments, if an athlete has one symptom, they may be hypo-hydrated, if two symptoms are present, then they are likely hypo-hydrated, and if three symptoms are present, then they are very likely hypo-hydrated. Based on these assessments, practical hydration strategies outlined above can then be pursued appropriately.
Athlete recommendations for rehydration could be useful for tactical athletes as well. As pre-hydration for a firefighting event is impractical, firefighters should be recommended to first meet their daily water needs as advised by the Institute of Medicine and do so by spreading the water intake out evenly throughout the day. After physically demanding training or event response, as well as after exercise, firefighters are advised to replenish fluids at a rate of 0.4-0.8L/h of activity. In conditions of high heat/humidity or activities >2 h in duration, fluid replacement should include electrolytes [Citation279].
4.5.2. Special Considerations for Hydration among Firefighters
During any structural or active-duty fire suppression, firefighters wear PPE that provides a protective barrier from dangerously hot environmental conditions [Citation280]. As such, PPE significantly limits the heat dissipation that occurs from sweat, resulting in increased heat accumulation and cardiovascular strain. This combination provides a continual physiological cue for sweat production that can ultimately lead to dehydration [Citation281–283]. It has been clearly established that dehydration decreases performance [Citation284,Citation285], compromises thermoregulation [Citation286–288], and impairs cardiovascular responses during exercise [Citation289,Citation290]. While limited data are available on firefighters’ hydration needs, it has been demonstrated that during a victim search and rescue firefighting training simulation, average skin temperature rose from 34.5 to 37.4◦C, average heart rate was 153 beats·min−1, and core temperature increased from 37.7 to 39◦C [Citation291], while ambient temperatures in close proximity to the fire routinely exceed 100oC. Although these responses are dependent on multiple factors, this evidence clearly highlights the importance of a firefighter being aware of the challenges of their body in terms of fluid balance and thermoregulation while performing their occupational duties. Similar to recommendations for many athletes, tactical populations are advised against using thirst as an indicator of hydration status, and they are further advised to regularly assess their body mass before and after training as well as active fire suppression activities. After dehydrating physical exertion, consuming a volume of water that is equivalent to 150% of the lost body mass is advised.
4.5.3. Key Points: Hydration
Like the general public, tactical athletes are poor predictors of their hydration status and generally wait too long to begin actively hydrating.
Dehydration is a serious threat to tactical performance as well as firefighter health. PPE exponentially decreases potential convection cooling currents as well as the evaporative potential of the skin. All told these considerations increase the rate of dehydration.
Tactical populations should implement a three-step approach (i.e., evaluate, monitor, weigh) within their departments to better assist in maintaining euhydration.
4.5.4. Diet considerations
As highlighted throughout an earlier part of this position stand, firefighters and first responders are challenged by irregular patterns of physical activity, hyperenergetic dietary patterns, and increasing rates of obesity, cardiovascular diseases, and other associated comorbidities. While both professions have the potential to operate in unique and oftentimes extremely stressful environments, very little is known regarding barriers that exist and preclude law enforcement officers and firefighters from being able to make appropriate changes to mitigate obesity development and poor fitness. From a dietary perspective, no evidence is available that warrants the recommendation of one type of dietary pattern in favor of another. Thus, all first responders who desire to maintain or improve their health should first do so by modifying their dietary habits that result in daily consumption of nutritious food that delivers an appropriate balance of energy, macronutrients, fiber, and micronutrients.
A foundational tenet of every dietary program that successfully achieves weight loss is the maintenance of a negative energy balance whereby the daily energy expended is greater than what is consumed in the diet. As advised to the general population and in position stands by Aragon et al. [Citation292] and Donnelly et al. [Citation293], tactical athletes who need to lose weight are advised to focus on creating a negative energy balance through a combination of energy restriction and increasing their amount of daily physical activity. While discussing the efficacy of every potential dietary strategy is beyond the scope of this position stand, approaches such as portion control, limiting the intake of energy-dense foods and ultra-processed foods, and increasing the intake of nutrient-dense foods and dietary fiber are excellent approaches upon which to focus. On the physical activity side of the energy balance equation, individuals are advised to follow ACSM guidelines for physical activity that advocate for a combination of aerobic and anaerobic activity, resulting in a minimum of 30 min of moderate-intensity exercise most days of the week [Citation294]. While other modes, styles, and patterns of exercise can certainly be employed with high prospects of success at supporting weight loss and improving health, detailing each of these options is beyond the scope of this position stand.
As one example, the Mediterranean Diet continues to garner interest and popularity for its less restrictive approach to food choices [Citation295] and pattern of evidence documenting its potential to reduce the risk for CVD including favorable changes in blood lipids, protection against cancer while promoting longevity [Citation296,Citation297], and supporting weight loss [Citation296]. Furthermore, Yang et al. [Citation298] previously reported that consumption of the Mediterranean Diet in a large group of Midwestern firefighters was inversely related to weight gain (odds ratio = 0.57, 95% confidence interval = 0.39–0.84), increased HDL cholesterol (p = 0.008), and decreased LDL cholesterol (p = 0.04). However, more research is needed in both firefighter and first responder populations to better understand how this eating approach can support weight loss, improvements in health, disease risk reduction, and performance optimization.
Currently, intervention approaches using firefighters and especially first responder populations are limited, with a small number of investigations having been completed using various time-restricted feeding approaches and others that have examined low-carbohydrate diets. Time-restricted feeding (TRF) has increased in popularity in recent years and collectively can be viewed as any feeding pattern that consists of distinct fasting and feeding windows [Citation292]. In this respect, a commonly used approach involves following a 16-h fasting period and an 8-h feeding period, while other human and rodent trials involving TRF have included a range of fasting durations ranging from 10 to 21 h [Citation299]. Previous work has illustrated the potential for TRF approaches to improve blood lipids and markers of inflammation in active, trained, and overweight populations [Citation299–301]. Additionally, recent work by McAllister et al. [Citation302] showed that such results achieved from TRF may not be entirely linked to caloric restriction, as individuals following a 16:8 (fasting: feeding) TRF intervention for 28 days experienced significant reductions in body fat percentage, blood pressure, and increased concentrations of HDL-cholesterol and adiponectin under both isocaloric and ad libitum conditions. More research is needed in different populations and for longer periods of evaluation before definitive conclusions can be drawn. In consideration of these findings, others have examined the potential impact that TRF may have on firefighter health [Citation303]. For example, McAllister et al. [Citation304] examined the impact of a 6-week TRF intervention among professional firefighters following a 14:10 fasting: feeding protocol. Significant reductions in markers of inflammation and oxidative stress were found, while no change was found in other markers of cardiometabolic health (lipids, glucose) or numerous inflammatory cytokines (i.e., IL-6, IL8, TNF-α). A later study by the same research group employed an 8-week TRF protocol and found reductions in IL-6 and IL-1β and reduced cortisol in response to a simulated protocol of fire ground activities (i.e., ladder carry, hose deployments, victim removal, and forced entry tasks) in firefighters [Citation228]. In terms of performance, two studies by Moro et al. [Citation305,Citation306] have demonstrated the ability of TRF to improve body composition while maintaining levels of strength and endurance. In firefighters, Gonzalez et al. [Citation307] demonstrated that 7 weeks of TRF (14:10 fasting: feeding) did not negatively impact fitness/performance variables, such as vertical jump height, one-repetition maximum for the bench press and back squat, or the number of repetitions completed for push-up and inverted row among firefighters; however, ventilatory threshold (VT) values did improve from 65.2% to 70.0% throughout the TRF intervention. Finally, McAllister et al. [Citation308] reported that 8 weeks of TRF (14:10 fasting: feeding) did not adversely impact time to completion during a fire simulated assessment consisting of nine occupation-specific tasks. These data suggest TRF may afford cardiometabolic health benefits to firefighters without negatively impacting physical performance; however, more work is needed to confirm these results.
While initially advocated for clinical outcomes (e.g., drug-resistant epilepsy patients) and later for reducing BF%, low-carbohydrate diets have garnered interest among tactical athletes, such as firefighters, as a means of reducing body fat and improving metabolic health and performance. In a 2015 survey, firefighters reported that the Paleo (~9%) and low-carbohydrate diets (~8%) were more frequently practiced than low-fat (~4%) or the Mediterranean Diet (1%), while diets that emphasized fruits and vegetables, as well as seafood and lean meats (i.e., Mediterranean Diet and Paleo), were viewed more favorably than diets that eliminated whole food groups [Citation295]. To date, only one study has implemented a carbohydrate-restricted diet (CRD) in a firefighter cohort [Citation309]. Briefly, after a 1-hour CRD nutrition counseling session, 15 firefighters followed a 28-day CRD protocol and examined markers of cardiometabolic health, CVD risk, and performance. Improvements included a reduction in fat mass (~2.4 kg; specifically, around the abdominal section), decreases in systolic (6.0 mm Hg) and diastolic (5.0 mm Hg) blood pressure, increased rates of fat oxidation (p < 0.05) during exercise, and several cardiometabolic markers of health (i.e. malondialdehyde, AOPP [advanced oxidation protein products], HDL cholesterol, and triglycerides). Moreover, occupational performance was also improved through observed decreases in their 2.41-km run time (~3%) and an increase in their maximal pull-up repetitions (~15%) in 2 min. Due to the small sample size and limited duration, more research is needed to further explore the potential impact a low-carbohydrate diet may have on the health and performance of first responders. In addition, a notable observation by Waldman et al. [Citation310] highlighted the previous findings by Yang et al. [Citation295] that indicated firefighter populations prefer a diet higher in animal proteins, which may increase feelings of satiety and naturally decrease overall caloric intake. Therefore, it is likely not the decrease in carbohydrates but rather the increase in protein consumption that makes the adoption of a CRD an effective nutrition intervention for fire communities. This observation aligns with well-established guidelines for optimizing body composition, where an emphasis is placed on calorie reduction while simultaneously increasing protein ingestion [Citation311]. So, as seen with other parts of the general population, the increased intake of protein in place of carbohydrates during CRD protocols may aid in reducing overall caloric intake in first responder populations. Finally, low-carbohydrate, ketogenic approaches have also increased in popularity. While these diets do have some data to demonstrate their potential to reduce CVD risk and fat mass [Citation312,Citation313], more research is needed, especially in first responders and other such populations, before broader recommendations can be made.
In summary, several dietary approaches can be employed to help first responder populations improve their health, lose fat, and improve performance. While nearly all available studies have examined the impact of dietary interventions among firefighters, it is highly likely these interventions will offer similar benefits to law enforcement personnel. As it stands, more research is needed, particularly randomized, controlled research studies, that seek to examine the impact of any potential modulator toward health and performance are strongly encouraged. Results from these types of investigations will best inform researchers and practitioners on the potential efficacy of various exercise and diet combinations to improve the health and performance of all first responders.
4.5.5. Key points: diet considerations
First responder populations are challenged by irregular and often low physical activity patterns and poor dietary choices that commonly deliver an excess of energy, dietary fat, and cholesterol.
Several dietary approaches exist that can aid in weight loss and improve cardiovascular health, with all efficacious approaches being grounded upon achieving a reduction in energy intake.
The Mediterranean Diet is a preferred diet by firefighters and has been shown to effectively aid in the prevention of CVD, numerous types of cancer, and obesity.
Initial research in TRF suggests it may help improve markers of cardiometabolic health and CVD risk in firefighters by reducing inflammation and stress markers without compromising physical performance.
Moderately restricting carbohydrates and increasing the proportion of protein in the diet may facilitate improvements in body composition and provide sufficient satiation in tactical athletes.
4.6. Dietary supplementation for first responders
Dietary supplement use in the general population is widespread, with estimates suggesting that as much as 50% of US adults regularly use some form of dietary supplements [Citation314]. Currently, the rate or pattern of usage of dietary supplements by first responder populations is unknown, and, to date, limited studies have been conducted using first responder populations. Several ingredients exist that could potentially afford some benefit to first responders, and these are outlined in . It is important to highlight that first responder populations should prioritize lifestyle changes that will help reduce their risk for obesity, cardiovascular disease, and other associated comorbidities. Dietary supplements may be a viable strategy to help first responders achieve these goals; however, very little research has been done in the area of dietary supplements in first responder populations. Subsequently, sufficient evidence to support the use of many dietary supplements within this population cannot be substantiated at this point. Certainly, there seems to be sufficient amount of literature to support the safety and efficacy of certain supplements, such as caffeine, creatine, protein, and essential amino acids, among first responder populations when used in the recommended amounts. The interested reader is encouraged to review the position stands on caffeine [Citation315], creatine [Citation116], and protein [Citation68] to better understand how these supplements may be incorporated into a first responder’s regimen. Additionally, many of the recommendations put forth in the military section of this document (Part I) offer relevant insight for those working with first responder populations. Finally, while these documents are comprehensive of the current literature for these ingredients, more research is needed to better understand how the unique demands of first responder environments interact with supplementation of caffeine, creatine, and protein. For example, caffeine is well-established for its ability to improve attention, vigilance, and ergogenic properties, but more research is needed in first responder populations to better understand how caffeine’s effects interact with the (sometimes) poor physical condition, poor cardiovascular health, and extreme occupational environments experienced by firefighters. While the safety profile of caffeine is well established, future work should clearly determine what dosages or daily intakes of caffeine are suitable for these specific populations. In this respect, previous work has highlighted that caffeine dosages of 6 mg/kg may elicit a higher coagulation response during firefighting drills [Citation316], while other studies reported that high caffeine intakes may increase core body temperature of firefighters when performance activities took place in full PPE [Citation317]. As a result, more research is warranted to assess how caffeine intake from supplementation interacts with firefighter cardiovascular risk profiles, strenuous firefighting activities with live fire drills, and analysis of hemostatic variables following engagement in firefighting activities. Moreover, creatine monohydrate supplementation in athletes during hot and humid exercise conditions has been shown to increase body water and reduce heart rate, rectal temperature, and sweat rate. The impact of these changes on firefighters during active fire suppression is largely unknown.
Other ingredients are available that may afford potential benefits for first responders. However, very few have published literature to inform specific recommendations for these populations. Toward this aim, isolated antioxidants such as curcumin and astaxanthin have been shown to act as antioxidative/anti-inflammatory agents [Citation318,Citation319] that can improve aspects of cardiometabolic health [Citation320–323], reduce biological markers of inflammation or oxidative stress, and improve recovery from muscle damaging exercise [Citation324–327]. The challenge, however, with both of these ingredients is that their literature bases are limited in scope overall and are largely non-existent for first responders. As a result, more research is needed before firm recommendations surrounding these ingredients can be made before generalizing these results to first responder populations. Finally, one study involving ketone supplementation has examined its potential to impact oxidative stress, performance, and cardiovascular outcomes among firefighters. In this study, [Citation328] supplemented firefighters with 7 days of an exogenous ketone salt and found no effect on pre- or post-exercise markers of oxidative stress or antioxidant status (glutathione, oxidized glutathione, superoxide dismutase, catalase, and total antioxidant capacity) [Citation329]. In addition, the ketone salts did appear to have a small but significant cardiovascular effect as exercising HR was lowered (~3%) in firefighters while performing steady-state exercise in their PPE. While several other areas of investigation have been completed for ketone salts for both health and ergogenic outcomes, more research is needed to better understand their impact.
4.6.1. Key points: dietary supplementation for first responders
First responders should emphasize the incorporation of regular physical activity and consumption of a nutrient-dense diet to support maintaining general health, healthy weight and body composition, and occupational performance.
First responders likely consume nutritional supplements in a similar pattern as what is observed in the general population or recreational athletes.
Due to the unique and extreme occupational conditions upon which first responders operate, future research should determine what supplements are being routinely consumed in this population.
While limited research has been conducted in first responders with ingredients such as caffeine, creatine, protein, and essential amino acids, the literature base on general populations of each of these ingredients provides widespread evidence of both their safety and efficacy.
Due to the unique and extreme environments in which some first responders operate, specific research studies using caffeine, creatine, protein, and amino acid should be completed to confirm their safety and efficacy in first responder populations.
Other ingredients such as curcumin, astaxanthin, and ketone salts have been shown to reduce markers of oxidative stress and inflammation as well as improve recovery from exercise or enhance physical performance; however, benefits in first responder populations have yet to be shown.
Future studies should assess the efficacy and safety of any dietary supplement that is purported to aid in the improvement in health, performance, or recovery first responder populations.
5. Conclusion
Tactical athletes, including military personnel and first responders, work in unique occupational environments that challenge them both physically and mentally. As such, maintaining and optimizing health and performance are critical issues for all tactical athletes. As outlined throughout, appropriate fueling and hydration are critical for tactical athlete readiness and to improve health status, reduce the risk of injury and disease, and improve performance. To date, limited research has been conducted among these populations to identify fueling and hydration requirements. Currently, most of the available recommendations are derived from both general and athletic populations, and further research is needed to best understand the specific health and performance needs of these populations. While performance optimization is important, focused efforts must first be made to establish a pattern of physical activity and dietary intake that reduces the risk for cardiometabolic disease and increases fitness. As more data emerge, specific guidelines surrounding various dietary patterns and dietary supplement use will be generated, and while warfighters, firefighters, and law enforcement personnel all fall under the single term ‘tactical athlete’, the focus of provisions and timing may significantly differ across occupations and designations. As this information becomes more available, practitioners working with these populations should continually consider the occupation-specific stressors, energy expenditure, physical fitness requirements/recommendations, and job-related health burdens to which these populations are exposed in order to effectively put forth recommendations and guidelines that are practical and will promote optimal health and performance.
6. Position of the International Society of Sports Nutrition (ISSN)
Based on a comprehensive review and critical analysis of the literature regarding tactical athlete nutrition, conducted by experts in the field and selected members of the International Society of Sports Nutrition (ISSN), the following conclusions represent the official Position of the Society:
6.1. Tactical athletes
Tactical, or occupational, athletes perform some of the most physically demanding jobs within our society. Considerations to facilitate optimal health and performance should include the provision and timing of adequate intake of calories, macronutrients, and fluid, as well as strategic supplementation practices. Optimal nutritional practices can greatly improve physical, cognitive, and occupational performance outcomes as well as offset the impact of sleep deprivation; reduce the risk of injury, obesity, and cardiometabolic disease; and reduce the potential for a fatal mistake.
Personal protective equipment (PPE), load carriage requirements, and certain environmental stressors increase the demands placed upon the tactical athlete and may entail increased rate of dehydration and risk of musculoskeletal injuries depending on the occupational tasks performed. These factors are important considerations for practitioners working with these populations, especially when assessing energy and nutrient needs, which may significantly vary depending on occupation and designations.
Whenever possible, tactical athletes should optimize their personal health and wellness by implementing nutrition and supplementation strategies, which will also enhance occupational readiness.
These occupations take a steep toll on the mental and physical health and wellness of these individuals; therefore, although on-the-job best nutritional practices are needed to optimize readiness, overall health and wellness of these operators is paramount.
6.2. Recommendations for military
Military personnel are faced with various tactical-specific stressors depending on their occupation and requirements for high operational tempo and sustainment operation conditions. The current body of evidence suggests several key nutritional and supplementation strategies for the warfighter to optimize readiness while reducing the risk of injury or fatal mistakes.
The warfighter should aim to meet the caloric demands of occupational tasks by utilizing the Military Dietary Reference Intakes (MDRIs) established and codified in the Army Regulation 40-25.
Caffeine anhydrous consumption of 100 to 200 mg (100 mg per 2 h or 200 mg per 4 h) is recommended for operational utility and during periods of sleep deprivation. Doses up to 200 to 300 mg have been suggested to improve reaction time and marksmanship. Available evidence indicates that total daily caffeine amounts of 800 mg (200 mg doses administered with 2- to 3-h intervals between doses over a 24-h period) may help attenuate the negative effects of successive sleep-deprived states (i.e. sustained training and combat operations).
Creatine monohydrate supplementation may afford the warfighter improved exercise capacity, enhanced recovery, and/or reduced muscle damage during periods of intense physical exertion, lowered the risk for skeletal muscle-related injuries and issues (i.e., strains/pulls), enhanced heat tolerance, and improved cognition. Recommended doses are 0.3 g/kg/day (about 20 g/day) for 5–7 days followed by 0.1 g/kg/day (about 3–5 g/day) thereafter. Larger individuals may need 5–10 g/day to maintain elevated creatine stores.
During sustained training and combat operations, the warfighter is subjected to rapid and pronounced catabolism of whole-body and skeletal muscle protein, which can be mitigated by matching energy consumption with energy expenditure. Protein intake should be a key focus, and supplementation with essential amino acids has previously been shown to help negate protein catabolism during sustained training and combat operations.
Cognitive performance is critical to mission success for the warfighter. Evidence exists to support supplementation and inclusion of omega-3 fatty acids, creatine monohydrate, beta-alanine, and L-tyrosine to aid cognitive performance, especially during sustained training and combat operations and periods of sleep deprivation or chronic sleep restriction.
6.3. Recommendations for first responders
First responders are exposed to various physiological, psychological, environmental, and occupational-specific stressors that can increase their risk for cardiometabolic disease and may negatively impact duty performance. Presently, specific energy needs for occupation-specific tasks are unknown, and further research is needed to elucidate and develop better nutritional practices for first responders.
Little is known about the energy and nutrient intake needs of first responders in relation to physical activity levels and occupational tasks. However, it is likely that these populations would benefit from the general caloric intake and macronutrient guidelines for recreational athletes, given the nature of their occupation.
Nutrition practitioners are advised to focus education efforts on healthy eating habits and strategies with a primary goal to support long-term health while simultaneously optimizing occupational performance.
Strategies such as implementing wellness policies, setting up supportive food environments, encouraging healthier food systems, and using community resources to offer evidence-based nutrition classes are inexpensive and potentially meaningful ways to improve physical activity and diet habits.
A prudent guideline is for first responders to follow general nutrition guidelines and consume energy and macronutrient amounts that fall in line with the Acceptable Macronutrient Distribution Ranges for the general healthy adult population.
Practitioners working with first responders should estimate total energy expenditure using the Mifflin St. Jeor or Harris-Benedict Equations to appropriately estimate nutritional needs. Of note to readers, this is a critical area of need for research to establish the daily energy requirements of this population.
Limited research exists concerning dietary interventions in first responders. Trials on time-restricted feeding and carbohydrate-restricted dieting have shown promise in reducing adverse health risks while maintaining or optimizing physical and occupational performance among firefighters. More research is needed to investigate dietary approaches in first responders.
The Mediterranean Diet is preferred by the fire community and is one of the several potential dietary interventions that may improve the health of first responders. More research is needed in both law enforcement and firefighter populations to better understand the potential benefits of various dietary interventions.
Research concerning supplementation in first responders is limited; however, dietary supplements such as caffeine, creatine, protein powders, antioxidants, and essential amino acids may afford these individuals benefits during high-stress conditions.
Ethics approval and consent to participate
This paper was reviewed by the International Society of Sports Nutrition Research Committee and represents the official position of the Society.
Acknowledgments
The authors are particularly grateful toward military Service members as well as first responders. We would like to thank all participants and researchers who contributed to the research studies and reviews discussed in this position stand.
Funding: No funding was provided.
Author Contributions: RBK, BIC, JA, DSK, and JRS conceived the position stand. RBK, BIC, JA, DSK, JRS, TAV, and DEG identified key contributors. RBK provided guidance and supervision to DEG in preparing the initial draft of the position stand. DEG, MJM, HSW, AAF, JJ, and CMK prepared and compiled the initial draft. NDB, JJD, AJK, TH, JRS, TNZ, AZ, JLT, JLH, TAV, DSK, BIC, JA, and RBK provided contributions and edits to the initial draft. All authors reviewed, edited, and approved the draft and the final manuscript.
Competing Interests: Authors of this paper have not received any financial remuneration for preparing or reviewing this paper. DEG, MJM, HSW, AAF, JJ, NDB, JJD, AJK, TH, TNZ, AZ, JLT, JLH, DSK, and BIC have no competing interests. All authors report the following competing interests: CMK consults with and receives external funding from companies for delivering scientific presentations at conferences and writes online, print, and other media on topics related to exercise, nutrition, and protein-related companies. CMK has also served as an expert witness and provided testimonies related to exercise, supplementation, and nutrition. JRS has conducted industry-sponsored research regarding creatine and other nutraceuticals over the past 25 years, as well as received financial support for presenting on the science of various nutraceuticals at industry-sponsored scientific conferences. TAV has received research grants related to dietary supplementals. JA is the CEO of the ISSN. RBK has conducted industry-sponsored research regarding creatine, received financial support for presenting on creatine at industry-sponsored scientific conferences, and has served as an expert witness on cases related to supplementation.
Disclosure statement
No potential conflict of interest was reported by the author(s).
Additional information
Funding
References
- Davis MR, Easter RL, Carlock JM, et al. Self-reported physical tasks and exercise training in Special Weapons and Tactics (SWAT) Teams. J Strength Cond Res. 2016 Nov;30(11):3242–3248.
- Charles DJJK, Crespo D, Elder CL, et al. Does body mass index influence the physiological and perceptual demands associated with defensive tactics training in state patrol officers?. Int J Exercise Sci. 2018;11(6):319–330.
- Johnson QR, Goatcher JD, Diehl C, et al. Heart rate responses during simulated fire ground scenarios among full-time firefighters. Int J Exercise Sci. 2020;13(2):374–382.
- Knapik JJ, Reynolds KL, Harman E. Soldier load carriage: historical, physiological, biomechanical, and medical aspects. Mil Med. 2004 Jan;169(1):45–56.
- Baran K, Dulla LTJ, Orr R, et al. Duty loads carried by the Los Angeles Sheriff’s department deputies. J Austr Str Cond. 2018;26(5):34–38.
- R. M. Orr, R. R., Pope, V., Johnston, J, Coyle.2012 The operational load carriage context of the Australian army soldier. In 1st Australian Conference on Physiological and Physical Employment Standards. Australia .
- Scofield DE, Kardouni JR. The tactical athlete: a product of 21st century strength and conditioning. Strength & Conditioning J. 2015;37(4):2–7.
- Kraemer WJ, Szivak TK. Strength training for the warfighter. J Strength Cond Res. 2012 Jul;26 Suppl 2(Supplement 2):S107–18.
- Murphy NE, Carrigan CT, Philip Karl J, et al. Threshold of energy deficit and lower-body performance declines in military personnel: a meta-regression. Sports Med. 2018 Sep;48(9):2169–2178.
- Nindl BC, Barnes BR, Alemany JA, et al. Physiological consequences of U.S. army ranger training. Med Sci Sports Exerc. 2007 Aug;39(8):1380–1387.
- Sepowitz JJ, Armstrong NJ, Pasiakos SM. Energy balance and diet quality during the us marine corps forces special operations command individual training course. J Spec Oper Med. 2017;17(4):109–113.
- Nindl BC, Leone CD, Tharion WJ, et al. Physical performance responses during 72 h of military operational stress. Med Sci Sports Exerc. 2002 Nov;34(11):1814–1822.
- Castellani J, Nindl B, Lieberman H, et al. Decrements in human performance during 72-84 hours of sustained operations. J Spec Oper Med. 2006;11(1):9.
- Abriat A, Brosset C, Brégigeon M, et al. Report of 182 cases of exertional heatstroke in the French armed forces. Mil Med. 2014;179(3):309–314.
- Edholm OG. 1969. The effec of heat on accliatized and unacclimatized groups of very fit men. Proceedings of the Royal Society of Medicine, 62: 1175–1179. SAGE Publications.
- Armstrong LE, Maresh CM. The induction and decay of heat acclimatisation in trained athletes. Sports Med. 1991;12(5):302–312.
- Carter IIIR, Cheuvront SN, Williams JO, et al. Epidemiology of hospitalizations and deaths from heat illness in soldiers. Medicine and science in sports and exercise, 2005, 37(8): 1338–1344. doi:10.1249/01.mss.0000174895.19639.ed .
- Garrett AT, Rehrer NJ, Patterson MJ. Induction and decay of short-term heat acclimation in moderately and highly trained athletes. Sports Med. 2011;41(9):757–771.
- Basnyat B, Murdoch DR. High-altitude illness. Lancet. 2003;361(9373):1967–1974.
- Institute of Medicine Committee on Military Nutrition R. In: Marriott BM, Carlson SJ, editors Nutritional needs in cold and in high-altitude environments: applications for military personnel in field operations. Washington (DC): National Academies Press (US) Copyright 1996 by the National Academy of Sciences. All rights reserved; 1996 A Review of the Physiology and Nutrition in Cold and in High-Altitude Environments by the Committee on Military Nutrition Research .
- Netzer N, Strohl K, Faulhaber M, et al. Hypoxia-related altitude illnesses. J Travel Med. 2013 Jul-Aug;20(4):247–255.
- Cudaback DD. 4-KM altitude effects on performance and health. Pub Astron Soc Paci. 1984; 96(1):463.
- Bahrke MS, Shukitt-Hale B. Effects of altitude on mood, behaviour and cognitive functioning. A review. Sports Med. 1993 Aug;16(2):97–125.
- Yan X. Cognitive impairments at high altitudes and adaptation. High Alt Med Biol. 2014 Jun;15(2):141–145.
- Hackney AC, Shaw JM, Hodgdon JA, et al. Cold exposure during military operations: effects on anaerobic performance. J Appl Physiol. 1985];71(1):125–130.
- Gefen A, Epstein Y. The mechanobiology and mechanophysiology of military-related injuries. Vol. 19. Berlin: Springer; 2016.
- Jaworski RL, Jensen A, Niederberger B, et al. Changes in combat task performance under increasing loads in active duty marines. Mil Med. 2015;180(suppl_3):179–186.
- Pedersen AV, Stokke R, Mamen A. Effects of extra load position on energy expenditure in treadmill running.Eur J Appl Physiol. 2007;102(1):27–31.
- Soule RG, Pandolf KB, Goldman RF. Energy expenditure of heavy load carriage. Ergonomics. 1978 May;21(5):373–381.
- Majumdar D, Pal MS, Majumdar D. Effects of military load carriage on kinematics of gait. Ergonomics. 2010 Jun;53(6):782–791.
- Seay JF. Biomechanics of load carriage—historical perspectives and recent insights. J Strength Cond Res. 2015;29(Supplement 11):S129–S133.
- Orr RM, Coyle J, Johnston V, et al. Self-reported load carriage injuries of military soldiers. Int J Inj Contr Saf Promot. 2017 Jun;24(2):189–197.
- Knapik JJ. Injuries and injury prevention during foot marching. J Spec Oper Med. 2014;14(4):131–135.
- Fallowfield JL, Blacker SD, Willems ME, et al. Neuromuscular and cardiovascular responses of royal marine recruits to load carriage in the field. Appl Ergon. 2012 Nov;43(6):1131–1137.
- Scott PA, Ramabhai L. Load carrying: in situ physiological responses of an infantry platoon. Ergonomics. 2000;1:18–24.
- Crowder TA, Beekley MD, Sturdivant RX, et al. Metabolic effects of soldier performance on a simulated graded road march while wearing two functionally equivalent military ensembles. Mil Med. 2007 Jun;172(6):596–602.
- Baker-Fulco CJ, Kramer FM, Lesher LL, et al. Dietary intakes of female and male combat support hospital personnel subsisting on meal-focused or standard versions of the meal, ready-to-eat. Army Res Inst Env Med. 2002;
- Hoyt R, Jones T, Stein T, et al. Doubly labeled water measurement of human energy expenditure during strenuous exercise. J Appl Physiol. 1991;71(1):16–22.
- Tharion WJ, Baker-Fulco CJ, Bovill ME, et al. Adequacy of garrison feeding for special forces soldiers during training. Mil Med. 2004 Jun;169(6):483–490.
- Shippee R, Askew E, Bernton E, et al. Nutritional and immunological assessment of ranger students with increased caloric intake. Army Res Inst Env Med. 1994;
- Fairbrother B, Shippee R, Kramer T, et al. Nutritional and immunological assessment of soldiers during the special forces assessment and selection course. Army Res Inst Env Med. 1995;
- Barringer ND, Pasiakos SM, McClung HL, et al. Prediction equation for estimating total daily energy requirements of special operations personnel. J Int Soc Sports Nutr. 2018;15(1):15.
- Shrestha A, Ho TE, Vie LL, et al. Comparison of cardiovascular health between us army and civilians. J Am Heart Assoc. 2019;8(12):e009056.
- McGraw LK, Turner BS, Stotts NA, et al. A review of cardiovascular risk factors in us military personnel. J Cardiovasc Nurs. 2008;23(4):338–344.
- Almond N, Kahwati L, Kinsinger L, et al. The prevalence of overweight and obesity among U.S Military Veterans. Military Med. 2008;173(6):544–549.
- Army US. 2016. Health of the Force Report. Available at https://phc.amedd.army.mil/Periodical%20Library/2016HealthoftheForcereport.pdf2016
- Shiozawa B, Madsen C, Banaag A, et al. Body mass index effect on health service utilization among active duty male United States army soldiers. Mil Med. 2019;184(9–10):447–453.
- Patel S. Reduced sleep as an obesity risk factor. Obesity Rev. 2009;10:61–68.
- Spiegal K, Tasali E, Penev P, et al. Sleep curtailment in healthy young men is associated with decreased leptin levels, elevated ghrelin levels and increased hunger and appetite. Ann Int Med. 2004;141(11):846–850.
- Nedeltcheva AV, Kilkus JM, Imperial J, et al. Sleep curtailment is accompanied by increased intake of calories from snacks. Am J Clin Nutr. 2009;89(1):126–133.
- Heath G, Roach GD, Dorrian J, et al. The effect of sleep restriction on snacking behaviour during a week of simulated shiftwork. Accid Anal Prev. 2012;45:62–67.
- Klingenberg L, Chaput J-P, Holmbäck U, et al. Acute sleep restriction reduces insulin sensitivity in adolescent boys. Sleep. 2013;36(7):1085–1090.
- Donga E, Van Dijk M, Van Dijk JG, et al. Partial sleep restriction decreases insulin sensitivity in type 1 diabetes. Diabetes Care. 2010;33(7):1573–1577.
- Spiegel K, Leproult R, Van Cauter E. Impact of sleep debt on metabolic and endocrine function. Lancet. 1999;354(9188):1435–1439.
- Ulmer CS, Bosworth HB, Germain A, et al. Associations between sleep difficulties and risk factors for cardiovascular disease in veterans and active duty military personnel of the Iraq and Afghanistan conflicts. J Behav Med. 2015 Jun;38(3):544–555.
- Grier T, Dinkeloo E, Reynolds M, et al. Sleep duration and musculoskeletal injury incidence in physically active men and women: a study of U.SArmy Special Operation Forces soldiers. Sleep Health. 2020Jun;63: 344–349.
- Jacobson IG, Ryan MA, Hooper TI, et al. Alcohol use and alcohol-related problems before and after military combat deployment. Jama. 2008 Aug 13;300(6):663–675.
- Mysliwiec V, McGraw L, Pierce R, et al. Sleep disorders and associated medical comorbidities in active duty military personnel. Sleep. 2013 Feb 1;36(2):167–174.
- Fox BD, Judge LW, Dickin DC, et al. Biomechanics of military load carriage and resulting musculoskeletal injury: a review. J orthopedic Sur. 2020;1(1).
- Sanders JW, Putnam SD, Frankart C, et al. Impact of illness and non-combat injury during operations Iraqi freedom and enduring freedom (Afghanistan). Am J Trop Med Hyg. 2005 Oct;73(4):713–719.
- Lincoln AE, Smith GS, Amoroso PJ, et al. The natural history and risk factors of musculoskeletal conditions resulting in disability among US Army personnel. Work (Reading, Mass). 2002;18(2):99–113.
- George SZ, Childs JD, Teyhen DS, et al. Predictors of occurrence and severity of first time low back pain episodes: findings from a military inception cohort. PLoS One. 2012;7(2):e30597.
- Jones BH, Knapik JJ. Physical training and exercise-related injuries. Surveillance, research and injury prevention in military populations. Sports Med. 1999 Feb;27(2):111–125.
- Cowan DN, Bedno SA, Urban N, et al. Musculoskeletal injuries among overweight army trainees: incidence and health care utilization. Occup Med (Lond). 2011 Jun;61(4):247–252.
- Sammito S, Hadzic V, Karakolis T, et al. Risk factors for musculoskeletal injuries in the military: a qualitative systematic review of the literature from the past two decades and a new prioritizing injury model. Mil Med Res. 2021;8(1):66.
- Kerksick CM, Arent S, Schoenfeld BJ, et al. International society of sports nutrition position stand: nutrient timing. J Int Soc Sports Nutr. 2017;14(1):33.
- Regulation A. 2017. 40–25, Medical Services Nutrition and Menu Standards for Human Performance Optimization. Headquarters Departments of the Army, The Navy and the Air Force, 1-20.
- Jager R, Kerksick CM, Campbell BI, et al. International society of sports nutrition position stand: protein and exercise. J Int Soc Sports Nutr. 2017;14(1):20.
- Kerksick CM, Wilborn CD, Roberts MD, et al. Exercise & sports nutrition review update: research & recommendations. J Int Soc Sports Nutr. 2018;15(1):38.
- Gwin JA, Church DD, Wolfe RR, et al. Muscle protein synthesis and whole-body protein turnover responses to ingesting essential amino acids, intact protein, and protein-containing mixed meals with considerations for energy deficit. Nutrients. 2020 Aug 15;12(8):2457.
- Pasiakos SM, Austin KG, Lieberman HR, et al. Efficacy and safety of protein supplements for U.S. armed forces personnel: consensus statement. J Nutr. 2013 Nov;143(11):1811S–1814S.
- Pasiakos SM, Margolis LM, Orr JS. Optimized dietary strategies to protect skeletal muscle mass during periods of unavoidable energy deficit. FASEB J. 2015 Apr;29(4):1136–1142.
- Margolis LM, Murphy NE, Martini S, et al. Effects of supplemental energy on protein balance during 4-d arctic military training. Med Sci Sports Exerc. 2016 Aug;48(8):1604–1612.
- Margolis LM, Murphy NE, Martini S, et al. Effects of winter military training on energy balance, whole-body protein balance, muscle damage, soreness, and physical performance. Appl Physiol Nutr Metab. 2014 Dec;39(12):1395–1401.
- Carbone JW, McClung JP, Pasiakos SM. Recent advances in the characterization of skeletal muscle and whole-body protein responses to dietary protein and exercise during negative energy balance. Adv Nutr. 2019 Jan 1; 10(1):70–79.
- Berryman CE, Young AJ, Karl JP, et al. Severe negative energy balance during 21 d at high altitude decreases fat-free mass regardless of dietary protein intake: a randomized controlled trial. FASEB J. 2018;32(2):894–905.
- Gwin JA, Church DD, Hatch-McChesney A, et al. Essential amino acid-enriched whey enhances post-exercise whole-body protein balance during energy deficit more than iso-nitrogenous whey or a mixed-macronutrient meal: a randomized, crossover study. J Int Soc Sports Nutr. 2021 Jan 7;18(1):4.
- Gwin JA, Church DD, Hatch-McChesney A, et al. Effects of high versus standard essential amino acid intakes on whole-body protein turnover and mixed muscle protein synthesis during energy deficit: a randomized, crossover study. Clin Nutr. 2021 Mar;40(3):767–777.
- Church DD, Hirsch KR, Park S, et al. Essential amino acids and protein synthesis: insights into maximizing the muscle and whole-body response to feeding. Nutrients. 2020 Dec 2;12(12):3717.
- Rodriguez NR, DiMarco NM, Langley S. Position of the American dietetic association, dietitians of Canada, and the American college of sports medicine: nutrition and athletic performance. J Am Diet Assoc. 2009 Mar;109(3):509–527.
- Kenefick RW, Hazzard MP, Mahood NV, et al. Thirst sensations and AVP responses at rest and during exercise-cold exposure. Med Sci Sports Exerc. 2004 Sep;36(9):1528–1534.
- Cheuvront SN, Sawka MN. Hydration assessment of athletes. Sports Sci Exchange. 2005;18(2):1–6.
- Fletcher RH, Fairfield KM. Vitamins for chronic disease prevention in adults: clinical applications. Jama. 2002;287(23):3127–3129.
- Alver BA, Sell K, Deuster PA. NSCA’s essentials of tactical strength and conditioning. Human Kinetics. 2017;
- Kris-Etherton PM, Harris WS, Appel LJ. Fish Consumption, Fish Oil, Omega-3 Fatty Acids, and Cardiovascular Disease. Circulation. 2002;106(21):2747–2757.
- Costello RB, Lentino CV, Boyd CC, et al. The effectiveness of melatonin for promoting healthy sleep: a rapid evidence assessment of the literature. Nutrition Journal. 2014;13(1):106
- Trexler ET, Smith-Ryan AE, Stout JR, et al. International society of sports nutrition position stand: beta-Alanine. J Int Soc Sports Nutr. 2015;12(1):30.
- Artioli GG, Gualano B, Smith A, et al. Role of beta-alanine supplementation on muscle carnosine and exercise performance. Med Sci Sports Exerc. 2010 Jun;42(6):1162–1173.
- Perim P, Marticorena FM, Ribeiro F, et al. Can the skeletal muscle carnosine response to beta-alanine supplementation be optimized?. Front Nutr. 2019;6:135.
- Hoffman J, Stout J, Harris R, et al. β-Alanine supplementation and military performance. Amino Acids. 2015 07/24; 47;47(12): 2463–2474.
- Hoffman JR, Landau G, Stout JR, et al. β-alanine supplementation improves tactical performance but not cognitive function in combat soldiers. J Int Soc Sports Nutr. 2014;11(1):15.
- Hoffman JR, Landau G, Stout JR, et al. β-Alanine ingestion increases muscle carnosine content and combat specific performance in soldiers. Amino Acids. 2015 Mar;47(3):627–636.
- Knapik JJ, Austin KG, McGraw SM, et al. Caffeine consumption among active duty United States Air Force personnel. Food Chem Toxicol. 2017 Jul;105:377–386.
- Knapik JJ, Trone DW, McGraw S, et al. Caffeine use among active duty navy and marine corps personnel. Nutrients. 2016;8(10):620.
- Chaudhary NS, Taylor BV, Grandner MA, et al. The effects of caffeinated products on sleep and functioning in the military population: a focused review. Pharmacol Biochem Behav. 2021 Jul;206:173206.
- Keisler BD, Armsey TD. Caffeine as an ergogenic aid. Curr Sports Med Rep. 2006;54:215–219.
- Guest NS, VanDusseldorp TA, Nelson MT, et al. International society of sports nutrition position stand: caffeine and exercise performance. J Int Soc Sports Nutr. 2021;18(1):1.
- McLellan TM, Bell DG, Kamimori GH. Caffeine improves physical performance during 24 h of active wakefulness. Aviat Space Environ Med. 2004;75(8):666–672.
- Kamimori GH, McLellan TM, Tate CM, et al. Caffeine improves reaction time, vigilance and logical reasoning during extended periods with restricted opportunities for sleep. Psychopharmacology (Berl). 2015 Jun;232(12):2031–2042.
- Amendola CA, Gabrieli JDE, Lieberman HR. Caffeine’s effects on performance and mood are independent of age and gender.Nutr Neurosci. 1998;1(4):269–280.
- Fine BJ, Kobrick JL, Lieberman HR, et al. Effects of caffeine or diphenhydramine on visual vigilance. Psychopharmacology (Berl). 1994;114(2):233–238.
- Rees K, Allen D, Lader M. The influences of age and caffeine on psychomotor and cognitive function. Psychopharmacology (Berl). 1999;145(2):181–188.
- Butt MS, Sultan MT. Coffee and its consumption: benefits and risks.Crit Rev Food Sci Nutr. 2011;51(4):363–373.
- McLellan TM, Riviere LA, Williams KW, et al. Caffeine and energy drink use by combat arms soldiers in Afghanistan as a countermeasure for sleep loss and high operational demands. Nutr Neurosci. 2019 Nov;22(11):768–777.
- Crawford C, Teo L, Lafferty L, et al. Caffeine to optimize cognitive function for military mission-readiness: a systematic review and recommendations for the field. Nutr Rev. 2017 Jun 1;75(suppl_2):17–35.
- Tharion WJ, Shukitt-Hale B, Lieberman HR. Caffeine effects on marksmanship during high-stress military training with 72 hour sleep deprivation. Aviat Space Environ Med. 2003 Apr;74(4):309–314.
- Tikuisis P, Keefe AA, McLellan TM, et al. Caffeine restores engagement speed but not shooting precision following 22 h of active wakefulness. Aviat Space Environ Med. 2004;75(9):771–776.
- Lieberman HR, Tharion WJ, Shukitt-Hale B, et al. Effects of caffeine, sleep loss, and stress on cognitive performance and mood during U.S. Navy SEAL training sea-air-land. Psychopharmacology (Berl). 2002 Nov;164(3):250–261.
- Childs E, Hohoff C, Deckert J, et al. Association between ADORA2A and DRD2 polymorphisms and caffeine-induced anxiety. Neuro Psycho. 2008;33(12):2791–2800.
- Rétey JV, Adam M, Khatami R, et al. A genetic variation in the adenosine A2A receptor gene (ADORA2A) contributes to individual sensitivity to caffeine effects on sleep. Clin Pharmacol Ther. 2007 May;81(5):692–698.
- Lopes-Silva JP, Choo HC, Franchini E, et al. Isolated ingestion of caffeine and sodium bicarbonate on repeated sprint performance: a systematic review and meta-analysis. J Sci Med Sport. 2019 Aug;22(8):962–972.
- Drake C, Roehrs T, Shambroom J, et al Caffeine effects on sleep taken 0, 3, or 6 hours before going to bed. J Clin Sleep Med. 2013;09(11):1195–1200.
- Toblin RL, Adrian AL, Hoge CW, et al. Energy drink use in U.S. Service members after deployment: associations with mental health problems, aggression, and fatigue. Mil Med. 2018 Nov 1;183(11–12):e364–e370.
- Johnson LA, Foster D, McDowell JC. Energy drinks: review of performance benefits, health concerns, and use by military personnel. Mil Med. 2014;179(4):375–380.
- Campbell B, Wilborn C, La Bounty P, et al. International society of sports nutrition position stand: energy drinks. J Int Soc Sports Nutr. 2013;10(1):1.
- Kreider RB, Kalman DS, Antonio J, et al. International society of sports nutrition position stand: safety and efficacy of creatine supplementation in exercise, sport, and medicine. J Int Soc Sports Nutr. 2017;14(1):18.
- Wax B, Kerksick CM, Jagim AR, et al. Creatine for exercise and sports performance, with recovery considerations for healthy populations. Nutrients. 2021 Jun 2;13(6):1915.
- Cooke MB, Rybalka E, Williams AD, et al. Creatine supplementation enhances muscle force recovery after eccentrically-induced muscle damage in healthy individuals. J Int Soc Sports Nutr. 2009 Jun 2;6(1):13.
- Greenwood M, Kreider RB, Greenwood L, et al. Cramping and injury incidence in collegiate football players are reduced by creatine supplementation. J Athl Train. 2003 Sep;38(3):216–219.
- Greenwood M, Kreider RB, Melton C, et al. Creatine supplementation during college football training does not increase the incidence of cramping or injury. Mol Cell Biochem. 2003 Feb;244(1–2):83–88.
- Kilduff LP, Georgiades E, James N, et al. The effects of creatine supplementation on cardiovascular, metabolic, and thermoregulatory responses during exercise in the heat in endurance-trained humans. Int J Sport Nutr Exerc Metab. 2004 Aug;14(4):443–460.
- Dolan E, Gualano B, Rawson ES. Beyond muscle: the effects of creatine supplementation on brain creatine, cognitive processing, and traumatic brain injury. Eur J Sport Sci. 2019 Feb;19(1):1–14.
- Cook CJ, Crewther BT, Kilduff LP, et al. Skill execution and sleep deprivation: effects of acute caffeine or creatine supplementation - a randomized placebo-controlled trial. J Int Soc Sports Nutr. 2011 Feb 16;8(1):2.
- McMorris T, Harris RC, Swain J, et al. Effect of creatine supplementation and sleep deprivation, with mild exercise, on cognitive and psychomotor performance, mood state, and plasma concentrations of catecholamines and cortisol. Psychopharmacology (Berl). 2006 Mar;185(1):93–103.
- Roschel H, Gualano B, Ostojic SM, et al. Creatine supplementation and brain health. Nutrients. 2021;13(2):586.
- Kreider RB, Melton C, Rasmussen CJ, et al. Long-term creatine supplementation does not significantly affect clinical markers of health in athletes. Mol Cell Biochem. 2003 Feb;244(1–2):95–104.
- Kreider RB, Stout JR. Creatine in health and disease. Nutrients. 2021 Jan 29; 13(2):447.
- Watanabe A, Kato N, Kato T. Effects of creatine on mental fatigue and cerebral hemoglobin oxygenation. Neurosci Res. 2002 Apr;42(4):279–285.
- Hammett ST, Wall MB, Edwards TC, et al. Dietary supplementation of creatine monohydrate reduces the human fMRI BOLD signal. Neurosci Lett. 2010 Aug 2;479(3):201–205.
- Dechent P, Pouwels P, Wilken B, et al. Increase of total creatine in human brain after oral supplementation of creatine-monohydrate. Am J Physiol Regul Integr Comp Physiol. 1999;277(3):R698–R704.
- Kreider RB. Effects of creatine supplementation on performance and training adaptations. Mol Cell Biochem. 2003 Feb;244(1–2):89–94.
- KREIDER RB, Klesges RC, Lotz D, et al. Effects of nutritional supplementations during off-season college football training on body composition and strength. J Exer Phys. 1999;244(1–2):89–94
- Kreider RB, Stout JR. Creatine in health and disease. Nutrients. 2021;13(2):447.
- Kreider RB, Jäger R, Bioavailability PM. Efficacy, safety, and regulatory status of creatine and related compounds: a critical review. Nutrients. 2022;14(5):1035.
- Tipton KD, Gurkin BE, Matin S, et al. Nonessential amino acids are not necessary to stimulate net muscle protein synthesis in healthy volunteers. J Nutr Biochem. 1999 Feb;10(2):89–95.
- Wolfe RR, Baum JI, Starck C, et al. Factors contributing to the selection of dietary protein food sources. Clin Nutr. 2018 Feb;37(1):130–138.
- Cuthbertson DJ, Babraj J, Leese G, et al. Anabolic resistance does not explain sarcopenia in patients with type 2 diabetes mellitus, compared with healthy controls, despite reduced mTOR pathway activity. Clin Nutr. 2017 Dec;36(6):1716–1719.
- Dillon EL, Sheffield-Moore M, Paddon-Jones D, et al. Amino acid supplementation increases lean body mass, basal muscle protein synthesis, and insulin-like growth factor-I expression in older women. J Clin Endocrinol Metab. 2009 May;94(5):1630–1637.
- Ispoglou T, White H, Preston T, et al. Double-blind, placebo-controlled pilot trial of L-Leucine-enriched amino-acid mixtures on body composition and physical performance in men and women aged 65-75 years. Eur J Clin Nutr. 2016 Feb;70(2):182–188.
- Negro M, Perna S, Spadaccini D, et al. Effects of 12 Weeks of Essential Amino Acids (EAA)-based multi-ingredient nutritional supplementation on muscle mass, muscle strength, muscle power and fatigue in healthy elderly subjects: a randomized controlled double-blind study. J Nutr Health Aging. 2019;23(5):414–424.
- Dreyer HC, Owen EC, Strycker LA, et al. Essential amino acid supplementation mitigates muscle atrophy after total knee arthroplasty: a randomized, double-blind, placebo-controlled trial. JB JS Open Access. 2018 Jun 28;3(2):e0006.
- Dreyer HC, Strycker LA, Senesac HA, et al. Essential amino acid supplementation in patients following total knee arthroplasty. J Clin Invest. 2013 Nov;123(11):4654–4666.
- Rasmussen BB, Tipton KD, Miller SL, et al. An oral essential amino acid-carbohydrate supplement enhances muscle protein anabolism after resistance exercise. J Appl Physiol. 2000;88(2):386–392.
- Tipton KD, Ferrando AA, Phillips SM, et al Postexercise net protein synthesis in human muscle from orally administered amino acids. A J Physiol. 1999 Apr;276(4 Pt 1):E628–34.
- Tipton KD, Rasmussen BB, Miller SL, et al. Timing of amino acid-carbohydrate ingestion alters anabolic response of muscle to resistance exercise. Am J Physiol Endocrinol Metab. 2001;281(2):E197–206.
- Kerksick CM, Arent S, Schoenfeld BJ, et al. International society of sports nutrition position stand: nutrient timing. J Int Soc Sports Nutr. 2017;14(1):33.
- Kerksick CM, Leutholtz B. Nutrient administration and resistance training. J Int Soc Sports Nutr. 2005;2(1):50–67.
- Miller SL, Tipton KD, Chinkes DL, et al. Independent and combined effects of amino acids and glucose after resistance exercise. Med Sci Sports Exerc. 2003 Mar;35(3):449–455.
- Tipton KD, Borsheim E, Wolf SE, et al. Acute response of net muscle protein balance reflects 24-h balance after exercise and amino acid ingestion. Am J Physiol (Endocrinol Metab). 2003 Jan;284(1):E76–89.
- Moore DR, Churchward-Venne TA, Witard O, et al. Protein ingestion to stimulate myofibrillar protein synthesis requires greater relative protein intakes in healthy older versus younger men. J Gerontol A Biol Sci Med Sci. 2015 Jan;70(1):57–62.
- Gorissen SHM, Crombag JJR, Senden JMG, et al. Protein content and amino acid composition of commercially available plant-based protein isolates. Amino Acids. 2018 Dec;50(12):1685–1695.
- Moore DR. Maximizing post-exercise anabolism: the case for relative protein intakes. Front Nutr. 2019;6:147.
- Pasiakos SM, Cao JJ, Margolis LM, et al. Effects of high-protein diets on fat-free mass and muscle protein synthesis following weight loss: a randomized controlled trial. FASEB J. 2013 Jun 5;27(9):3837–3847.
- Longland TM, Oikawa SY, Mitchell CJ, et al. Higher compared with lower dietary protein during an energy deficit combined with intense exercise promotes greater lean mass gain and fat mass loss: a randomized trial. Am J Clin Nutr. 2016 Mar;103(3):738–746.
- Beary JF 3rd, Walter LJ Jr., Johns JH. Leading causes of death for active duty military personnel. Mil Med. 1984 Jun;149(6):316–317.
- Castro CA, Kintzle S. Suicides in the military: the post-modern combat veteran and the Hemingway effect.Curr Psychiatry Rep. 2014;16(8):460.
- Vincent AS, Roebuck-Spencer T, Lopez MS, et al. Effects of military deployment on cognitive functioning. Mil Med. 2012 Mar;177(3):248–255.
- Kim HY. Neuroprotection by docosahexaenoic acid in brain injury. Mil Med. 2014 Nov;179(11 Suppl):106–111.
- Montain S, Jonas WB. Nutritional armor: omega-3 for the warfighter. Mil Med. 2014 Nov;179(11 Suppl):1.
- Brenna JT, Salem N Jr., Sinclair AJ, et al. alpha-Linolenic acid supplementation and conversion to n-3 long-chain polyunsaturated fatty acids in humans. Prostaglandins Leukot Essent Fatty Acids. 2009 Feb-Mar;80(2–3):85–91.
- Kitson AP, Metherel AH, Chen CT, et al. Effect of dietary docosahexaenoic acid (DHA) in phospholipids or triglycerides on brain DHA uptake and accretion. J Nutr Biochem. 2016 Jul;33:91–102.
- Bailes JE, Patel V. The potential for DHA to mitigate mild traumatic brain injury. Mil Med. 2014 Nov;179(11 Suppl):112–116.
- Oliver JM, Jones MT, Kirk KM, et al. Effect of docosahexaenoic acid on a biomarker of head trauma in American football. Med Sci Sports Exerc. 2016 Jun;48(6):974–982.
- Heileson JL, Anzalone AJ, Carbuhn AF, et al. The effect of omega-3 fatty acids on a biomarker of head trauma in NCAA football athletes: a multi-site, non-randomized study. J Int Soc Sports Nutr. 2021 ;18(1):65.
- Black KE, Witard OC, Baker D, et al. Adding omega-3 fatty acids to a protein-based supplement during pre-season training results in reduced muscle soreness and the better maintenance of explosive power in professional Rugby Union players. Eur J Sport Sci. 2018 Nov;18(10):1357–1367.
- Fontani G, Corradeschi F, Felici A, et al. Cognitive and physiological effects of omega-3 polyunsaturated fatty acid supplementation in healthy subjects. Eur J Clin Invest. 2005 Nov;35(11):691–699.
- Fontani G, Lodi L, Migliorini S, et al. Effect of omega-3 and policosanol supplementation on attention and reactivity in athletes. J Am Coll Nutr. 2009 Aug;28 Suppl(sup4):473s–481s.
- Guzmán JF, Esteve H, Pablos C, et al. DHA- rich fish oil improves complex reaction time in female elite soccer players. J Sports Sci Med. 2011;10(2):301–305.
- Lewis MD, Hibbeln JR, Johnson JE, et al. Suicide deaths of active-duty US military and omega-3 fatty-acid status: a case-control comparison. J Clin Psychiatry. 2011 Dec;72(12):1585–1590.
- Hallahan B, Hibbeln JR, Davis JM, et al. Omega-3 fatty acid supplementation in patients with recurrent self-harm. Br J Psychiatry. 2007 Feb;190(2):118–122.
- Hibbeln JR, Gow RV. The potential for military diets to reduce depression, suicide, and impulsive aggression: a review of current evidence for omega-3 and omega-6 fatty acids. Mil Med. 2014 Nov;179(11 Suppl):117–128.
- Philpott JD, Witard OC, Galloway SDR. Applications of omega-3 polyunsaturated fatty acid supplementation for sport performance. Res Sports Med. 2019 Apr-Jun;27(2):219–237.
- Lewis NA, Daniels D, Calder PC, et al. Are there benefits from the use of fish oil supplements in athletes? a systematic review. Adv Nutr. 2020 Sep 1;11(5):1300–1314.
- Shei RJ, Lindley MR, Mickleborough TD. Omega-3 polyunsaturated fatty acids in the optimization of physical performance. Mil Med. 2014 Nov;179(11 Suppl):144–156.
- Coulter ID. The response of an expert panel to nutritional armor for the warfighter: can omega-3 fatty acids enhance stress resilience, wellness, and military performance?. Mil Med. 2014 Nov;179(11 Suppl):192–198.
- Heileson JL, Funderburk LK. The effect of fish oil supplementation on the promotion and preservation of lean body mass, strength, and recovery from physiological stress in young, healthy adults: a systematic review. Nutr Rev. 2020 Dec 1; 78(12):1001–1014.
- VanDusseldorp TA, Escobar KA, Johnson KE, et al. Impact of varying dosages of fish oil on recovery and soreness following eccentric exercise. Nutrients. 2020 Jul 27;12(8):2246.
- Kyriakidou Y, Wood C, Ferrier C, et al. The effect of omega-3 polyunsaturated fatty acid supplementation on exercise-induced muscle damage. J Int Soc Sports Nutr. 2021 Jan 13;18(1):9.
- Tsuchiya Y, Ueda H, Yanagimoto K, et al. 4-week eicosapentaenoic acid-rich fish oil supplementation partially protects muscular damage following eccentric contractions. J Int Soc Sports Nutr. 2021 Mar 1;18(1):18.
- Lieberman HR. Amino acid and protein requirements: cognitive performance, stress and brain function the role of protein and amino acids in sustaining and enhancing performance.J Int Soc Sports Nutr. 1999: 289–307.
- Mahoney CR, Castellani J, Kramer FM, et al Tyrosine supplementation mitigates working memory decrements during cold exposure. Physiol Behav. 2007;92(4):575–582.
- Shurtleff D, Thomas JR, Schrot J, et al Tyrosine reverses a cold-induced working memory deficit in humans. Pharmacol Biochem Behav. 1994;47(4):935–941.
- Ott T, Nieder A. Dopamine and cognitive control in prefrontal cortex. Trends Cogn Sci. 2019;23(3):213–234.
- Spencer RC, Devilbiss DM, Berridge CW. The cognition-enhancing effects of psychostimulants involve direct action in the prefrontal cortex. Biol Psychiatry. 2015;77(11):940–950.
- Gao W-J, Krimer LS, Goldman-Rakic PS. Presynaptic regulation of recurrent excitation by D1 receptors in prefrontal circuits. Proc Nat Acad Sci. 2001;98(1):295–300.
- Pomeroy DE, Tooley KL, Probert B, et al.A systematic review of the effect of dietary supplements on cognitive performance in healthy young adults and military personnel. Nutrients. 2020;12(2):545.
- Orr R, Hinton B, Wilson A, et al.Investigating the routine dispatch tasks performed by police officers. Safety. 2020;6(4):54.
- Bissett D, Bissett J, Snell C. Physical agility tests and fitness standards: perceptions of law enforcement officers Police Practice & Research An Int J. 2012;13(3):208–223.
- Carlton S, Carbone P, Stierli M, et al. The impact of occupational load carriage on the mobility of the tactical police officer. J Aust Str Cond. 2014;22:32–37.
- Thomas M, Pohl MB, Shapiro R, et al. Effect of load carriage on tactical performance in special weapons and tactics operators. J Strength Cond Res. 2018 Feb;32(2):554–564.
- Ramstrand N, Zügner R, Larsen LB, et al. Evaluation of load carriage systems used by active duty police officers: relative effects on walking patterns and perceived comfort. Appl Ergon. 2016 Mar;53:36–43.
- Marins EF, Cabistany L, Farias C, et al Effects of personal protective equipment on metabolism and performance during an occupational physical ability test for federal highway police officers. J Strength Cond Res. 2020 Apr;34(4):1093–1102.
- Keeler JM, Pohl MB, Bergstrom HC, et al. The effect of tactical tasks and gear on muscle activation of SWAT officers. J Strength Cond Res. 2022;36(1):238–244.
- Violanti JM, Burchfiel CM, Miller DB, et al. The Buffalo Cardio-Metabolic Occupational Police Stress (BCOPS) pilot study: methods and participant characteristics. Ann Epidemiol. 2006 Feb;16(2):148–156.
- Berg BL. First day at the police academy: stress-reaction-training as a screening-out technique. J Cont Crim Just. 1990;6(2):89–105.
- Pryor RR, Colburn D, Crill MT, et al. Fitness characteristics of a suburban special weapons and tactics team. J Strength Cond Res. 2012;26(3):752–757.
- DiVencenzo HR, Morgan AL, Laurent CM, et al. Metabolic demands of law enforcement personal protective equipment during exercise tasks. Ergonomics. 2014;57(11):1760–1765.
- Dorman LE, Havenith G. The effects of protective clothing on energy consumption during different activities. Eur J Appl Physiol. 2009 Feb;105(3):463–470.
- Maupin D, Robinson J, Wills T, et al. Profiling the metabolic fitness of a special operations police unit. J Occup Health. 2018;60(5):356–360.
- Ramey SL, Downing NR, Knoblauch A. Developing strategic interventions to reduce cardiovascular disease risk among law enforcement officers: the art and science of data triangulation. AAOHN J. 2008;56(2):54–62.
- Varvarigou V, Farioli A, Korre M, et al. Law enforcement duties and sudden cardiac death among police officers in United States: case distribution study. BMJ. 2014;349(nov18 2):g6534.
- Kales SN, Soteriades ES, Christoudias SG, et al. Firefighters and on-duty deaths from coronary heart disease: a case control study. Environ Health. 2003;2(1):14.
- Sörensen L, Smolander J, Louhevaara V, et al. Physical activity, fitness and body composition of Finnish police officers: a 15-year follow-up study. Occup Med. 2000;50(1):3–10.
- Boyce R, JONES G, Lloyd C, et al. A longitudinal observation of police: body composition changes over 12 years with gender and race comparisonS. J Exercise Physiol Online. 2008;11(6): 1–13.
- Michell V, Samaria C, Júnior Rudy N, et al. Effects of a concurrent physical exercise program on aerobic power and body composition in adults. J Sports Med Phys Fitness. 2014;54(4):441–446.
- Violanti JM, Ma CC, Fekedulegn D, et al. Associations between body fat percentage and fitness among police officers: a statewide study. Saf Health Work. 2017;8(1):36–41.
- Williford HN, Scharff-Olson M. Fitness and body fat: an issue of performance. Fire Engineering. 1998.
- Ricciardi R, Deuster PA, Talbot LA. Effects of gender and body adiposity on physiological responses to physical work while wearing body armor. Mil Med. 2007;172(7):743–748.
- Dawes J, Orr RM, Elder C, et al. Association between body fatness and measures of muscular endurance among part-time SWAT officers. J Aust Str Cond. 2014;22(4): 33–37.
- Anderson AA, Yoo H, Franke WD. Associations of physical activity and obesity with the risk of developing the metabolic syndrome in law enforcement officers. J Occup Environ Med. 2016 Sep;58(9):946–951.
- Andrew ME, Shengqiao L, Wactawski-Wende J, et al. Adiposity, muscle, and physical activity: predictors of perturbations in heart rate variability. Am J Hum Biol. 2013 May-Jun;25(3):370–377.
- Frykman P, Harman E, Pandorf C. Correlates of obstacle course performance among female soldiers carrying two different loads. Army Res Inst Env Med. 2001;25(3):370–377.
- Pandorf CE, Harman EA, Frykman PN, et al. Correlates of load carriage and obstacle course performance among women. Work. 2002;18(2):179–189.
- Abel MG, Palmer TG, Trubee N. Exercise program design for structural firefighters. Strength & Conditioning J. 2015;37(4):8–19.
- Rodríguez-Marroyo JA, López-Satue J, Pernía R, et al. Physiological work demands of Spanish wildland firefighters during wildfire suppression. Int Arch Occup Environ Health. 2012;85(2):221–228.
- Eves ND, Jones RL, Petersen SR. The influence of the self-contained breathing apparatus (SCBA) on ventilatory function and maximal exercise. Can J Appl Physiol. 2005 Oct;30(5):507–519.
- Louhevaara V, Smolander J, Tuomi T, et al. Effects of an SCBA on breathing pattern, gas exchange, and heart rate during exercise. J Occ Med Asso. 1985;27(3): 213–216.
- Louhevaara V, Tuomi T, Korhonen O, et al. Cardiorespiratory effects of respiratory protective devices during exercise in well-trained men. Eur J Appl Physiol Occup Physiol. 1984;52(3):340–345.
- Punakallio A, Lusa S, Luukkonen R. Protective equipment affects balance abilities differently in younger and older firefighters. Aviat Space Environ Med. 2003;74(11):1151–1156.
- Park K, Rosengren KS, Horn GP, et al. Assessing gait changes in firefighters due to fatigue and protective clothing. Saf Sci. 2011;49(5):719–726.
- Lesniak AY, Bergstrom HC, Clasey JL, et al. The effect of personal protective equipment on firefighter occupational performance. J Strength Cond Res. 2020 Aug;34(8):2165–2172.
- Taylor NAS, Lewis MC, Notley SR, et al. A fractionation of the physiological burden of the personal protective equipment worn by firefighters. Eur J Appl Physiol. 2012;112(8):2913–2921.
- Bugajska J, Zuzewicz K, Szmauz-Dybko M, et al. Cardiovascular stress, energy expenditure and subjective perceived ratings of fire fighters during typical fire suppression and rescue tasks. Int J Occup Saf Ergon. 2007;13(3):323–331.
- Sothmann MS, Saupe K, Jasenof D, et al. Heart rate response of firefighters to actual emergencies. implications for cardiorespiratory fitness. J Occup Med. 1992 Aug;34(8):797–800.
- Williams-Bell FM, Villar R, Sharratt MT, et al. Physiological demands of the firefighter Candidate physical ability test. Med Sci Sports Exerc. 2009 Mar;41(3):653–662.
- Lemon PW, Hermiston RT. The human energy cost of fire fighting. J Occup Med. 1977 Aug;19(8):558–562.
- O’Connell ER, Thomas PC, Cady LD, et al. Energy costs of simulated stair climbing as a job-related task in fire fighting. J Occup Med. 1986 Apr;28(4):282–284.
- McAllister MJ, Gonzalez AE, Waldman HS. Time restricted feeding reduces inflammation and cortisol response to a firegrounds test in professional firefighters. J Occup Environ Med. 2021;63(5):441–447.
- von Heimburg ED, Rasmussen AK, Medbø JI. Physiological responses of firefighters and performance predictors during a simulated rescue of hospital patients. Ergonomics. 2006 Feb 10; 49(2):111–126.
- Gledhill N, Jamnik VK. Characterization of the physical demands of firefighting. Can J Sport Sci. 1992 Sep;17(3):207–213.
- Sharkey B, Rothwell T. Validation and field evaluation of a work capacity test for wildland firefighters 467. Med Sci Sports Exercise. 1996;28(5):79.
- Sol JA, Ruby BC, Gaskill SE, et al. Metabolic demand of hiking in wildland firefighting. Wilderness Environ Med. 2018 Sep;29(3):304–314.
- Fahy RF, Petrillo JT, Molis JL. Firefighter fatalities in the US-2019. NFPA Res. 2019: 1–26.
- Fahy RF. US firefighter fatalities due to sudden cardiac death, 1995-2004. MA: National Fire Protection Association Quincy; 2005.
- Kales SN, Soteriades ES, Christophi CA, et al. Emergency duties and deaths from heart disease among firefighters in the United States. N Engl J Med. 2007 Mar 22;356(12):1207–1215.
- Walker A, Keene T, Argus C, et al. Immune and inflammatory responses of Australian firefighters after repeated exposures to the heat. Ergonomics. 2015;58(12):2032–2039.
- Wolkow A, Aisbett B, Jefferies S, et al. Effect of heat exposure and simulated physical firefighting work on acute inflammatory and cortisol responses. Ann Work Expo Health. 2017 Jun 1;61(5):600–603.
- Ridker PM, Rifai N, Stampfer MJ, et al. Plasma concentration of interleukin-6 and the risk of future myocardial infarction among apparently healthy men. Circulation. 2000 Apr 18;101(15):1767–1772.
- Hunter AL, Shah ASV, Langrish JP, et al. Fire simulation and cardiovascular health in firefighters. Circulation. 2017;135(14):1284–1295.
- Fahs CA, Yan H, Ranadive S, et al. Acute effects of firefighting on arterial stiffness and blood flow. Vascular Medicine. 2011;16(2):113–118.
- Fernhall B, Fahs CA, Horn G, et al. Acute effects of firefighting on cardiac performance. Eur J Appl Physiol. 2012;112(2):735–741.
- Smith DL, Fehling PC, Frisch A, et al. The prevalence of cardiovascular disease risk factors and obesity in firefighters. J Obes. 2012;2012:908267.
- Bilzon JL, Scarpello EG, Smith CV, et al. Characterization of the metabolic demands of simulated shipboard royal navy fire-fighting tasks. Ergonomics. 2001 Jun 20;44(8):766–780.
- Budd G, Brotherhood J, Hendrie A, et al. Project aquarius 5. activity distribution, energy expenditure, and productivity of men suppressing free-running wildland fires with hand tools. Int J Wil Fire. 1997;7(2):105–118.
- Smith DL. Firefighter fitness: improving performance and preventing injuries and fatalities. Curr Sports Med Rep. 2011;10(3):167–172.
- Rhea MR, Alvar BA, Gray R. Physical fitness and job performance of firefighters. J Strength Cond Res. 2004 May;18(2):348–352.
- Xu D, Song Y, Meng Y, et al. Relationship between firefighter physical fitness and special ability performance: predictive research based on machine learning algorithms. Int J Environ Res Public Health. 2020;17(20):7689.
- Houck JM, Mermier CM, Beltz NM, et al. Physical fitness evaluation of career urban and wildland firefighters. J Occup Environ Med. 2020;62(7):e302–e307.
- Drew-Nord DC, Hong O, Froelicher ES, et al. Cardiovascular risk factors among career firefighters. AAOHN J. 2009;57(10):415–424.
- Eastlake AC, Knipper BS, He X, et al. Lifestyle and safety practices of firefighters and their relation to cardiovascular risk factors. Work. 2015 Jan 1;50(2):285–294.
- Webb HE, Weldy ML, Fabianke-Kadue EC, et al. Psychological stress during exercise: cardiorespiratory and hormonal responses. Eur J Appl Physiol. 2008 Dec;104(6):973–981.
- Webb HE, Garten RS, McMinn DR, et al. Stress hormones and vascular function in firefighters during concurrent challenges. Biol Psychol. 2011 Apr;87(1):152–160.
- Huang C-J, Webb HE, Garten RS, et al. Stress hormones and immunological responses to a dual challenge in professional firefighters. Int J Psychophysiol. 2010;75(3):312–318.
- Dobson M, Choi B, Schnall PL, et al. Exploring occupational and health behavioral causes of firefighter obesity: a qualitative study. Am J Ind Med. 2013;56(7):776–790.
- Poston WSC, Haddock CK, Jahnke SA, et al. The prevalence of overweight, obesity, and substandard fitness in a population-based firefighter cohort. J Occup Environ Med. 2011;53(3):266–273.
- Can SH, Hendy HM. Behavioral variables associated with obesity in police officers. Ind Health. 2014;52(3):240–247.
- Rona RJ, Sundin J, Wood P, et al. Agreement between body mass index, waist circumference and skin-fold thickness in the United Kingdom Army. Ann Hum Biol. 2011 May;38(3):257–264.
- Alasagheirin M. H., Clark M. K., Ramey S. L., Grueskin, E. F., et al. Body mass index misclassification of obesity among community police officers. American Association of Occupational Health Nurses. 2011. 59(11): 469–475. doi:10.3928/08910162-20111017-01.
- Jitnarin N, Poston WSC, Haddock CK, et al. Accuracy of body mass index-defined obesity status in US firefighters. Saf Health Work. 2014;5(3):161–164.
- Ramey SL, Downing NR, Franke WD. Milwaukee police department retirees: cardiovascular disease risk and morbidity among aging law enforcement officers. Aaohn J. 2009 Nov;57(11):448–453.
- Kales SN, Tsismenakis AJ, Zhang C, et al. Blood pressure in firefighters, police officers, and other emergency responders. Am J Hypertens. 2009 Jan;22(1):11–20.
- Soteriades ES, Smith DL, Tsismenakis AJ, et al. Cardiovascular disease in US firefighters: a systematic review. Cardiol Rev. 2011 Jul-Aug;19(4):202–215.
- Fatalities among volunteer and career firefighters–United States, 1994-2004. MMWR Morb Mortal Wkly Rep. 2006 Apr 28;55(16):453–455.
- Tsismenakis AJ, Christophi CA, Burress JW, et al. The obesity epidemic and future emergency responders. Obesity (Silver Spring). 2009 Aug;17(8):1648–1650.
- Yoo HL, Franke WD. Prevalence of cardiovascular disease risk factors in volunteer firefighters. J Occup Environ Med. 2009 Aug;51(8):958–962.
- Mifflin MD, St Jeor ST, Hill LA, et al. A new predictive equation for resting energy expenditure in healthy individuals. Am J Clin Nutr. 1990 Feb;51(2):241–247.
- Roza AM, Shizgal HM. The Harris benedict equation reevaluated: resting energy requirements and the body cell mass. Am J Clin Nutr. 1984 Jul;40(1):168–182.
- Harris JA, Benedict FG. A biometric study of human basal metabolism. Proc Natl Acad Sci U S A. 1918;4(12):370–373.
- Ainsworth BE, Haskell WL, Herrmann SD, et al. Compendium of physical activities: a second update of codes and MET values. Med Sci Sports Exerc. 2011 Aug;438:1575–1581. 2011.
- Kreider RB, Wilborn CD, Taylor L, et al. Exercise & sport nutrition review: research & recommendations. J Int Soc Sports Nutr. 2010;7(1):7.
- Lupton, J. R., Brooks, J., Butte, N. F., Caballero, B., Flatt, J. P., Fried, S. K. Dietary reference intakes for energy, carbohydrate, fiber, fat, fatty acids, cholesterol, protein, and amino acids, 5, 589–768: 2002. National Academy Press: Washington, DC, USA.
- Popkin BM, D’Anci KE, Rosenberg IH. Water, hydration, and health. Nutr Rev. 2010;68(8):439–458.
- Press Na, editor institute of medicine dietary reference intake for water. Washington DC; 2005, p. 75–134.
- Peacock OJ, Stokes K, Thompson D. Initial hydration status, fluid balance, and psychological affect during recreational exercise in adults. J Sports Sci. 2011 Jun;29(9):897–904.
- Cronin C, O’Neal E, Simpson J, et al. Natural training hydration status, sweat rates, and perception of sweat losses during crossfit training. J Sports Sci. 2016;9:576–586.
- O’Neal E, Boy T, Davis B, et al. Post-exercise sweat loss estimation accuracy of athletes and physically active adults: a review. Sports (Basel). 2020 Aug 11;8(8).
- Armstrong LE, Maresh CM, Castellani JW, et al. Urinary indices of hydration status. Int J Sport Nutr. 1994 Sep;4(3):265–279.
- Armstrong LE, Soto JA, Hacker FT Jr., et al. Urinary indices during dehydration, exercise, and rehydration. Int J Sport Nutr. 1998 Dec;8(4):345–355.
- Thomas DT, Erdman KA, Burke LM. Position of the academy of nutrition and dietetics, dietitians of Canada, and the American college of sports medicine: nutrition and athletic performance. J Acad Nutr Diet. 2016 Mar;116(3):501–528.
- Smith DL, Arena L, DeBlois JP, et al. Effect of base layer materials on physiological and perceptual responses to exercise in personal protective equipment. Appl Ergon. 2014 May;45(3):428–436.
- Smolander J, Louhevaara V, Tuomi T, et al. Cardiorespiratory and thermal effects of wearing gas protective clothing. Int Arch Occup Environ Health. 1984;54(3):261–270.
- Larsen B, Snow R, Williams-Bell M, et al. Simulated firefighting task performance and physiology under very hot conditions [original research]. Front Physiol. 2015;6(322).
- Cheung SS, McLellan TM, Tenaglia S. The thermophysiology of uncompensable heat stress physiological manipulations and individual characteristics. Sports Med. 2000May;29(5):329–359.
- Cheuvront SN, Kenefick RW. Dehydration: physiology, assessment, and performance effects. Compr Physiol. 2014 Jan;4(1):257–285.
- Baker LB, Conroy DE, Kenney WL. Dehydration impairs vigilance-related attention in male basketball players. Med Sci Sports Exerc. 2007 Jun;39(6):976–983.
- Cheuvront SN, Haymes EM. Thermoregulation and marathon running: biological and environmental influences. Sports Med. 2001;31(10):743–762.
- Casa DJ, Armstrong LE, Hillman SK, et al. National athletic trainers’ association position statement: fluid replacement for athletes. J Athl Train. 2000 Apr;35(2):212–224.
- Wendt D, van Loon LJ, Lichtenbelt WD. Thermoregulation during exercise in the heat: strategies for maintaining health and performance. Sports Med. 2007;37(8):669–682.
- González-Alonso J. Human thermoregulation and the cardiovascular system. Exp Physiol. 2012;97(3):340–346.
- González-Alonso J, Crandall CG, Johnson JM. The cardiovascular challenge of exercising in the heat. J Physiol. 2008;586(1):45–53.
- Romet TT, Frim J. Physiological responses to fire fighting activities. Eur J Appl Physiol Occup Physiol. 1987;56(6):633–638.
- Aragon AA, Schoenfeld BJ, Wildman R, et al. International society of sports nutrition position stand: diets and body composition. J Int Soc Sports Nutr. 2017;14(1):16.
- Donnelly JE, Blair SN, Jakicic JM, et al. American college of sports medicine position stand. appropriate physical activity intervention strategies for weight loss and prevention of weight regain for adults. Med Sci Sports Exerc. 2009 Feb;41(2):459–471.
- Liguori G, Medicine Aco S. ACSM’s guidelines for exercise testing and prescription. Philadelphia, PA: Lippincott Williams & Wilkins; 2020.
- Yang J, Farioli A, Korre M, et al. Dietary preferences and nutritional information needs among career firefighters in the United States. Glob Adv Health Med. 2015;4(4):16–23.
- Sofi F, Cesari F, Abbate R, et al. Adherence to Mediterranean diet and health status: meta-analysis. Bmj. 2008 Sep 11;337(sep11 2):a1344.
- Mertens E, Mullie P, Deforche B, et al. Cross-sectional study on the relationship between the Mediterranean diet score and blood lipids. Nutr J. 2014;13(1):88.
- Yang J, Farioli A, Korre M, et al. Modified Mediterranean diet score and cardiovascular risk in a North American working population. PLOS ONE. 2014;9(2):e87539.
- Patterson RE, Sears DD. Metabolic effects of intermittent fasting. Annu Rev Nutr. 2017;37(1):371–393.
- Ravussin E, Beyl RA, Poggiogalle E, et al. Early time-restricted feeding reduces appetite and increases fat oxidation but does not affect energy expenditure in humans. Obesity (Silver Spring). 2019 Aug;27(8):1244–1254.
- Peeke PM, Greenway FL, Billes SK, et al. Effect of time restricted eating on body weight and fasting glucose in participants with obesity: results of a randomized, controlled, virtual clinical trial. Nutr Diabetes. 2021;11(1):6.
- McAllister MJ, Pigg BL, Renteria LI, et al. Time-restricted feeding improves markers of cardiometabolic health in physically active college-age men: a 4-week randomized pre-post pilot study. Nutr Res. 2020 Mar;75:32–43.
- Waldman HS, Renteria LI, McAllister MJ. Time-restricted feeding for the prevention of cardiometabolic diseases in high-stress occupations: a mechanistic review. Nutr Rev. 2020 Jun 1; 78(6):459–464.
- McAllister MJ, Gonzalez AE, Waldman HS. Impact of time restricted feeding on markers of cardiometabolic health and oxidative stress in resistance-trained Firefighters. J Strength Cond Res. 2020 Oct 30;
- Moro T, Tinsley G, Longo G, et al. Time-restricted eating effects on performance, immune function, and body composition in elite cyclists: a randomized controlled trial. J Int Soc Sports Nutr. 2020;17(1):65.
- Moro T, Tinsley G, Bianco A, et al. Effects of eight weeks of time-restricted feeding (16/8) on basal metabolism, maximal strength, body composition, inflammation, and cardiovascular risk factors in resistance-trained males. J Transl Med. 2016;14(1):290.
- Gonzalez AE, Waldman HS, Abel MG, et al. Impact of time restricted feeding on fitness variables in professional resistance trained firefighters. J Occup Environ Med. 2021;63(4):343–349.
- McAllister MJ, Gonzalez AE, Waldman HS. Time restricted feeding reduces inflammation and cortisol response to a firegrounds test in professional firefighters. J Occup Environ Med. 2021 May 1;63(5):441–447.
- Waldman H, Smith J, Lamberth J, et al. A 28-day carbohydrate-restricted diet improves markers of cardiometabolic health and performance in professional firefighters. J Strength Cond Res. 2019;33(12):1.
- Waldman HS, Smith JW, Lamberth J, et al. A 28-day carbohydrate-restricted diet improves markers of cardiovascular disease in professional firefighters. J Strength Cond Res. 2020 Oct;34(10):2785–2792.
- Aragon AA, Schoenfeld BJ, Wildman R, et al. International society of sports nutrition position stand: diets and body composition. J Int Soc Sports Nutr. 2017;14(1):16.
- Mansoor N, Vinknes KJ, Veierød MB, et al. Effects of low-carbohydrate diets v. low-fat diets on body weight and cardiovascular risk factors: a meta-analysis of randomised controlled trials. Br J Nutr. 2016 Feb 14;115(3):466–479.
- Hashimoto Y, Fukuda T, Oyabu C, et al. Impact of low-carbohydrate diet on body composition: meta-analysis of randomized controlled studies. Obes Rev. 2016 Jun;17(6):499–509.
- Kantor ED, Rehm CD, Du M, et al. Trends in dietary supplement use among us adults from 1999-2012. JAMA. 2016;316(14):1464–1474.
- Guest NS, VanDusseldorp TA, Nelson MT, et al. International society of sports nutrition position stand: caffeine and exercise performance. J Int Soc Sports Nutr. 2021 Jan 2;18(1):1.
- Wassell SD, Edwards ES, Saunders MJ, et al. Effect of caffeine on the hemostatic response to firefighting drills. J Caff Aden Resh. 2020;10(3):117–123.
- Kellawan JM, Stuart-Hill LA, Petersen SR. The effects of caffeine during exercise in fire protective ensemble. Ergonomics. 2009 Nov;52(11):1445–1454.
- Menon VP, Sudheer AR. Antioxidant and anti-inflammatory properties of curcumin. Adv Exp Med Biol. 2007;595:105–125.
- Ambati RR, Phang SM, Ravi S, et al. Astaxanthin: sources, extraction, stability, biological activities and its commercial applications–a review. Mar Drugs. 2014;12(1):128–152.
- Cao Y, Yang L, Qiao X, et al. Dietary astaxanthin: an excellent carotenoid with multiple health benefits. Crit Rev Food Sci Nutr. 2021;28:1–27.
- Yoshida H, Yanai H, Ito K, et al. Administration of natural astaxanthin increases serum HDL-cholesterol and adiponectin in subjects with mild hyperlipidemia. Atherosclerosis. 2010 Apr;209(2):520–523.
- Fassett RG, Coombes JS. Astaxanthin in cardiovascular health and disease. Molecules. 2012 Feb 20;17(2):2030–2048.
- K-c C, S-c C, P-c C. Astaxanthin attenuated thrombotic risk factors in type 2 diabetic patients. J Funct Foods. 2019 ;53:22–27.
- Tanabe Y, Maeda S, Akazawa N, et al. Attenuation of indirect markers of eccentric exercise-induced muscle damage by curcumin. Eur J Appl Physiol. 2015 Sep;115(9):1949–1957.
- Choi Y, Tanabe Y, Akazawa N, et al. Curcumin supplementation attenuates the decrease in endothelial function following eccentric exercise. J Exerc Nutrition Biochem. 2019 Jun 30;23(2):7–12.
- Nakhostin-Roohi B, Nasirvand Moradlou A, Mahmoodi Hamidabad S, et al. The effect of curcumin supplementation on selected markers of Delayed Onset Muscle Soreness (DOMS). Ann App Spo Sc. 2016;4(2):25–31.
- Mallard AR, Briskey D, Richards BA, et al. Curcumin improves delayed onset muscle soreness and postexercise lactate accumulation. J Diet Suppl. 2021;18(5):531–542.
- McAllister MJ, Holland AM, Chander H, et al. Impact of ketone salt containing supplement on cardiorespiratory and oxidative stress response in firefighters exercising in personal protective equipment [research article]. Asian J Sports Med. 2019;10(1):e82404.
- McAllister MJ, Holland AM, Chander H, et al. Impact of ketone salt containing supplement on cardiorespiratory and oxidative stress response in firefighters exercising in personal protective equipment [research article]. Asian J Sports Med. 2019;10(1):e82404.