ABSTRACT
Background
The aim of this study was to explore the effects of a low dose (LD) of 0.625 mg and a high dose (HD) of 2.5 mg of phenylcapsaicin (PC) on full squat (SQ) performance, active muscle (RPE-AM) and overall body (RPE-OB) ratings of perceived exertion, muscle damage, protein breakdown, metabolic response, and 24-h recovery in comparison to placebo (PLA).
Method
Twenty-five resistance-trained males (age = 21.00 ± 2.15 years, SQ 1-repetition maximum [1RM] normalized = 1.66 ± 0.22 kg) were enrolled in this randomized, triple-blinded, placebo-controlled, crossover trial. Participants completed 2 weekly sessions per condition (LD, HD, and PLA). The first session consisted of pre-blood testing of lactate, urea, and aspartate aminotransferases (AST) and 2 SQ repetitions with 60% 1RM followed by the resistance exercise protocol, which consisted of SQ sets of 3 × 8 × 70% 1RM monitoring lifting velocity. RPE-OB and RPE-AM were assessed after each set. After the first session, 2 SQ repetitions with 60% 1RM were performed, and blood lactate and urea posttests were collected. After 24 h, AST posttest and 1 × 2 × 60% 1RM were determined as biochemical and mechanical fatigue outcomes.
Results
HD reported significant differences for RPE-AM, AST, and SQ performance compared to LD and PLA. Post-hoc analyses revealed that HD attained faster velocities in SQ than LD (p = 0.008). HD induced a lower RPE-AM when compared with LD (p = 0.02) and PLA (p = 0.004). PLA resulted in higher AST concentrations at 24-h post than HD (p = 0.02). No significant differences were observed for the rest of the comparisons.
Conclusions
This study suggests that PC may favorably influence SQ performance, RPE-AM, and muscle damage compared to PLA. However, HD exhibited most of the biochemical and mechanical anti-fatigue effects instead of LD.
1. Introduction
Sports supplements are popular ergogenic aids among athletes aiming to improve their sport performance [Citation1,Citation2]. Recently, sports nutrition research has focused on the discovery of new active compounds which may be able to improve high-intensity training in different modalities [Citation3,Citation4]. Within this context, capsaicinoids have emerged as a plausible ergogenic aid for strength conditioning and high-intensity sports [Citation5,Citation6]. Capsaicinoids are a group of compounds naturally found in spicy chili peppers which are characterized by their vanilloid structure [Citation7]. Capsaicin (8-methyl-N-vanillyl-trans-6-nonenamide), which is found in the placental tissue of Capsicum fruit, has emerged as the primary and most abundant capsaicinoid with therapeutic and physical performance relevance [Citation6,Citation8]. In this sense, capsaicin has exhibited improvements on several pathophysiological conditions, such as chronic musculoskeletal and neuropathic pain, gastrointestinal disruptions (e.g. gastroduodenal mucosal injury), and metabolic disorders (e.g. overweight) [Citation9,Citation10]. On the other hand, capsaicin seems to enhance sport performance by reducing ratings of perceived exertion and perceived discomfort while improving mechanical performance (e.g. total volume load) [Citation5,Citation11].
The aforementioned physiological effects of capsaicin have been associated with the transient receptor vanilloid 1 (TRPV1) [Citation12]. TRPV1 content is especially high in afferents III and IV nerve fibers, which are linked to peripheral and central fatigue during high-intensity tasks [Citation13–15]. The feedback from afferents III and IV could directly or indirectly reduce motoneuron firing and motor unit recruitment [Citation14,Citation15]. In this sense, the TRPV1 content of these nerve fibers (mainly in group IV afferents) has been proposed as a target for enhancing physical performance by using capsaicin [Citation5,Citation6,Citation16]. Thus, as a TRPV1 agonist, capsaicin could improve muscle contraction by increasing the perceived heat analgesia and the release of calcium from the sarcoplasmic reticulum, which are linked to motor units function [Citation5].
To date, capsaicinoid supplementation has mainly been tested on sports performance with encapsulated capsaicin formulations [Citation5]. Purified acute (i.e. 45 min before exercise) oral capsaicin supplementation has demonstrated an ergogenic effect in upper- and lower-limb resistance training tasks [Citation5,Citation17]. For instance, de Freitas et al. [Citation6] in a double-blind, randomized placebo-controlled trial found that acute 12 mg of capsaicin significantly increased the number of repetitions until failure, increased the total weight lifted, and reduced overall body RPE (RPE-OB) in squat with a not-volume-matched design. By contrast, a lower dose of purified capsaicin (i.e. 1.2 mg in gummy format) did not reach significant differences in summed torque or fatigue index of an isokinetic knee extension exercise in a randomized, double-blinded, controlled trial [Citation18]. Within this context, although velocity-based training has been proposed as an objective approach to monitoring resistance training load by reflecting the state of the neuromuscular system to produce force against a load [Citation19,Citation20], only one study has examined the effects of capsaicin on velocity variables in resistance training [Citation17]. Furthermore, research approaching capsaicinoids effects on velocity-derived outcomes has only been reported for upper-limb exercises but not for any lower-limb activity (e.g. squat) [Citation5,Citation17].
Although capsaicin TRPV1 activity may be linked to its spiciness [Citation8,Citation9], encapsulated capsaicin has not gotten a direct pungent taste. However, previous research has reported intestinal discomfort after high-dose (HD) oral encapsulated capsaicin supplementation (25.8 mg) [Citation21], which suggests that capsaicin may be irritating, in spite of the vehicle of administration used. Recently, a new synthetic analog called phenylcapsaicin (PC) has emerged as an alternative to traditional oral purified capsaicin supplementation [Citation22]. PC is a microencapsulation of 98% of PC and 1–1.5% of cellulose and lipidic excipients as primary vehicles [Citation22]. Through hepatic glucuronidation, PC is fast presented in tissues such as the small intestine, stomach, and liver after 0.5 h of ingestion [Citation22]. Therefore, it is supposed that microencapsulation might entail a lower pungency power, less digestive system mucosa irritation, and potentially a higher bioavailability [Citation10,Citation22]. Therefore, given that purified capsaicin ergogenic dose oscillates around 12 mg [Citation6], PC might exert positive ergogenic effects with lower doses (i.e. less than 2.5 mg). This reasoning may be aligned with the current European Food Safety Authority (EFSA) PC permitted limits for dietary supplements (i.e. 2.5 mg) [Citation22].
On the other hand, acute muscle damage, protein breakdown, and recovery variables have not been assessed in response to capsaicin supplementation yet. As intense exercise may increase metabolic, biochemical, and neuromuscular fatigue in acute and short-term ways [Citation11,Citation23], exploring the biochemical, perceptual, and neuromuscular effects of capsaicin on these topics may also be relevant for researchers and practitioners.
Therefore, the aim of this study was to explore the effects of low dose (LD) and HD of PC on lower-limb performance in the full squat (SQ) exercise under velocity-based control, metabolic responses to exercise, acute biochemical muscle damage, protein breakdown, RPE, and perceived recovery in resistance-trained men. These assessments were approached under a randomized, triple-blinded, placebo-controlled, crossover design. It was hypothesized that PC may exert a positive impact in a dose–response way on velocity outcomes and RPE. However, a concomitant increment of perceived fatigue, protein breakdown, and muscle damage would have appeared after PC supplementation due to the increase in physical performance. It was expected that impairments in performance as well as muscle and protein damage were higher in the HD condition.
2. Materials and methods
2.1. Participants
Twenty-five healthy men (age = 21.0 ± 2.2 years, body mass = 76.5 ± 9.5 kg, height = 176.4 ± 7.5 cm, and SQ 1-repetition maximum [1RM] normalized to body mass = 1.66 ± 0.22) enrolled voluntarily to this study. Of the total sample, two participants dropped out of the study, one due to causes not related to the study, and the other after the placebo session. All participants were resistance-trained men with at least 2 years of experience (experience = 3.61 ± 1.43 years). Exclusion criteria comprised cardiovascular, neurological, physical, and/or metabolic disorders that may disturb primary outcomes.
One week before the beginning of the study, SQ strength and anthropometric measurements (i.e. body mass and height) were tested for all participants. Participants were asked not to consume alcohol, caffeine, or other ergogenic aids. Besides, they could not to perform intense exercise or modify their macronutrients distribution, calorie intake, and food selection 24 and 48 h before each session. The experimental protocol was explained before the informed consent, and sample collection agreement was signed prior to the first experimental session. The study protocol adhered to and respected the tenets of the Declaration of Helsinki and was approved by the Institutional Review Board of Pablo of Olavide University (Code: 0513-N-22).
2.2. Experimental design
A randomized, triple-blinded, placebo-controlled crossover trial was used to explore the effects of PC on resistance training performance, muscle damage, and metabolic responses. Participants attended the laboratory twice per week for a total study duration of 3 weeks. Each week of the study consisted of a main experimental session and a follow-up session. For each condition, six capillary blood extractions, a warm-up, an SQ testing protocol, and a 24-h recovery and muscle damage follow-up session were performed.
The order of the interventions was randomized for all participants by an external researcher in a balanced way before the beginning of the study in order to reduce training bias risk. The Research Randomizer website (www.randomizer.org) was used. All procedures were completed at the same time of the day and under stable environmental conditions (22ºC–24ºC and 55% humidity) for each participant. The overall design of the study is depicted in .
2.3. Supplementation procedures
To ensure blinding, supplements and placebo (Life Pro Nutrition industries, Madrid, Spain) were encapsulated and packaged with numbered labels by an independent researcher (i.e. not involved in the study). Packages and capsules were indistinguishable in appearance, smell, and taste, and their content was only revealed after an independent researcher performed the statistical analyses.
Oral ingestion of each condition was performed 45 min before the first physical testing session of each week, according to previous research [Citation5,Citation22]. Participants were encouraged to freely select one capsule of the assigned numbered condition under researcher supervision. Participants were only allowed to consume the selected capsule with water. Capsules contained a 0.625 mg LD of PC, a 2.5 mg HD of PC (Axivite, Malmö, Sweden), and a PLA composed of maltodextrin and excipients. According to the EFSA, both PC doses are considered in the safety range proposed by its expert panel judgment [Citation22].
2.4. Blood testing
Blood samples were extracted and analyzed at four different times each week. Before the beginning of each first session, baseline capillary lactate, blood urea, and aspartate aminotransferase (AST) samples were collected from the index fingertip of each participant. The timeline for each biomarker was chosen due to suitability and reproducibility according to previous data [Citation23,Citation24]. Capillary blood extractions were conducted with a sterilized lancet after cleaning and drying the fingertip of participants before each attempt. Lactate Pro 2 LT-1730 (Arkray, Kyoto, Japan) was used for lactate measurements as it has been shown previously to be reliable throughout the physiological range of 1.0–18.00 mmol·l−1 [Citation25]. Posttest lactate was approached 90 s after the last SQ set as a metabolic indicator of the exercise intensity [Citation26]. Urea and AST were tested with 28.5–31.5 µl blood samples using automatic reflectance photometry (Reflotron, Roche, Boehringer Mannheim, Germany) [Citation27,Citation28] as whole-body protein breakdown and muscle damage measurements, respectively. For the determination of urea and AST, heparinized capillary tubes, pipettes, and the manufacturer’s reagent strips were used immediately after each extraction [Citation23]. According to previous research, posttests extractions and analyses were performed 40 min [Citation23] after the last SQ set for urea as a purine cycle indicator [Citation29] and before starting the 24-h follow-up session for AST [Citation24] ().
2.5. Resistance training protocol
2.5.1. Progressive loading test
Before the beginning of the study, each participant undertook an initial test with increasing loads for the individual determination of the 1RM and the load–velocity relationship in the SQ exercise [Citation30]. For this purpose, a smith machine with no counterweight mechanism was used (Multipower Fitness Line, Peroga, Murcia, Spain). The mean propulsive velocity (MPV) was directly measured for each repetition with a linear velocity transducer (T-Force System, Ergotech, Murcia, Spain) attached perpendicularly to the barbell [Citation19].
Concerning the progressive loading test, the initial load was set at 30 kg, and it was progressively increased in 10 kg until the mean propulsive velocity (MPV) was 0.50 m·s−1. Then, the load was increased with smaller increments (2.5–5.0 kg) until the repetition could not be completed. Three repetitions were completed for light loads (≥1.00 m·s−1), two for medium loads (1.00–0.80 m·s−1), and one for the heaviest loads (≤0.80 m·s−1). Rest periods were 3 min for light and medium loads and 5 min for heavy loads. Only the best repetition (i.e. highest MPV) with each load was considered for load–velocity relationship calculation [Citation31].
The SQ was performed with subjects starting from the upright position with the knees and hips fully extended, parallel feet and stance approximately shoulder-width apart, and the barbell resting across the back at the level of the acromion. Each subject descended in a continuous motion until the top of the thighs was below the horizontal plane (~35°–40° knee flexion), then immediately reversed motion, and raised back to the upright position. Unlike the eccentric phase that was performed at a controlled mean velocity (~0.50–0.65 m·s−1), participants were encouraged to always perform the concentric phase of the SQ at maximal intended velocity [Citation32].
2.5.2. Warm-up
The standardized warm-up of the 2 weekly SQ sessions consisted of (I) 5 min of running at 9 km·h−1, (II) three sets of 10 repetitions of bodyweight squat, (III) three progressive countermovement jumps, and (IV) 3 sets of 2 SQ repetitions with the 40%, 50%, and 60% of 1RM resting 2 min between sets. These warm-up intensities were chosen based on previous research of the field [Citation31] in order to progressively prepare subjects but not to fatigue them.
2.5.3. Full squat protocol
The execution technique has been described in the progressive loading test section. The SQ protocol consisted of three sets of eight repetitions with 70% 1RM with a 2-min rest period between sets. Therefore, training volume was matched for all sessions. Thus, velocity loss could be compared for the same training performed after different interventions.
According to warm-up sets velocities and the individual load–velocity relationship, 70% 1RM load was daily established for each participant. This load was chosen because this 1RM percentage may involve a submaximal non-extenuating (i.e. without reaching muscle failure) high effort for the selected repetitions [Citation20]. Finally, as MPV and, consequently, the percentage of velocity loss (VL) are indicators of neuromuscular fatigue [Citation20], two repetitions with 60% 1RM load were performed 3 min and 24 h after the last set of the 3 × 8 protocol. Velocity values were treated as the fastest, mean, and slowest obtained for the three sets. The percentage of mean and maximal VL was calculated in agreement with previous research [Citation33].
2.6. Rating of perceived exertion and perceived recovery status assessment
Subjective fatigue and recovery assessment was conducted using the perceived recovery status (PRS), RPE-OB, and active muscle RPE (RPE-AM) scales. PRS, RPE-AM, and RPE-OB are subjective recovery and fatigue status pictographs where cutoff points ranged from 0 to 10. Although subjects were familiarized with both pictographs before the start of the study, both pictographs were presented during the previous session to the study start. In PRS, the perceived recovery is set between “very poorly recovered/extremely tired” (value 0) and “very well recovered/highly energetic” (value 10) [Citation34,Citation35]. PRS was explained again and evaluated before the start of the post-24-h follow-up session for each condition.
Fatigue was evaluated using RPE-AM and RPE-OB immediately after each 3 × 8 set [Citation36]. RPE scales were explained after the 60% 1RM load and before the 3 × 8 protocol for each condition. The maximum perceived exertion was set on the value 10, which corresponds to reaching exhaustion (i.e. last set rating of exertion of the progressive loading test), and the basal intensity is represented with the value 0. RPE-AM was set up as the locally perceived exertion of the quadriceps and RPE-OB as the traditional general perceived exertion of the whole body [Citation36]. Both validated scales were printed, and participants were able to visualize each one when they required them.
2.7. Sample size calculation
Sample size calculation was performed using the G* POWER software (Heinrich-Heine-Universität Düsseldorf, Germany) with an alpha of 0.05, a statistical power of 0.80, and an effect size of 0.60 based on previous resistance training performance (i.e. total mass lifted) in the previous literature [Citation6]. Accordingly, at least 21 participants were required for this study. Plausible drop-out rate was set on 15%, and 25 subjects were recruited [Citation37,Citation38].
2.8. Statistical analysis
Data are presented as means and standard deviations (Mean ± SD). The normal distribution of the variables (Shapiro–Wilk test) and the homogeneity of the variances (Levene’s test) were tested for each variable (p > 0.05). A two-way repeated measures analysis of variance (ANOVA) (condition × time) was used to explore the effect of the interventions (LD, HD, and PLA) along the time on the magnitude of each dependent biochemical and perceptual variable. Bonferroni post-hoc comparison was performed when ANOVA significance was reached. A one-way repeated measures ANOVA was used to compare velocity analyses. The Greenhouse–Geisser correction was applied when Mauchly’s sphericity test was significant (p ≤ 0.05). The Cohen’s d effect size (ES) with 95% confidence intervals was calculated to evaluate the magnitude of the differences using the following scale: negligible (<0.20), small (0.20–0.49), moderate (0.50–0.79), and large (≥0.80) [Citation39]. If non-parametric data were examined, Friedman and post-hoc Wilcoxon were used instead. Statistical analyses were performed using the software package SPSS (IBM SPSS version 25.0, Chicago, IL, USA). Statistical significance was set at p ≤ 0.05.
3. Results
3.1. Blood testing
Two-way repeated measures ANOVAs reported significant differences of lactate and urea concentrations for time (pre-post comparisons) (F = 269.62, p < 0.001) but not for condition (F = 1.49, p = 0.23) or condition × time interaction (F = 0.94, p = 0.34) (). For AST, Friedman's test revealed no significant differences between conditions in pre-values (p = 0.77), showing similar baseline levels. However, significant differences between the conditions were found for AST post-values (p = 0.03, ). Post-hoc Wilcoxon test revealed significantly higher post-levels of AST for PLA compared to HD (p = 0.02).
Table 1. Metabolic response to the different conditions of phenylcapsaicin supplementation.
3.2. Resistance training protocol
One-way repeated measures ANOVAs of movement velocity outcomes revealed significant effects for mean velocity and maximal VL (p range ≤ 0.001 to 0.05, ). Bonferroni post-hoc comparisons revealed significant differences between HD and LD for maximal VL (p = 0.008), and it was almost achieved between HD and PLA for the mean velocity variable (p = 0.06). The magnitude of the differences between the different conditions ranged from negligible to large ().
Table 2. Mechanical characteristics of the training session carried out under different conditions of phenylcapsaicin supplementation.
Table 3. Cohen’s d effect size (ES) with 95% confidence intervals (CI) comparing mechanical outcomes between conditions.
3.3. Rating of perceived exertion and perceived recovery status assessments
Two-way repeated measures ANOVAs for RPE-OB reported significant differences for time (F = 49.00, p < 0.001) but not for condition (F = 2.77, p = 0.07) or condition × time interaction (F = 1.339, p = 0.26) (). However, Bonferroni post-hoc analyses revealed no significant differences. For RPE-AM, both condition (F = 9.19, p < 0.001) and time (F = 36.154, p < 0.001) reached significant differences but not condition × time interaction (F = 0.553, p = 0.70). Bonferroni post-hoc analyses showed significant differences for all time comparisons (p range ≤ 0.001 to 0.002) and for comparisons between PLA and HD (p = 0.004) and between HD and LD (p = 0.02) (). On the other hand, one-way repeated measures ANOVAs found no significant differences between conditions for PRS (F = 0.698, p = 0.46, ).
Figure 2. Individual (points) and mean (bars) values of: (a) RPE-OB, overall body rating of perceived exertion; (b) RPE-AM, active muscle rating of perceived exertion; (c) PRS, perceived recovery status for the different supplementation conditions (PLA, HD, and LD). PLA, Placebo; HD, High dose; LD, Low dose. (* p ANOVA≤0.05; # p Bonferroni≤0.05).
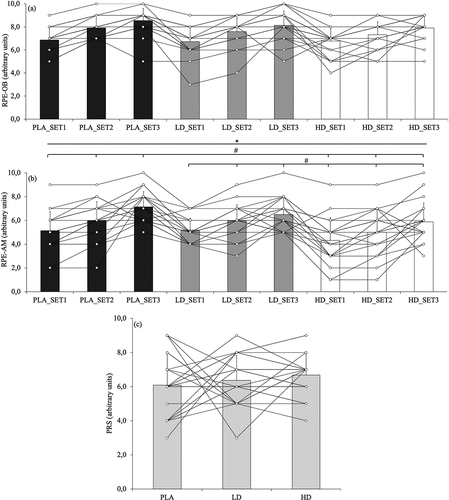
4. Discussion
This is the first study where the impact of a capsaicinoid has been evaluated on several topics, such as muscle damage, protein breakdown, recovery, peripheral perceived exertion, and velocity-performance variables with an acute 24-h design in strength-trained men. The main finding of this study was that although HD reduced RPE-AM and enhanced mechanical performance, it also exhibited lower muscle damage in comparison to PLA and LD. The main HD effects were documented through reductions in maximal VL and eliciting a positive trend in the velocity of the slowest repetitions compared to LD and PLA. Collectively, contrary to the initial hypothesis, the ergogenic effects of PC on performance variables were only verified for HD. In addition, a significant dose–response relationship for LD and HD was not fulfilled for any of the particular outcomes. On the other hand, contrary to our initial hypothesis, HD was effective in reducing muscle damage. Therefore, the results of the present study confirm a plausible ergogenic effect of PC on strength training and performance, but an HD seems to be necessary.
Regarding metabolic blood testing, previous literature has described that the involvement of major muscle mass areas (e.g. lower-limb exercise) and the execution time are key factors in lactate response [Citation40]. In this sense, a higher velocity loss is linearly correlated with an increase in lactate levels after SQ exercise [Citation20]. Accordingly, resistance training stimulus applied in the present study was effective inducing a lactate response between pre- and post-exercise states. These results agree with the only previous study where lactate was assessed after capsaicin supplementation [Citation6]. Within this context, in our study, post-exercise lactate levels tended to be lower in HD conditions compared to PLA and LD. Preclinical data have shown that lactate is a potent endogenous inhibitor of TRPV1 activity [Citation41]. For this reason, PC may have modulated sarcoplasmic calcium efflux channels lowering lactate levels [Citation41]. This finding agrees with a previous study [Citation6], in which although subjects significantly performed more repetitions after capsaicin ingestion compared to placebo, non-significant lower lactate levels were reported for capsaicin condition. This reduction on lactate kinetics after oral capsaicin administration has also been reported in other high-intensity exercise modalities, such as running time trial [Citation42].
Concerning protein breakdown, previous literature has observed an accelerated catabolic effect on purine nucleotides in skeletal muscle after strenuous exercise [Citation29,Citation43]. As a waste product of ammonium, urea levels are linked to exercise intensity (i.e. closeness to muscle failure), which can be assessed through velocity loss [Citation20,Citation29]. Previous research has fixed purine breakdown threshold on 35% of VL in the SQ exercise [Citation20]. In the present study, all protocols induced moderate increases in urea levels following exercise. However, none of the protocols achieved this cutoff threshold. Nevertheless, although significant differences were not reported between conditions, the absolute increase tended to be lower in HD, which reported a significantly lower maximal VL. In agreement with protein breakdown, strenuous exercise also raises inflammation and oxidative stress, which can be observed in different muscle damage biomarkers [Citation44,Citation45]. AST is a liver and musculoskeletal enzyme which reacts 24 h after demanding exercise [Citation24,Citation45,Citation46]. In this regard, HD exhibited lower levels of AST than PLA after 24 h. This finding may be linked to the lower mechanical stress suffered during the HD conditions since HD obtained higher velocities and lower VL values. This mechanical hypothesis may be more intuitive instead of a direct “antioxidant” or recovery effect of PC due to its synthetic composition [Citation22].
Related to the SQ protocol, the present results agree with the previous literature where capsaicin enhanced performance when it was acutely ingested [Citation5,Citation6]. In the previous research, capsaicin (i.e. 12 mg) raised the total number of repetitions performed until failure in a 4 × 70% 1RM protocol [Citation6]. Within this context, as MPV is a reliable predictor of the number of repetitions [Citation47], the observed trend of higher MPV values in the last repetitions (i.e. the slowest repetitions) of HD might have implied an increase in the total repetitions until exhaustion if the volume had not been matched. Furthermore, as previous research elicited further benefits of capsaicin in the last set of higher-volume training protocols, plausible greater effects would have been reported by increasing the total number of sets [Citation3,Citation6]. Regarding the potential ergogenic mechanisms underlying capsaicin effects on SQ performance, according to previous research, they may be linked to an increase of calcium released by the sarcoplasmic reticulum, higher acetylcholine levels, and its analgesic effect [Citation5,Citation48]. As afferents III and IV fibers seem to contribute to the development of central fatigue at spinal and supraspinal levels of the central nervous system [Citation13,Citation49], this “desensitizer” agent may provide a higher tolerance to firing rate reductions during strenuous exercise. Thus, as muscle force is critically affected by the motor unit activity [Citation50], and MPV is a mechanical manifestation of muscle force [Citation20], the analgesic effect of PC may have played a retardant effect on neuromuscular fatigue improving MPV, especially at the last repetitions. Consequently, the ergogenic effect of PC on mechanical outcomes might be explained by a plausible reduction in neural fatigue [Citation5,Citation51].
Concerning perceived effort variables, previous research has reported RPE-OB reductions after 12 mg of acute capsaicin supplementation [Citation3,Citation6]. Although a positive trend was observed, our results did not support these RPE-OB reductions when PC, in HD or LD, was ingested in comparison to PLA. In this sense, most of the previous research protocols were performed until muscle failure where the plausible analgesic effect of capsaicinoids may be higher [Citation6,Citation48]. On the other hand, in the present study, local quadriceps RPE-AM was highly affected by HD in comparison to LD and PLA. Considering that the RPE can be modulated by changes in the neuronal circuitry of the brain [Citation52,Citation53] and afferent feedback from III and IV afferents may raise exercise-related discomfort [Citation11] and RPE [Citation16], this effect of PC on RPE-AM could be mediated by TRPV1 interaction. In addition, since HD but not LD produced significant effects on RPE-AM, peripheral analgesic effects of PC [Citation54] during exercise could be dose-dependent. This conjecture agrees with the only previous study where a LD of a regularly bioavailable capsaicin supplement was consumed before a resistance training protocol [Citation18]. In this study, fatigue index and isometric knee torque were not affected after 1.2 mg of capsaicin [Citation18]. On the other hand, although PC in HD exerted a positive effect on muscle damage and RPE-AM, PC effects did not produce any improvements in PRS. Hence, the possible analgesic effect of PC may only appear acutely. Intriguingly, as previous research [Citation5] hypothesized that capsaicin may increase injury risk due to its analgesic effect, in this study, only two participants dropped out, one for personal reason and the other during the placebo session.
Collectively, our findings suggest that a 2.5 mg dose of PC provides a plausible ergogenic effect on strength performance, muscle damage, and peripheral perceived exertion in comparison to PLA and a lower dose of 0.625 mg. This novel information is valuable because never before a capsaicinoid has been evaluated concerning muscle damage, protein breakdown, and peripheral fatigue. Finally, this study presents important strengths such as a triple-blinded, placebo-controlled crossover design, trained participant enrollment, and the use of velocity measures as performance indicators. However, some limitations should be addressed. First, electromyographical assessment may have completed the internal reliability of the peripheral effects of PC. Another possible limitation is that the study was only carried out in male athletes, for this reason, these results may not be extrapolated to other populations such as female athletes or untrained subjects. Furthermore, these results should be cautiously interpreted for other exercises and tasks not performed in a laboratory environment. Finally, as in this study only three sets of SQ in a single training session were performed, further research may evaluate the effects of phenylcapsaicin during longer workouts and chronic trials to verify whether or not these benefits are replicated in these conditions.
5. Conclusions
The results of the present study suggest that an HD (2.5 mg) of PC supplementation ingested 45 min before exercise may increase SQ performance and reduce muscle damage, as well as peripheral quadriceps perceived exertion in strength-trained subjects in comparison to an LD (0.625 mg) and PLA. Therefore, the ergogenic effect of PC may appear after a “dose” threshold is reached.
Publication statement
This manuscript has not been published elsewhere.
Authors´ contributions
The study and methodology were conceived using JCC, PJM, FPB, and CAF. PCD, JSV, IAI, CCC, PJM were involved in data collection and data treatment, writing was conducted by CAF and PJM, all authors reviewed critically the manuscript under JCC and FPB supervision.
Acknowledgments
We would like to thank all the participants involved in this study and Axichem for supporting us with scientific material. We also acknowledge AMG., MC, and Life Pro Nutrition for their involvement on the preparation of the blinded packages.
Disclosure statement
PJM and CAF are scientific advisors of a supplement brand (Life Pro Nutrition, Madrid, Spain).
Data availability statement
Datasets used in this study are available from the corresponding author under reasonable request.
Additional information
Funding
References
- MaughanRJ, BurkeLM, DvorakJ, et al. IOC consensus statement: dietary supplements and the high-performance athlete. Int J Sport Nutr Exerc Metab. 2018;28(2):22.
- Cano-GonzálezH, Jiménez-MartínezP, Baz-ValleE, et al. Nutritional and supplementation strategies of Spanish natural elite bodybuilders in pre-contest. Gazzetta Medica Italiana. 2022;181.
- de FreitasMC, CholewaJM, PanissaVLG, et al. Acute capsaicin supplementation improved resistance exercise performance performed after a high-intensity intermittent running in resistance-trained men. J Strength Cond Res [Internet]. 2019 Sep 16;36(1):130–207. cited 2021 Publish Ahead of Print. Available from. DOI:10.1519/JSC.0000000000003431
- WalterAA, HerdaTJ, RyanED, et al. Acute effects of a thermogenic nutritional supplement on cycling time to exhaustion and muscular strength in college-aged men. J Int Soc Sports Nutr. 2009;6(1):15. DOI:10.1186/1550-2783-6-15
- Jiménez-MartínezP, Ramírez-CampilloR, FlandezJ, et al. Effects of oral capsaicinoids and capsinoids supplementation on resistance and high intensity interval training: a systematic review of randomized controlled trials. J HumSportexer. 2022;18(2). DOI:10.14198/jhse.2023.182.09
- de FreitasMC, CholewaJM, FreireRV, et al. Acute capsaicin supplementation improves resistance training performance in trained men. J Strength Cond Conditioning Res. 2018;32(8):2227–2232. DOI:10.1519/JSC.0000000000002109
- LangY, KisakaH, SugiyamaR, et al. Functional loss of pAMT results in biosynthesis of capsinoids, capsaicinoid analogs, in capsicum annuum cv. CH-19 sweet. Plant J. 2009;59(6):953–961. DOI:10.1111/j.1365-313X.2009.03921.x
- SharmaSK, VijAS, SharmaM. Mechanisms and clinical uses of capsaicin. Eur J Pharmacol. 2013;720:55–62.
- BasithS, CuiM, HongS, et al. Harnessing the therapeutic potential of capsaicin and its analogues in pain and other diseases. Molecules. 2016;21(8):966.
- FramrozeZ. Placebo controlled study to investigate the relationship between lowering serum zonulin levels and improved body weight composition using a daily oral dose of phenylcapsaicin. Int J Clin Ski. 2022;16(7):251.
- TaylorJL, AmannM, DuchateauJ, et al. Neural contributions to muscle fatigue: from the brain to the muscle and back again. Med Sci Sports Exerc. 2016;48(11):2294–2306.
- de Mourae E Silva Viton E L, Cholewa Jason M, Francois Billaut, Ralf, Jäger, Marcelo C de, Freitas, Fabio S, Lira, Fabrício E, Rossi. Capsaicinoid and capsinoids as an ergogenic aid: a systematic review and the potential mechanisms involved. Int J Sports Physiol Perform. 2021;16:464–473.
- CollinsBW, PearceyGEP, BuckleNCM, et al. Neuromuscular fatigue during repeated sprint exercise: underlying physiology and methodological considerations. Appl Physiol Nutr Metab. 2018;43(11):1166–1175.
- Alix-FagesC, Del VecchioA, Baz-ValleE, et al. The role of the neural stimulus in regulating skeletal muscle hypertrophy. 2022. [[cited 2022 Mar 17]; Available from]. Internet Eur J Appl Physiol. 122(5):1111–1128. 10.1007/s00421-022-04906-6.
- Alix-FagesC, GrgicJ, Jiménez-MartínezP, et al. Effects of mental fatigue on strength endurance: a systematic review and meta-analysis. Motor Control. 2022;27(2):442–461.
- AmannM, BlainGM, ProctorLT, et al. Group III and IV muscle afferents contribute to ventilatory and cardiovascular response to rhythmic exercise in humans. J Appl Physiol. 2010;109(4):966–976.
- da SilvaBVC, MotaGR, MarocoloM, et al. Acute supplementation with capsaicin enhances upper-limb performance in male jiu-jitsu athletes. Sports. 2022;10(8):120.
- CrossBL, ParkerD, LanganSP, et al. Effect of a commercially available low-dose capsaicin supplement on knee extensor contractile function. Int J Exer Sci 2020;7: 312.
- González-BadilloJJ, Sánchez-MedinaL. Movement velocity as a measure of loading intensity in resistance training. Int J Sports Med. 2010;31(05):347–352.
- Sánchez-MedinaL, González-BadilloJJ. Velocity loss as an indicator of neuromuscular fatigue during resistance training. Med Sci Sports Exerc. 2011;43(9):1725–1734.
- OpheimMN, RankinJW. Effect of capsaicin supplementation on repeated sprinting performance. J Strength Cond Conditioning Res. 2012;26(2):319–326.
- EFSA Panel on Nutrition, Novel Foods and Food Allergens (EFSA NDA Panel), Turck D, Castenmiller J, Safety of phenylcapsaicin as a novel food pursuant to regulation (EU) 2015/2283.EFSA J.InternetAvailable from2019:[cited 2022 Sep 14]17.10.2903/j.efsa.2019.57186.
- IzquierdoM, Lez-IzalMG, Navarro-AmezquetaI, et al. Effects of strength training on muscle fatigue mapping from surface EMG and blood metabolites. Appl Sci. 2010;43(2):303–311. DOI:10.1249/MSS.0b013e3181edfa96
- PalS, ChakiB, ChattopadhyayS, et al. High-intensity exercise induced oxidative stress and skeletal muscle damage in postpubertal boys and girls: a comparative study. J Strength Cond Conditioning Res. 2018;32(4):1045–1052.
- PyneDB, BostonT, MartinDT, et al. Evaluation of the lactate pro blood lactate analyser. Eur J Appl Physiol. 2000;82(1–2):112–116.
- Mora-CustodioR, Rodríguez-RosellD, Yáñez-GarcíaJM, et al. Effect of different inter-repetition rest intervals across four load intensities on velocity loss and blood lactate concentration during full squat exercise. J Sports Sci. 2018;36(24):10.
- CattozzoG, FranziniC, HubbuchA, et al. Evaluation of determination of uric acid in serum and whole blood with the reflotron. Clin Chem. 1988;34(2):414–416.
- PearsonJR, DusenburyLJ, Bakes-MartinR, et al. Evaluation of a simple method for measuring blood cholesterol levels using non-laboratory observers. Am J Med. 1988;85(3):369–374.
- WarburtonDER, WelshRC, HaykowskyMJ. Biochemical changes as a result of prolonged strenuous exercise. Br J Sports Med. 2002;36(4):301–303.
- González-BadilloJJ, Yañez-GarcíaJM, Mora-CustodioR, et al. Velocity loss as a variable for monitoring resistance exercise. Int J Sports Med. 2017;38(03):217–225.
- Piqueras-SanchizF, Cornejo-DazaPJ, Sánchez-ValdepeñasJ, et al. Acute Mechanical, Neuromuscular, and Metabolic Responses to Different Set Configurations in Resistance Training. J Strength Cond Conditioning Res. 2021;36(11):2983–2991. Internet. [cited 2022 Sep 18];Publish Ahead of Print. Available from. https://journals.lww.com/10.1519/JSC.0000000000004068
- Pareja-BlancoF, AlcazarJ, Sánchez-ValdepeñasJ, et al. Velocity loss as a critical variable determining the adaptations to strength training. Med Sci Sports Exerc. 2020;52(8):1752–1762. DOI:10.1249/MSS.0000000000002295
- González-BadilloJ, Rodríguez-RosellD, Sánchez-MedinaL, et al. Short-term recovery following resistance exercise leading or not to failure. Int J Sports Med. 2015;37(04):295–304. DOI:10.1055/s-0035-1564254
- LaurentCM, GreenJM, BishopPA, et al. A practical approach to monitoring recovery: development of a perceived recovery status scale. Journal of Strength and Conditioning Research. 2010;25(3):620–628.
- ColadoJC, PedrosaFM, JuesasA, et al. Concurrent validation of the OMNI-Resistance exercise scale of perceived exertion with elastic bands in the elderly. Exp Gerontol. 2018;103:11–16.
- SwankA, RobertsonRJ. Effect of induced alkalosis on perception of exertion during intermittent exercise. J Appl Physiol. 1989;67(5):6.
- de MortonNA. The PEDro scale is a valid measure of the methodological quality of clinical trials: a demographic study. Aust J Physiother. 2009;55(2):129–133.
- Sánchez-MorenoM, Cornejo-DazaPJ, González-BadilloJJ, et al. Effects of velocity loss during body mass prone-grip pull-up training on strength and endurance performance. J Strength Cond Conditioning Res. 2020;34(4):911–917.
- CohenJ. Statistical power analysis for the behavioral sciences. 2nd ed. Hillsdale, N.J: Lawrence Erlbaum Associates; 1988.
- WirtzN, WahlP, KleinöderH, et al. Lactate kinetics during multiple set resistance exercise. Journal of sports science & medicine. 2014;13(1):73–77.
- de la RocheJ, WaltherI, LeonowW, et al. Lactate is a potent inhibitor of the capsaicin receptor TRPV1. Sci Rep. 2016;6(1):36740. DOI:10.1038/srep36740
- de FreitasMC, CholewaJM, GobboLA, et al. Acute capsaicin supplementation improves 1,500-m running time-trial performance and rate of perceived exertion in physically active adults. J Strength Cond Conditioning Res. 2018;32(2):572–577.
- SuttonJR, ToewsCJ, WardGR, et al. Purine metabolism during strenuous muscular exercise in man. Metabolism. 1980;29(3):254–260.
- BairdMF, GrahamSM, BakerJS, et al. Creatine-kinase- and exercise-related muscle damage implications for muscle performance and recovery. J Nutr Metab. 2012;2012:1–13.
- BessaAL, OliveiraVN, AgostiniGG, et al. Exercise intensity and recovery biomarkers of injury. Inflamm Oxidative Stress. 2016;30(2):311–319. DOI:10.1519/JSC.0b013e31828f1ee9
- Villavicencio KimJ, WuGY. Body building and aminotransferase elevations: a review. J Clin Transl Hepatol. 2020;8(2):161–167.
- Morán-NavarroR, Martínez-CavaA, Sánchez-MedinaL, et al. Movement velocity as a measure of level of effort during resistance exercise. J Strength Cond Conditioning Res. 2019;33(6):1496–1504.
- de MouraE, SilvaVEL, CholewaJM, et al. Chronic capsiate supplementation increases fat-free mass and upper body strength but not the inflammatory response to resistance exercise in young untrained men: a randomized, placebo-controlled and double-blind study. J Int Soc Sports Nutr. 2021;18(1):50. DOI:10.1186/s12970-021-00446-0
- KaufmanMP, IwamotoGA, LonghurstJC, et al. Effects of capsaicin and bradykinin on afferent fibers with ending in skeletal muscle. Circ Res. 1982;50(1):133–139.
- Del VecchioA, CasoloA, NegroF, et al. The increase in muscle force after 4 weeks of strength training is mediated by adaptations in motor unit recruitment and rate coding. J Physiol. 2019;597(7):1873–1887. DOI:10.1113/JP277250
- HeckmanCJ, EnokaRM.Physiology of the motor neuron and the motor unit.Handb Clin Neurophysiol.InternetAvailable fromElsevier.2004:cited 2021 Nov 17119–147https://linkinghub.elsevier.com/retrieve/pii/S1567423104040067.
- Alix-FagesC, García-RamosA, Calderón-NadalG, et al. Anodal transcranial direct current stimulation enhances strength training volume but not the force–velocity profile. Eur J Appl Physiol. 2020;120(8):1881–1891. DOI:10.1007/s00421-020-04417-2
- OkanoAH, FontesEB, MontenegroRA, et al. Brain stimulation modulates the autonomic nervous system, rating of perceived exertion and performance during maximal exercise. Br J Sports Med. 2015;49(18):1213–1218. DOI:10.1136/bjsports-2012-091658
- AroraV, CampbellJN, ChungM-K. Fight fire with fire: neurobiology of capsaicin-induced analgesia for chronic pain. Pharmacol Ther. 2021;220:107743.